- 1Division of Endocrinology, Diabetes and Metabolism, Department of Pediatrics, Medical College of Wisconsin, Milwaukee, WI, United States
- 2Division of Adolescent Medicine, Department of Pediatrics, Medical College of Wisconsin Affiliated Hospitals, Milwaukee, WI, United States
- 3Division of Adolescent Medicine, Department of Pediatrics, Medical College of Wisconsin, Milwaukee, WI, United States
Obesity is a complex condition that interweaves biological, developmental, environmental, behavioral, and genetic factors; it is a significant public health problem. The most common cause of obesity throughout childhood and adolescence is an inequity in energy balance; that is, excess caloric intake without appropriate caloric expenditure. Adiposity rebound (AR) in early childhood is a risk factor for obesity in adolescence and adulthood. The increasing prevalence of childhood and adolescent obesity is associated with a rise in comorbidities previously identified in the adult population, such as Type 2 Diabetes Mellitus, Hypertension, Non-alcoholic Fatty Liver disease (NAFLD), Obstructive Sleep Apnea (OSA), and Dyslipidemia. Due to the lack of a single treatment option to address obesity, clinicians have generally relied on counseling dietary changes and exercise. Due to psychosocial issues that may accompany adolescence regarding body habitus, this approach can have negative results. Teens can develop unhealthy eating habits that result in Bulimia Nervosa (BN), Binge- Eating Disorder (BED), or Night eating syndrome (NES). Others can develop Anorexia Nervosa (AN) as they attempt to restrict their diet and overshoot their goal of “being healthy.” To date, lifestyle interventions have shown only modest effects on weight loss. Emerging findings from basic science as well as interventional drug trials utilizing GLP-1 agonists have demonstrated success in effective weight loss in obese adults, adolescents, and pediatric patients. However, there is limited data on the efficacy and safety of other weight-loss medications in children and adolescents. Nearly 6% of adolescents in the United States are severely obese and bariatric surgery as a treatment consideration will be discussed. In summary, this paper will overview the pathophysiology, clinical, and psychological implications, and treatment options available for obese pediatric and adolescent patients.
Introduction
Obesity is a complex issue that affects children across all age groups (1–3). One-third of children and adolescents in the United States are classified as either overweight or obese. There is no single element causing this epidemic, but obesity is due to complex interactions between biological, developmental, behavioral, genetic, and environmental factors (4). The role of epigenetics and the gut microbiome, as well as intrauterine and intergenerational effects, have recently emerged as contributing factors to the obesity epidemic (5, 6). Other factors including small for gestational age (SGA) status at birth, formula rather than breast feeding in infancy, and early introduction of protein in infant's dietary intake have been reportedly associated with weight gain that can persist later in life (6–8). The rising prevalence of childhood obesity poses a significant public health challenge by increasing the burden of chronic non-communicable diseases (1, 9).
Obesity increases the risk of developing early puberty in children (10), menstrual irregularities in adolescent girls (1, 11), sleep disorders such as obstructive sleep apnea (OSA) (1, 12), cardiovascular risk factors that include Prediabetes, Type 2 Diabetes, High Cholesterol levels, Hypertension, NAFLD, and Metabolic syndrome (1, 2). Additionally, obese children and adolescents can suffer from psychological issues such as depression, anxiety, poor self-esteem, body image and peer relationships, and eating disorders (13, 14).
So far, interventions for overweight/obesity prevention have mainly focused on behavioral changes in an individual such as increasing daily physical exercise or improving quality of diet with restricting excess calorie intake (1, 15, 16). However, these efforts have had limited results. In addition to behavioral and dietary recommendations, changes in the community-based environment such as promotion of healthy food choices by taxing unhealthy foods (17), improving lunch food quality and increasing daily physical activity at school and childcare centers, are extra measures that are needed (16). These interventions may include a ban on unhealthy food advertisements aimed at children as well as access to playgrounds and green spaces where families can feel their children can safely recreate. Also, this will limit screen time for adolescents as well as younger children.
However, even with the above changes, pharmacotherapy and/or bariatric surgery will likely remain a necessary option for those youth with morbid obesity (1). This review summarizes our current understanding of the factors associated with obesity, the physiological and psychological effects of obesity on children and adolescents, and intervention strategies that may prevent future concomitant issues.
Definition of Childhood Obesity
Body mass index (BMI) is an inexpensive method to assess body fat and is derived from a formula derived from height and weight in children over 2 years of age (1, 18, 19). Although more sophisticated methods exist that can determine body fat directly, they are costly and not readily available. These methods include measuring skinfold thickness with a caliper, Bioelectrical impedance, Hydro densitometry, Dual-energy X-ray Absorptiometry (DEXA), and Air Displacement Plethysmography (2).
BMI provides a reasonable estimate of body fat indirectly in the healthy pediatric population and studies have shown that BMI correlates with body fat and future health risks (18). Unlike in adults, Z-scores or percentiles are used to represent BMI in children and vary with the age and sex of the child. BMI Z-score cut off points of >1.0, >2.0, and >3.0 are recommended by the World Health Organization (WHO) to define at risk of overweight, overweight and obesity, respectively (19). However, in terms of percentiles, overweight is applied when BMI is >85th percentile <95th percentile, whereas obesity is BMI > 95th percentile (20–22). Although BMI Z-scores can be converted to BMI percentiles, the percentiles need to be rounded and can misclassify some normal-weight children in the under or overweight category (19). Therefore, to prevent these inaccuracies and for easier understanding, it is recommended that the BMI Z-scores in children should be used in research whereas BMI percentiles are best used in the clinical settings (20).
As BMI does not directly measure body fat, it is an excellent screening method, but should not be used solely for diagnostic purposes (23). Using 85th percentile as a cut off point for healthy weight may miss an opportunity to obtain crucial information on diet, physical activity, and family history. Once this information is obtained, it may allow the provider an opportunity to offer appropriate anticipatory guidance to the families.
Pathophysiology of Obesity
The pathophysiology of obesity is complex that results from a combination of individual and societal factors. At the individual level, biological, and physiological factors in the presence of ones' own genetic risk influence eating behaviors and tendency to gain weight (1). Societal factors include influence of the family, community and socio-economic resources that further shape these behaviors (Figure 1) (3, 24).
Biological Factors
There is a complex architecture of neural and hormonal regulatory control, the Gut-Brain axis, which plays a significant role in hunger and satiety (Figure 2). Sensory stimulation (smell, sight, and taste), gastrointestinal signals (peptides, neural signals), and circulating hormones further contribute to food intake (25–27).
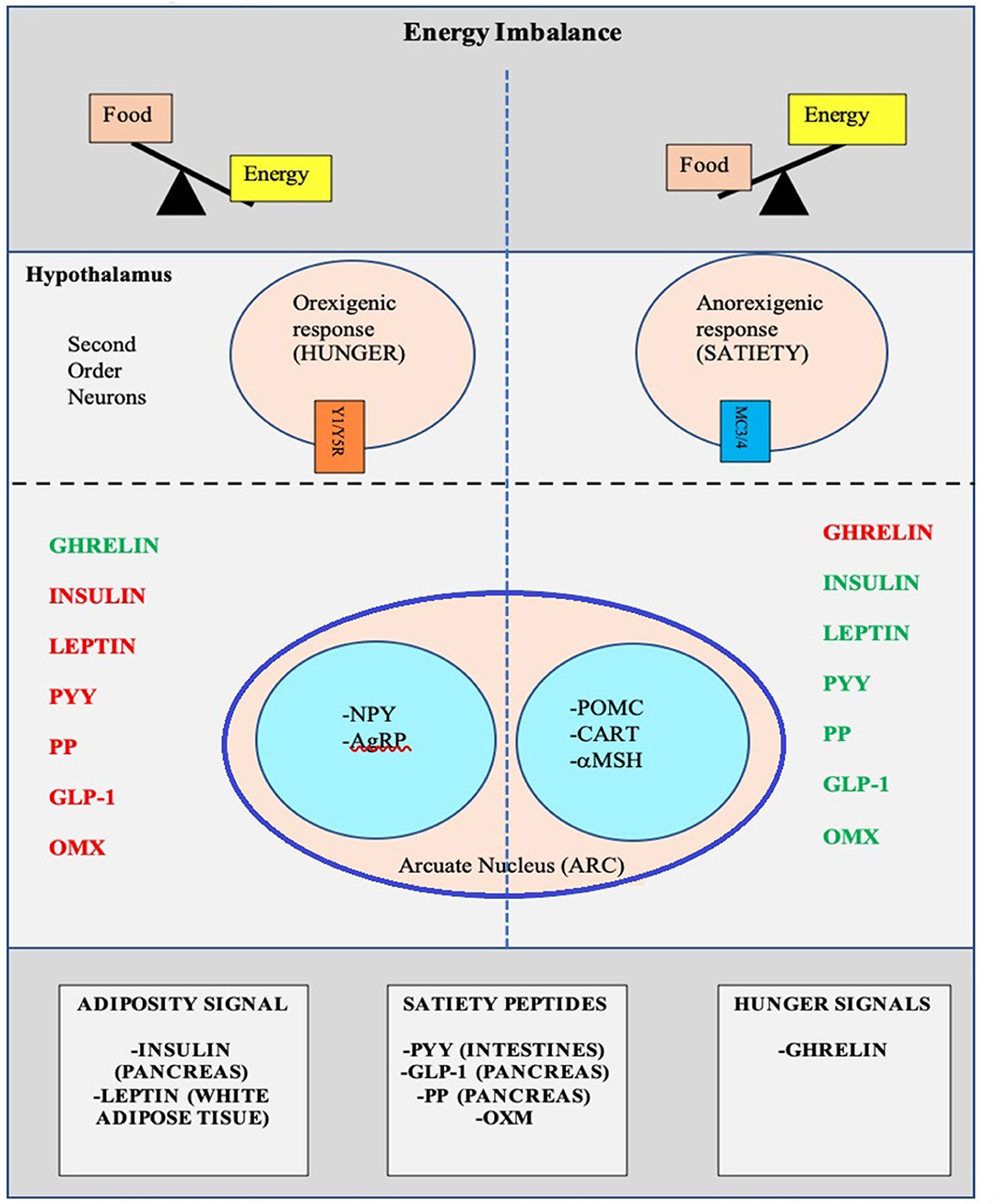
Figure 2. Pictorial representation of the Hunger-Satiety pathwaya and the various hormonesb involved in the pathway. a, Y1/Y5R and MC3/4 are second order neuro receptors which are responsible in either the hunger or satiety pathway. Neurons in the ARC include: NPY, Neuropeptide Y; AgRP, Agouti-Related Peptide; POMC, Pro-Opiomelanocortin; CART, Cocaine-and Amphetamine-regulated Transcript; α-MSH, α-Melanocyte Stimulating Hormone. b, PYY, Peptide YY; PP, Pancreatic Polypeptide; GLP-1, Glucagon-Like Peptide- I; OMX, Oxyntomodulin.
The hypothalamus is the crucial region in the brain that regulates appetite and is controlled by key hormones. Ghrelin, a hunger-stimulating (orexigenic) hormone, is mainly released from the stomach. On the other hand, leptin is primarily secreted from adipose tissue and serves as a signal for the brain regarding the body's energy stores and functions as an appetite -suppressing (anorexigenic) hormone. Several other appetite-suppressing (anorexigenic) hormones are released from the pancreas and gut in response to food intake and reach the hypothalamus through the brain-blood barrier (BBB) (28–32). These anorexigenic and orexigenic hormones regulate energy balance by stimulating hunger and satiety by expression of various signaling pathways in the arcuate nucleus (ARC) of the hypothalamus (Figure 2) (28, 33). Dysregulation of appetite due to blunted suppression or loss of caloric sensing signals can result in obesity and its morbidities (34).
Emotional dysfunction due to psychiatric disorders can cause stress and an abnormal sleep-wake cycles. These modifications in biological rhythms can result in increased appetite, mainly due to ghrelin, and can contribute to emotional eating (35).
Recently, the role of changes in the gut microbiome with increased weight gain through several pathways has been described in literature (36, 37). The human gut serves as a host to trillions of microorganisms, referred to as gut microbiota. The dominant gut microbial phyla are Firmicutes, Bacteroidetes, Actinobacteria, Proteobacteria, Fusobacteria, and Verrucomicrobia, with Firmicutes and Bacteroidetes representing 90% of human gut microbiota (5, 38). The microbes in the gut have a symbiotic relationship within their human host and provide a nutrient-rich environment. Gut microbiota can be affected by various factors that include gestational age at birth, mode of infant delivery, type of neonatal and infant feeding, introduction of solid food, feeding practices and external factors like antibiotic use (5, 38). Also, the maturation of the bacterial phyla that occurs from birth to adulthood (39), is influenced by genetics, environment, diet, lifestyle, and gut physiology and stabilizes in adulthood (5, 39, 40). Gut microbiota is unique to each individual and plays a specific role in maintaining structural integrity, and the mucosal barrier of the gut, nutrient metabolism, immune response, and protection against pathogens (5, 37, 38). In addition, the microbiota ferments the indigestible food and synthesizes other essential micronutrients as well as short chain fatty acids (SCFAs') (40, 41). Dysbiosis or imbalance of the gut microbiota, in particularly the role of SCFA has been linked with the patho-physiology of obesity (36, 38, 41, 42). SCFAs' are produced by anaerobic fermentation of dietary fiber and indigestible starch and play a role in mammalian energy metabolism by influencing gut-brain communication axis. Emerging evidence has shown that increased ratio of Firmicutes to Bacteroidetes causes increased energy extraction of calories from diets and is evidenced by increased production of short chain fatty acids (SCFAs') (43–45). However, this relationship is not affirmed yet, as a negative relationship between SCFA levels and obesity has also been reported (46). Due to the conflicting data, additional randomized control trials are needed to clarify the role of SCFA's in obese and non-obese individuals.
The gut microbiota also has a bidirectional interaction with the liver, and various additional factors such as diet, genetics, and the environment play a key role in this relationship. The Gut- Liver Axis is interconnected at various levels that include the mucus barrier, epithelial barrier, and gut microbiome and are essential to maintain normal homeostasis (47). Increased intestinal mucosal permeability can disrupt the gut-liver axis, which releases various inflammatory markers, activates an innate immune response in the liver, and results in a spectrum of liver diseases that include hepatic steatosis, non-alcoholic steatohepatitis (NASH), cirrhosis, and hepatocellular carcinoma (HCC) (48, 49).
Other medical conditions, including type 2 Diabetes Mellitus, Metabolic Syndrome, eating disorders as well as psychological conditions such as anxiety and depression are associated with the gut microbiome (50–53).
Genetic Factors
Genetic causes of obesity can either be monogenic or polygenic types. Monogenic obesity is rare, mainly due to mutations in genes within the leptin/melanocortin pathway in the hypothalamus that is essential for the regulation of food intake/satiety, body weight, and energy metabolism (54). Leptin regulates eating behaviors, the onset of puberty, and T-cell immunity (55). About 3% of obese children have mutations in the leptin (LEP) gene and the leptin receptor (LEPR) and can also present with delayed puberty and immune dysfunction (55, 56). Obesity caused by other genetic mutations in the leptin-melanocortin pathway include proopiomelanocortin (POMC) and melanocortin receptor 4 (MC4R), brain-derived neurotrophic factor (BDNF), and the tyrosine kinase receptor B (NTRK2) genes (57, 58). Patients with monogenic forms generally present during early childhood (by 2 years old) with severe obesity and abnormal feeding behaviors (59). Other genetic causes of severe obesity are Prader Willi Syndrome (PWS), Alström syndrome, Bardet Biedl syndrome. Patients with these syndromes present with additional characteristics, including cognitive impairment, dysmorphic features, and organ-specific developmental abnormalities (60). Individuals who present with obesity, developmental delay, dysmorphic features, and organ dysfunction should receive a genetics referral for further evaluation.
Polygenic obesity is the more common form of obesity, caused by the combined effect of multiple genetic variants. It is the result of the interplay between genetic susceptibility and the environment, also known as the Gene-Environment Interaction (GEI) (61–64). Genome-wide association studies (GWAS) have identified gene variants [single nucleotide polymorphism (SNPs)] for body mass index (BMI) that likely act synergistically to affect body weight (65). Studies have identified genetic variants in several genes that may contribute to excessive weight gain by increasing hunger and food intake (66–68). When the genotype of an individual confers risk for obesity, exposure to an obesogenic environment may promote a state of energy imbalance due to behaviors that contribute to conserving rather than expending energy (69, 70). Research studies have shown that obese individuals have a genetic variation that can influence their actions, such as increased food intake, lack of physical activity, a decreased metabolism, as well as an increased tendency to store body fat (63, 66, 67, 69, 70).
Recently the role of epigenetic factors in the development of obesity has emerged (71). The epigenetic phenomenon may alter gene expression without changing the underlying DNA sequence. In effect, epigenetic changes may result in the addition of chemical tags known as methyl groups, to the individual's chromosomes. This alteration can result in a phenomenon where critical genes are primed to on and off regulate. Complex physiological and psychological adjustment occur during infancy and can thereafter set the stage for health vs. disease. Developmental origins of health and disease (DOHaD) shows that early life environment can impact the risk of chronic diseases later in life due to fetal programming secondary to epigenetic changes (72). Maternal nutrition during the prenatal or early postnatal period may trigger these epigenetic changes and increase the risk for chronic conditions such as obesity, metabolic and cardiovascular disease due to epigenetic modifications that may persist and cause intergenerational effect on the health children and adults (58, 73, 74). Similarly, adverse childhood experiences (ACE) have been linked to a broad range of negative outcomes through epigenetic mechanisms (75) and promote unhealthy eating behaviors (76, 77). Other factors such as diet, physical activity, environmental and psychosocial stressors can cause epigenetic changes and place an individual at risk for weight gain (78).
Developmental Factors
Eating behaviors evolve over the first few years of life. Young children learn to eat through their direct experience with food and observing others eating around them (79). During infancy, feeding defines the relationship of security and trust between a child and the parent. Early childhood eating behaviors shift to more self-directed control due to rapid physical, cognitive, communicative, and social development (80). Parents or caregivers determine the type of food that is made available to the infant and young child. However, due to economic limitations and parents having decreased time to prepare nutritious meals, consumption of processed and cheaper energy-dense foods have occurred in Western countries. Additionally, feeding practices often include providing large or super-sized portions of palatable foods and encouraging children to finish the complete meal (clean their plate even if they do not choose to), as seen across many cultures (81, 82). Also, a segment of parents are overly concerned with dietary intake and may pressurize their child to eat what they perceive as a healthy diet, which can lead to unintended consequences (83). Parents' excessive restriction of food choices may result in poor self-regulation of energy intake by their child or adolescent. This action may inadvertently promote overconsumption of highly palatable restricted foods when available to the child or adolescent outside of parental control with resultant excessive weight gain (84, 85).
During middle childhood, children start achieving greater independence, experience broader social networks, and expand their ability to develop more control over their food choices. Changes that occur in the setting of a new environment such as daycare or school allow exposure to different food options, limited physical activity, and often increased sedentary behaviors associated with school schedules (24). As the transition to adolescence occurs, physical and psychosocial development significantly affect food choices and eating patterns (25). During the teenage years, more independence and interaction with peers can impact the selection of fast foods that are calorically dense. Moreover, during the adolescent years, more sedentary behaviors such as video and computer use can limit physical exercise. Adolescence is also a period in development with an enhanced focus on appearance, body weight, and other psychological concerns (86, 87).
Environmental Factors
Environmental changes within the past few decades, particularly easy access to high-calorie fast foods, increased consumption of sugary beverages, and sedentary lifestyles, are linked with rising obesity (88). The easy availability of high caloric fast foods, and super-sized portions, are increasingly common choices as individuals prefer these highly palatable and often less expensive foods over fruits and vegetables (89). The quality of lunches and snacks served in schools and childcare centers has been an area of debate and concern. Children and adolescents consume one-third to one-half of meals in the above settings. Despite policies in place at schools, encouraging foods, beverages, and snacks that are deemed healthier options, the effectiveness of these policies in improving children's dietary habits or change in obesity rate has not yet been seen (90). This is likely due to the fact that such policies primarily focus on improving dietary quality but not quantity which can impact the overweight or obese youth (91). Policies to implement taxes on sugary beverages are in effect in a few states in the US (92) as sugar and sugary beverages are associated with increased weight gain (2, 3). This has resulted in reduction in sales of sugary drinks in these states, but the sales of these types of drinks has risen in neighboring states that did not implement the tax (93). Due to advancements in technology, children are spending increased time on electronic devices, limiting exercise options. Technology advancement is also disrupting the sleep-wake cycle, causing poor sleeping habits, and altered eating patterns (94). A study published on Canadian children showed that the access to and night-time use of electronic devices causes decreased sleep duration, resulting in excess body weight, inferior diet quality, and lower physical activity levels (95).
Infant nutrition has gained significant popularity in relation to causing overweight/obesity and other diseases later in life. Breast feeding is frequently discussed as providing protection against developing overweight/obesity in children (8). Considerable heterogeneity has been observed in studies and conducting randomized clinical trials between breast feeding vs. formula feeding is not feasible (8). Children fed with a low protein formula like breast milk are shown to have normal weight gain in early childhood as compared to those that are fed formulas with a high protein load (96). A recent Canadian childbirth cohort study showed that breast feeding within first year of life was inversely associated with weight gain and increased BMI (97). The effect was stronger if the child was exclusively breast fed directly vs. expressed breast milk or addition of formula or solid food (97). Also, due to the concern of poor growth in preterm or SGA infants, additional calories are often given for nutritional support in the form of macronutrient supplements. Most of these infants demonstrate “catch up growth.” In fact, there have been reports that in some children the extra nutritional support can increase the risk for overweight/obesity later in life. The association, however, is inconsistent. Recently a systemic review done on randomized controlled trials comparing the studies done in preterm and SGA infants with feeds with and without macronutrient supplements showed that macronutrient supplements may increase weight and length in toddlers but did not show a significant increase in the BMI during childhood (98). Increased growth velocity due to early introduction of formula milk and protein in infants' diet, may influence the obesity pathways, and can impact fetal programming for metabolic disease later in life (99).
General pediatricians caring for children with overweight/obesity, generally recommend endocrine testing as parents often believe that there may be an underlying cause for this condition and urge their primary providers to check for conditions such as thyroid abnormalities. Endocrine etiologies for obesity are rarely identified and patients with underlying endocrine disorders causing excessive weight gain usually are accompanied by attenuated growth patterns, such that a patient continues to gain weight with a decline in linear height (100). Various endocrine etiologies that one could consider in a patient with excessive weight gain in the setting of slow linear growth: severe hypothyroidism, growth hormone deficiency, and Cushing's disease/syndrome (58, 100).
Clinical-Physiology of Pediatric Obesity
It is a well-known fact that early AR(increased BMI) before the age of 5 years is a risk factor for adult obesity, obesity-related comorbidities, and metabolic syndrome (101–103). Typically, body mass index (BMI) declines to a minimum in children before it starts increasing again into adulthood, also known as AR. Usually, AR happens between 5 and 7 years of age, but if it occurs before the age of 5 years is considered early AR. Early AR is a marker for higher risk for obesity-related comorbidities. These obesity-related health comorbidities include cardiovascular risk factors (hypertension, dyslipidemia, prediabetes, and type 2 diabetes), hormonal issues, orthopedic problems, sleep apnea, asthma, and fatty liver disease (Figure 3) (9).
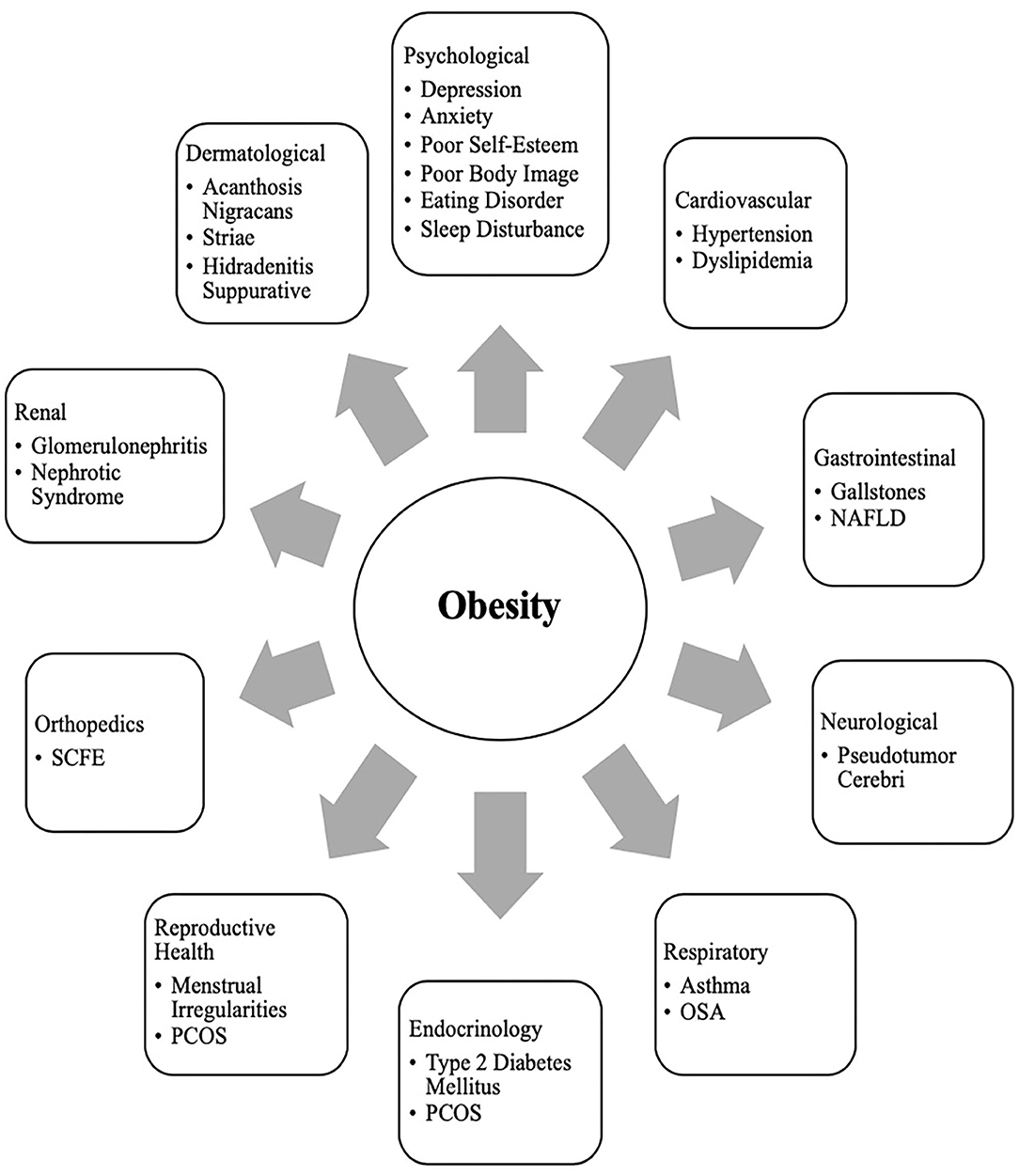
Figure 3. Obesity related co-morbiditiesa in children and adolescents. a, NAFLD, Non-Alcoholic Fatty Liver Disease; SCFE, Slipped Capital Femoral Epiphysis; PCOS, Polycystic Ovary Syndrome; OSA, Obstructive Sleep Apnea.
Clinical Comorbidities of Obesity in Children
Growth and Puberty
Excess weight gain in children can influence growth and pubertal development (10). Childhood obesity can cause prepubertal acceleration of linear growth velocity and advanced bone age in boys and girls (104). Hyperinsulinemia is a normal physiological state during puberty, but children with obesity can have abnormally high insulin levels (105). Leptin resistance also occurs in obese individuals who have higher leptin levels produced by their adipose tissue (55, 106). The insulin and leptin levels can act on receptors that impact the growth plates with a resultant bone age advancement (55).
Adequate nutrition is essential for the typical timing and tempo of pubertal onset. Excessive weight gain can initiate early puberty, due to altered hormonal parameters (10). Obese children may present with premature adrenarche, thelarche, or precocious puberty (PP) (107). The association of early pubertal changes with obesity is consistent in girls, and is well-reported; however, data is sparse in boys (108). One US study conducted in racially diverse boys showed obese boys had delayed puberty, whereas overweight boys had early puberty as compared to normal-weight boys (109). Obese girls with PP have high leptin levels (110, 111). Healthy Lifestyle in Europe by Nutrition in Adolescence (HELENA) is a cross-sectional study and suggested an indirect relationship between elevated leptin levels, early puberty, and cardiometabolic and inflammatory markers in obese girls (112). Additionally, obese girls with premature adrenarche carry a higher risk for developing polycystic ovary syndrome (PCOS) in the future (113, 114).
Sleep Disorders
Obesity is an independent risk factor for obstructive sleep apnea (OSA) in children and adolescents (12, 115). Children with OSA have less deleterious consequences in terms of cardiovascular stress of metabolic syndrome when compared to adolescents and adults (116, 117). In children, abnormal behaviors and neurocognitive dysfunction are the most critical and frequent end-organ morbidities associated with OSA (12). However, in adolescents, obesity and OSA can independently cause oxidative systemic stress and inflammation (118, 119), and when this occurs concurrently, it can result in more severe metabolic dysfunction and cardiovascular outcomes later in life (120).
Other Comorbidities
Obesity is related to a clinical spectrum of liver abnormalities such as NAFLD (121); the most important cause of liver disease in children (122–124). NAFLD includes steatosis (increased liver fat without inflammation) and NASH (increased liver fat with inflammation and hepatic injury). While in some adults NAFLD can progress to an end-stage liver disease requiring liver transplant (125, 126), the risk of progression during childhood is less well-defined (127). NAFLD is closely associated with metabolic syndrome including central obesity, insulin resistance, type 2 diabetes, dyslipidemia, and hypertension (128).
Obese children are also at risk for slipped capital femoral epiphysis (SCFE) (129), and sedentary lifestyle behaviors may have a negative influence on the brain structure and executive functioning, although the direction of causality is not clear (130, 131).
Clinical Comorbidities of Obesity in Adolescents
Menstrual Irregularities and PCOS
At the onset of puberty, physiologically, sex steroids can cause appropriate weight gain and body composition changes that should not affect normal menstruation (132, 133). However, excessive weight gain in adolescent girls can result in irregular menstrual cycles and puts them at risk for PCOS due to increased androgen levels. Additionally, they can have excessive body hair (hirsutism), polycystic ovaries, and can suffer from distorted body images (134, 135). Adolescent girls with PCOS also have an inherent risk for insulin resistance irrespective of their weight. However, weight gain further exacerbates their existing state of insulin resistance and increases the risk for obesity-related comorbidities such as metabolic syndrome, and type 2 diabetes. Although the diagnosis of PCOS can be challenging at this age due to an overlap with predictable pubertal changes, early intervention (appropriate weight loss and use of hormonal methods) can help restore menstrual cyclicity and future concerns related to childbearing (11).
Metabolic Syndrome and Sleep Disorders
Metabolic syndrome (MS) is a group of cardiovascular risk factors characterized by acanthosis nigricans, prediabetes, hypertension, dyslipidemia, and non-alcoholic steatohepatitis (NASH), that occurs from insulin resistance caused by obesity (136). Diagnosis of MS in adults requires at least three out of the five risk factors: increased central adiposity, hypertension, hyperglycemia, hypertriglyceridemia, or low HDL level. Definitions to diagnose MS are controversial in younger age groups, and many definitions have been proposed (136). This is due to the complex physiology of growth and development during puberty, which causes significant overlap between MS and features of normal growth. However, childhood obesity is associated with an inflammatory state even before puberty (137). In obese children and adolescents, hyperinsulinemia during puberty (138, 139) and unhealthy sleep behaviors increase MS's risk and severity (140). Even though there is no consensus on diagnosis regarding MS in this age group, when dealing with obese children and adolescents, clinicians should screen them for MS risk factors and sleep behaviors and provide recommendations for weight management.
Social Psychology of Pediatric Obesity in Children and Adolescents
Obese children and adolescents may experience psychosocial sequelae, including depression, bullying, social isolation, diminished self-esteem, behavioral problems, dissatisfaction with body image, and reduced quality of life (13, 141). Compared with normal-weight counterparts, overweight/obesity is one of the most common reasons children and adolescents are bullied at school (142). The consequence of stigma, bullying, and teasing related to childhood obesity are pervasive and can have severe implications for emotional and physical health and performance that can persist later in life (13).
In adolescents, psychological outcomes associated with obesity are multifactorial and have a bidirectional relationship (Figure 4). Obese adolescents due to their physique may have a higher likelihood of psychosocial health issues, including depression, body image/dissatisfaction, lower self-esteem, peer victimization/bullying, and interpersonal relationship difficulties. They may also demonstrate reduced resilience to challenging situations compared to their non-obese/overweight counterparts (9, 143–146). Body image dissatisfaction has been associated with further weight gain but can also be related to the development of a mental health disorder or an eating disorder (ED) or disorder eating habits (DEH). Mental health disorders such as depression are associated with poor eating habits, a sedentary lifestyle, and altered sleep patterns. ED or DEH that include anorexia nervosa (AN), bulimia nervosa (BN), binge-eating disorder (BED) or night eating syndrome (NES) may be related to an individual's overvaluation of their body shape and weight or can result during the treatment for obesity (147–150). The management of obesity can place a patient at risk of AN if there is a rigid focus on caloric intake or if a patient overcorrects and initiates obsessive self-directed dieting. Healthcare providers who primarily care for obese patients, usually give the advice to diet to lose weight and then maintain it. However, strict dieting (hypocaloric diet), which some patients may later engage in can lead to an eating disorder such as anorexia nervosa (151). This behavior leads to a poor relationship with food, and therefore, adolescents perseverate on their weight and numbers (152).
Providers may not recognize DEHs when a morbidly obese patient loses the same weight as a healthy weight individual (149). It may appear as a positive result with families and others praising the individual without realizing that this youth may be engaging in destructive behaviors related to weight control. Therefore, it is essential to screen regarding the process of how weight loss was achieved (144, 150).
Support and attention to underlying psychological concerns can positively affect treatment, overall well-being, and reduce the risk of adult obesity (150). The diagram above represents the complexity of the different psychological issues which can impact the clinical care of the obese adolescent.
Eating family meals together can improve overall dietary intake due to enhanced food choices mirrored by parents. It has also may serve as a support to individuals with DEHs if there is less attention to weight and a greater focus on appropriate, sustainable eating habits (148).
Treatment
Prevention and Anticipatory Guidance
It is essential to recognize and provide preventive measures for obesity during early childhood and adolescence (100, 153, 154). It is well-established that early AR is a risk factor for adult obesity (66–68). Therefore, health care providers caring for the pediatric population need to focus on measures such as BMI but provide anticipatory guidance regarding nutritional counseling without stigmatizing or judging parents for their children's overweight/obesity (155). Although health care providers continue to pursue effective strategies to address the obesity epidemic; ironically, they frequently exhibit weight bias and stigmatizing behaviors. Research has demonstrated that the language that health care providers use when discussing a patient's body weight can reinforce stigma, reduce motivation for weight loss, and potentially cause avoidance of routine preventive care (155). In adolescents, rather than motivating positive changes, stigmatizing language regarding weight may negatively impact a teen and result in binge eating, decreased physical activity, social isolation, avoidance of health care services, and increased weight gain (156, 157). Effective provider-patient communication using motivational interviewing techniques are useful to encourage positive behavior changes (155, 158).
Anticipatory guidance includes educating the families on healthy eating habits and identifying unhealthy eating practices, encouraging increased activity, limiting sedentary activities such as screen time. Lifestyle behaviors in children and adolescents are influenced by many sectors of our society, including the family (Figure 1) (3, 24). Therefore, rather than treating obesity in isolation as an individual problem, it is crucial to approach this problem by focusing on the family unit. Family-based multi-component weight loss behavioral treatment is the gold standard for treating childhood obesity, and it is having been found useful in those between 2 and 6 years old (150, 159). Additionally, empowering the parents to play an equal role in developing and implementing an intervention for weight management has shown promising results in improving the rate of obesity by decreasing screen time, promoting healthy eating, and increasing support for children's physical activity (160, 161).
When dietary/lifestyle modifications have failed, the next option is a structured weight -management program with a multidisciplinary approach (15). The best outcomes are associated with an interdisciplinary team comprising a physician, dietician, and psychologist generally 1–2 times a week (15, 162). However, this treatment approach is not effective in patients with severe obesity (122). Although healthier lifestyle recommendations for weight loss are the current cornerstone for obesity management, they often fail. As clinicians can attest, these behavioral and dietary changes are hard to achieve, and all too often is not effective in patients with severe obesity. Failure to maintain substantial weight loss over the long term is due to poor adherence to the prescribed lifestyle changes as well as physiological responses that resist weight loss (163). American TV hosts a reality show called “The Biggest Loser” that centers on overweight and obese contestants attempting to lose weight for a cash prize. Contestants from “The Biggest Loser” competition, had metabolic adaptation (MA) after drastic weight loss, regained more than they lost weight after 6 years due to a significant slow resting metabolic rate (164). MA is a physiological response which is a reduced basal metabolic rate seen in individuals who are losing or have lost weight. In MA, the body alters how efficient it is at turning the food eaten into energy; it is a natural defense mechanism against starvation and is a response to caloric restriction. Plasma leptin levels decrease substantially during caloric restriction, suggesting a role of this hormone in the drop of energy expenditure (165).
Pharmacological Management
The role of pharmacological therapy in the treatment of obesity in children and adolescents is limited.
Orlistat is the only FDA approved medication for weight loss in 12-18-year-olds but has unpleasant side effects (166). Another medicine, Metformin, has been used in children with signs of insulin resistance, may have some impact on weight, but is not FDA approved (167). The combination of phentermine/topiramate (Qsymia) has been FDA approved for weight loss in obese individuals 18 years and older. In studies, there has been about 9–10% weight loss over 2 years. However, caution must be taken in females as it can lead to congenital disabilities, especially with use in the first trimester of pregnancy (167).
GLP-1 agonists have demonstrated great success in effective weight loss and are approved by the FDA for adult obesity (168–170). A randomized control clinical trial recently published showed a significant weight loss in those using liraglutide (3.0 mg)/day plus lifestyle therapy group compared to placebo plus lifestyle therapy in children between the ages of 12–18 years (171).
Recently during the EASL conference, academic researchers and industry partners presented novel interventions targeting different gut- liver axis levels that include intestinal content, intestinal microbiome, intestinal mucosa, and peritoneal cavity (47). The focus for these therapeutic interventions within the gut-liver axis was broad and ranged anywhere from newer drugs protecting the intestinal mucus lining, restoring the intestinal barriers and improvement in the gut microbiome. One of the treatment options was Hydrogel technology which was shown to be effective toward weight loss in patients with metabolic syndrome. Hydrogel technology include fibers and high viscosity polysaccharides that absorb water in the stomach and increasing the volume, thereby improving satiety (47). Also, a clinical trial done in obese pregnant mothers using Docosahexaenoic acid (DHA) showed that the mothers' who got DHA had children with lower adiposity at 2 and 4 years of age (172). Recently the role of probiotics in combating obesity has emerged. Probiotics are shown to alter the gut microbiome that improves intestinal digestive and absorptive functions of the nutrients. Intervention including probiotics may be a possible solution to manage pediatric obesity (173, 174). Additionally, the role of Vitamin E for treating the comorbidities of obesity such as diabetes, hyperlipidemia, NASH, and cardiovascular risk, has been recently described (175, 176). Vitamin E is a lipid- soluble compound and contains both tocopherols and tocotrienols. Tocopherols have lipid-soluble antioxidants properties that interact with cellular lipids and protects them from oxidation damage (177). In metabolic disease, certain crucial pathways are influenced by Vitamin E and some studies have summarized the role of Vitamin E regarding the treatment of obesity, metabolic, and cardiovascular disease (178). Hence, adequate supplementation of Vitamin E as an appropriate strategy to help in the treatment of the prevention of obesity and its associated comorbidities has been suggested. Nonetheless, some clinical trials have shown contradictory results with Vitamin E supplementation (177). Although Vitamin E has been recognized as an antioxidant that protects from oxidative damage, however, a full understanding of its mechanism of action is still lacking.
Bariatric Surgery
Bariatric surgery has gained popularity since the early 2000s in the management of severe obesity. If performed earlier, there are better outcomes for reducing weight and resolving obesity-related comorbidities in adults (179–182). Currently, the indication for bariatric in adolescents; those who have a BMI >35 with at least one severe comorbidity (Type 2 Diabetes, severe OSA, pseudotumor cerebri or severe steatohepatitis); or BMI of 40 or more with other comorbidities (hypertension, hyperlipidemia, mild OSA, insulin resistance or glucose intolerance or impaired quality of life due to weight). Before considering bariatric surgery, these patients must have completed most of their linear growth and participated in a structured weight-loss program for 6 months (159, 181, 183). The American Society for Metabolic and Bariatric Surgery (AMBS) outlines the multidisciplinary approach that must be taken before a patient undergoing bariatric surgery. In addition to a qualified bariatric surgeon, the patient must have a pediatrician or provider specialized in adolescent medicine, endocrinology, gastroenterology and nutrition, registered dietician, mental health provider, and exercise specialist (181). A mental health provider is essential as those with depression due to obesity or vice versa may have persistent mental health needs even after weight loss surgery (184).
Roux-en-Y Gastric Bypass (RYGB), laparoscopic Sleeve Gastrectomy (LSG), and Gastric Banding are the options available. RYGB and LSG currently approved for children under 18 years of age (166, 181, 185). At present, gastric banding is not an FDA recommended procedure in the US for those under 18y/o. One study showed some improvements in BMI and severity of comorbidities but had multiple repeat surgeries and did not believe a suitable option for obese adolescents (186).
Compared to LSG, RYGB has better outcomes for excess weight loss and resolution of obesity-related comorbidities as shown in studies and clinical trials (183, 184, 187). Overall, LSG is a safer choice and may be advocated for more often (179–181). The effect on the Gut-Brain axis after Bariatric surgery is still inconclusive, especially in adolescents, as the number of procedures performed is lower than in adults. Those who underwent RYGB had increased fasting and post-prandial PYY and GLP-1, which could have contributed to the rapid weight loss (185); this effect was seen less often in patients with gastric banding (185). Another study in adult patients showed higher bile acid (BA) subtype levels and suggested a possible BA's role in the surgical weight loss response after LSG (188). Adolescents have lower surgical complication rates than their adult counterparts, hence considering bariatric surgery earlier rather than waiting until adulthood has been entertained (180). Complications after surgery include nutritional imbalance in iron, calcium, Vitamin D, and B12 and should be monitored closely (180, 181, 185). Although 5-year data for gastric bypass in very obese teens is promising, lifetime outcome is still unknown, and the psychosocial factors associated with adolescent adherence post-surgery are also challenging and uncertain.
Conclusion
Obesity in childhood and adolescence is not amenable to a single easily modified factor. Biological, cultural, and environmental factors such as readily available high-density food choices impact youth eating behaviors. Media devices and associated screen time make physical activity a less optimal choice for children and adolescents. This review serves as a reminder that the time for action is now. The need for interventions to change the obesogenic environment by instituting policies around the food industry and in the schools needs to be clarified. In clinical trials GLP-1 agonists are shown to be effective in weight loss in children but are not yet FDA approved. Discovery of therapies to modify the gut microbiota as treatment for overweigh/obesity through use of probiotics or fecal transplantation would be revolutionary. For the present, ongoing clinical research efforts in concert with pharmacotherapeutic and multidisciplinary lifestyle programs hold promise.
Author Contributions
AK, SL, and MJ contributed to the conception and design of the study. All authors contributed to the manuscript revision, read, and approved the submitted version.
Conflict of Interest
The authors declare that the research was conducted in the absence of any commercial or financial relationships that could be construed as a potential conflict of interest.
References
1. Gurnani M, Birken C, Hamilton. J. Childhood obesity: causes, consequences, and management. Pediatr Clin North Am. (2015) 62:821–40. doi: 10.1016/j.pcl.2015.04.001
2. Sahoo K, Sahoo B, Choudhury AK, Sofi NY, Kumar R, Bhadoria. AS. Childhood obesity: causes and consequences. J Family Med Prim Care. (2015) 4:187–92. doi: 10.4103/2249-4863.154628
3. Brown CL, Halvorson EE, Cohen GM, Lazorick S, Skelton JA. Addressing childhood obesity: opportunities for prevention. Pediatr Clin North Am. (2015) 62:1241–61. doi: 10.1016/j.pcl.2015.05.013
4. Qasim A, Turcotte M, de Souza RJ, Samaan MC, Champredon D, Dushoff J, et al. On the origin of obesity: identifying the biological, environmental, and cultural drivers of genetic risk among human populations. Obes Rev. (2018) 19:121–49. doi: 10.1111/obr.12625
5. Rinninella E, Raoul P, Cintoni M, Fransceschi F, Miggiano GAD, Gasbarrini A, et al. What is the healthy gut microbiota composition? a changing ecosystem across age, environment, diet, and diseases. Microorganisms. (2019) 7:14. doi: 10.3390/microorganisms7010014
6. Indrio F, Martini S, Francavilla R, Corvaglia L, Cristofori F, Mastrolia SA, et al. Epigenetic matters: the link between early nutrition, microbiome, and long-term health development. Front Pediatr. (2017) 5:178. doi: 10.3389/fped.2017.00178
7. Marcovecchio ML, Gorman S, Watson LPE, Dunger DB, Beardsall K. Catch-up growth in children born small for gestational age related to body composition and metabolic risk at six years of age in the UK. Horm Res Paediatr. (2020) 93:119–27. doi: 10.1159/000508974
8. Koletzko B, Fishbein M, Lee WS, Moreno L, Mouane N, Mouzaki M, et al. Prevention of childhood obesity: a position paper of the global federation of international societies of paediatric gastroenterology, hepatology nutrition (FISPGHAN). J Pediatr Gastroenterol Nutr. (2020) 70:702–10. doi: 10.1097/MPG.0000000000002708
9. Pulgarón ER. Childhood obesity: a review of increased risk for physical and psychological comorbidities. Clin Ther. (2013) 35:A18–32. doi: 10.1016/j.clinthera.2012.12.014
10. De Leonibus C, Marcovecchio ML, Chiarelli F. Update on statural growth and pubertal development in obese children. Pediatr Rep. (2012) 4:e35. doi: 10.4081/pr.2012.e35
11. Witchel SF, Burghard AC, Tao RH, Oberfield SE. The diagnosis and treatment of PCOS in adolescents. Curr Opin Pediatr. (2019) 31:562–9. doi: 10.1097/MOP.0000000000000778
12. Marcus CL, Brooks LJ, Draper KA, Gozal D, Halbower AC, Jones J, et al. Diagnosis and management of childhood obstructive sleep apnea syndrome. Pediatrics. (2012) 130:e714–55. doi: 10.1542/peds.2012-1672
13. Rankin J, Matthews L, Cobley S, Han A, Sanders R, Wiltshire HD, et al. Psychological consequences of childhood obesity: psychiatric comorbidity and prevention. Adolesc Health Med Ther. (2016) 7:125–46. doi: 10.2147/AHMT.S101631
14. Topçu S, Orhon FS, Tayfun M, Uçaktürk SA, Demirel F. Anxiety, depression, and self-esteem levels in obese children: a case-control study. J Pediatr Endocrinol Metabol. (2016) 29:357–61. doi: 10.1515/jpem-2015-0254
15. Katzmarzyk PT, Barlow S, Bouchard C, Catalano PM, Hsia DS, Inge TH, et al. An evolving scientific basis for the prevention and treatment of pediatric obesity. Int J Obes. (2014) 38:887–905. doi: 10.1038/ijo.2014.49
16. Brown T, Moore TH, Hooper L, Gao Y, Zayegh A, Ijaz S, et al. Interventions for preventing obesity in children. Cochrane Database Syst Rev. (2019) 7:CD001871. doi: 10.1002/14651858.CD001871.pub4
17. Smith E, Scarborough P, Rayner M, Briggs ADM. Should we tax unhealthy food and drink? Proc Nutr Soc. (2019) 77:314–20. doi: 10.1017/S0029665117004165
18. Adab P, Pallan M, Whincup PH. Is BMI the best measure of obesity? BMJ. (2018) 360:k 1274. doi: 10.1136/bmj.k1274
19. Anderson LN, Carsley S, Lebovic G, Borkhoff CM, Maguire JL, Parkin PC, et al. Misclassification of child body mass index from cut-points defined by rounded percentiles instead of Z-scores. BMC Res Notes. (2017) 10:639. doi: 10.1186/s13104-017-2983-0
20. Must A, Anderson SE. Body mass index in children and adolescents: consideration for population-based applications. Int J Obes. (2006) 30:590–4. doi: 10.1038/sj.ijo.0803300
21. Flegal KM, Wei R, Ogden C. Weight-for-stature compared with body mass index-for-age growth charts for the United States from the centers for disease control and prevention. Am J Clin Nutr. (2002) 75:761–6.22. doi: 10.1093/ajcn/75.4.761
22. Himes JH, Dietz WH. Guidelines for overweight in adolescent preventive services: recommendations from an expert committee. The expert committee on clinical guidelines for overweight in adolescent preventive services. Am J Clin Nutr. (1994) 59:307–16. doi: 10.1093/ajcn/59.2.307
23. Lazarus R, Baur L, Webb K, Blyth F. Body mass index in screening for adiposity in children and adolescents: systematic evaluation using receiver operating characteristic curves. Am J Clin Nutr. (1996) 63:500–6. doi: 10.1093/ajcn/63.4.500
24. McGinnis JM, Gootman JA. Food Marketing to Children and Youth: Threat or Opportunity? Institute of Medicine of the National Academies. Washington, DC: The National Academies Press. (2006).
25. Chaudhri OB, Salem V, Murphy KG, Bloom SR. Gastrointestinal satiety signals. Annu Rev Physiol. (2008) 70:239–55. doi: 10.1146/annurev.physiol.70.113006.100506
26. Scaglioni S, De Cosmi V, Ciappolino V, Parazzini F, Brambilla P, Agostoni C. Factors influencing children's eating behaviours. Nutrients. (2018) 10:706. doi: 10.3390/nu10060706
27. Ahima RS, Antwi DA. Brain regulation of appetite and satiety. Endocrinol Metab Clin North Am. (2008) 37:811–23. doi: 10.1016/j.ecl.2008.08.005
28. Niswender KD, Baskin DG, Schwartz MW. Review insulin and its evolving partnership with leptin in the hypothalamic control of energy homeostasis. Trends Endocrinol Metab. (2004) 15:362–9. doi: 10.1016/j.tem.2004.07.009
29. Niswender KD, Schwartz MW. Review insulin and leptin revisited: adiposity signals with overlapping physiological and intracellular signaling capabilities. Front Neuroendocrinol. (2003) 24:1–10. doi: 10.1016/S0091-3022(02)00105-X
30. Amitani M, Asakawa A, Amitani H, Inui. A. The role of leptin in the control of insulin-glucose axis. Front Neurosci. (2013) 7:51. doi: 10.3389/fnins.2013.00051
31. Cowley MA, Smith RG, Diano S, Tschöp M, Pronchuk N, Grove KL, et al. The distribution and mechanism of action of ghrelin in the CNS demonstrates a novel hypothalamic circuit regulating energy homeostasis. Neuron. (2003) 37:649–61. doi: 10.1016/S0896-6273(03)00063-1
32. Buhmann H, le Roux CW, Bueter M. The gut–brain axis in obesity. Best Prac Res Clin Gastroenterol. (2014) 28:559–71. doi: 10.1016/j.bpg.2014.07.003
33. Cone RD. Review anatomy and regulation of the central melanocortin system. Nat Neurosci. (2005) 8:571–8. doi: 10.1038/nn1455
34. Timper K, Brüning JC. Hypothalamic circuits regulating appetite and energy homeostasis: pathways to obesity. Dis Model Mech. (2017) 10:679–89. doi: 10.1242/dmm.026609
35. Labarthe A, Fiquet O, Hassouna R, Zizzari P, Lanfumey L, Ramoz N, et al. Ghrelin-derived peptides: a link between appetite/reward, gh axis, and psychiatric disorders? Front Endocrinol. (2014) 5:163. doi: 10.3389/fendo.2014.00163
36. Hills R. D Jr, Pontefract BA, Mishcon HR, Black CA, Sutton SC, Theberge CR. Gut microbiome: profound implications for diet and disease. Nutrients. (2019) 11:1613. doi: 10.3390/nu11071613
37. Torres-Fuentes C, Schellekens H, Dinan TG, Cryan JF. The microbiota-gut-brain axis in obesity. Lancet Gastroenterol Hepatol. (2017) 2:747–56. doi: 10.1016/S2468-1253(17)30147-4
38. Gérard P. Gut microbiota and obesity. Cell Mol Life Sci. (2016) 73:147–62. doi: 10.1007/s00018-015-2061-5
39. Derrien M, Alvarez AS, de Vos WM. The gut microbiota in the first decade of life. Trends Microbiol. (2019) 27:997–1010.40. doi: 10.1016/j.tim.2019.08.001
40. Dao MC, Clément K. Gut microbiota and obesity: concepts relevant to clinical care. Eur J Intern Med. (2018) 48:18–24.41. doi: 10.1016/j.ejim.2017.10.005
41. Kim KN, Yao Y., Ju SY. Short chain fatty acids and fecal microbiota abundance in humans with obesity: a systematic review and meta-analysis. Nutrients. (2019) 11:2512. doi: 10.3390/nu11102512
42. Castaner O, Goday A, Park YM, Lee SH, Magkos F, Shiow STE, et al. The gut microbiome profile in obesity: a systematic review. Int J Endocrinol. (2018) 2018:4095789. doi: 10.1155/2018/4095789
43. Riva A, Borgo F, Lassandro C, Verduci E, Morace G, Borghi E, et al. Pediatric obesity is associated with an altered gut microbiota and discordant shifts in firmicutes populations. Enviroin Microbiol. (2017) 19:95–105. doi: 10.1111/1462-2920.13463
44. Fernandes J, Su W, Rahat-Rozenbloom S, Wolever TMS, Comelli EM. Adiposity, gut microbiota and faecal short chain fatty acids are linked in adult humans. Nutr Diabetes. (2014) 4:e121. doi: 10.1038/nutd.2014.23
45. Rahat-Rozenbloom S, Fernandes J, Gloor GB, Wolever TMS. Evidence for greater production of colonic short-chain fatty acids in overweight than lean humans. Int J Obes. (2014) 38:1525–31. doi: 10.1038/ijo.2014.46
46. Barczyńska R, Litwin M, Slizewska K, Szalecki M, Berdowska A, Bandurska K, et al. Bacterial microbiota fatty acids in the faeces of overweight obese children. Pol. J. Microbiol. (2018) 67:339–45. doi: 10.21307/pjm-2018-041
47. Albillos A, de Gottardi A, Rescigno M. The gut-liver axis in liver disease: Pathophysiological basis for therapy. J Hepatol. (2020) 72:558–77. doi: 10.1016/j.jhep.2019.10.003
48. Yu EL, Golshan S, Harlow KE, Angeles JE, Durelle J, Goyal NP, et al. Prevalence of nonalcoholic fatty liver disease in children with obesity. J Pediatr. (2019) 207:64–70. doi: 10.1016/j.jpeds.2018.11.021
49. Ranucci G, Spagnuolo MI, Iorio R. Obese children with fatty liver: Between reality and disease mongering. World J Gastroenterol. (2017) 23:8277–82. doi: 10.3748/wjg.v23.i47.8277
50. Cox AJ, West NP, Cripps A. W. Obesity, inflammation, and the gut microbiota. Lancet Diabet Endocrinol. (2015) 3:207–15. doi: 10.1016/S2213-8587(14)70134-2
51. Seitz J, Trinh S, Herpertz-Dahlmann B. The microbiome and eating disorders. Psychiatr Clin North Am. . (2019) 42:93–103. doi: 10.1016/j.psc.2018.10.004
52. Deans E. Microbiome and mental health in the modern environment. J Physiol Anthropol. (2016) 36:1. doi: 10.1186/s40101-016-0101-y
53. Peirce JM, Alviña K. The role of inflammation and the gut microbiome in depression and anxiety. J Neurosci Res. (2019) 97:1223–41. doi: 10.1002/jnr.24476
54. Ranadive SA, Vaisse C. Lessons from extreme human obesity: monogenic disorders. Endocrinol Metab Clin North Am. (2008) 37:733–51. doi: 10.1016/j.ecl.2008.07.003
55. Soliman AT, Yasin M, Kassem A. Leptin in pediatrics: a hormone from adipocyte that wheels several functions in children. Indian J Endocrinol Metab. (2012) 16(Suppl. 3):S577–87. doi: 10.4103/2230-8210.105575
56. Farooqi IS, Wangensteen T, Collins S, Kimber W, Matarese G, Keogh JM, et al. Clinical and molecular genetic spectrum of congenital deficiency of the leptin receptor. N Engl J Med. (2007) 356:237–47. doi: 10.1056/NEJMoa063988
57. Mutch DM, Clément K. Unraveling the genetics of human obesity. PLoS Genet. (2006) 2:e188. doi: 10.1371/journal.pgen.0020188
58. Crocker MK, Yanovski JA. Pediatric obesity: etiology and treatment. Endocrinol Metab Clin North Am. (2009) 38:525–48. doi: 10.1016/j.ecl.2009.06.007
59. Huvenne H, Dubern B, Clément K, Poitou C. Rare genetic forms of obesity: clinical approach and current treatments in 2016. Obes Facts. (2016) 9:158–73. doi: 10.1159/000445061
60. Stefan M, Nicholls RD. What have rare genetic syndromes taught us about the pathophysiology of the common forms of obesity? Curr Diab Rep. (2004) 4:143–50. doi: 10.1007/s11892-004-0070-0
61. Hetherington MM, Cecil JE. Gene-Environment interactions in obesity. Forum Nutr. (2009) 63:195–203. doi: 10.1159/000264407
62. Reddon H, Guéant JL, Meyre D. The importance of gene-environment interactions in human obesity. Clin Sci. (2016) 130:1571–97. doi: 10.1042/CS20160221
63. Castillo JJ, Orlando RA, Garver WS. Gene-nutrient interactions and susceptibility to human obesity. Genes Nutr. (2017) 12:29. doi: 10.1186/s12263-017-0581-3
64. Heianza Y, Qi L. Gene-Diet interaction and precision nutrition in obesity. Int J Mol Sci. (2017) 18:787. doi: 10.3390/ijms18040787
65. Goodarzi MO. Genetics of obesity: what genetic association studies have taught us about the biology of obesity and its complications. Lancet Diabetes Endocrinol. (2018) 6:223–36. . doi: 10.1016/S2213-8587(17)30200-0
66. Bouchard L, Drapeau V, Provencher V, Lemieux S, Chagnon Y, Rice T, et al. Neuromedin beta: a strong candidate gene linking eating behaviors and susceptibility to obesity. Am J Clin Nutr. (2004) 80:1478–86. . doi: 10.1093/ajcn/80.6.1478
67. Grimm ER, Steinle NI. Genetics of eating behavior: established and emerging concepts. Nutr Rev. (2011) 69:52–60. . doi: 10.1111/j.1753-4887.2010.00361.x
68. van der Klaauw AA, Farooqi IS. The hunger genes: pathways to obesity. Cell. (2015) 161:119–32. . doi: 10.1016/j.cell.2015.03.008
69. Martinez JA. Bodyweight regulation causes of obesity. Proc Nutr Soc. (2000) 59:337–45. Review. doi: 10.1017/S0029665100000380
70. Rask-Andersen M, Karlsson T, Ek WE, Johansson Å. Gene-environment interaction study for BMI reveals interactions between genetic factors and physical activity, alcohol consumption and socioeconomic status. PLoS Genet. (2017) 5:1. doi: 10.1371/journal.pgen.1006977
71. Xulong S, Pengzhou L, Xiangwu Y, Weizheng L, Xianjie Q, Shaihong Z, et al. From genetics and epigenetics to the future of precision treatment for obesity. Gastroenterol Rep. (2017) 5:266–70. doi: 10.1093/gastro/gox033
72. Bianco-Miotto T, Craig JM, Gasser YP, van dijk SJ, Ozanne SE. Epigenetics and DOHaD: from basics to birth and beyond. J Dev Orig Health Dis. (2017) 8:513–9. doi: 10.1017/S2040174417000733
73. van Dijk SJ, Molloy PL, Varinli H, Morrison JL, Muhlhausler BS, Members of EpiSCOPE. Epigenetics and human obesity. Int J Obes. (2015) 39:85–97. doi: 10.1038/ijo.2014.34
74. Li Y. Epigenetic mechanisms link maternal diets and gut microbiome to obesity in the offspring. Front Genet. (2018) 9:342. doi: 10.3389/fgene.2018.00342
75. Kaufman J, Montalvo-Ortiz JL, Holbrook H, O'Loughlin K, Orr C, Kearney C, et al. Adverse childhood experiences, epigenetic measures, and obesity in youth. J Pediatr. (2018) 202:150–6.76. doi: 10.1016/j.jpeds.2018.06.051
76. May Gardner R, Feely A, Layte R, Williams J, McGavock J. Adverse childhood experiences are associated with an increased risk of obesity in early adolescence: a population-based prospective cohort study. Pediatr Res. (2019) 86:522–28. doi: 10.1038/s41390-019-0414-8
77. Cheon BK„ Hong YY. Mere experience of low subjective socioeconomic status stimulates appetite food intake. Proc Natl Acad Sci USA. (2017) 114:72–7. doi: 10.1073/pnas.1607330114
78. Alegría-Torres JA, Baccarelli A, Bollati V. Epigenetics lifestyle. Epigenomics. (2011) 3:267-77. doi: 10.2217/epi.11.22
79. Birch LL, Fisher JO. Development of eating behaviors among children and adolescents. Pediatrics. (2011) 101:539–49.
80. Birch L, Savage JS, Ventura A. Influences on the development of children's eating behaviours: from infancy to adolescence. Can J Diet Pract Res. (2007) 68:s1–s56.
81. Nielsen SJ, Popkin BM. Patterns and trends in food portion sizes, 1977- 1998. JAMA. (2003) 289:450–53. . doi: 10.1001/jama.289.4.450
82. Munoz KA, Krebs-Smith SM, Ballard-Barbash R, Cleveland LE. Food intakes of US children and adolescents compared with recommendations. Pediatrics. (1997) 100:323–29. doi: 10.1542/peds.100.3.323
83. Fisher JO, Birch LL. Restricting access to palatable foods affects children's behavioral response, food selection, and intake. Am J Clin Nutr. (1999) 69:1264–72. doi: 10.1093/ajcn/69.6.1264
84. Faith MS, Scanlon KS, Birch LL, Francis LA, Sherry B. Parent-child feeding strategies and their relationships to child eating and weight status. Obes Res. (2004) 12:1711–22. . doi: 10.1038/oby.2004.212
85. Smith AD, Sanchez N, Reynolds C, Casamassima M, Verros M, Annameier SK, et al. Associations of parental feeding practices and food reward responsiveness with adolescent stress-eating. Appetite. (2020) 152:104715. doi: 10.1016/j.appet.2020.104715
86. Lowe CJ, Morton JB, Reichelt AC. Adolescent obesity and dietary decision making-a brain-health perspective. Lancet Child Adolesc Health. (2020) 4:388–96. doi: 10.1016/S2352-4642(19)30404-3
87. Goran MI, Treuth MS. Energy expenditure, physical activity, and obesity in children. Pediatr Clin North Am. (2001) 48:931–53. doi: 10.1016/S0031-3955(05)70349-7
88. Romieu I, Dossus L, Barquera S, Blottière HM, Franks PW, Gunter M, et al. Energy balance and obesity: what are the main drivers? Cancer Causes Control. (2017) 28:247–58. doi: 10.1007/s10552-017-0869-z
89. Mattes R, Foster GD. Food environment and obesity. Obesity. (2014) 22:2459–61. doi: 10.1002/oby.20922
90. Ickovics JR, O'Connor Duffany K, Shebl FM, Peters SM, Read MS, Gilstad-Hayden KR, et al. Implementing school-based policies to prevent obesity: cluster randomized trial. Am J Prev Med. (2019) 56:e1–11. doi: 10.1016/j.amepre.2018.08.026
91. Micha R, Karageorgou D, Bakogianni I, Trichia E, Whitsel LP, Story M, et al. Effectiveness of school food environment policies on children's dietary behaviors: A systematic review and meta-analysis. PLoS ONE. (2018) 13:e0194555. doi: 10.1371/journal.pone.0194555
92. Cawley J, Frisvold D, Hill A, Jones DJ. The impact of the philadelphia beverage tax on purchases and consumption by adults and children. Health Econ. (2019) 67:102225. doi: 10.1016/j.jhealeco.2019.102225
93. John Cawley J, Thow AM, Wen K, Frisvold D. The economics of taxes on sugar-sweetened beverages: a review of the effects on prices, sales, cross-border shopping, and consumption. Annu Rev Nutr. (2019) 39:317–38. doi: 10.1146/annurev-nutr-082018-124603
94. Fuller C, Lehman E, Hicks S, Novick MB. Bedtime use of technology and associated sleep problems in children. Glob Pediatr Health. (2017) 4:2333794X17736972. doi: 10.1177/2333794X17736972
95. Chahal H, Fung C, Kuhle S, Veugelers PJ. Availability and night-time use of electronic entertainment and communication devices are associated with short sleep duration and obesity among Canadian children. Pediatr Obes. (2012) 8:42–51. doi: 10.1111/j.2047-6310.2012.00085.x
96. Minghua T. Protein intake during the first two years of life and its association with growth and risk of overweight. Int J Environ Res Public Health. (2018) 15:1742. doi: 10.3390/ijerph15081742
97. Azad MB, Vehling L, Chan D, Klopp A, Nickel NC, McGavock JM, et al. Infant feeding and weight gain: separating breast milk from breastfeeding and formula from food. Pediatrics. (2018) 142:e20181092. doi: 10.1542/peds.2018-1092
98. Lin L, Amissah E, Gamble GD, Crowther CA, Harding JE. Impact of macronutrient supplements on later growth of children born preterm or small for gestational age: a systematic review and meta-analysis of randomised and quasirandomised controlled trials. PLoS Med. (2020) 17:e1003122. . doi: 10.1371/journal.pmed.1003122
99. Rzehak P, Oddy WH, Mearin ML, Grote V, Mori TA, Szajewska H, et al. Infant feeding and growth trajectory patterns in childhood and body composition in young adulthood. Am J Clin Nutr. (2017) 106:568–80. doi: 10.3945/ajcn.116.140962
100. Styne DM, Arslanian SA, Connor EL, Farooqi IS, Murad MH, Silverstein JH. Pediatric obesity-assessment, treatment, and prevention: an endocrine society clinical practice guideline. J Clin Endocrinol Metab. (2017) 102:709–57. doi: 10.1210/jc.2016-2573
101. Whitaker RC, Pepe MS, Wright JA, Seidel KD, Dietz WH. Early adiposity rebound and the risk of adult obesity. Pediatrics. (1998) 101:E5. doi: 10.1542/peds.101.3.e5
102. Geserick M, Vogel M, Gausche R, Lipek T, Spielau U, Keller E, et al. Acceleration of BMI in early childhood and risk of sustained obesity. N Engl J Med. (2018) 379:1303–12. doi: 10.1056/NEJMoa1803527
103. Jabakhanji SB, Boland F, Ward M, Biesma RJ. Body mass index changes in early childhood. Pediatrics. (2018) 202:106–14. doi: 10.1016/j.jpeds.2018.06.049
104. Chung S. Growth and puberty in obese children and implications of body composition. J Obes Metab Syndr. (2017) 26:243–50. doi: 10.7570/jomes.2017.26.4.243
105. Tagi VM, Giannini C, Chiarelli F. Insulin resistance in children. Front Endocrinol. (2019) 10:342. doi: 10.3389/fendo.2019.00342
106. Kelesidis I, Mantzoros CS. Leptin and its emerging role in children and adolescents. Clin Pediatr Endocrinol. (2006) 15:1–14. doi: 10.1297/cpe.15.1
107. Burt Solorzano CM, McCartney CR, Obesity and the pubertal transition in girls and boys. Reproduction. (2010) 140:399–410. doi: 10.1530/REP-10-0119
108. Li W, Liu Q, Deng X, Chen Y, Liu S, Story M. Association between obesity and puberty timing: a systematic review and meta-analysis. Int J Environ Res Public Health. (2017) 14:1266. doi: 10.3390/ijerph14101266
109. Lee JM, Wasserman R, Kaciroti N, Gebremariam A, Steffes J, Dowshen S, et al. Timing of puberty in overweight vs. obese boys. Pediatrics. (2016) 137:e20150164. doi: 10.1542/peds.2015-0164
110. He J, Kang Y, Zheng L. Serum levels of LH, IGF-1 and leptin in girls with idiopathic central precocious puberty (ICPP) and the correlations with the development of ICPP. Minerva Pediatr. (2018). doi: 10.23736/S0026-4946.18.05069-7
111. Kang MJ, Oh YJ, Shim YS, Baek JW, Yang S, Hwang IT. The usefulness of circulating levels of leptin, kisspeptin, and neurokinin B in obese girls with precocious puberty. Gynecol Endocrinol. (2018) 34:627–30. doi: 10.1080/09513590.2017.1423467
112. Rendo-Urteaga T, Ferreira de Moraes AC, Torres-Leal FL, Manios Y, Gottand F, Sjöström M, et al. Leptin and adiposity as mediators on the association between early puberty and several biomarkers in European adolescents: the helena study. J Pediatr Endocrinol Metab. (2018) 31:1221–29. doi: 10.1515/jpem-2018-0120
113. Franks S. Adult polycystic ovary syndrome begins in childhood. Best Pract Res Clin Endocrinol Metab. (2002) 16:263–72. doi: 10.1053/beem.2002.0203
114. Franks S. Polycystic ovary syndrome in adolescents. Int J Obes. (2008) 32:1035–41. doi: 10.1038/ijo.2008.61
115. Jehan S, Zizi F, Pandi-Perumal SR, Wall S, Auguste E, Myers K, et al. Obstructive sleep apnea and obesity: implications for public health. Sleep Med Disord. (2017) 1:00019.
116. Patinkin ZW, Feinn R, Santos M. Metabolic consequences of obstructive sleep apnea in adolescents with obesity: a systematic literature review and meta-analysis. Childhood Obes. (2017) 13:102–10. doi: 10.1089/chi.2016.0248
117. Kaditis A. From obstructive sleep apnea in childhood to cardiovascular disease in adulthood: what is the evidence? Sleep. (2010) 33:1279–80. doi: 10.1093/sleep/33.10.1279
118. Marseglia L, Manti S, D'Angelo G, Nicotera A, Parisi E, Di Rose G, et al. Oxidative stress in obesity: a critical component in human diseases. Int J Mol Sci. (2014) 16:378–400. doi: 10.3390/ijms16010378
119. Eisele HJ, Markart P, Schulz R. Obstructive sleep apnea, oxidative stress, and cardiovascular disease: evidence from human studies. Oxid Med Cell Longev. (2015) 2015:608438. doi: 10.1155/2015/608438
120. Hui W, Slorach C, Guerra V, Parekh RS, Hamilton J, Messiha S, et al. Effect of obstructive sleep apnea on cardiovascular function in obese youth. Am J Cardiol. (2019) 123:341–7. doi: 10.1016/j.amjcard.2018.09.038
121. Matteoni CA, Younossi Z .m., Gramlich T, Boparai N, Liu YC, et al. Nonalcoholic fatty liver disease: a spectrum of clinical and pathological severity. Gastroenterology. (1999) 1999:116:1413. doi: 10.1016/S0016-5085(99)70506-8
122. Lavine JE, Schwimmer JB. Nonalcoholic fatty liver disease in the pediatric population. Clin Liver Dis. (2004) 8:549. doi: 10.1016/j.cld.2004.04.010
123. Huang JS, Barlow SE, Quiros-Tejeira RE, Scheimann A, Skelton J, Suskind D, et al. Childhood obesity for pediatric gastroenterologists. J Pediatr Gastroenterol Nutr. (2013) 2013:56:99. doi: 10.1097/MPG.0b013e31826d3c62
124. Anderson EL, Howe LD, Jones HE, Higgins JPT, Lawlor DA, Fraser A. The prevalence of non-alcoholic fatty liver disease in children and adolescents: a systematic review and meta-analysis. PLoS ONE. (2015) 10:e0140908. doi: 10.1371/journal.pone.0140908
125. Nobili V, Alisi A, Newton KP, Schwimmer JB. Comparison of the phenotype and approach to pediatric vs adult patients with nonalcoholic fatty liver disease. Gastroenterology. (2016) 150:1798–810. doi: 10.1053/j.gastro.2016.03.009
126. Rafiq N, Bai C, Fang Y, Srishord M, McCullough A, Gramlich T, et al. Long-term follow-up of patients with nonalcoholic fatty liver. Clin Gastroenterol Hepatol. (2009) 7:234–38. doi: 10.1016/j.cgh.2008.11.005
127. Feldstein AE, Charatcharoenwitthaya P, Treeprasertsuk S, Benson JT, Enders FB, Angula P. The natural history of non-alcoholic fatty liver disease in children: a follow-up study for up to 20 years. Gut. (2009) 58:1538. doi: 10.1136/gut.2008.171280
128. Schwimmer JB, Pardee PE, Lavine JE, Blumkin AK, Cook S. Cardiovascular risk factors and the metabolic syndrome in pediatric nonalcoholic fatty liver disease. Circulation. (2008) 118:277. doi: 10.1161/CIRCULATIONAHA.107.739920
129. Perry DC, Metcalfe D, Lane S, Turner S. Childhood obesity and slipped capital femoral epiphysis. Pediatrics. (2018) 142:e20181067. doi: 10.1542/peds.2018-1067
130. Zavala-Crichton JP, Esteban-Cornejo I, Solis-Urra P, Mora-Gonzalez J, Cadenas-Sanchez C, Rodriguez-Ayllon M, et al. Association of sedentary behavior with brain structure and intelligence in children with overweight or obesity: Active Brains Project. (2020) 9:1101. doi: 10.3390/jcm9041101
131. Ronan L, Alexander-Bloch A, Fletcher PC. Childhood obesity, cortical structure, and executive function in healthy children. Cereb Cortex. (2019) 30:2519–28. doi: 10.1093/cercor/bhz257
132. Baker ER. Body weight and the initiation of puberty. Clin Obstetr Gynecol. (1985) 28:573–9. doi: 10.1097/00003081-198528030-00013
133. Siervogel RM, Demerath EW, Schubert C, Remsberg KE, Chumlea WM, Sun S, et al. Puberty and body composition. Horm Res. (2003) 60:36–45. doi: 10.1159/000071224
134. Sadeeqa S, Mustafa T, Latif S. Polycystic ovarian syndrome- related depression in adolescent girls. J Pharm Bioallied Sci. (2018) 10:55–9. doi: 10.4103/JPBS.JPBS_1_18
135. Himelein MJ, Thatcher SS. Depression and body image among women with polycystic ovary syndrome. J Health Psychol. (2006) 11:613–25. doi: 10.1177/1359105306065021
136. Magge SN, Goodman E, Armstrong SC. The metabolic syndrome in children and adolescents: shifting the focus to cardiometabolic risk factor clustering. Pediatrics. (2017) 140:e20171603. doi: 10.1542/peds.2017-1603
137. Mauras N, Delgiorno C, Kollman C, Bird K, Morgan M, Sweeten S, et al. Obesity without established comorbidities of the metabolic syndrome is associated with a proinflammatory and prothrombotic state, even before the onset of puberty in children. J Clin Endocrinol Metab. (2010) 95:1060–8. doi: 10.1210/jc.2009-1887
138. Weiss R, Dziura J, Burgert TS, Tamborlane WV, Taksali SE, Yeckel CW, et al. Obesity and the metabolic syndrome in children and adolescents. N Engl J Med. (2004) 350:2362–74. doi: 10.1056/NEJMoa031049
139. Erdmann J, Kallabis B, Oppel U, Sypchenko O, Wagenpfeil S, Schusdziarra V. Development of hyperinsulinemia and insulin resistance during the early stage of weight gain. Am J Physiol Endocrinol Metabol. (2008) 294:e568–75. . doi: 10.1152/ajpendo.00560.2007
140. Pulido-Arjona L, Correa-Bautista JE, Agostinis-Sobrinho C, Mota J, Santos R, Correa-Rodrigues M, et al. Role of sleep duration and sleep- related problems in the metabolic syndrome among children and adolescents. Ital J Pediatr. (2018) 44:9. doi: 10.1186/s13052-018-0451-7
141. Harriger JA, Thompson JK. Psychological consequences of obesity: weight bias and body image in overweight and obese youth. Int Rev Psychiatry. (2012) 24:247–53. . doi: 10.3109/09540261.2012.678817
142. Bacchini D, Licenziati MR, Garrasi A, Corciulo N, Driul D, Tanas R, et al. Bullying and victimization in overweight and obese outpatient children and adolescents: an italian multicentric study. PLoS ONE. (2015) 10:e0142715. doi: 10.1371/journal.pone.0142715
143. Loth KA, Watts AW, Berg PVD, Neumark-Sztainer D. Does body satisfaction help or harm overweight teens? A 10-year longitudinal study of the relationship between body satisfaction and body mass index. J Adolesc Health. (2015) 57:559–61. doi: 10.1016/j.jadohealth.2015.07.008
144. Gowey MA, Lim CS, Clifford LM, Janicke DM. Disordered eating and health-related quality of life in overweight and obese children. J Pediatr Psychol. (2014) 39:552–61. doi: 10.1093/jpepsy/jsu012
145. Mannan M, Mamun A, Doi S, Clavarino A. Prospective associations between depression and obesity for adolescent males and females- a systematic review and meta-analysis of longitudinal studies. PLoS ONE. (2016) 11:e0157240. doi: 10.1371/journal.pone.0157240
146. Ruiz LD, Zuelch ML, Dimitratos SM, Scherr RE. Adolescent obesity: diet quality, psychosocial health, and cardiometabolic risk factors. Nutrients. (2019) 12:43. doi: 10.3390/nu12010043
147. Goldschmidt AB, Aspen VP, Sinton MM, Tanofsky-Kraff M, Wilfley DE. Disordered eating attitudes and behaviors in overweight youth. Obesity. (2008) 16:257–64. doi: 10.1038/oby.2007.48
148. Golden NH, Schneider M, Wood C. Preventing obesity and eating disorders in adolescents. Pediatrics. (2016) 138:e1–e12. doi: 10.1542/peds.2016-1649
149. Rastogi R, Rome ES. Restrictive eating disorders in previously overweight adolescents and young adults. Cleve Clin J Med. (2020) 87:165–71. doi: 10.3949/ccjm.87a.19034
150. Hayes JF, Fitzsimmons-Craft EE, Karam AM, Jakubiak JL, Brown ME, Wilfley D. Disordered eating attitudes and behaviors in youth with overweight and obesity: implications for treatment. Curr Obes Rep. (2018) 7:235. doi: 10.1007/s13679-018-0316-9
151. Goldschmidt AB, Wall MM, Loth KA, Neumark-Sztainer D. Risk factors for disordered eating in overweight adolescents and young adults: Table I. J Pediatr Psychol. (2015) 40:1048–55. doi: 10.1093/jpepsy/jsv053
152. Follansbee-Junger K, Janicke DM, Sallinen BJ. The influence of a behavioral weight management program on disordered eating attitudes and behaviors in children with overweight. J Am Diet Assoc. (2010) 110:653–9. doi: 10.1016/j.jada.2010.08.005
153. Blake-Lamb TL, Locks LM, Perkins ME, Woo Baidal JA, Cheng ER, Taveras EM. Interventions for childhood obesity in the first 1,000 days a systematic review. Am J Prev Med. (2016) 50:780–9. doi: 10.1016/j.amepre.2015.11.010
154. McGuire S. Institute of Medicine (IOM). Early childhood obesity prevention policies. Washington, DC: The National Academies Press. Adv Nutr. (2011) 3:56–7. doi: 10.3945/an.111.001347
155. Pont SJ, Puhl R, Cook SR, Slusser W. Stigma experienced by children and adolescents with obesity. Pediatrics. (2017) 140:e20173034. doi: 10.1542/peds.2017-3034
156. Puhl R, Suh Y. Health consequences of weight stigma: implications for obesity prevention and treatment. Curr Obes Rep. (2015) 4:182–90. doi: 10.1007/s13679-015-0153-z
157. Schwimmer JB, Burwinkle TM, Varni JW. Health-related quality of life of severely obese children and adolescents. JAMA. (2003) 289:1813–9. doi: 10.1001/jama.289.14.1813
158. Carcone AI, Jacques-Tiura AJ, Brogan Hartlieb KE, Albrecht T, Martin T. Effective patient-provider communication in pediatric obesity. Pediatr Clin North Am. (2016) 63:525–38. doi: 10.1016/j.pcl.2016.02.002
159. Coppock JH, Ridolfi DR, Hayes JF, Paul MS, Wilfley DE. Current approaches to the management of pediatric overweight and obesity. Curr Treat Options Cardiovasc Med. (2014) 16:343. doi: 10.1007/s11936-014-0343-0
160. Davison KK, Jurkowski JM, Li K, Kranz S, Lawson HA. A childhood obesity intervention developed by families for families: results from a pilot study. Int J Behav Nutr Phys Act. (2013) 10:3. doi: 10.1186/1479-5868-10-3
161. Krystia O, Ambrose T, Darlington G, Ma DWL, Buchholz AC, Haines J. A randomized home- based childhood obesity prevention pilot intervention has favourable effects on parental body composition: preliminary evidence from the guelph family health study. BMC Obes. (2019) 6:10. doi: 10.1186/s40608-019-0231-y
162. Skjåkødegård HF, Danielsen YS, Morken M, Linde SRF, Kolko RP, Balantekin KN, et al. Study protocol: a randomized controlled trial evaluating the effect of family-based behavioral treatment of childhood and adolescent obesity–The FABO-study. BMC Public Health. (2016) 16:1106. doi: 10.1186/s12889-016-3755-9
163. Hall KD, Kahan S. Maintenance of lost weight and long-term management of obesity. Med Clin North Am. (2018) 102:183–97. doi: 10.1016/j.mcna.2017.08.012
164. Hall KD. Diet vs. exercise in “the biggest loser” weight loss competition. Obesity. (2013) 21:957–9. doi: 10.1002/oby.20065
165. Lecoultre V, Ravussin E, Redman LM. The fall in leptin concentration is a major determinant of the metabolic adaptation induced by caloric restriction independently of the changes in leptin circadian rhythms. J Clin Endocrinol Metabol. (2011) 96:E1512–E516. doi: 10.1210/jc.2011-1286
166. Kaur KK, Allahbadia G, Singh M. Childhood obesity: a comprehensive review of epidemiology, aetiopathogenesis and management of this global threat of the 21st century. Acta Sci Paediatr. (2019) 2:56–66. doi: 10.31080/ASPE.2019.02.0132
167. Crimmins NA, Xanthakos SA. Obesity. in Neinstein's Adolescent and Young Adult Health, Guide. Philadelphia, PA: Wolters Kluwer (2016). p. 295–300.
168. Astrup A, Rossner S, Van Gaal L, Rissanen A, Niskanen L, Al Hakim M, et al. Effects of liraglutide in the treatment of obesity: a randomized, double-blind, placebo-controlled study. Lancet. (2009) 374:1606–16. doi: 10.1016/S0140-6736(09)61375-1
169. Monami M, Dicembrini I, Marchionni N, Rotella CM, Mannucci E. Effects of glucagon-like peptide-1 receptor agonists on body weight: a meta-analysis. Exp Diabetes Res. (2012) 2012:672658. doi: 10.1155/2012/672658
170. Pi-Sunyer X, Astrup A, Fujioka K, Greenway F, Halpern A, Krempf, et al. A randomized, controlled trial of 3.0 mg of liraglutide in weight management. N Engl J Med. (2015) 373:11–22 . doi: 10.1056/NEJMoa1411892
171. Kelly AS, Auerbach P, Barrientos-Perez M, Gies I, Hale PM, Marcus C, et al. A randomized, controlled trial of liraglutide for adolescents with obesity. N Engl J Med. (2020) 382:2117–28. doi: 10.1056/NEJMoa1916038
172. Foster BA, Escaname E, Powell T, Larsen B, Siddiqui SK, Menchaca J, et al. Randomized controlled trial of DHA supplementation during pregnancy: child adiposity outcomes. Nutrients. (2017) 9:566. doi: 10.3390/nu9060566
173. Abenavoli L, Scarpellini E, Colica C, Boccuto L, Salehi B, Sharifi-Rad J, et al. Gut microbiota and obesity: a role for probiotics. Nutrients. (2019) 11:2690. doi: 10.3390/nu11112690
174. Vajro P, Mandato C, Veropalumbo C, De Micco I. Probiotics: a possible role in treatment of adult and pediatric nonalcoholic fatty liver disease. Ann Hepatol. (2013) 12:161–63. doi: 10.1016/S1665-2681(19)31401-2
175. Zhao L, Fang X, Marshall M, Chung S. Regulation of obesity and metabolic complications by gamma and delta tocotrienols. Molecules. (2016) 21:344. doi: 10.3390/molecules21030344
176. Wong SK, Chin K-Y, Suhaimi FH, Ahmad F, Ima-Nirwana S. Vitamin E as a potential interventional treatment for metabolic syndrome: evidence from animal and human studies. Front Pharmacol. (2017) 8:444. doi: 10.3389/fphar.2017.00444
177. Galli F, Azzi A, Birringer A, Cook-Mills JM, Eggersdorfer M, Frank J, et al. Vitamin E: Emerging aspects and new directions. Free Radic Biol Med. (2017) 102:16–36. doi: 10.1016/j.freeradbiomed.2016.09.017
178. Galmés S, Serra F, Palou A. Vitamin E metabolic effects and genetic variants: a challenge for precision nutrition in obesity and associated disturbances. Nutrients. (2018) 10:1919. doi: 10.3390/nu10121919
179. Ahn SM. Current issues in bariatric surgery for adolescents with severe obesity: durability, complications, and timing of intervention. J. Obes Metabol Syndrome. (2020) 29:4–11. doi: 10.7570/jomes19073
180. Lamoshi A, Chernoguz A, Harmon CM, Helmrath M. Complications of bariatric surgery in adolescents. Semin Pediatr Surg. (2020) 29:150888. doi: 10.1016/j.sempedsurg.2020.150888
181. Weiss AL, Mooney A, Gonzalvo JP. Bariatric surgery. Adv Pediatr. (2017) 6:269–83. doi: 10.1016/j.yapd.2017.03.005
182. Stanford FC, Mushannen T, Cortez P, Reyes KJC, Lee H, Gee DW, et al. Comparison of short and long-term outcomes of metabolic and bariatric surgery in adolescents and adults. Front Endocrinol. (2020) 11:157. doi: 10.3389/fendo.2020.00157
183. Inge TH, Zeller MH, Jenkins TM, Helmrath M, Brandt ML, Michalsky MP, et al. Perioperative outcomes of adolescents undergoing bariatric surgery: the teen-longitudinal assessment of bariatric surgery (Teen-LABS) study. JAMA Pediatr. (2014) 168:47–53. doi: 10.1001/jamapediatrics.2013.4296
184. Järvholm K, Bruze G, Peltonen M, Marcus C, Flodmark CE, Henfridsson P, et al. 5-year mental health and eating pattern outcomes following bariatric surgery in adolescents: a prospective cohort study. Lancet Child AdolescHealth. (2020) 4:210–9. doi: 10.1016/S2352-4642(20)30024-9
185. Xanthakos SA. Bariatric surgery for extreme adolescent obesity: indications, outcomes, and physiologic effects on the gut–brain axis. Pathophysiology. (2008) 15:135–46. doi: 10.1016/j.pathophys.2008.04.005
186. Zitsman JL, Digiorgi MF, Kopchinski JS, Sysko R, Lynch L, Devlin M, et al. Adolescent Gastric Banding: a five-year longitudinal study in 137 individuals. Surg Obes Relat Dis. (2018) 14. doi: 10.1016/j.soard.2018.09.030
187. Inge TH, Jenkins TM, Xanthakos SA, Dixon JB, Daniels SR, Zeller MH, et al. Long-term outcomes of bariatric surgery in adolescents with severe obesity (FABS-5+). A prospective follow-up analysis. Lancet Diabet Endocrinol. (2017) 5:165–73. doi: 10.1016/S2213-8587(16)30315-1
Keywords: obesity, childhood, review (article), behavior, adolescent
Citation: Kansra AR, Lakkunarajah S and Jay MS (2021) Childhood and Adolescent Obesity: A Review. Front. Pediatr. 8:581461. doi: 10.3389/fped.2020.581461
Received: 08 July 2020; Accepted: 23 November 2020;
Published: 12 January 2021.
Edited by:
Yen-Hsuan Ni, National Taiwan University, TaiwanReviewed by:
Claudia Mandato, AORN Santobono-Pausilipon, ItalyHuey-Ling Chen, National Taiwan University Hospital, Taiwan
Copyright © 2021 Kansra, Lakkunarajah and Jay. This is an open-access article distributed under the terms of the Creative Commons Attribution License (CC BY). The use, distribution or reproduction in other forums is permitted, provided the original author(s) and the copyright owner(s) are credited and that the original publication in this journal is cited, in accordance with accepted academic practice. No use, distribution or reproduction is permitted which does not comply with these terms.
*Correspondence: Alvina R. Kansra, YWthbnNyYSYjeDAwMDQwO21jdy5lZHU=