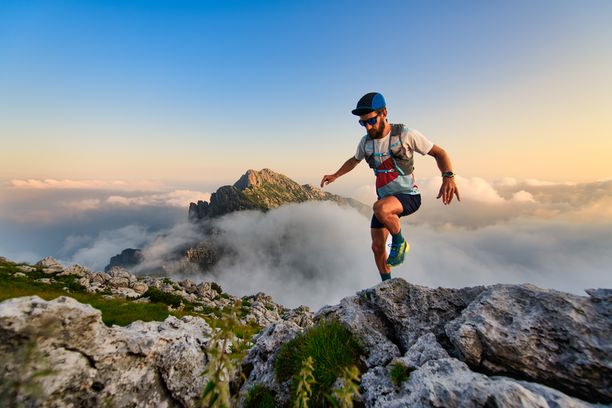
94% of researchers rate our articles as excellent or good
Learn more about the work of our research integrity team to safeguard the quality of each article we publish.
Find out more
ORIGINAL RESEARCH article
Front. Pediatr., 27 May 2020
Sec. Pediatric Cardiology
Volume 8 - 2020 | https://doi.org/10.3389/fped.2020.00267
This article is part of the Research TopicHighlights in Pediatric Cardiology: 2021View all 15 articles
Objectives: 1 Measure serial serum intestinal fatty acid binding protein levels in infants undergoing cardiac surgery with cardiopulmonary bypass to evaluate for evidence of early post-operative enterocyte injury. 2 Determine the association between immediate post-operative circulating intestinal fatty acid binding protein levels and subsequent development of necrotizing enterocolitis.
Design: Observational cohort study. Intestinal fatty acid binding protein was measured pre-operatively, at rewarming, and at 6 and 24 h post-operatively. Percent of goal enteral kilocalories on post-operative day 5 and episodes of necrotizing enterocolitis were determined. Multivariable analysis assessed for factors independently associated with clinical feeding outcomes and suspected/definite necrotizing enterocolitis.
Setting: Quaternary free-standing children's hospital pediatric cardiac intensive care unit.
Patients: 103 infants <120 days of age undergoing cardiothoracic surgery with cardiopulmonary bypass.
Interventions: None.
Results: Median pre-operative intestinal fatty acid binding protein level was 3.93 ng/ml (range 0.24–51.32). Intestinal fatty acid binding protein levels rose significantly at rewarming (6.35 ng/ml; range 0.54–56.97; p = 0.008), continued to rise slightly by 6 h (6.57 ng/ml; range 0.75–112.04; p = 0.016), then decreased by 24 h (2.79 ng/ml; range 0.03–81.74; p < 0.0001). Sixteen subjects (15.7%) developed modified Bell criteria Stage 1 necrotizing enterocolitis and 9 subjects (8.8%) developed Stage 2 necrotizing enterocolitis. Infants who developed necrotizing enterocolitis demonstrated a significantly higher distribution of intestinal fatty acid binding protein levels at both 6 h (p = 0.005) and 24 h (p = 0.005) post-operatively. On multivariable analysis, intestinal fatty acid binding protein was not associated with percentage of goal enteral kilocalories delivered on post-operative day 5. Higher intestinal fatty acid binding protein was independently associated with subsequent development of suspected/definite necrotizing enterocolitis (4% increase in odds of developing necrotizing enterocolitis for each unit increase in intestinal fatty acid binding protein; p = 0.0015).
Conclusions: Intestinal fatty acid binding protein levels rise following infant cardiopulmonary bypass, indicating early post-operative enterocyte injury. Intestinal fatty acid binding protein was not associated with percent of goal enteral nutrition achieved on post-operative day 5, likely due to protocolized feeding advancement based on clinically observable factors. Higher intestinal fatty acid binding protein at 6 h post-operatively was independently associated with subsequent development of necrotizing enterocolitis and may help identify patients at risk for this important complication.
Infants undergoing surgery for congenital heart disease (CHD) with cardiopulmonary bypass (CPB) are at high risk for intestinal injury, both during the surgery itself and subsequently from ongoing post-operative low cardiac output (1, 2). This intestinal injury can lead to major postoperative complications including intestinal barrier dysfunction, dysmotility, post-operative feeding intolerance, and post-operative necrotizing enterocolitis (NEC) (3–13). NEC, in particular, is a dangerous complication. Comorbid CHD and NEC have been shown to have high overall mortality (50–57%) (14, 15) and significantly increased length of hospitalization compared to CHD alone (36 vs. 19 days) (16). At this time, no clinically available biomarker exists to help identify specific intestinal damage following infant cardiac surgery.
Intestinal epithelial injury can be assessed by minimally invasive testing of intestinal fatty acid-binding protein (IFABP). IFABP is a 15-kD cytosolic protein localized mainly to mature enterocytes of the small intestinal villi with normally low circulating levels (17–20). IFABP plasma concentration has been shown to correlate to intestinal epithelial injury in animal models (21, 22). Much of the recent research involving IFABP has been as a predictor of NEC in the neonatal population (23–25), but it has also been utilized as an indicator of intestinal injury across many different contexts including cardiac surgery in both adults and children (3, 26–30). Previous studies performed in mixed age cohorts of children undergoing surgery for CHD indicate that IFABP levels rise immediately following surgery and then fall to below preoperative levels in the subsequent recovery period (3, 26). However, no previous studies have looked at IFABP levels in specifically the infant CHD population, which is a unique and important age group given the complexity of the surgeries performed at this age and the risk for diffuse organ injury. Additionally, associations between IFABP levels and feeding tolerance or clinical suspicion of NEC have not been assessed in this high-risk population.
In this study, we sought to describe the pattern of serum IFABP in infants undergoing CPB. In addition, we aimed to determine the clinical risk factors associated with elevated preoperative and postoperative IFABP levels, hypothesizing that higher IFABP levels would be associated with worse clinical severity of disease. Finally, we investigated whether there was an association between IFABP and early enteral feeding outcomes/development of NEC, hypothesizing that greater elevation of IFABP levels in the immediate post-operative period would predict worse enteral feeding outcomes and greater odds of developing NEC.
This study was a pre-specified secondary aim of a prospective cohort study evaluating alkaline phosphatase activity after infant CPB (31), with prospective collection of samples for IFABP analysis and post-hoc analysis of infant feeding outcomes. Over a period of two-and-a-half years from September 2013 to February 2016, infants ≤ 120 days at time of cardiothoracic surgery with use of CPB were enrolled. Exclusion criteria were weight <2 kg (as limited blood volume could increase risk of anemia with research blood draws), adjusted gestation age <34 weeks (given concerns for altered biomarker production), and prior participation in this protocol. The protocol was approved by the Colorado Multiple Institution Review Board, and informed consent was obtained from the subjects' parents prior to enrollment. The primary aims of the current study were to measure pre- and post-op IFABP levels in an infant cohort undergoing CPB and determine the association between IFABP levels and early enteral feeding outcomes and development of NEC.
As previously published (32), CPB was performed using a neonatal circuit consisting of a roller head pump (S5, LivaNova, Arvada, CO, USA) and a Terumo FX05 oxygenator with a blood prime. The blood prime routinely underwent pre-bypass hemofiltration using a Minntech Hemocor HPH Junior hemoconcentrator (Medivators Inc., Minneapolis, MN, USA) with a polysulfone membrane prior to initiating bypass, allowing for partial filtration of molecules up to 65,000 Daltons. Anticoagulation was achieved prior to CPB by administering 500 units/kg of heparin systemically to the patient. Initial target flow rate was ~200 ml/kg/minute. Cardioplegia was accomplished using del Nido formula cardioplegia solution at an initial dose of 30 ml/kg and subsequent dosing was considered after 60 min of aortic cross-clamp time. Per our clinical protocol, all neonates (age <1 month) received high dose methylprednisolone (10 mg/kg) at 10 and 4 h prior to surgery to attempt to reduce post-CPB systemic inflammation.
IFABP levels were obtained pre-operation, immediately prior to withdrawal of CPB, and 6 and 24-h post-operation. Serum concentration of IFABP was analyzed at the University of Colorado, Denver using Meso Scale Discovery (MSD) multiplex immunoassay system, Meso Scale Diagnostics, LLC, Gaithersburg, MD.
Pre-operative, intraoperative, and post-operative variables were collected to evaluate for potential risk factors for pre- and post-operative intestinal damage. Pre-operative variables included age, weight, sex, Aristotle score (comprehensive and basic) (33), pre-operative mechanical ventilation, pre-operative inotropic support, pre-operative initiation of enteral feeding, prostaglandin use, single ventricle physiology, and prematurity. Intraoperative and post-operative variables included CPB time, cross-clamp time, deep hypothermic circulatory arrest time, selective cerebral perfusion time, duration of mechanical ventilation, vasoactive inotropic score (VIS) (34, 35) at 6-h post-operative, and clinically obtained peak creatinine and peak lactate levels.
Diagnosis and staging of NEC was made based on the modified Bell's Staging Criteria via retrospective chart review (36). Although the Bell Staging Criteria are designed primarily for NEC associated with prematurity, they remain the primary diagnostic criteria for cardiac-associated NEC (16, 37–39). Only post-operative cases of NEC during the same hospitalization as the operation were included.
A post-hoc data collection from the electronic medical record was utilized to gather nutrition and feeding data. We examined the primary feeding outcome of percent of goal feeds achieved enterally on post-op day 5. Additionally, we examined secondary outcomes of percent of goal feeds achieved on post-op day 3. To calculate the percent of goal feeds achieved enterally, we took the patient's achieved enteral feeds (in kcals) as recorded in the patient's electronic medical record flowsheet and divided this by the goal kcals as documented daily by the cardiac intensive care unit dieticians. The feeding protocol utilized in our CICU is included as Supplemental Figure 1.
Patients' demographics and clinical characteristics are summarized using descriptive statistics. The comparison groups were divided by IFABP level below and equal or above 50th percentile at the given time point. The distributions of continuous variables were inspected before data analysis, all the continuous variables were summarized with median and range (min and max). Wilcoxon rank sum test was conducted to determine the significant difference in distributions between the comparison groups. The categorical data was summarized as count and percentage for the “Yes” category for a giving measurement. To model the association between percentage of goal enteral kilocalories achieved and IFABP, multivariable general linear model was used with pre-selected clinical variables as covariates. Backwards model selection strategy was used, and R-square and type III P-values were used to evaluate the most harmonious model. Multivariable logistic regression model was performed to assess the association between NEC and IFABP, demographic and clinical covariates were selected through univariate logistic regression with area under curve (AUC) >0.6, then added to the multivariable model. Akaike information criterion (AIC) was used to evaluate the best fitting model. P-values < 0.05 were considered as statistically significant. All statistical analysis was performed using SAS version 9.4 (SAS Institute, Cary, NC) and all plots were produced with GraphPad Prism version 8.
The raw data supporting the conclusions of this manuscript will be made available by the authors, without undue reservation, to any qualified researcher.
The study consecutively enrolled 100 and two subjects, plus one screen failure (converted to non-bypass surgery after enrollment). Of these subjects, one patient did not have a preoperative IFABP level, one patient did not have an IFABP level at rewarming, three patients did not have a 6 h IFABP level, and two patients did not have a 24 h IFABP level due to insufficient sample volume, leaving a total of 101 preoperative and rewarming samples, 99 six hour samples, and 100 twenty-four hour samples (99% overall collection rate). Table 1 lists the cardiac diagnoses and the operations performed. Clinical characteristics for this cohort are shown in Table 2.
Table 2. Baseline clinical characteristics (described in full cohort column) and comparison of clinical characteristics between infants with lower (<50th percentile) and higher (>50th percentile) pre-operative IFABP levels.
The distribution of IFABP levels initially rose at rewarming, increased slightly at 6 h, and by 24 h had trended down to near preoperative levels as shown in Figure 1. The association between clinical characteristics and IFABP levels pre-operatively are shown in Table 2. Pre-operative clinical characteristics found to be significantly associated with higher IFABP include older age, higher weight, prior initiation of pre-operative enteral nutrition, and lack of prostaglandin use. The association of operative and post-operative variables and IFABP levels at 6 h and at 24 h are shown in Table 3. The only variable found to be significantly associated with higher IFABP at 6-h post-operative was higher vasopressor-inotropic score (VIS). At 24 h, single ventricle physiology, longer CPB time, longer duration of mechanical ventilation, higher VIS, and higher peak creatinine were all found to be significantly associated with higher IFABP.
Figure 1. IFABP levels (ng/ml) shown over time at 4 different time points: pre-operative, rewarming, 6 h post-operative, and 24 h post-operative.
Table 3. Comparison of baseline, intra-operative, and post-operative characteristics between infants with lower (<50th percentile) and higher (>50th percentile) post-operative IFABP levels at 6 and 24 h.
The median number of days from surgery to NEC onset was 10 days (IQR of 4–14.5; range 1–52). The association between clinical characteristics and post-operative NEC are shown in Table 4. Clinical characteristics found to be significantly associated with post-operative NEC include lower weight, longer deep hypothermic circulatory arrest time, longer duration of mechanical ventilation, higher VIS, higher peak lactate, and higher peak creatinine.
Table 4. Comparison of baseline, intra-operative, and post-operative characteristics between infants without NEC or with NEC as defined by bell stage 1 or Stage 2.
Infants steadily progressed in terms of amount of enteral feeds achieved over time, with mean percent of goals kcals achieved by enteral nutrition advancing from 26% on post-op day 1, to 48% on post op day 3, to 71% on post-op day 5, to 82% on post-op day 7. On multivariable analysis, IFABP was not associated with percentage of goal enteral kcal achieved. Rather, selective cerebral perfusion time, higher Aristotle score, longer intubation time, and the need for pre-operative intubation were all independently associated with lower percentage of goal Kcals given enterally on post-op day 5, as shown in Table 5. Higher Aristotle score, longer length of intubation, need for pre-operative intubation, lower weight, and higher VIS at 24 h were independently associated with a lower percentage of goal Kcals given enterally on post-operative day 3 (Table 5).
Of our patients, by modified Bell Staging Criteria, 75.5% (n = 77) were categorized as Stage 0, 15.7% (n = 16) were categorized as Stage 1 (suspected NEC), and 8.8% (n = 9) were categorized as Stage 2 (definite NEC). We did not have any patients categorized as Stage 3 (advanced NEC). None of the patients in our study had previous clinical NEC, though prior subclinical intestinal stress cannot be completely ruled out by this study design. A comparison of the distribution of IFABP levels at 6 and 24 h demonstrates a significantly higher distribution of IFABP levels at both time points in patients who subsequently developed suspected or definite NEC vs. patients who did not develop post-operative NEC (Figure 2). On multivariable analysis, IFABP and peak creatinine were independently associated with development of suspected or definite NEC, as shown in Table 6. Of note, both age and weight were initially included in our multivariable model for predicting IFABP, but they were removed in backwards selection, as they did not demonstrate a significant association with NEC when controlling for other independent variables in the model. While not significantly independently associated with development of suspected or definite NEC, VIS at 24 h improved the overall fit of the model and was therefore included.
Figure 2. Comparison of early IFABP levels (6 and 24 h) in patients without NEC vs. with suspected or definite NEC (Modified Bell Criteria scores 1 or 2).
Table 6. Multivariable model for predicting suspected or definite NEC (modified bell criteria scores 1 or 2).
In this study, we present the first evaluation of IFABP levels following cardiothoracic surgery with CPB in a specifically infant population. Additionally, we present the associations between clinical factors and pre-operative and post-operative IFABP levels. Counter to our initial hypothesis, immediate post-operative IFABP levels were not found to be associated with the percentage of goal kcals achieved enterally on post-operative day 3 or 5; rather, clinician-observable factors indicating illness severity were associated with achieved enteral kcals. We present the novel finding of an independent association in infants undergoing CPB between early enterocyte injury, as identified by circulating IFABP levels, and subsequent post-operative NEC.
We show that IFABP levels rise immediately after CPB, continue to rise at 6 h, and then return back to pre-operative levels by 24 h after surgery. A physiologic understanding of IFABP offers an explanation for this pattern of early rise and rapid fall. IFABP is located predominantly in the mature enterocytes in the villi as opposed to younger enterocytes in the crypts (20). These mature enterocytes are at the distal extreme of mucosal blood flow and therefore susceptible to early ischemic damage in the setting of compromised intestinal blood flow, consequently leading to a spike in systemic IFABP (20). IFABP is eliminated renally (40), resulting in a downtrend to pre-operative levels once adequate intestinal perfusion is restored. Prior studies in mixed-age pediatric cardiac surgery cohorts demonstrated a similar pattern of rise and fall in IFABP with comparable magnitudes of IFABP elevation (3, 26). Adult cardiac surgery cohorts have also shown this pattern of rise and fall in IFABP, but with smaller magnitudes of IFABP elevation (27–30, 41). This discrepancy in magnitude of IFABP elevation would be potentially explained by the differences in GI complication rates in pediatric patients vs. adult patients after cardiac surgery, with pediatric rates of just NEC ranging from 3.3 to 13% (42) and adult rates of any GI complication ranging from 0.3 to 2% (43). The early rise in IFABP is particularly notable, as early rise in IFABP and the associated intestinal barrier injury has been associated with a post-bypass inflammatory cascade (26). Normal IFABP levels are not well-defined in the infant population, and future studies would ideally include age-matched controls.
Higher pre-operative IFABP levels were associated with increased patient age, increased patient weight, prior initiation of pre-operative nutrition, and no prior prostaglandin use. The association of IFABP with age is both a pronounced and an interesting finding, as previously Pathan et al. (3) found no association between age or weight and IFABP levels. A potential explanation for this discrepancy is that during gestation and early infancy, IFABP levels rise as gut mass increases and IFABP accumulates. Pathan et al. (3) examined an older population (median age was 11.2 months as opposed to our median of 21.5 days) and more heterogeneous age range, and therefore potentially did not capture the initial rise in IFABP during infancy. In support of this explanation, Guthman et al. (44) has demonstrated an association between birth weight and IFABP in premature infants <33 weeks gestation age, and our data suggests that this trend may continue during early infancy as well. Examining normal IFABP levels during the infancy period would be helpful to further clarify the natural trajectory of IFABP. Likely the finding of pre-operative nutrition being associated with higher IFABP levels is collinear with the age and weight, as older patients are more likely to already have nutrition started. Another interesting finding was that prostaglandin use was associated with lower levels of IFABP, which is in contrast to a previous finding in Pathan et al. (3), who demonstrated increased IFABP in patients with ductal dependent lesions. Again, this discrepancy is most likely explained by the difference in populations: In our younger population, patients requiring prostaglandins were younger than the rest of our population and therefore the effect of their age on IFABP likely outweighed the effect of increased disease severity and decreased gut perfusion due to their ductal dependent lesions. Similarly, other indications of pre-operative disease severity (pre-operative mechanical ventilation, pre-operative inotropic support, single ventricle physiology) were also likely outweighed by the effect of age, as the more severe patients were also likely to go for their surgery at a younger age. Knowing which factors are associated with higher pre-operative IFABP is helpful in further understanding IFABP and how to properly interpret its levels.
Higher post-operative IFABP levels were associated with longer CPB time, higher VIS, higher peak creatinine, single ventricle physiology, and duration of mechanical ventilation. Increased VIS has been prospectively shown to be associated with longer length of intubation, ICU stay, and hospital stay (35), and its correlation with higher IFABP could be due to its association with disease severity. Additionally, the physiologic state driving high VIS score (hypotension, decreased cardiac output, poor perfusion) would also likely lead to poor mesenteric blood flow, which would then be expected to cause more enterocyte injury and higher IFABP levels. A direct effect of vasoactive inotropes on mesenteric blood flow could be contributing as well, as there is evidence that vasopressin and epinephrine decrease mesenteric blood flow in both humans and (more extensively) in animal models of critical illness (45–50). Creatinine reflects perfusion of another abdominal organ, and so a physiologic state of hypoperfusion would intuitively affect both similarly. Additionally, since IFABP is renally cleared, acute kidney injury would be expected to potentiate elevated serum IFABP levels (40). Patient with single ventricle physiology have been shown to have low postprandial blood flow velocities (51), and therefore it is not surprising that higher levels of IFABP are seen in these patients. Longer CPB time's association with higher IFABP levels could potentially be due to insufficient flow during CPB, the continuous nature of flow during CPB, or it could be another surrogate for disease severity. This association has previously been documented in in adult and pediatric populations undergoing CPB (26, 41). Finally, duration of mechanical ventilation would indicate worsening disease severity and also lead to increased central pressures, venous congestion, and subsequent intestinal edema and injury. Alternatively, the intestinal injury and subsequent endotoxin leak could cause direct lung injury and cause longer duration of mechanical ventilation (52, 53). Our data confirm the previous finding in Typpo et al. (26) that CPB time and vasopressor use are associated with higher post-operative IFABP, but the remainder of the associations are novel in a pediatric population. It is of further note that none of our clinical factors found to be significantly associated with pre-operative IFABP were significantly associated with post-operative IFABP. This difference indicates that the previously discussed operative and post-operative variables outweigh preoperative variables and exemplifies the high degree of stress that cardiac surgery with CPB places on the intestine. Knowing which clinical factors are associated with higher IFABP levels can potentially help predict which infants are at higher risk for intestinal damage and its clinical sequelae.
We did not find a significant association between IFABP levels and early enteral feeding initiation/advancement, as measured by percentage of goal enteral kcal achieved by post-operative days 3 or 5. Our lack of a significant association is in contrast to the study by Typpo et al. (26) which does show an association with clinical feeding outcomes, though interestingly demonstrating that lower IFABP levels were associated with more feeding dysfunction. This discrepancy could potentially be explained by the fact that younger infants based on our data would have lower levels of IFABP based purely on age and decreased intestinal mass compared to older pediatric patients, confounding the association with feeding dysfunction.
We did show that higher Aristotle score, longer selective cerebral perfusion time, the need for pre-operative intubation, longer post-operative intubation time, and higher VIS were all associated with slower enteral feeding advances. A prior study by Alten et al., using the Pediatric Cardiac Critical Care Consortium registry to examine perioperative feeding management showed a wide variety of clinical practice surrounding feeding advancements (8), highlighting the uncertainty of perioperative feeding. The authors demonstrated a delay in initiation of post-operative feeds in hypoplastic left heart syndrome patients, who typically require longer duration of mechanical ventilation and vasoactive medications, although only univariate analysis was performed (8). Our findings on multivariable analysis are consistent with Alten's study, identifying independent associations between both surgical complexity (Aristotle scores) and post-operative clinical illness (mechanical ventilation and VIS) and delayed provision of post-operative enteral nutrition.
In both our study and the study by Alten et al., the variables found to be associated with enteral feeding initiation/advancement were known to the clinicians making the decisions regarding the speed of enteral feed advances. This finding is not surprising, as decisions to initiate and advance enteral nutrition in the early post-operative period generally hinge on observable physiologic markers and clinically visible risk factors. Whether an infant will tolerate advancing nutrition is more difficult to assess, as evidenced by the findings of Lannucci et al., who elegantly demonstrated similar clinical characteristics among infants who were initiated on post-operative enteral nutrition and did or did not subsequently proceed to develop NEC (38). Therefore development of novel biomarkers could be useful as a supplement to clinical risk factors in the assessment of readiness to feed and tolerance of enteral nutrition, particularly with the current movement toward early enteral feeding after congenital heart surgery and protocolized feeding advances (54, 55). Ideally, candidate biomarkers would provide an early signal that could be detected prior to initiation of post-operative enteral nutrition and be predictive of clinically significant feeding intolerance, especially development of NEC.
In this study, we demonstrate for the first time in CHD patients an independent association between early IFABP levels and subsequent development of suspected or definite post-operative NEC. This association is consistent with prior studies in the premature neonate population (23–25, 56). Specifically, our data suggest that infants with immediate post-operative enterocyte injury, as evidenced by higher circulating IFABP at 6 h post-operatively, are at increased risk of subsequent development of suspected or definite NEC. The early appearance of IFABP in the blood (prior to the time enteral nutrition would be initiated in our post-operative infants) increases its potential value for risk stratification for timing of enteral nutrition initiation as well as speed of enteral nutrition advancement. The mechanism linking early enterocyte injury with subsequent development of NEC remains unclear. It is possible that early enterocyte injury is simply a marker for patients with an underlying predisposition for recurrent injury based on their cardiovascular or intestinal physiology, but who then have an intervening period of healing. Alternatively, it is possible that the early enterocyte injury results in an ongoing local process that does not lead to the persistent presence of circulating IFABP but remains incompletely healed. Translational animal modeling would be helpful in differentiating these two potential mechanisms. Finally, while our study was not designed to evaluate the longitudinal use of IFABP in early detection of NEC, there is growing body of evidence supporting IFABP use for this purpose in premature infants (23–25, 57), making this a promising and worthwhile target for future investigations in the CHD population.
Our study has several limitations. The subjects were recruited from a single institution, limiting generalizability to other centers. Additionally, while limited to infants, this study incorporated a range of congenital defects and subsequently a range of clinical severity. A large multi-institutional study would better be able to account for variances in disease process and clinical severity. The study was designed to only look at pre- and immediate post-operative IFABP, and did not look at ongoing IFABP levels, which would help better establish the temporal relationship between serum IFABP and NEC, as well as its potential utility as a point-of-care biomarker. Finally, the modified Bell Staging Criteria for NEC is imperfect in its application in the cardiac population, as it was designed primarily for the neonatal population. In particular, it is very challenging to differentiate post-operative ileus from NEC, as there is significant overlap between the radiographic and gastrointestinal findings in these two disease processes.
IFABP levels rise initially following surgery with CPB in infants with CHD, then begin to fall by 24 h. IFABP levels were not associated with the clinical feeding outcome of percent of goal kcals achieved enterally on post-operative day 5. Instead, the primary predictors of clinical feeding outcomes were clinically observable factors. IFABP at 6 h was independently associated with subsequent development of suspected or definite NEC and may be useful for identifying patients at risk for development of post-operative NEC.
The datasets generated for this study are available on request to the corresponding author.
The studies involving human participants were reviewed and approved by Colorado Multiple Institution Review Board. Written informed consent to participate in this study was provided by the participants' legal guardian/next of kin.
JW, PW, and JD conceived the design of the study. TU and JD performed patient recruitment. JW and TU performed data extraction. LK and JD performed IFABP analysis. JW, ST, JZ, and JD performed the data analysis and interpretation. JW and JD wrote the primary manuscript. All authors were responsible for reviewing and approving the final version of the manuscript.
This study was supported by National Institute of Health/National Heart, Lung, and Blood Institute 1K23HL123634 (PI Davidson), American Heart Association 13CRP17300016 (PI Davidson), and National Institute of Health/National Center for Advancing Translational Sciences Colorado Clinical and Translational Science Awards Grant Number UL1 TR001082 (University of Colorado). Contents are the authors' sole responsibility and do not necessarily represent official National Institute of Health views.
The authors declare that the research was conducted in the absence of any commercial or financial relationships that could be construed as a potential conflict of interest.
We would like to acknowledge the contributions of the nurses in the cardiac intensive care unit and the Clinical and Translational Research Center, without whom our study would not have been possible.
The Supplementary Material for this article can be found online at: https://www.frontiersin.org/articles/10.3389/fped.2020.00267/full#supplementary-material
1. Kaufman J, Almodovar MC, Zuk J, Friesen RH. Correlation of abdominal site near-infrared spectroscopy with gastric tonometry in infants following surgery for congenital heart disease. Pediatr Crit Care Med. (2008) 9:62–8. doi: 10.1097/01.PCC.0000298640.47574.DA
2. Booker PD, Romer H, Franks R. Gut mucosal perfusion in neonates undergoing cardiopulmonary bypass. Br J Anaesth. (1996) 77:597–602. doi: 10.1093/bja/77.5.597
3. Pathan N, Burmester M, Adamovic T, Berk M, Ng KW, Betts H, et al. Intestinal injury and endotoxemia in children undergoing surgery for congenital heart disease. Am J Respir Crit Care Med. (2011) 184:1261–9. doi: 10.1164/rccm.201104-0715OC
4. Malagon I, Onkenhout W, Klok G, van der Poel PF, Bovill JG, Hazekamp MG. Gut permeability in paediatric cardiac surgery. Br J Anaesth. (2005) 94:181–5. doi: 10.1093/bja/aei014
5. Sondheimer JM, Hamilton JR. Intestinal function in infants with severe congenital heart disease. J Pediatr. (1978) 92:572–8. doi: 10.1016/S0022-3476(78)80290-X
6. Cabrera AG, Prodhan P, Bhutta AT. Nutritional challenges and outcomes after surgery for congenital heart disease. Curr Opin Cardiol. (2010) 25:88–94. doi: 10.1097/HCO.0b013e3283365490
7. Natarajan G, Reddy Anne S, Aggarwal S. Enteral feeding of neonates with congenital heart disease. Neonatology. (2010) 98:330–6. doi: 10.1159/000285706
8. Alten JA, Rhodes LA, Tabbutt S, Cooper DS, Graham EM, Ghanayem N, et al. Perioperative feeding management of neonates with CHD: analysis of the pediatric cardiac critical care consortium (PC4) registry. Cardiol Young. (2015) 25:1593–601. doi: 10.1017/S1047951115002474
9. Rodgers BM, Hollenbeck JI, Donnelly WH, Talbert JL. Intrahepatic cholestasis with parental alimentation. Am J Surg. (1976) 131:149–55. doi: 10.1016/0002-9610(76)90088-X
10. Postuma R, Trevenen CL. Liver disease in infants receiving total parenteral nutrition. Pediatrics. (1979) 63:110–5.
11. Kelly DA. Liver complications of pediatric parenteral nutrition–epidemiology. Nutrition. (1998) 14:153–7. doi: 10.1016/S0899-9007(97)00232-3
12. Jacobi SK, Odle J. Nutritional factors influencing intestinal health of the neonate. Adv Nutr. (2012) 3:687–96. doi: 10.3945/an.112.002683
13. Veenstra M, Danielson L, Brownie E, Saba M, Natarajan G, Klein M. Enteral nutrition and total parenteral nutrition components in the course of total parenteral nutrition-associated cholestasis in neonatal necrotizing enterocolitis. Surgery. (2014) 156:578–83. doi: 10.1016/j.surg.2014.04.031
14. Cheng W, Leung MP, Tam PK. Surgical intervention in necrotizing enterocolitis in neonates with symptomatic congenital heart disease. Pediatr Surg Int. (1999) 15:492–5. doi: 10.1007/s003830050647
15. Kessler U, Hau EM, Kordasz M, Haefeli S, Tsai C, Klimek P, et al. Congenital heart disease increases mortality in neonates with necrotizing enterocolitis. Front Pediatr. (2018) 6:312. doi: 10.3389/fped.2018.00312
16. McElhinney DB, Hedrick HL, Bush DM, Pereira GR, Stafford PW, Gaynor JW, et al. Necrotizing enterocolitis in neonates with congenital heart disease: risk factors and outcomes. Pediatrics. (2000) 106:1080–7. doi: 10.1542/peds.106.5.1080
17. Montoudis A, Delvin E, Menard D, Beaulieu JF, Jean D, Tremblay E, et al. Intestinal-fatty acid binding protein and lipid transport in human intestinal epithelial cells. Biochem Biophys Res Commun. (2006) 339:248–54. doi: 10.1016/j.bbrc.2005.10.202
18. Pelsers MM, Namiot Z, Kisielewski W, Namiot A, Januszkiewicz M, Hermens WT, et al. Intestinal-type and liver-type fatty acid-binding protein in the intestine. Tissue distribution and clinical utility. Clin Biochem. (2003) 36:529–35. doi: 10.1016/S0009-9120(03)00096-1
19. Tso P, Nauli A, Lo CM. Enterocyte fatty acid uptake and intestinal fatty acid-binding protein. Biochem Soc Trans. (2004) 32(Pt 1):75–8. doi: 10.1042/bst0320075
20. Pelsers MM, Hermens WT, Glatz JF. Fatty acid-binding proteins as plasma markers of tissue injury. Clin Chim Acta. (2005) 352:15–35. doi: 10.1016/j.cccn.2004.09.001
21. Grootjans J, Thuijls G, Verdam F, Derikx JP, Lenaerts K, Buurman WA. Non-invasive assessment of barrier integrity and function of the human gut. World J Gastrointest Surg. (2010) 2:61–9. doi: 10.4240/wjgs.v2.i3.61
22. Thuijls G, van Wijck K, Grootjans J, Derikx JP, van Bijnen AA, Heineman E, et al. Early diagnosis of intestinal ischemia using urinary and plasma fatty acid binding proteins. Ann Surg. (2011) 253:303–8. doi: 10.1097/SLA.0b013e318207a767
23. Edelson MB, Sonnino RE, Bagwell CE, Lieberman JM, Marks WH, Rozycki HJ. Plasma intestinal fatty acid binding protein in neonates with necrotizing enterocolitis: a pilot study. J Pediatr Surg. (1999) 34:1453–7. doi: 10.1016/S0022-3468(99)90102-1
24. Schurink M, Kooi EM, Hulzebos CV, Kox RG, Groen H, Heineman E, et al. Intestinal fatty acid-binding protein as a diagnostic marker for complicated and uncomplicated necrotizing enterocolitis: a prospective cohort study. PLoS ONE. (2015) 10:e0121336. doi: 10.1371/journal.pone.0121336
25. Schurink M, Scholten IG, Kooi EM, Hulzebos CV, Kox RG, Groen H, et al. Intestinal fatty acid-binding protein in neonates with imminent necrotizing enterocolitis. Neonatology. (2014) 106:49–54. doi: 10.1159/000358582
26. Typpo KV, Larmonier CB, Deschenes J, Redford D, Kiela PR, Ghishan FK. Clinical characteristics associated with postoperative intestinal epithelial barrier dysfunction in children with congenital heart disease. Pediatr Crit Care Med. (2015) 16:37–44. doi: 10.1097/PCC.0000000000000256
27. Camkiran A, Donmez A, Aldemir D, Isguzar RA, Gultekin B. Clinical significance of intestinal type fatty acid binding protein in patients undergoing coronary artery bypass surgery. Anadolu Kardiyol Derg. (2011) 11:536–41. doi: 10.5152/akd.2011.139
28. Holmes JHT, Lieberman JM, Probert CB, Marks WH, Hill ME, Paull DL, et al. Elevated intestinal fatty acid binding protein and gastrointestinal complications following cardiopulmonary bypass: a preliminary analysis. J Surg Res. (2001) 100:192–6. doi: 10.1006/jsre.2001.6237
29. Kano H, Takahashi H, Inoue T, Tanaka H, Okita Y. Transition of intestinal fatty acid-binding protein on hypothermic circulatory arrest with cardiopulmonary bypass. Perfusion. (2016) 32:200–5. doi: 10.1177/0267659116667807
30. Vermeulen Windsant IC, Hellenthal FA, Derikx JP, Prins MH, Buurman WA, Jacobs MJ, et al. Circulating intestinal fatty acid-binding protein as an early marker of intestinal necrosis after aortic surgery: a prospective observational cohort study. Ann Surg. (2012) 255:796–803. doi: 10.1097/SLA.0b013e31824b1e16
31. Davidson JA, Urban TT, Baird C, Tong S, Woodruff A, Twite M, et al. Alkaline phosphatase in infant cardiopulmonary bypass: kinetics and relationship to organ injury and major cardiovascular events. J Pediatr. (2017) 190:49–55.e2. doi: 10.1016/j.jpeds.2017.07.035
32. Persson JN, Baird CH, Tong S, Urban TT, Klawitter J, Wischmeyer PE, et al. Infant cardiopulmonary bypass: CD73 kinetics, association with clinical outcomes, and influence on serum adenosine production capacity. Pediatr Res. (2018) 83:858–65. doi: 10.1038/pr.2017.325
33. Lacour-Gayet F, Clarke D, Jacobs J, Gaynor W, Hamilton L, Jacobs M, et al. The aristotle score for congenital heart surgery. Semin Thorac Cardiovasc Surg Pediatr Card Surg Annu. (2004) 7:185–91. doi: 10.1053/j.pcsu.2004.02.011
34. Gaies MG, Gurney JG, Yen AH, Napoli ML, Gajarski RJ, Ohye RG, et al. Vasoactive-inotropic score as a predictor of morbidity and mortality in infants after cardiopulmonary bypass. Pediatr Crit Care Med. (2010) 11:234–8. doi: 10.1097/PCC.0b013e3181b806fc
35. Davidson J, Tong S, Hancock H, Hauck A, da Cruz E, Kaufman J. Prospective validation of the vasoactive-inotropic score and correlation to short-term outcomes in neonates and infants after cardiothoracic surgery. Intensive Care Med. (2012) 38:1184–90. doi: 10.1007/s00134-012-2544-x
36. Walsh MC, Kliegman RM. Necrotizing enterocolitis: treatment based on staging criteria. Pediatr Clin North Am. (1986) 33:179–201. doi: 10.1016/s0031-395534975-6
37. DeWitt AG, Charpie JR, Donohue JE, Yu S, Owens GE. Splanchnic near-infrared spectroscopy and risk of necrotizing enterocolitis after neonatal heart surgery. Pediatr Cardiol. (2014) 35:1286–94. doi: 10.1007/s00246-014-0931-5
38. Iannucci GJ, Oster ME, Mahle WT. Necrotising enterocolitis in infants with congenital heart disease: the role of enteral feeds. Cardiol Young. (2013) 23:553–9. doi: 10.1017/S1047951112001370
39. Schuchardt EL, Kaufman J, Lucas B, Tiernan K, Lujan SO, Barrett C. Suspected necrotising enterocolitis after surgery for CHD: an opportunity to improve practice and outcomes. Cardiol Young. (2018) 28:639–46. doi: 10.1017/S1047951117002815
40. Lieberman JM, Sacchettini J, Marks C, Marks WH. Human intestinal fatty acid binding protein: report of an assay with studies in normal volunteers and intestinal ischemia. Surgery. (1997) 121:335–42. doi: 10.1016/S0039-6060(97)90363-9
41. Adamik B, Kubler A, Gozdzik A, Gozdzik W. Prolonged cardiopulmonary bypass is a risk factor for intestinal ischaemic damage and endotoxaemia. Heart Lung Circ. (2017) 26:717–23. doi: 10.1016/j.hlc.2016.10.012
42. Iliopoulos I, Branco RG, Brinkhuis N, Furck A, LaRovere J, Cooper DS, et al. Mesenteric near-infrared spectroscopy and risk of gastrointestinal complications in infants undergoing surgery for congenital heart disease. Cardiol Young. (2016) 26:772–80. doi: 10.1017/S1047951115001365
43. D'Ancona G, Baillot R, Poirier B, Dagenais F, de Ibarra JI, Bauset R, et al. Determinants of gastrointestinal complications in cardiac surgery. Tex Heart Inst J. (2003) 30:280–5.
44. Guthmann F, Borchers T, Wolfrum C, Wustrack T, Bartholomaus S, Spener F. Plasma concentration of intestinal- and liver-FABP in neonates suffering from necrotizing enterocolitis and in healthy preterm neonates. Mol Cell Biochem. (2002) 239:227–34. doi: 10.1007/978-1-4419-9270-3_29
45. De Backer D, Creteur J, Silva E, Vincent JL. Effects of dopamine, norepinephrine, and epinephrine on the splanchnic circulation in septic shock: which is best? Crit Care Med. (2003) 31:1659–67. doi: 10.1097/01.CCM.0000063045.77339.B6
46. Di Giantomasso D, Bellomo R, May CN. The haemodynamic and metabolic effects of epinephrine in experimental hyperdynamic septic shock. Intensive Care Med. (2005) 31:454–62. doi: 10.1007/s00134-005-2580-x
47. Martikainen TJ, Tenhunen JJ, Giovannini I, Uusaro A, Ruokonen E. Epinephrine induces tissue perfusion deficit in porcine endotoxin shock: evaluation by regional CO(2) content gradients and lactate-to-pyruvate ratios. Am J Physiol Gastrointest Liver Physiol. (2005) 288:G586–92. doi: 10.1152/ajpgi.00378.2004
48. Meier-Hellmann A, Reinhart K, Bredle DL, Specht M, Spies CD, Hannemann L. Epinephrine impairs splanchnic perfusion in septic shock. Crit Care Med. (1997) 25:399–404. doi: 10.1097/00003246-199703000-00005
49. van Haren FM, Rozendaal FW, van der Hoeven JG. The effect of vasopressin on gastric perfusion in catecholamine-dependent patients in septic shock. Chest. (2003) 124:2256–60. doi: 10.1378/chest.124.6.2256
50. Westphal M, Freise H, Kehrel BE, Bone HG, Van Aken H, Sielenkamper AW. Arginine vasopressin compromises gut mucosal microcirculation in septic rats. Crit Care Med. (2004) 32:194–200. doi: 10.1097/01.CCM.0000104201.62736.12
51. del Castillo SL, Moromisato DY, Dorey F, Ludwick J, Starnes VA, Wells WJ, et al. Mesenteric blood flow velocities in the newborn with single-ventricle physiology: modified Blalock-Taussig shunt versus right ventricle-pulmonary artery conduit. Pediatr Crit Care Med. (2006) 7:132–7. doi: 10.1097/01.PCC.0000200999.89777.92
53. Davidson JA, Urban TT, Tong S, Maddux A, Hill G, Frank BS, et al. Alkaline phosphatase activity and endotoxemia after infant cardiothoracic surgery. Shock. (2019) 51:328–36. doi: 10.1097/SHK.0000000000001162
54. Braudis NJ, Curley MA, Beaupre K, Thomas KC, Hardiman G, Laussen P, et al. Enteral feeding algorithm for infants with hypoplastic left heart syndrome poststage I palliation. Pediatr Crit Care Med. (2009) 10:460–6. doi: 10.1097/PCC.0b013e318198b167
55. del Castillo SL, McCulley ME, Khemani RG, Jeffries HE, Thomas DW, Peregrine J, et al. Reducing the incidence of necrotizing enterocolitis in neonates with hypoplastic left heart syndrome with the introduction of an enteral feed protocol. Pediatr Crit Care Med. (2010) 11:373–7. doi: 10.1097/PCC.0b013e3181c01475
56. Cheng S, Yu J, Zhou M, Tu Y, Lu Q. Serologic intestinal-fatty acid binding protein in necrotizing enterocolitis diagnosis: a meta-analysis. Biomed Res Int. (2015) 2015:156704. doi: 10.1155/2015/156704
Keywords: biomarker, pediatric, congenital heart disease, IFABP, post-operative care, nutrition, NEC, cardiopulmonary bypass
Citation: Watson JD, Urban TT, Tong SS, Zenge J, Khailova L, Wischmeyer PE and Davidson JA (2020) Immediate Post-operative Enterocyte Injury, as Determined by Increased Circulating Intestinal Fatty Acid Binding Protein, Is Associated With Subsequent Development of Necrotizing Enterocolitis After Infant Cardiothoracic Surgery. Front. Pediatr. 8:267. doi: 10.3389/fped.2020.00267
Received: 25 November 2019; Accepted: 28 April 2020;
Published: 27 May 2020.
Edited by:
Giovanni Battista Luciani, University of Verona, ItalyReviewed by:
Massimo Caputo, University of Bristol, United KingdomCopyright © 2020 Watson, Urban, Tong, Zenge, Khailova, Wischmeyer and Davidson. This is an open-access article distributed under the terms of the Creative Commons Attribution License (CC BY). The use, distribution or reproduction in other forums is permitted, provided the original author(s) and the copyright owner(s) are credited and that the original publication in this journal is cited, in accordance with accepted academic practice. No use, distribution or reproduction is permitted which does not comply with these terms.
*Correspondence: John D. Watson, am9obi53YXRzb24yQGNoaWxkcmVuc2NvbG9yYWRvLm9yZw==
Disclaimer: All claims expressed in this article are solely those of the authors and do not necessarily represent those of their affiliated organizations, or those of the publisher, the editors and the reviewers. Any product that may be evaluated in this article or claim that may be made by its manufacturer is not guaranteed or endorsed by the publisher.
Research integrity at Frontiers
Learn more about the work of our research integrity team to safeguard the quality of each article we publish.