- 1Neonatal Research Group, Health Research Institute La Fe, Valencia, Spain
- 2Division of Neonatology, University and Polytechnic Hospital La Fe, Valencia, Spain
Fetal to neonatal transition entails cardiorespiratory, hemodynamic, and metabolic changes coinciding with the switch from placental to airborne respiration with partial pressures of oxygen of 4–5 kPa in utero raising to 8–9 kPa ex utero in few minutes. Preterm infants have immature lung and antioxidant defense system. Very preterm infants (<32 weeks' gestation) frequently require positive pressure ventilation and oxygen to establish lung aeration, a functional residual capacity, and overcome a tendency toward hypoxemia and bradycardia in the first minutes after birth. Recent studies have shown that prolonged bradycardia (heart rate <100 beats per minute) and/or hypoxemia (oxygen saturation <80%) are associated with increased mortality and/or intracranial hemorrhage. However, despite the accumulated evidence, the way in which oxygen should be supplemented in the first minutes after birth still has not yet been clearly established. The initial inspired fraction of oxygen and its adjustment within a safe arterial oxygen saturation range measured by pulse oximetry that avoids hyper-or-hypoxia is still a matter of debate. Herewith, we present a current summary aiming to assist the practical neonatologist who has to aerate the lung and establish an efficacious respiration in very preterm infants in the delivery room.
Introduction
Fetal life elapses in a lower oxygen environment as compared to extrauterine one. Oxygen is driven from the maternal side to the fetus across the placental intervillous spaces by a positive partial pressure delta gradient of oxygen between mother and fetus. The delta gradient evolves along gestation until the third trimester when it stabilizes around 4–5 kPa (30–40 mmHg). In parallel, the lung undergoes sequential structural changes from the canalicular stage at around 24–28 weeks of gestation to the saccular stage between 28 and 34 weeks, and finally reaches the alveolar stage in the last weeks of gestation. Simultaneously, the non-enzymatic and the enzymatic antioxidant defenses and the synthesis of surfactant only reach full functionality toward the end of gestation. The lung is then prepared for ex utero air respiration (1). Under these circumstances, premature infants have to aerate the lung and establish a functional residual capacity and an adequate pattern of respiration. Initial breathings have to achieve a negative pressure gradient between the atmospheric and the alveolar pressures that opens the lung and extrudes the fluid filling the lung into the alveolar interstitium. The muscular weakness, the thoracic cage compliance and the lack of surfactant present in preterm infants contribute to an uneven air distribution with overdistended areas coexisting with atelectatic ones. In addition, lack of surfactant causes alveolar collapse during expiration hindering the establishment of a functional residual capacity and subsequently causing respiratory distress and respiratory insufficiency (2).
To overcome this situation, the international guidelines recommend invasive or non-invasive positive pressure ventilation using a gas admixture containing oxygen to keep the lung open and favor gas exchange (3). Despite the existing body of experimental and clinical evidence, fundamental questions such as what initial inspired fraction of oxygen (FiO2) is optimal, what is the optimal SpO2 according to the timing from birth on, and how should FiO2 be titrated have not been conclusively answered. Moreover, there is an increasing conviction that oxygen supplementation should be individualized according to different parameters such as antenatal complications, gestational age, type of delivery or gender (1).
The present review article summarizes the available evidence regarding the use of supplemental oxygen in preterm infants during the golden first minutes after birth aiming to assist clinicians attending newborn infants in the delivery room to achieve optimal results.
Oxygen Monitoring and Reference Range For Oxygen Postnatal Stabilization
Oxygen Monitoring in the Clinical Setting
Oxygen diffuses from the alveoli to the capillary blood and is distributed by the arterial vascular network bound to hemoglobin (oxyhemoglobin) or physically dissolved in blood throughout the body (4). Oxygen monitoring devices inform about the concentration of oxygen in blood and detect the degree of hypoxia or hyperoxia thus allowing the clinicians to take therapeutic decisions. Oxygen content can be assessed as partial pressure of oxygen in arterial blood samples (paO2). PaO2, probably provides the most reliable information from a physiological and pathophysiological perspective because it precisely reflects the amount of oxygen that actually reaches the tissues (5). However, the need for an arterial access and frequent blood extractions limits its applicability to monitor oxygenation in newborn infants. Transcutaneous oxygen (tcPO2) is also a reliable method to measure arterial blood oxygen content but has also important constraints. The electrode needs to be heated causing skin irritation and occasionally serious burns especially in extremely preterm infants. Moreover, frequent and time-consuming electrode calibration is required and readings become less precise as newborn infants get older and the skin barrier becomes thicker (6, 7). Nowadays, the most employed method to non-invasively assess arterial blood oxygen saturation (SaO2) is pulse oximetry (SpO2). SpO2 measures the percentage of hemoglobin (Hb) that is bound to oxygen in arterial blood. Pulse oximeters measure changes in the light absorption emitted by a transductor with two different wavelengths (red and infrared) and captured by a photodetector coinciding with pulsatile dilatation and contraction of peripheral arteries using spectrophotometric principles. Using an ad hoc algorithm, changes in both light transduction wave lengths are converted into arterial oxygen saturation values (8). The interpretation of the values of oxygen saturation given by the pulse oximeter correlate with the affinity of Hb for oxygen and this is clearly influenced by the amount of fetal Hb (HbF) (9). The higher the amount of HbF the higher the SpO2 for a given PaO2. In a study performed by Castillo et al. (10), oxygen saturation values between 85 and 93% correlated with a mean PaO2 of 56 ± 14.8 mmHg and when SpO2 > 93% the mean PaO2 was 107.3 ± 59.3 mmHg. Hence, when establishing the upper limits of SpO2 the risk of hyperoxemia when HbF is predominant should be taken into consideration.
Postnatal changes in SpO2 have been widely explored in term and preterm infants, born by vaginal or C-section, or setting up the sensor pre-or-postductal (11). SpO2 rises rapidly in the first 5 min to values around 80–90% and thereafter reaches a plateau around 90–95% between 6 and 10 min after birth.
Dawson's Nomogram
Dawson et al. (12) merged three different databases with a total of 468 healthy newborn infants that were continuously monitored with preductal pulse oximetry during the first 10 min after birth. None of these babies required resuscitation or oxygen supplementation. SpO2 interquartile ranges at 3, 5, and 10 min were 81 (71–90%), 92 (83–96%), and 97% (94–98%), respectively, for term infants and 76 (67–83%), 86 (80–92%), and 94% (91–97%), respectively, for preterm infants. However, preterm infants included were mainly late preterm infants since only few babies were below 32 weeks gestational age. Thus, no reference range for “normal” postnatal oxygenation for very preterm infants is yet available. The most recent guidelines of the American Heart Association in 2015 have recommended SpO2 targets at 3, 5, and 10 min of 70–75, 80–85, and 85–95%, respectively (13). AHA recommendation was based in the achievement of a preductal oxygen saturation that approximates the inter-quartile range measured in healthy term infants after vaginal delivery at sea level (80–85%) based on the study by Mariani et al. (14). Almost simultaneously, the 2015 European Resuscitation Council guidelines (15) based their targeted saturation recommendations on the 25th centile of Dawson's nomogram which represents a SpO2 of 85% at 5 min (12), and the Spanish Neonatal Society recommended achieving a saturation value above the 15th centile of Dawson's nomogram (75%) at 5 min (16). Although based in different reference studies and centiles, it has been widely accepted that preterm infants should achieve a SpO2 of 75–85% at 5 min after birth. However, preterm infants using positive pressure ventilation and air achieved targeted SpO2 of 80–85% significantly earlier than those breathing spontaneously which should be taken into consideration for saturation targeting (17). Figure 1 depicts the ranges for arterial oxygen saturation and heart rate (HR) measured with pulse oximetry in the first minutes after birth according to the median and ICR described by Dawson et al. (12).
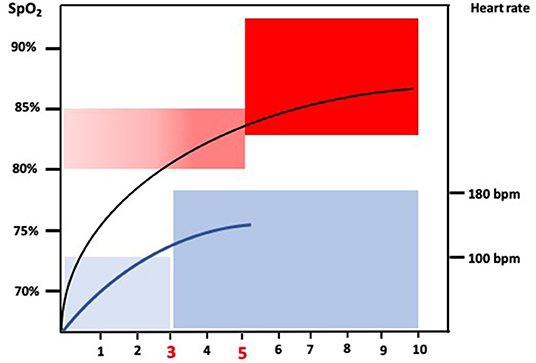
Figure 1. The figure depicts the recommended arterial oxygen saturation ranges based on preductal pulse oximetry measurements for newborn infants in the first 10 min after birth based on Dawson et al. (12). It also shows the recommended heart rate recommended ranges expressed in beats per minute based on Dawson et al. (18).
Initial Inspired Fraction of Oxygen in the Delivery Room: Contradictory Results
Studies Supporting the Use of Higher Initial Inspired Oxygen
In 2006, the Australian/New Zealand Neonatal Society changed the policy regarding the initial inspired fraction of oxygen (FiO2) in the delivery room from 1.0 to 0.21 (19). Dawson et al. (20) compared postnatal adaptation of a historical cohort of preterm infants vs. a new cohort. Of note, a significant number of babies stabilized with room air needed oxygen supplementation to overcome hypoxemia in the first minutes after birth while 80% of babies resuscitated with 100% oxygen had SpO2 ≥ 95%. No differences in HR in the first 10 min were observed between groups (20). The Canadian Neonatal Network did a similar study comparing infants ≤27 weeks' gestation before and after 2006 when initial FiO2 for preterm was changed from 1.0 to <1.0 oxygen (21). Adjusted OR (AOR) for the primary outcome of severe neurologic injury or death was higher in the lower oxygen group (AOR 1.36; 95% CI 1.11–1.66) and room air (AOR 1.33; 95% CI 1.04–1.69) groups as compared with the 100% oxygen group. Besides, the randomized controlled Torpido trial compared clinical outcomes of preterm infants resuscitated with room air vs. 100% oxygen. SpO2 targets were 65–85% in the 5th minute after birth and 85–95% upon admission in the NICU (22). In non-prespecified analyses, preterm <28 weeks' gestation resuscitated with air had a higher mortality (RR 3.9; 1.1–13.4; p = 0.01); however, the investigators admitted that the study was underpowered to address this post hoc hypothesis reliably (22). Follow up of the Torpido trial informed of the absence of differences in death or neurodevelopmental impairment at 2 years corrected age between the group resuscitated with an initial FiO2 of 0.21 and 1.0 (19). Of note, in post hoc exploratory analyses infants who did not achieve SpO2 >80% at 5 min after birth were more likely to die or have neurodevelopmental impairment (OR, 1.85; 1.07–3.2; P = 0.03) (23).
Studies Supporting the Use of Lower Initial Inspired Oxygen
Stola et al. (24) gradually reduced the initial FiO2 in the delivery room from 1.0 to 0.42, 0.28 and finally to 0.21 over a 12-month period. Compared with a historical cohort both the PaO2 at admission in the NICU and the median FiO2 employed during hospital stay were significantly lower in the study group (24). Escrig et al. (25) randomized preterm infants ≤28 weeks' gestation in the delivery room to an initial FiO2 of 0.3 or 0.9. Independently of the initial FiO2 both study groups achieved targeted saturations and HRs in the first 10 min after birth (25). However, in a subsequent study, preterm infants ≤ 28 weeks' gestation randomized to an initial FiO2 of 0.9 exhibited significantly higher levels of oxidative stress biomarkers and increased incidence of BPD than babies assigned to an initial FiO2 of 0.3 (26). Kapadia et al. (27) randomized preterm infants with gestational ages 24–34 weeks to a limited (initial FiO2 0.21) vs. a high oxygen (initial FiO2 1.0) strategy during DR stabilization. Both groups aimed to keep preductal SpO2 at 85–94%. The biochemical and clinical outcomes described by Vento et al. (26), were similar to those found in preterm infants in the high oxygen strategy group who exhibited significantly higher values for oxidative stress biomarkers and had a higher incidence of ventilator days and BPD (23). Kapadia et al. (28), recently published the follow up results of their study (27), and showed that the lower oxygen strategy was associated with improved respiratory morbidities and neurodevelopmental outcomes with no increase in mortality (28). Tataranno et al. (29) and Kato et al. (30) in blood and urine analysis, respectively, also found that using FiO2 of 1.0 significantly increased biomarkers of protein, lipids and DNA oxidation in preterm infants <32 weeks' gestation using high performance liquid chromatography coupled to mass spectrometry.
Two RCT studies blinded for oxygen by Aguar et al. (31) and Rook et al. (32) respectively, compared resuscitation of preterm infants with initial FiO2s of 0.30 vs. 0.60 and 0.30 vs. 0.65, respectively. No significant differences in achievement of targeted saturations, oxidative stress biomarkers or clinical outcomes (BPD, ROP, IVH, NEC) were reported. There was, however, an almost significant tendency toward decreased mortality in the lower oxygen group in both studies (31, 32). Follow up of these babies at 2 years of corrected age didn't show any differences in neurodevelopmental or sensory impairment among survivors (33). Oei et al. (34) published a meta-analysis comparing outcomes such as death during hospital stay or severe clinical conditions such as BPD, ROP >grade 2, IVH, ROP, PDA, and NEC of 504 preterm infants ≤28+6 weeks gestation who received higher (≥0.6) vs. lower (≤0.3) initial FiO2 in the delivery room. Mortality was lower in the masked studies of the low oxygen group (RR 0.46; 0.23–0.92; p=0.03) and higher in the low oxygen arms of unmasked studies (RR 1.94; 1.02–3.68; p = 0.04) (34). The authors concluded that there was no difference in the overall risk of death or severe morbidities depending on the initial FiO2 employed in extremely preterm infants. Differences in mortality between higher and lower oxygen groups were attributed to a type I error. Interestingly, babies in the low oxygen group needed more time to achieve targeted SpO2 (34).
Comprehensive Updated Reviews and Meta-Analyses
Recent systematic reviews have analyzed the influence of initial FiO2 in preterm stabilization in the DR on death and/or relevant preterm morbidities have been published by Lui et al. (35) and Welsford et al. (36), in 2018 and 2019, respectively. The primary objective of the study by Lui et al. (35) was to determine whether lower (<0.4) or higher (>0.4) initial oxygen concentrations, when titrated according to oxygen saturation targets during postnatal stabilization of preterm infants led to improved short-and-long-term mortality and morbidity. The study followed the Cochrane Systematic Review methodology for searching and analyzing data and included randomized studies comparing lower (<0.4) vs. higher (≥0.4) initial FiO2 titrated to target oxygen saturations during resuscitation of preterm infants (35). The search included 914 infants the majority born before 32 weeks' gestation in 10 RCTs published between 2008 and 2016. It is relevant to note that in each of these studies SpO2 and heart rate were monitored and FiO2 titrated according to targeted saturations. No differences in mortality between both groups could be assessed (RR 1.05, 95% CI 0.68–1.63). Besides, no significant differences to relevant preterm morbidities such as ROP, PVL, IVH, NEC, BPD, or PAD could be established (35). A sub-study including two trials showed no difference in neurodevelopmental disability at 24 months corrected age between groups (35). Given the low-grade quality of the studies due to risk of bias and imprecision there is still uncertainty as to whether the use of higher or lower initial FiO2 affects mortality, major morbidities or long-term neurodevelopmental disability in preterm infants (35). Welsford et al. performed a systematic review and meta-analysis gathering evidence comparing different levels of FiO2 employed to initiate resuscitation of preterm infants who require positive pressure ventilation in the DR (36). A total of 5697 preterm infants <35 weeks' gestation participating in 10 randomized/quasi randomized controlled and 4 retrospective observational cohort studies were analyzed. Higher initial FiO2 was defined as ≥0.5, and lower <0.5. Results concluded that starting with either initial FiO2 did not influence the primary outcome of short-term mortality [risk ratio (RR) 0.83, 95% confidence interval (CI) 0.50–1.37], long-term mortality, neurodevelopmental impairment, or other morbidities (36). It should be underscored that the meta-analysis included studies from 1980 to 2018 encompassing a wide range of different guidelines, study designs, devices, and gestational ages. Hence, the use of pulse oximetry and air/oxygen blender were not routinely started until late 90's. Welsford et al. recognized relevant limitations to the review, especially due to the risk of bias and imprecision and concluded that the ideal initial FiO2 for preterm infants is yet unknown and requires further investigation (36).
The Significant First 5 min After Birth
In the evaluation of postnatal adaptation of very preterm infants, HR and SpO2 have rendered especially valuable for predicting mortality and relevant clinical outcomes especially intraventricular hemorrhage. Virginia Apgar considered HR as the most important diagnostic and prognostic of the five signs in the Apgar score (37). Very preterm infants have physical characteristics that make clinical evaluation in the first minutes after birth difficult. Hence, although Apgar scores correlate with short-term and long-term outcomes in preterm infants there is a significant interobserver variability (38). Attempting to improve the predictability of the Apgar score gestational age and resuscitative interventions have been added to the conventional Apgar score to enhance the predictability of perinatal mortality and/or complications (39). Saugstad et al. (40) in 591 term infants resuscitated either with 21 or 100% oxygen analyzed the predictive value of different variables such as time to first breath, evolving HR, Apgar scores, SpO2 and base deficit. Of note, the highest odds ratio (OR) for death in the first week of life or for development of hypoxic-ischemic encephalopathy were 5 min HR ≤60 bpm (OR 16.5 for both death and HIE) and Apgar score <4 (OR 14 and 18.8) (40).
Seeking objectivity, the best means to assess HR rate from the first seconds after birth is the use of ECG. ECG is faster and more accurate that traditional stethoscope, palpation of the cord, peripheral pulses or even pulse oximeter as has been highlighted by recent systematic reviews (summarized in 40); however, existing evidence does not support substituting pulse oximetry with an ECG monitor (41). HR is the most accurate indicator of a positive response to stabilization maneuvers in the DR; hence, after a few puffs of effective ventilation and oxygenation HR rapidly increases to values above 100 bpm. Failure to do so, reveals either a profound and prolonged hypoxemia with extremely poor prognosis or an inadequate resuscitation technique or ventilatory/oxygenation parameters that need to be rapidly readjusted (42). Kapadia et al. (43) studied the association between bradycardia (HR <100 bpm) duration during preterm stabilization in the first 10 min of life with neonatal morbidity and mortality to determine if there was an interaction between prolonged bradycardia and low oxygen saturation. Results revealed that 38% of preterm infants <32 weeks' gestation experienced prolonged bradycardia and were more prone to die and/or develop IVH. Moreover, the risk of death was even higher if 5 min SpO2 was <80% (43). Independently of HR, the achievement of saturation targets in the first 5 min has also an important predictive value for death and/or intracranial hemorrhage. Oei et al. (44) established an association between SpO2 at 5 min and preterm outcomes. Individualized minute by minute data from 768 infants <32 weeks' gestation from 8 RCT of lower (<0.3) vs. higher (>0.6) initial FiO2 were analyzed. SpO2 <80% at 5 min was associated with higher risk of IVH (OR 2.04; CI 1.01–4.11; p < 0.05). Bradycardia at 5 min increased the risk of death (OR 4.57; CI 1.62–13.98; p < 0.05). It was concluded that not reaching SpO2 80% at 5 min is associated with an increase rate of death or IVH (44).
The first 5 min after birth are vital for the survival of preterm infants. During this extraordinary and brief period of time, cardiocirculatory and respiratory changes to adapt to the extrauterine life are sequentially accomplished in an orderly manner. Strictly monitoring both HR and SpO2 are reliable means to assess if fetal to neonatal transition is adequate and if resuscitation maneuvers when necessary are being efficient. SpO2 readings at 5 min after birth should be incorporated to the Apgar score as an additional predictive value, and HR in the Apgar score when possible should reflect values assessed with an ECG or pulse oximeter.
Future Studies
Delaying cord clamping (DCC) has been globally accepted as an advantageous intervention for stabilization of preterm infants in the delivery room (45). DCC increases the concentration of circulating HbF and enhances oxygen availability to tissue. Consequently, SpO2 ranges, targeted oxygen saturations, and oxygen titration procedure should be re-defined. Moreover, in experimental studies ventilation of the newborn while keeping the cord patent improves pulmonary circulation and oxygenation and reduces the post-asphyxia rebound hypertension which most likely protects the brain from cerebrovascular injury (46). Several clinical studies have shown the feasibility of this new approach in preterm infants; however, to date no improvement in clinical outcomes have obtained using the new modality of ventilation with intact cord when compared to the standard procedure (47–49).
The use of near infrared spectroscopy (NIRS) provides information of regional oxygen saturation and oxygen extraction of the brain almost immediately after birth and allows to better assess the impact of oxygen titration and ventilation upon cerebral oxygen metabolism. The combined use of pulse oximetry and NIRS has been successfully employed in clinical research (50, 51). Perhaps, in the future, simultaneous monitoring of brain regional oxygenation with NIRS and general oxygenation using pulse oximetry will become a standard in the delivery room.
Conclusions
Initial FiO2 should avoid hyper-or-hypoxemia and overcome bradycardia in the first minutes after birth. HR and SpO2 should be continuously monitored in very preterm infants and readings of the monitors incorporated to the resuscitation report. In addition, we would suggest to apply interventions to overcome both low HR and SpO2 within 5 min after fetal expulsion which are associated with adverse outcomes.
Recent comprehensive updated reviews and meta-analyses don't show long-or-short term improvements in the use of higher or lower initial FiO2. However, accumulated evidence supports starting resuscitation in very preterm infants (<32 weeks' gestation) with FiO2 of 0.21–0.3 depending on their clinical status. Moreover, using FiO2 0.3 in extremely preterm (<28 weeks' gestation) could contribute to avoid prolonged hypoxemia and bradycardia in the first five golden minutes.
Author Contributions
All authors have contributed to the design, reference search, drafting of the manuscript, and approval of its final form.
Funding
IL-C and AG-R acknowledge a predoctoral research grant from the Health Research Institute La Fe. AP-L acknowledges a Rio Hortega grant (CM18/00165) and MV acknowledges RETICS funded by the PN 2018-2021 (Spain), ISCIII- Sub-Directorate General for Research Assessment and Promotion and the European Regional Development Fund (FEDER), reference RD16/0022 from the Health Research Institute Carlos III (Ministry of Science, Universities and Innovation; Kingdom of Spain). IL-C acknowledges grant GV/2018/021.
Conflict of Interest
The authors declare that the research was conducted in the absence of any commercial or financial relationships that could be construed as a potential conflict of interest.
Acknowledgments
The authors would like to express their gratitude to Dr. Vishal Kapadia (UT Southwestern Medical Center at Dallas, Texas, USA) for reviewing the manuscript.
References
1. Vento M, Saugstad OD. Targeting oxygen in term and preterm infants starting at birth. Clin Perinatol. (2019) 46:459–73. doi: 10.1016/j.clp.2019.05.013
2. Vento M, Lista G. Managing preterm infants in the first minutes of life. Paediatr Respir Rev. (2015) 16:151–6. doi: 10.1016/j.prrv.2015.02.004
3. Sweet DG, Carnielli V, Greisen G, Hallman M, Ozek E, Te Pas A, et al. European consensus guidelines on the management of respiratory distress syndrome – 2019 update. Neonatology. (2019) 115:432–50. doi: 10.1159/000499361
4. Vali P, Lakshminrusimha S. The fetus can teach us: oxygen and the pulmonary vasculature. Children. (2017) 4:E67. doi: 10.3390/children4080067
5. Ortiz-Prado E, Dunn JF, Vasconez J, Castillo D, Viscor G. Partial pressure of oxygen in the human body: a general review. Am J Blood Res. (2019) 9:1–14.
6. Peabody JL, Willis MM, Gregory GA, Tooley WH, Lucey JF. Clinical limitations and advantages of transcutaneous oxygen electrodes. Acta Anaesthesiol Scand Suppl. (1978) 68:76–82. doi: 10.1111/j.1399-6576.1978.tb01397.x
7. Rome ES, Stork EK, Carlo WA, Martin RJ. Limitations of transcutaneous PO2 and PCO2 monitoring in infants with bronchopulmonary dysplasia. Pediatrics. (1984) 74:217–20.
8. Sola A, Chow L, Rogido M. Pulse oximetry in neonatal care in 2005. A comprehensive state of the art review. An Pediatr (Barc). (2005) 62:266–81. doi: 10.1157/13071843
9. Shiao SY, Ou CN, Pierantoni H. The measurement of accurate fetal hemoglobin and related oxygen saturation by the hemoximeter. Clin Chim Acta. (2006) 374:75–80. doi: 10.1016/j.cca.2006.05.042
10. Castillo A, Sola A, Baquero H, Neira F, Alvis R, Deulofeut R, et al. Pulse oxygen saturation levels and arterial oxygen tension values in newborns receiving oxygen therapy in the neonatal intensive care unit: is 85% to 93% an acceptable range? Pediatrics. (2008) 121:882–9. doi: 10.1542/peds.2007-0117
11. Finer N, Leone T. Oxygen saturation monitoring for the preterm infant: the evidence basis for current practice. Pediatr Res. (2009) 65:375–80. doi: 10.1203/PDR.0b013e318199386a
12. Dawson JA, Kamlin CO, Vento M, Wong C, Cole TJ, Donath SM, et al. Defining the reference range for oxygen saturation for infants after birth. Pediatrics. (2010) 125:e1340–7. doi: 10.1542/peds.2009-1510
13. Wyckoff MH, Aziz K, Escobedo MB, Kapadia VS, Kattwinkel J, Perlman JM, et al. Part 13: Neonatal Resuscitation: 2015 American Heart Association Guidelines update for cardiopulmonary resuscitation and emergency cardiovascular care. Circulation. (2015) 132:S543–60. doi: 10.1161/CIR.0000000000000267
14. Mariani G, Dik PB, Ezquer A, Aguirre A, Esteban ML, Perez C, et al. Pre-ductal and post-ductal O2 saturation in healthy term neonates after birth. J Pediatr. (2007) 150:418–21. doi: 10.1016/j.jpeds.2006.12.015
15. Wyllie J, Bruinenberg J, Roehr CC, Rüdiger M, Trevisanuto D, Urlesberger B. European resuscitation council guidelines for resuscitation 2015: section 7. Resuscitation and support of transition of babies at birth. Resuscitation. (2015) 95:249–63. doi: 10.1016/j.resuscitation.2015.07.029
16. Zeballos Sarrato G, Salguero García E, Aguayo Maldonado J, Gómez Robles C, Thió Lluch M, Iriondo Sanz M, et al. Changes in the international recommedations on neonatal stabilization and resuscitation (2015). An Pediatr (Barc). (2017) 86:51.e1-51.e9. doi: 10.1016/j.anpede.2016.08.004
17. Vento M, Cubells E, Escobar JJ, Escrig R, Aguar M, Brugada M, et al. Oxygen saturation after birth in preterm infants treated with continuous positive airway pressure and air: assessment of gender differences and comparison with a published nomogram. Arch Dis Child Fetal Neonatal Ed. (2013) 98:F228–32. doi: 10.1136/archdischild-2012-302369
18. Dawson JA, Kamlin CO, Wong C, te Pas AB, Vento M, Cole TJ, et al. Changes in heart rate in the first minutes after birth. Arch Dis Child Fetal Neonatal Ed. (2010) 95:F177–81. doi: 10.1136/adc.2009.169102
19. Australian Resuscitation Council. Guideline 13.1 Introduction to Resuscitation of the Newborn Infant. (2006). Available online at: http://www.resus.org.au/ (accessed November 30, 2019).
20. Dawson JA, Kamlin CO, Wong C, te Pas AB, O'Donnell CP, Donath SM, et al. Oxygen saturation and heart rate during delivery room resuscitation of infants <30 week's gestation with air or 100% oxygen. Arch Dis Child Fetal Neonatal Ed. (2009) 94:F87–91. doi: 10.1136/adc.2008.141341
21. Rabi Y, Lodha A, Soraisham A, Singhal N, Barrington K, Shah PS. Outcomes of preterm infants following the introduction of room air resuscitation. Resuscitation. (2015) 96:252–9. doi: 10.1016/j.resuscitation.2015.08.012
22. Oei JL, Saugstad OD, Lui K, Wright IM, Smyth JP, Craven P, et al. Targeted oxygen in the resuscitation of preterm infants, a randomized clinical trial. Pediatrics. (2017) 139:e20161452. doi: 10.1542/peds.2016-1452
23. Thamrin V, Saugstad OD, Tarnow-Mordi W, Wang YA, Lui K, Wright IM, et al. Preterm infant outcomes after randomization to initial resuscitation with FiO2 0.21 or 1.0. J Pediatr. (2018) 201:55–61. doi: 10.1016/j.jpeds.2018.05.053
24. Stola A, Schulman J, Perlman J. Initiating delivery room stabilization/resuscitation in very low birth weigth (VLBW) infants with an FiO2 less than 100% is feasible. J Perinatol. (2009) 29:548–52. doi: 10.1038/jp.2009.34
25. Escrig R, Arruza L, Izquierdo I, Villar G, Saénz P, Gimeno A, et al. Achievement of targeted saturation values in extremely low gestational age neonates resuscitated with low or high oxygen concentrations: a prospective, randomized trial. Pediatrics. (2008) 121:875–81. doi: 10.1542/peds.2007-1984
26. Vento M, Moro M, Escrig R, Arruza L, Villar G, Izquierdo I, et al. Preterm resuscitation with low oxygen causes less oxidative stress, inflammation, and chronic lung disease. Pediatrics. (2009) 124:e439–49. doi: 10.1542/peds.2009-0434
27. Kapadia VS, Chalak LF, Sparks JE, Allen JR, Savani RC, Wyckoff MH. Resuscitation of preterm neonates with limited versus high oxygen strategy. Pediatrics. (2013) 132:e1488–96. doi: 10.1542/peds.2013-0978
28. Kapadia VS, Lal CV, Kakkilaya V, Heune R, Savani RC, Wyckoff MH. Impact of the Neonatal Resuscitation Program-recommended low oxygen strategy on outcomes of infants born preterm. J Pediatr. (2017) 191:35–41. doi: 10.1016/j.jpeds.2017.08.074
29. Tataranno ML, Oei JL, Perrone S, Wright IM, Smyth JP, Lui K, et al. Resuscitating preterm infants with 100% oxygen is associated with higher oxidative stress than room air. Acta Paediatr. (2015) 104:759–65. doi: 10.1111/apa.13039
30. Kato E, Ibara S, Kumazawa K, Maruyama Y, Tokuhisa T, Matsui T, et al. Effects of supplemental oxygen on urinary 8-hydroxy-2'-deoxyguanosine levels in extremely low birth weight infants. Free Radic Res. (2014) 48:1285–90. doi: 10.3109/10715762.2014.951841
31. Aguar M, Cubells E, Escobar J, Kuligowski J, Iriondo M, Izquierdo M, et al. Preterm babies randomly assigned to be blindly resuscitated with higher (60%) vs. Lower (30%) initial FiO2: Effects on oxidative stress and mortality. EPAS. (2014) 3843:540.
32. Rook D, Schierbeek H, Vento M, Vlaardingerbroek H, van der Eijk AC, Longini M, et al. Resuscitation of preterm infants with different inspired oxygen fractions. J Pediatr. (2014) 164:1322–6.e3. doi: 10.1016/j.jpeds.2014.02.019
33. Boronat N, Aguar M, Rook D, Iriondo M, Brugada M, Cernada M, et al. Survival and neurodevelopmental outcomes of preterms resuscitated with different oxygen fractions. Pediatrics. (2016) 138:e20161405. doi: 10.1542/peds.2016-1405
34. Oei JL, Vento M, Rabi Y, Wright I, Finer N, Rich W, et al. higher or lower oxygen for delivery room resuscitation of preterm infants below 28 completed weeks gestation: a meta-analysis. Arch Dis Child Fetal Neonatal Ed. (2017) 102:F24–30. doi: 10.1136/archdischild-2016-310435
35. Lui K, Jones LJ, Foster JP, Davis PG, Ching SK, Oei JL, et al. Lower versus higher oxygen concentrations titrated to target oxygen saturations during resuscitation of preterm infants at birth. Cochrane Database Syst Rev. (2018) 5:CD010239. doi: 10.1002/14651858.CD010239.pub2
36. Welsford M, Nishiyama C, Shortt C, Weiner G, Roehr CC, Isayama T, et al. Initial oxygen use for preterm newborn resuscitation: a systematic review with meta-analysis. Pediatrics. (2019) 143:e20181828. doi: 10.1542/peds.2018-1828
37. Apgar VA. A proposal for a new method of evaluation of the newborn infant. Curr Res Anesth Analg. (1953) 32:260–7. doi: 10.1213/00000539-195301000-00041
38. O'Donnell CP, Kamlin CO, Davis PG, Carlin JB, Morley CJ. Interobserver variability of the 5-minute Apgar score. J Pediatr. (2006) 149:486–89. doi: 10.1016/j.jpeds.2006.05.040
39. Rüdiger M, Braun N, Aranda J, Aguar M, Bergert R, Bystricka A, et al. Neonatal assessment in the delivery room- Trial to evaluate a specified type of Apgar (TEST-Apgar). BMC Pediatr. (2015) 15:18. doi: 10.1186/s12887-015-0334-7
40. Saugstad OD, Ramji S, Rootwelt T, Vento M. Response to resuscitation of the newborn: early prognostic variables. Acta Paediatr. (2005) 94:890–5. doi: 10.1111/j.1651-2227.2005.tb02007.x
41. Manley BJ, Owen LS, Hooper SB, Jacobs SE, Cheong JLY, Doyle LW, et al. Towards evidence-based resuscitation of the newborn infant. Lancet. (2017) 389:1639–48. doi: 10.1016/S0140-6736(17)30547-0
42. Liley HG, Zestic J. The beating heart of newborn resuscitation. Resuscitation. (2019) 143:223–4. doi: 10.1016/j.resuscitation.2019.08.017
43. Kapadia VS, Oei JL, Saugstad OD, Rabi Y, Finer N, Wright I, et al. BradyPrem study: heart rate is most vital of vital signs during resuscitation of preterms. EPAS. (2018).
44. Oei JL, Finer NN, Saugstad OD, Wright IM, Rabi Y, Tarnow-Mordi W, et al. Outcomes of oxygen saturation targeting during delivery room stabilization of preterm infants. Arch Dis Child Fetal Neonatal Ed. (2018) 103:F446–54. doi: 10.1136/archdischild-2016-312366
45. Fogarty M, Osborn DA, Askie L, Seidler AL, Hunter K, Lui K, et al. Delayed vs. early umbilical cord clamping for preterm infants: a systematic review and meta-analysis. Am J Obstet Gynecol. (2018) 218:1–18. doi: 10.1016/j.ajog.2017.10.231
46. Polglase GR, Blank DA, Barton SK, Miller SL, Stojanowska V, Kluckow M, et al. Physiologically based cord clamping stabilizes cardiac output and reduces cerebrovascular injury in asphyxiated near-term lambs. Arch Dis Child Fetal Neonatal Ed. (2018) 103:F530–8. doi: 10.1136/archdischild-2017-313657
47. Katheria A, Poeltler D, Durham J, Steen J, Rich W, Arnell K, et al. Neonatal resuscitation with an intact cord: a randomized clinical trial. J Pediatr. (2016) 178:75–80. doi: 10.1016/j.jpeds.2016.07.053
48. Winter J, Kattwinkel J, Chisholm C, Blackman A, Wilson S, Fairchild K. Ventilation of preterm infants during delayed cord clamping (VentFirst): a pilot study of feasibility and safety. Am J Perinatol. (2017) 34:111–6. doi: 10.1055/s-0036-1584521
49. Brouwer E, Knol R, Vemooij ASN, vand den Akker T, Vlasman PE, Klumper FJCM, et al. Physiological-based cord clamping in preterm infants using a new purpose-built resuscitation table: a feasibility study. Arch Dis Child Fetal Neonatal Ed. (2019) 104:F396–402. doi: 10.1136/archdischild-2018-315483
50. Ziehenberger E, Urlesberger B, Binder-Heschl C, Schwaberger B, Baik-Schneditz N, Pichler G. Near-Infrated spectroscopy monitoring during immediate transition after birth: time to obtain cerebral tissue oxygenation. J Clin Monit Comput. (2018) 32:465–9. doi: 10.1007/s10877-017-0052-9
Keywords: preterm, fetal-to-neonatal transition, oxygen, pulse oximetry, oxidative stress
Citation: Lara-Cantón I, Solaz A, Parra-Llorca A, García-Robles A, Millán I, Torres-Cuevas I and Vento M (2020) Oxygen Supplementation During Preterm Stabilization and the Relevance of the First 5 min After Birth. Front. Pediatr. 8:12. doi: 10.3389/fped.2020.00012
Received: 27 August 2019; Accepted: 10 January 2020;
Published: 31 January 2020.
Edited by:
Graeme R. Polglase, Monash University, AustraliaReviewed by:
Jennifer A. Dawson, Royal Women's Hospital, AustraliaHelmut Dietmar Hummler, University of Ulm, Germany
Calum Roberts, Monash University, Australia
Copyright © 2020 Lara-Cantón, Solaz, Parra-Llorca, García-Robles, Millán, Torres-Cuevas and Vento. This is an open-access article distributed under the terms of the Creative Commons Attribution License (CC BY). The use, distribution or reproduction in other forums is permitted, provided the original author(s) and the copyright owner(s) are credited and that the original publication in this journal is cited, in accordance with accepted academic practice. No use, distribution or reproduction is permitted which does not comply with these terms.
*Correspondence: Maximo Vento, bWF4aW1vLnZlbnRvQHV2LmVz