- 1Department of Nephrology, Akron Children's Hospital and Cleveland Clinic Akron General, Akron, OH, United States
- 2Akron Nephrology Associates, Cleveland Clinic Akron General, Akron, OH, United States
- 3Department of Nephrology, Kidney & Urology Institute, Medanta, The Medicity, Gurgaon, India
- 4College of Medicine, Northeast Ohio Medical University, Rootstown, OH, United States
- 5College of Graduate Studies, Northeast Ohio Medical University, Rootstown, OH, United States
Background: A common practice in the management of critically ill patients is fluid resuscitation. An excessive administration of fluids can lead to an imbalance in fluid homeostasis and cause fluid overload (FO). In pediatric critical care patients, FO can lead to a multitude of adverse effects and increased risk of morbidity.
Objectives: To review the literature highlighting impact of FO on a multitude of outcomes in critically-ill children, causative vs. associative relationship of FO with critical illness and current pediatric fluid management guidelines.
Data Sources: A literature search was conducted using PubMed/Medline and Embase databases from the earliest available date until June 2017.
Data Extraction: Two authors independently reviewed the titles and abstracts of all articles which were assessed for inclusion. The manuscripts of studies deemed relevant to the objectives of this review were then retrieved and associated reference lists hand-searched.
Data Synthesis: Articles were segregated into various categories namely pathophysiology and sequelae of fluid overload, assessment techniques, epidemiology and fluid management. Each author reviewed the selected articles in categories assigned to them. All authors participated in the final review process.
Conclusions: Recent evidence has purported a relationship between mortality and FO, which can be validated by prospective RCTs (randomized controlled trials). The current literature demonstrates that “clinically significant” degree of FO could be below 10%. The lack of a standardized method to assess FB (fluid balance) and a universal definition of FO are issues that need to be addressed. To date, the impact of early goal directed therapy and utility of hemodynamic parameters in predicting fluid responsiveness remains underexplored in pediatric resuscitation.
Introduction
In a critically ill patient, fluid balance is imperative in management and maintaining homeostasis. More often than not, patients are resuscitated with fluids to maintain adequate intravascular volume. A common issue seen in pediatric intensive care units is fluid imbalances and hemodynamic instability. Aggressive fluid administration can lead to fluid overload (FO), a condition in which there is a positive fluid balance in the patient. This phenomenon has been associated with a multitude of unfavorable effects and can further complicate the patient's condition. While FO itself is no indicator of mortality, the adverse effects of FO on an already at risk population puts them at an increased risk of morbidity and mortality. Research on this commonly practiced standard of care has shown that inundation is not always the best method for hemodynamic optimization. Current literature shows that there is a lack of a standard definition of fluid overload and a standard guideline by which to direct fluid therapy. The purpose of this study was to review the literature highlighting the impact of FO on a multitude of outcomes in critically-ill children, analyzing the causative vs. associative relationship of FO with critical illness, and addressing the current pediatric fluid management guidelines.
Methods
The literature search was conducted using PubMed/Medline, and Embase databases for studies on fluid overload in pediatric critical care patients <18 years, from the earliest available date until June 2017. A total of 477 articles were obtained; after duplicate removal and review, 48 articles were selected for data extraction (Figure 1). The initial literature review consisted of two authors who independently reviewed the articles. The manuscripts of studies deemed relevant to the objectives of this review were then retrieved and associated reference lists hand-searched. Articles were categorized into FO associated with renal replacement therapy, extracorporeal membrane oxygenation, cardiac surgery, neonates, respiratory tract disease, and sepsis. All relevant publications were considered for data extraction. Tables were created summarizing the outcomes of the included studies.
Pathophysiology of Fluid Overload
Current understanding of FO is contingent upon the revised Starling law which ascribes an important role to EGL (endothelial glycocalyx) in maintaining an intact vascular biology. EGL, a web of membrane-bound glycoproteins and proteoglycans on the luminal surface of endothelium, binds to plasma proteins and effectively excludes proteins from the sub-endothelial cleft, thereby resulting in a local oncotic gradient between plasma and cleft which opposes trans-capillary efflux (1). The law states that a constant oncotic gradient present along capillaries opposes pressure-mediated fluid efflux without ever causing fluid reabsorption from interstitium (except for vascular beds in renal tubules and intestines), and almost all vascular refilling from interstitium occurs via lymphatics (2). EGL breakdown can be triggered by various processes and stresses such as sepsis, surgery, postischemic states, and hyperglycemia (2–7). Once the EGL is damaged, fluid efflux becomes primarily dependent on capillary hydrostatic pressure and the manipulation of plasma oncotic pressure with colloids does not encourage any vascular refilling. In addition to fluid and protein extravasation, EGL disruption potentiates ongoing inflammatory responses by exposing endothelial cell-adhesion molecules and may account for pathologies with interstitial edema such as acute lung injury (4). In experimental and perioperative settings, volume expansion has been shown to hasten glycocalyx disintegration and reduce lymphatic drainage through release of natriuretic peptides. In addition to cleaving membrane-bound proteoglycans and glycoproteins (most notably syndecan-1 and hyaluronic acid) off endothelial glycocalyx, they also reduce lymphatic drainage by inhibiting lymphatic propulsive motor activity (3, 4). This worsens FO because interstitial fluid is primarily channeled back into circulation via lymphatics.
Of note, some data regarding glycocalyx and natriuretic peptides is partly theoretical, and partly derived from adult studies and there is no pediatric-specific data in this context (8–10).
Quantifying Fluid Overload
Despite the burgeoning evidence regarding association between FO and adverse patient outcomes, there is no consensus on the optimal method for calculating FO. Lombel et al. highlighted that depending upon the definition used, there was a significant variability in the number of patients identified with >10% FO (11).
Most pediatric studies utilize the FB method introduced by Goldstein et al. (12). The formula is:
More practical WB(weight based) methods can be alternatively used to estimate degree of FO. The formulas are:
or
These are particularly useful in neonates because of uncertainties in estimating fluid input via breastmilk, fluid lost into diapers/bedsheets, and insensible losses apart from losses which can be markedly influenced by a number of factors (13). ICU admission weights when taken as baseline might under-estimate degree of FO in comparison to hospital admission weight. This discrepancy may also delay CRRT (continuous renal replacement therapy) initiation as most clinicians still consider 10% as the threshold to intervene (14). Multiple studies in both adult and pediatric literature have demonstrated poor agreement between changes in body weight and FO (Table 2). Nonetheless, reports have shown correlation between the two and a similar predictive potential of mortality (15). These inconsistent results warrant further research to recognize the most appropriate method of FO measurement.
Is Fluid Overload Ever Independent of AKI?
FO has detrimental effects on all organs, notably kidneys (2) (Figure 2). It is not just an epiphenomenon in AKI because FO per se renders an individual vulnerable to AKI and often predates it (23, 24). The development of abdominal hypertension and abdominal compartment syndrome impairs renal perfusion, and can culminate in a vicious cycle of AKI and FO (25). Deferral of AKI diagnosis correlates with the degree of fluid overload (Table 1). The risk of FO in this setting is that it can increase the volume of distribution of serum creatinine, a measure of AKI, leading to an overestimation of kidney function and a delayed diagnosis of AKI (26, 27). In scenarios where FO was not accounted for, patients with a decreasing trend in sCr (serum creatinine) had increased mortality than patients with high sCr and severe AKI (28).
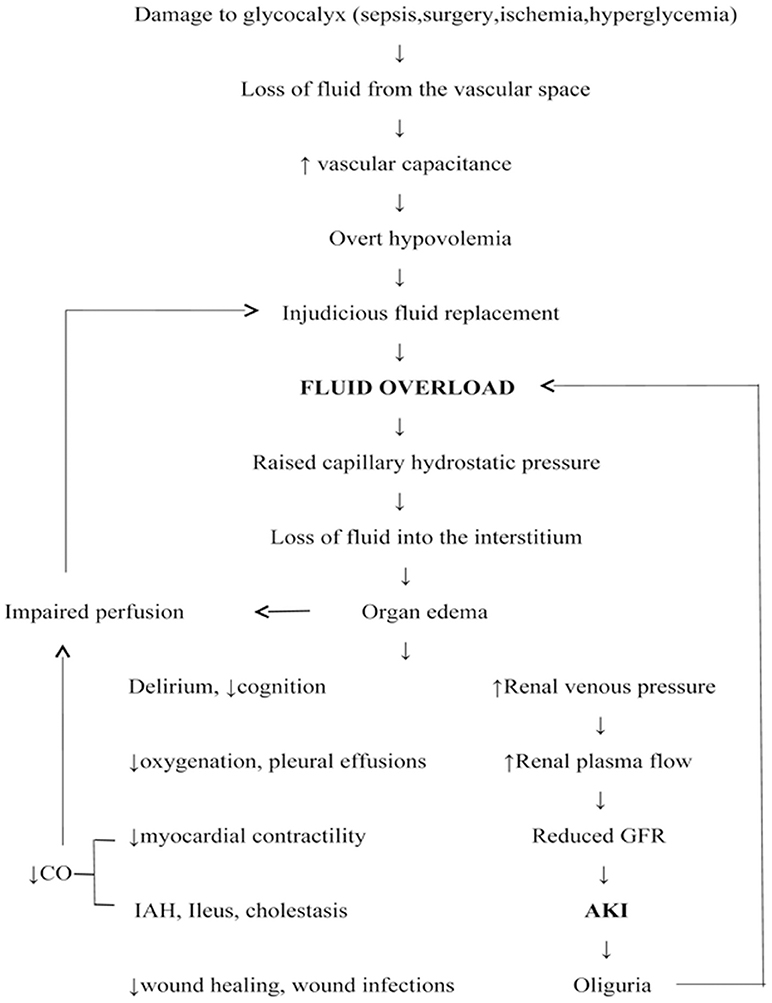
Figure 2. Fluid overload: pathogenesis and detrimental effects. CO, Cardiac output; AKI, acute kidney injury; IAH, Intra-abdominal hypertension; GFR, Glomerular filtration rate.
The following equation can be used to adjust sCr for fluid accumulation: adjusted Cr = sCr [1+ (Net fluid balance/Total body water)], where TBW = 0.6 × weight (kg) (29). However, use of the constant 0.6 in estimating TBW may not be accurate in all patients as it can be affected by age, sex, and various pathologic states (heart failure, cirrhosis, nephrosis).
Re-analysis of FACTT (Fluid and Catheter Treatment Trial) showed that presence of covert AKI was independently associated with a higher risk of death. Incidence of AKI was greater in the conservative arm before adjustment but in liberal arm post-adjustment (30). Secondary analysis of PICARD (Program to Improve Care in Renal Disease) cohort also revealed that correction for %FO uncovered missed diagnoses and reclassified AKI in some patients. Although no mortality differences were noted, the need for subsequent dialysis was higher in the misdiagnosed patients (27). Basu et al. reiterated these findings in 92 infants undergoing arterial switch operation wherein correction of sCr for FO not only increased AKI prevalence but also strengthened its association with postoperative morbidities (29).
Epidemiology and Outcomes
Fluid Overload and Adverse Outcomes: Does Current Evidence Endorse Causality?
With the exception of select adult trials and recent findings of Grams et al. (31–36), no pediatric study to date has demonstrated FO as a causal contributor to mortality rather than just a marker of disease severity. In their post-hoc analysis of FACTT, Grams et al. showed FO as a causal intermediate in the association between diuretic administration and decreased mortality in AKI patients, suggesting that the mortality benefit associated with furosemide was due to a decrease in FO (35). Few pediatric studies have in fact shown that FO is a significant co-morbidity that is highly associated with adverse outcomes only in patients with limited disease unlike patients with severe disease in whom the ultimate outcome is independent of %FO, suggesting that the disease severity itself is the primary determinant (37–39).
Renal Replacement Therapy
There are many indications for initiating renal replacement therapy (RRT) in a critically ill patient, FO being one of them. RRT provides control in fluid management and allows for the ability to achieve a negative fluid balance (40). Association between FO and mortality was first observed by pediatricians in critically ill children with AKI requiring RRT. Several pediatric studies have demonstrated an independent association between FO at the time of continuous renal replacement therapy (CRRT) initiation with mortality (41–45). A study by Goldstein looked at 116 pediatric intensive care unit patients with multiorgan dysfunction who received CRRT. The results showed a significant survival different in patients who were in a state of FO while on CRRT vs. those who are able to achieve their dry weight (36 vs. 76%) (43). The results from the ppCRRT Registry stratifying 297 patients into <10%, 10–20%, and >20% FO groups remain most comprehensive in this regard (41). Analysis revealed that presence of FO at CRRT initiation was associated with raised odds for mortality (aOR 1.03) after controlling for the severity of illness, with the maximum risk noted in the cohort with >20% FO(aOR 8.5,95% CI 2.8–25.7). The RENAL study (Randomized Evaluation of Normal vs. Augmented Levels of renal replacement therapy) showed that a negative mean daily FB was significantly associated with reduced mortality (p < 0.0001) and increased RRT-free days (p = 0.0017) (46). Non- surviving CRRT recipients (n = 610) in the PICARD study also had a significantly higher %FO at CRRT initiation (p = 0.01) and cessation (p = 0.004) (47). A subsequent re-analysis highlighted for the first time an increased risk of sepsis in patients after AKI. Authors speculated that FO compromises epithelial integrity by causing gut edema, thus favoring bacterial translocation (48).
Extracorporeal Membrane Oxygenation
Patients on ECMO are vulnerable to develop significant degree of FO due to the inflammatory milieu triggered by their critical illness, exposure to extracorporeal circuit and iatrogenic administration of fluids to minimize venous access insufficiency. The association between FO and mortality in children on ECMO has been reaffirmed in various pediatric and adult cohorts. Per literature, the need for initiating RRT arises in almost 50% ECMO patients (49, 50). Selewski et al. recently showed that median FO at CRRT initiation and discontinuation were significantly lower in ECMO survivors (49). FO reduction to <10% at CRRT conclusion was not associated with improved survival, emphasizing that early intervention is the key to enhanced survival. Although a growing body of evidence favors concomitant use of CRRT in ECMO patients, reports on this issue have been contentious (51, 52). In another study, Selewski showed that the degree of peak FO was associated with significant increases in duration of ECMO. Patients who died during hospitalization had higher FO at ECMO initiation, along with higher peak FO. Also noted was a graded increase in both ECMO and hospital mortality by 10% interval increases in FO (Table 4) (40).
Cardiac Surgery
In neonates with congenital heart disease undergoing cardiac surgery, fluid management is of particular importance. These patients are administered large amounts of fluid pre, intra, and post-operatively to prevent hypotension. The mainstay of management in these patients is diuretic therapy, with furosemide being the most widely administered drug (16). FO in these patients can also result from AKI (incidence = 30–50%) and systemic inflammation stemming from cardiopulmonary bypass (CPB) (53). FO in this cohort is significantly associated with increased in-hospital mortality (15, 54, 55); longer ICU and hospital stay, prolonged inotrope and ventilator support, and delayed sternal closure (56–59). Novel FO mitigating strategies, namely passive peritoneal drainage and modified ultrafiltration techniques are increasingly being employed. Given the high risk status of CHD patients, many are considered for preventative use of PD to reduce fluid accumulation. In some institutions the practice of inserting PD catheters intra-operatively is considered routine (16). Several studies have purported that prophylactic PD (peritoneal dialysis) achieves a net negative FB, ameliorates post-CPB inflammation via cytokine removal and reduces inotrope requirement (60–62). However, few single center studies do not reaffirm above findings (63). Most recent data on this population suggests that the potentially deleterious FO cut-off is likely <10% as studies have demonstrated equally poor outcomes with a FO level as low as 5% (54, 59).
Neonates
Association between FO and BPD (bronchopulmonary dysplasia) has been an active area of research. Studies document an association between FB and weight loss in the first few weeks of life and subsequent development of BPD in preterm neonates (64–66). In the neonatal period, a physiologic contraction of the extracellular fluid compartment leads to post-natal weight loss and a negative fluid and sodium balance. When this process is hindered by excess fluid intake, the retention of extracellular fluid can lead to fluid accumulation in pulmonary interstitial tissue. This puts the neonate at risk of barotrauma leading to BPD (64). Askenazi et al. reported that neonates with AKI had a higher maximum weight change in first 4 days of life (p = 0.05), higher risk of death and were more likely to require prolonged MV (p < 0.03) and oxygen support than neonates without AKI even post-adjustment (67). These studies suggest that ameliorating FO and AKI can reduce mortality and their sequelae (BPD and chronic kidney disease) which incur long term morbidity and excessive medical costs (Tables 3, 4). In the treatment of BPD, diuretics have been used; though studies have only shown short term effects without any long term benefits (80). Literature has suggested the possible benefit of practicing fluid restriction in preterm infants to prevent BPD. However, recent systematic review showed no evidence that supported fluid restriction in infants would prevent the subsequent development of BPD (81).
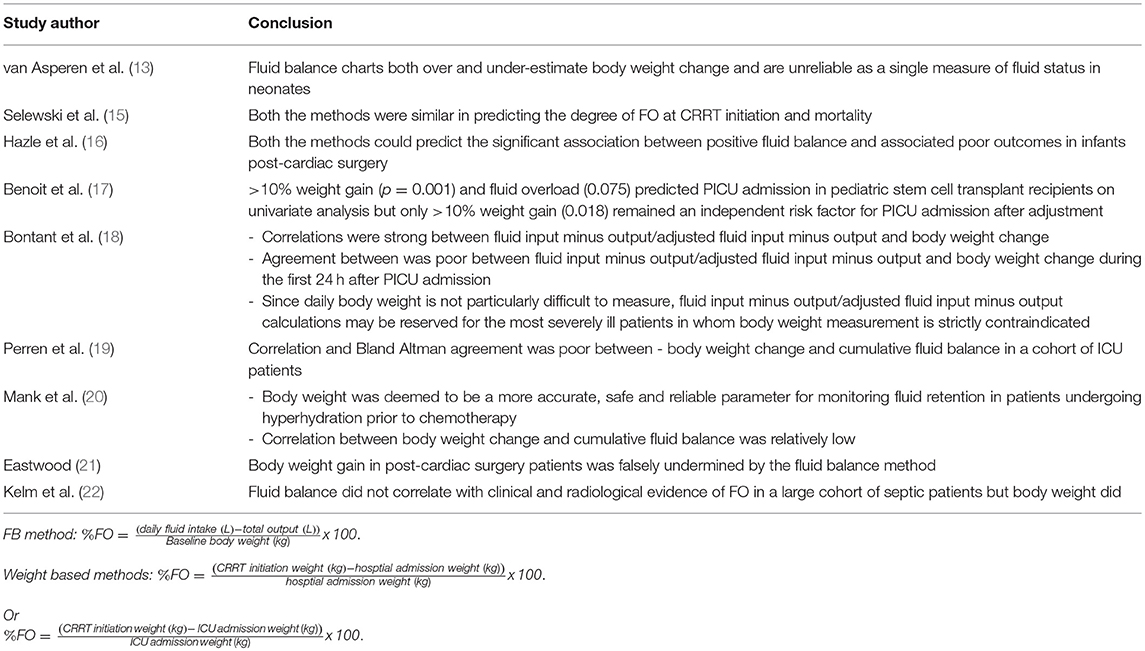
Table 2. Studies assessing the correlation and agreement between the fluid balance and weight based methods of fluid overload estimation.

Table 4. Summary of the studies evaluating outcomes associated with fluid overload in different pediatric populations.
Acute Respiratory Distress Syndrome
Several studies have demonstrated association between FO and worse respiratory outcomes (72–74). The adult FACTT trial showed that mean CFB (p < 0.001), VFDs (ventilator-free days) and ICU-free days were significantly lower in the conservative arm (31). Similarly, Valentine et al. noted that daily FO on D1-3 and CFB on D1-7 were higher in children with FO compared to adults in FACTT conservative arm (p < 0.001, each day) but similar to adults in the liberal arm, and promulgated the use of a Bayesian pediatric trial mirroring FACTT. No association with mortality was noted (73). A simplified fluid restrictive strategy employed in “FACTT lite” study also led to significantly lower FO than FACTT liberal protocol and no difference in VFDs compared to FACTT conservative protocol (82). Interestingly, mortality has been shown to correlate with increasing CFB (46), but not to a restrictive fluid management (31, 82).
Use of Early Goal Directed Resuscitation- Has the Impasse Ended?
The early goal directed therapy (EGDT) has been an area of intense research since its inception in the landmark Rivers' study wherein a mortality benefit was sought using protocolized (maintaining a CVP of 8–12 mmHg and SvO2 ≥70%) fluid resuscitation within first 6 h in the treatment of septic patients (83). An early up-front aggressive resuscitation was effective but late and excessive fluid administration was associated with worse survival at hospital discharge, 28 and 60 days. The critical difference emphasized by this protocol was the timing of the administration since the overall amount of fluid administered was equal in the two groups: a corollary to the conclusions drawn by Arikan et al., who recently evaluated outcomes in a controlled before and after study using a protocol driven resuscitation bundle in children with septic shock (84). Even though the total volume of fluid boluses did not differ between the two groups, thoughtful fluid administration earlier during the course of illness decreased mortality, AKI incidence, and need for RRT in comparison to historical controls. This time-sensitive nature of shock resuscitation has been stressed upon many times due to evidence of higher mortality in children with a delay in reversal of shock (85–88).
A number of studies, however, have questioned the efficacy of EGDT. Three multicenter RCTs- ProCESS, ProMISE and ARISE, did not show a statistically significant reduction in 90d mortality with EGDT but reported mortality was lower than the anticipated rate (89–91). Current surviving sepsis campaign guidelines (SSCG) do not endorse EGDT but still permit the use of previous targets as no harm was documented in these trials (92). Subsequent meta-analyses have yielded inconclusive results and equipoise still exists (93). Nevertheless, recruitment of less sick patients, treatment crossover due to non-blinding, and early institution of aggressive antibiotic therapy could haves biased the results of these negative trials (94).
Workman and colleagues recently showed that compliance with SSCG was not associated with better outcomes compared with usual therapy, which was administered more slowly in the emergency department (95). Since all children were treated rapidly, they reported low morbidity and mortality, and underscored the importance of rapid recognition and treatment of septic shock.
Fluids: Type in Critically Ill Children?
In children with diabetic ketoacidosis, NS (normal saline) is the preferred resuscitating fluid but development of significant hyperchloremic acidosis necessitates a switch to hypotonic fluids such as 1/2NS or more physiological choices with lower chloride but high sodium concentrations such as LR (lactated Ringer's), Hartmann's solution, or plasmalyte (96). In pediatric acute respiratory distress syndrome, the PALICC recommends monitoring and titrating fluid balance to maintain adequate intravascular volume, while aiming to prevent positive fluid balance (97). Mechanical ventilation in acute lung injury reduces the amount of maintenance fluids, so in these patients the preferred choice is a dextrose containing solution with NS (or one of the other more physiological solutions) at two-thirds of the maintenance rate that is used recommended for non-mechanically ventilated patients (96).
In cases of pediatric sepsis, different studies have shown varying and conflicting data on which fluids provide adequate resuscitation without increasing mortality. The SSCG recommend crystalloids as the initial resuscitation fluid of choice, with addition of albumin only if substantial amounts of crystalloids are required (98). However, UK investigators obtained outstanding results with use of albumin boluses in resuscitation of children with meningococcal septic shock (99). In an RCT, Maitland et al. demonstrated a mortality benefit in malarial septic shock with albumin when compared to crystalloids (100). Nevertheless, evidence strongly recommends against using hydroxyethyl starch in adults and children due to an acute increase in RRT and red cell transfusion requirement (92, 98).
In a recent large matched cohort study on pediatric sepsis, LR resuscitation was not associated with reduced mortality, AKI, or dialysis even when matched by fluid volume and proportionate LR utilization (101). Mortality increased with larger fluid volumes and decreased with a greater proportion of fluid given as LR, but there were no differences between LR and NS groups after matching within volume quartiles, by proportionate LR utilization, or in the separate matched analysis of LR-only patients. However, LR was preferentially used either as first-line fluid in patients with lower illness severity or as an adjunctive fluid in patients who received large amounts of fluid, and the matching algorithm was least effective in the most severely ill patients receiving the largest total fluid volumes. This could have masked a true benefit of LR. Further studies and well-designed RCTs are needed to clarify the optimal type and dose of resuscitation fluid.
Management of Fluid Overload
Early FO has garnered much attention as it seems to be a more decisive factor than late FO in predicting survival because the peak of illness is typically in first few days post-admission and the CFB curve generally flattens out after this period (58, 59, 71, 75, 76). Hence early fluid resuscitation is most likely beneficial, whereas early FO is not. Many studies advocate use of standard concentration drug infusion to prevent iatrogenic FO because many drugs are delivered as dilute solutions which significantly add to the daily fluid intake. This effect can be drastic in infants who are unnecessarily exposed to excess fluid through weight-based infusions (102). The concerns about perioperative hyponatremia in children with use of hypotonic maintenance fluids have been addressed lately via a switch to isotonic fluids (96, 103, 104).
The lack of a specific recommendation on optimal timing of RRT institution still plagues clinicians. CRRT remains the modality of choice in pediatric critical care. The American College of Critical Care Medicine recommends that a threshold of 10% should be construed as the time for intervention (14). An expert discussion of the pharmacologic management of FO in critically ill patients was recently published (105). Furosemide effectively achieves diuresis in critically ill children, but RCTs have failed to demonstrate any benefit of diuretics in preventing or treating AKI (106, 107). The furosemide stress test can be employed to assess the response to diuretics as resistance only delays RRT initiation (108). Aminophylline and fenoldopam have received medical attention owing to their potential in ameliorating AKI. The KDIGO guidelines recommend the use of a single dose of prophylactic theophylline in infants with severe perinatal asphyxia who are vulnerable to develop AKI (109). Use of these agents in post-cardiac surgery infants has also resulted in an improved excretory function and a reduction in urinary AKI biomarkers (110, 111).
One Size Does Not Fit All – Is It Worthwhile to Predict Fluid Responsiveness?
The prediction of FR (fluid responsiveness) in critically ill children remains largely unembraced to date despite its potential role in obviating FO as only 40–60 % of patients typically respond to fluids by increasing the cardiac output by more than 10–15% (112). The hemodynamic predictor variables have been classified as static and dynamic. Static variables are volumetric and include preload measures based on a single observation in time, namely maximal end diastolic volume, CVP or pulmonary artery occlusion pressure. Dynamic variables consider the cyclical changes in preload occurring during ventilation which are apparent as changes in stroke volume via the Frank-Starling law. These include systolic pressure variation (SPV), pulse pressure variation (PPV), and stroke volume variation (SVV). Respiratory variation in aortic blood flow peak velocity has been shown to be the only variable predictive of FR in children (112, 113). A recent systematic review demonstrated the limited predictive ability of static and dynamic variables in pediatric patients (112). Static variables did not predict FR in children, similar to the evidence in adults. However, dynamic variables based on arterial blood pressure also did not predict FR unlike adults. This probably could be due to age-related differences in vascular mechanical properties which affect the arterial waveform behavior. Evidence for dynamic variables based on plethysmography was deemed inconclusive. The insensitivity of these variables can be ascribed to numerous reasons, namely low tidal volume ventilation, spontaneous respirations, arrhythmias and valvular regurgitation. Inclusion of baseline contractility may improve the predictive potential of these variables. Standardized volume loading (e.g., 10 ml/kg) may not lead to consistent changes in ventricular EDV due to inter-individual variance (114). There is a need to clarify how differences in lung, vascular, and cardiac compliances affect predictive potential of dynamic variables in children as volume loading should be tailored for only those patients who are likely to increase stroke volume.
Limitations
Literature on fluid resuscitation in pediatric patients is vast but there is no general consensus on the type and dose of resuscitation fluid. Though there is an association between fluid overload and the risk of BPD in a neonate, there is no consensus or guideline on whether fluid restriction or the management of FO will reduce BPD incidence. This is just one example showing the current lack of knowledge on fluid management in children. Further studies and well-designed RCTs are necessary to provide physicians with optimal guidelines. The limited predictability of fluid responsiveness in children along with limited knowledge on the utility of early goal directed therapy further complicates the management of FO. There is a need for more studies to better clarify the effects of FO and create a standardized set of guidelines to assist physicians with management and decrease adverse outcomes.
Conclusions
Managing fluid overload in a critically ill pediatric patient is no simple task and poses a multitude of complications. To begin with, the lack of a proper consensus on a method for calculating FO due to the significant number of uncertainties in estimating intake and output makes management a challenge. While FO itself is not a direct marker of mortality, the adverse effects of FO lead to patients being vulnerable to an increased risk of morbidity and mortality. FO puts patients at a risk of being underdiagnosed with AKI and delays treatment, raises odds for mortality associated with CCRT complications, can lead to increased hospital and ICU stay, and prolonged ventilator support in the critically ill population. Given the current lack of knowledge on fluid management in children, further studies and RCTs are necessary to provide guidelines to physicians.
Author Contributions
All authors listed have made a substantial, direct and intellectual contribution to the work, and approved it for publication. RR, SKS, NW and SBB conceptualized the study. RR, SKS, NW and SBB wrote the review. MV and VK made significant contributions in writing, editing and revising the study.
Conflict of Interest Statement
The authors declare that the research was conducted in the absence of any commercial or financial relationships that could be construed as a potential conflict of interest.
References
1. Woodcock TE, Woodcock TM. Revised starling equation and the glycocalyx model of transvascular fluid exchange: an improved paradigm for prescribing intravenous fluid therapy. Br J Anaes. (2012) 108:384–94. doi: 10.1093/bja/aer515
2. O'Connor ME, Prowle JR. Fluid overload. Crit Care Clin. (2015) 31:803–21. doi: 10.1016/j.ccc.2015.06.013
3. Chelazzi C, Villa G, Mancinelli P, De Gaudio A, Adembri C. Glycocalyx and sepsis-induced alterations in vascular permeability. Crit Care (2015) 19:26. doi: 10.1186/s13054-015-0741-z
4. Schmidt EP, Yang Y, Janssen WJ, Gandjeva A, Perez MJ, Barthel L, et al. The pulmonary endothelial glycocalyx regulates neutrophil adhesion and lung injury during experimental sepsis. Nat Med. (2012) 18:1217–23. doi: 10.1038/nm.2843
5. Steppan J, Hofer S, Funke B, Brenner T, Henrich M, Martin E, et al. Sepsis and major abdominal surgery lead to flaking of the endothelial glycocalix. J Surg Res. (2011) 165:136–41. doi: 10.1016/j.jss.2009.04.034
6. Nieuwdorp M, van Haeften TW, Gouverneur LG, Mooij HL, van Lieshout HP, Levi M, et al. Loss of endothelial glycocalyx during acute hyperglycemia coincides with endothelial dysfunction and coagulation activation in vivo. Diabetes (2006) 55:480–6. doi: 10.2337/diabetes.55.02.06.db05-1103
7. Rehm M, Bruegger D, Christ F, Conzen P, Thiel M, Jacob M, et al. Shedding of the endothelial glycocalyx in patients undergoing major vascular surgery with global and regional ischemia. Circulation (2007) 116:1896–906. doi: 10.1161/CIRCULATIONAHA.106.684852
8. Bruegger D, Jacob M, Rehm M, Loetsch M, Welsch U, Conzen P, et al. Atrial natriuretic peptide induces shedding of endothelial glycocalyx in coronary vascular bed of guinea pig hearts. Am J Physiol Heart Circul Physiol. (2005) 289:H1993–9. doi: 10.1152/ajpheart.00218.2005
9. Chappell D, Bruegger D, Potzel J, Jacob M, Brettner F, Vogeser M, et al. Hypervolemia increases release of atrial natriuretic peptide and shedding of the endothelial glycocalyx. Crit Care (2014) 18:538. doi: 10.1186/s13054-014-0538-5
10. Bruegger D, Schwartz L, Chappell D, Jacob M, Rehm M, Vogeser M, et al. Release of atrial natriuretic peptide precedes shedding of the endothelial glycocalyx equally in patients undergoing on- and off-pump coronary artery bypass surgery. Basic Res Cardiol. (2011) 106:1111–21. doi: 10.1007/s00395-011-0203-y
11. Lombel RM, Kommareddi M, Mottes T, Selewski DT, Han YY, Gipson DS, et al. Implications of different fluid overload definitions in pediatric stem cell transplant patients requiring continuous renal replacement therapy. Intens Care Med. (2012) 38:663–69. doi: 10.1007/s00134-012-2503-6
12. Goldstein SL, Currier H, Graf JM, Cosio CC, Brewer ED, Sachdeva R. Outcome in children receiving continuous venovenous hemofiltration. Pediatrics (2001) 107:1309–12. doi: 10.1542/peds.107.6.1309
13. van Asperen Y, Brand LP, Bekhof J. Reliability of the fluid balance in neonates. Acta Paediatr. (2012) 101:479–83. doi: 10.1111/j.1651-2227.2012.02591.x
14. Davis AL, Carcillo JA, Aneja RK, Deymann AJ, Lin JC, Nguyen TC, et al. American College of critical care medicine clinical practice parameters for hemodynamic support of pediatric and neonatal septic shock. Crit Care Med. (2017) 45:1061–93. doi: 10.1097/CCM.0000000000002425
15. Selewski DT, Cornell TT, Lombel RM, Blatt NB, Han YY, Mottes T, et al. Weight-based determination of fluid overload status and mortality in pediatric intensive care unit patients requiring continuous renal replacement therapy. Intens Care Med. (2011) 37:1166–73. doi: 10.1007/s00134-011-2231-3
16. Hazle MA, Gajarski RJ, Yu S, Donohue J, Blatt NB. Fluid overload in infants following congenital heart surgery. Pediatr Crit Care Med. (2013) 14:44–9. doi: 10.1097/PCC.0b013e3182712799
17. Benoit G, Phan V, Duval M, Champagne M, Litalien C, Merouani A. Fluid balance of pediatric hematopoietic stem cell transplant recipients and intensive care unit admission. Pediatr Nephrol. (2006) 22:441–7. doi: 10.1007/s00467-006-0331-z
18. Bontant T, Matrot B, Abdoul H, Aizenfisz S, Naudin J, Jones P, et al. Assessing fluid balance in critically ill pediatric patients. Eur J Pediatr. (2014) 174:133–7. doi: 10.1007/s00431-014-2372-9
19. Perren A, Markmann M, Merlani G, Marone C, Merlani P. Fluid balance in critically ill patients. Should we really rely on it? Minerva Anestesiol. (2011) 77:802–11.
20. Mank A, Semin-Goossens A, Lelie JV, Bakker P, Vos R. Monitoring hyperhydration during high-dose chemotherapy: body weight or fluid balance? Acta Haematol. (2003) 109:163–8. doi: 10.1159/000070964
21. Eastwood GM. Evaluating the reliability of recorded fluid balance to approximate body weight change in patients undergoing cardiac surgery. Heart Lung J Acute Crit Care (2006) 35:27–33. doi: 10.1016/j.hrtlng.2005.06.001
22. Kelm DJ, Perrin JT, Cartin-Ceba R, Gajic O, Schenck L, Kennedy CC. Fluid overload in patients with severe sepsis and septic shock treated with early goal-directed therapy is associated with increased acute need for fluid-related medical interventions and hospital death. Shock (2015) 43:68–73. doi: 10.1097/SHK.0000000000000268
23. Prowle JR, Echeverri JE, Ligabo EV, Ronco C, Bellomo R. Fluid balance and acute kidney injury. Nat Rev Nephrol. (2009) 6:107–15. doi: 10.1038/nrneph.2009.213
24. Rajendram R, Prowle JR. Venous congestion: are we adding insult to kidney injury in sepsis? Crit Care (2014) 18:104. doi: 10.1186/cc13709
25. Carlotti APCP, Carvalho WB. Abdominal compartment syndrome: a review. Pediatr Crit Care Med. (2009) 10:115–20. doi: 10.1097/PCC.0b013e31819371b2
26. Basu RK, Chawla LS, Wheeler DS, Goldstein SL. Renal angina: an emerging paradigm to identify children at risk for acute kidney injury. Pediatr Nephrol. (2011) 27:1067–78. doi: 10.1007/s00467-011-2024-5
27. Macedo E, Bouchard J, Soroko SH, Chertow GM, Himmelfarb J, Ikizker TA, et al. Fluid accumulation, recognition and staging of acute kidney injury in critically-ill patients. Crit Care (2010) 14:R82. doi: 10.1186/cc9004
28. Cerda J, Cerda M, Kilcullen P, Prendergast J. In severe acute kidney injury, a higher serum creatinine is paradoxically associated with better patient survival. Nephrol Dialys Transplant. (2007) 22:2781–4. doi: 10.1093/ndt/gfm395
29. Basu RK, Andrews A, Krawczeski C, Manning P, Wheeler DS, Goldstein SL. Acute kidney injury based on corrected serum creatinine is associated with increased morbidity in children following the arterial switch operation. Pediatr Crit Care Med. (2013) 14:e218–24. doi: 10.1097/PCC.0b013e3182772f61
30. Liu KD, Thompson BT, Ancukiewicz M, Steingrub JS, Douglas IS, Matthay MA, et al. Acute kidney injury in patients with acute lung injury: Impact of fluid accumulation on classification of acute kidney injury and associated outcomes*. Crit Care Med. (2011) 39:2665–71. doi: 10.1097/CCM.0b013e318228234b
31. Wiedemann HP, Wheeler AP, Bernard GR. Comparison of two fluid-management strategies in acute lung injury. J Vasc Surg. (2006) 44:909. doi: 10.1016/j.jvs.2006.08.053
32. Willson DF, Thomas NJ, Tamburro R, Truemper E, Truwit J, Conaway M, et al. The relationship of fluid administration to outcome in the pediatric calfactant in acute respiratory distress syndrome trial*. Pediatr Crit Care Med. (2013) 14:666–72. doi: 10.1097/PCC.0b013e3182917cb5
33. Arikan AA, Zappitelli M, Goldstein SL, Naipaul A, Jefferson LS, Loftis LL. Fluid overload is associated with impaired oxygenation and morbidity in critically ill children*. Pediatr Crit Care Med. (2012) 13:253–8. doi: 10.1097/PCC.0b013e31822882a3
34. Li Y, Wang J, Bai Z, Chen J, Wang X, Pan J, et al. Early fluid overload is associated with acute kidney injury and PICU mortality in critically ill children. Eur J Pediatr. (2015) 175:39–48. doi: 10.1007/s00431-015-2592-7
35. Brandstrup B, Tønnesen H, Beier-Holgersen R, Hjortsø E, Ørding H, Lindorff-Larsen K, et al. Effects of intravenous fluid restriction on postoperative complications: comparison of two perioperative fluid regimens. Ann Surg. (2003) 238:641–8. doi: 10.1097/01.sla.0000094387.50865.23
36. Grams ME, Estrella MM, Coresh J, Brower RG, Liu KD. Fluid balance, diuretic use, and mortality in acute kidney injury. Clin J Am Soc Nephrol. (2011) 6:966–73. doi: 10.2215/CJN.08781010
37. Abulebda K, Cvijanovich NZ, Thomas NJ, Allen GL, Anas N, Bigham MT, et al. Post-ICU admission fluid balance and pediatric septic shock outcomes. Crit Care Med. (2014) 42:397–403. doi: 10.1097/CCM.0b013e3182a64607
38. de Galasso L, Emma F, Picca S, Di Nardo M, Rossetti E, Guzzo I. Continuous renal replacement therapy in children: fluid overload does not always predict mortality. Pediatr Nephrol. (2016) 31:651–9. doi: 10.1007/s00467-015-3248-6
39. Flores FX, Brophy D, Symons JM, Fortenberry JD, Chua AN, Alexander SR, et al. Continuous renal replacement therapy (CRRT) after stem cell transplantation. A report from the prospective pediatric CRRT Registry Pediatric Nephrol. (2008) 23:625–30. doi: 10.1007/s00467-007-0672-2
40. Selewski DT, Askenazi DJ, Bridges BC, Cooper DS, Fleming GM, Paden ML, et al. The impact of fluid overload on outcomes in children treated with extracorporeal membrane oxygenation: a multicenter retrospective cohort study. Pediatr Crit Care Med. (2017) 18:1126–35. doi: 10.1097/PCC.0000000000001349
41. Sutherland SM, Zappitelli M, Alexander SR, Chua AN, Brophy D, Bunchman TE, et al. Fluid overload and mortality in children receiving continuous renal replacement therapy: the prospective pediatric continuous renal replacement therapy registry. Am J Kidney Dis. (2010) 55:316–25. doi: 10.1053/j.ajkd.2009.10.048
42. Hayes LW, Oster RA, Tofil NM, Tolwani AJ. Outcomes of critically ill children requiring continuous renal replacement therapy. J Crit Care (2009) 24:394–400. doi: 10.1016/j.jcrc.2008.12.017
43. Goldstein SL, Somers JG, Baum MA, Symons JM, Brophy D, Blowey D, et al. Pediatric patients with multi-organ dysfunction syndrome receiving continuous renal replacement therapy. Kidney Int. (2005) 67:653–8. doi: 10.1111/j.1523-1755.2005.67121.x
44. Gillespie RS, Seidel K, Symons JM. Effect of fluid overload and dose of replacement fluid on survival in hemofiltration. Pediatr Nephrol. (2004) 19:1394–9. doi: 10.1007/s00467-004-1655-1
45. Foland JA, Fortenberry JD, Warshaw BL, Pettignano R, Merritt RK, Heard ML, et al. Fluid overload before continuous hemofiltration and survival in critically ill children: A retrospective analysis*. Crit Care Med. (2004) 32:1771–76. doi: 10.1097/01.CCM.0000132897.52737.49
46. Bellomo R, Cass A, Cole L, Finfer S, Gallagher M, Lee J, et al. An observational study fluid balance and patient outcomes in the randomized evaluation of normal vs. augmented level of replacement therapy trial. Crit Care Med. (2012) 40:1753–60. doi: 10.1097/CCM.0b013e318246b9c6
47. Bouchard J, Soroko SB, Chertow GM, Himmelfarb J, Ikizler TA, Paganini EP, et al. Fluid accumulation, survival and recovery of kidney function in critically ill patients with acute kidney injury. Kidney Int. (2009) 76:422–7. doi: 10.1038/ki.2009.159
48. Mehta RL, Bouchard J, Soroko SB, Ikizler TA, Paganini EP, Chertow GM, et al. Sepsis as a cause and consequence of acute kidney injury: Program to improve care in acute renal disease. Intens Care Med. (2010) 37:241–8. doi: 10.1007/s00134-010-2089-9
49. Selewski DT, Cornell TT, Blatt NB, Han YY, Mottes T, Kommareddi M, et al. Fluid overload and fluid removal in pediatric patients on extracorporeal membrane oxygenation requiring continuous renal replacement therapy*. Crit Care Med. (2012) 40:2694–9. doi: 10.1097/CCM.0b013e318258ff01
50. Zangrillo A, Landoni G, Biondi-Zoccai G, Greco M, Greco T, Frati G, et al. A meta-analysis of complications and mortality of extracorporeal membrane oxygenation. Crit Care Resusc. (2013) 15:172–8.
51. Wolf MJ, Chanani NK, Heard ML, Kanter KR, Mahle WT. Early renal replacement therapy during pediatric cardiac extracorporeal support increases mortality. Ann Thorac Surg. (2013) 96:917–22. doi: 10.1016/j.athoracsur.2013.05.056
52. Paden ML, Warshaw BL, Heard ML, Fortenberry JD. Recovery of renal function and survival after continuous renal replacement therapy during extracorporeal membrane oxygenation*. Pediatr Crit Care Med. (2011) 12:153–8. doi: 10.1097/PCC.0b013e3181e2a596
53. Blinder JJ, Goldstein SL, Lee V, Baycroft A, Fraser CD, Nelson D, et al. Congenital heart surgery in infants: effects of acute kidney injury on outcomes. J Thorac Cardiovasc Surg. (2012) 143:368–74. doi: 10.1016/j.jtcvs.2011.06.021
54. Lex DJ, Tóth R, Czobor NR, Alexander SI, Breuer T, Sápi E, et al. Fluid overload is associated with higher mortality and morbidity in pediatric patients undergoing cardiac surgery*. Pediatr Crit Care Med. (2016) 17:307–14. doi: 10.1097/PCC.0000000000000659
55. Grist G, Whittaker C, Merrigan K, Fenton J, Worrall E, O'Brien J, et al. The correlation of fluid balance changes during cardiopulmonary bypass to mortality in pediatric and congenital heart surgery patients. J Extra Corpor Technol. (2011) 43:215–26.
56. Sampaio TZAL, O'Hearn K, Reddy D, Menon K. The influence of fluid overload on the length of mechanical ventilation in pediatric congenital heart surgery. Pediatr Cardiol. (2015) 36:1692–9. doi: 10.1007/s00246-015-1219-0
57. Piggott KD, Soni M, Decampli WM, Ramirez JA, Holbein D, Fakioglu H, et al. Acute kidney injury and fluid overload in neonates following surgery for congenital heart disease. World J Pediatr Cong Heart Surg. (2015) 6:401–6. doi: 10.1177/2150135115586814
58. Seguin J, Albright B, Vertullo L, Lai P, Dancea A, Bernier L, et al. Extent, risk factors, and outcome of fluid overload after pediatric heart surgery*. Crit Care Med. (2014) 42:2591–9. doi: 10.1097/CCM.0000000000000517
59. Hassinger AB, Wald EL, Goodman DM. Early postoperative fluid overload precedes acute kidney injury and is associated with higher morbidity in pediatric cardiac surgery patients. Pediatr Crit Care Med. (2014) 15:131–8. doi: 10.1097/PCC.0000000000000043
60. Saini A, Delius RE, Seshadri S, Walters H, Mastropietro CW. Passive peritoneal drainage improves fluid balance after surgery for congenital heart disease. Eur J Cardio Thorac Surg. (2011) 41:256–60. doi: 10.1016/j.ejcts.2011.05.037
61. Sasser WC, Dabal RJ, Askenazi DJ, Borasino S, Moellinger AB, Kirklin JK, et al. Prophylactic peritoneal dialysis following cardiopulmonary bypass in children is associated with decreased inflammation and improved clinical outcomes. Cong Heart Dis. (2013) 9:106–15. doi: 10.1111/chd.12072
62. Kwiatkowski DM, Menon S, Krawczeski CD, Goldstein SLD, Morales LS, Phillips A, et al. Improved outcomes with peritoneal dialysis catheter placement after cardiopulmonary bypass in infants. J Thorac Cardiovasc Surg. (2015) 149:230–6. doi: 10.1016/j.jtcvs.2013.11.040
63. Ryerson LM, Mackie AS, Atallah J, Joffe AR, Rebeyka IM, Ross DB, et al. Prophylactic peritoneal dialysis catheter does not decrease time to achieve a negative fluid balance after the Norwood procedure: a randomized controlled trial. J Thorac Cardiovasc Surg. (2015) 149:222–8. doi: 10.1016/j.jtcvs.2014.08.011
64. Oh W, Poindexter BB, Perritt R, Lemons JA, Bauer CR, Ehrenkranz RA, et al. Association between fluid intake and weight loss during the first ten days of life and risk of bronchopulmonary dysplasia in extremely low birth weight infants. J Pediatr. (2005) 147:786–90. doi: 10.1016/j.jpeds.2005.06.039
65. Schmidt B, Roberts RS, Fanaroff A, Davis P, Kirpalani HM, Nwaesei C, et al. Indomethacin prophylaxis, patent ductus arteriosus, and the risk of bronchopulmonary dysplasia: further analyses from the Trial of Indomethacin Prophylaxis in Preterms (TIPP). J Pediatr. (2006) 148:730–4.e1. doi: 10.1016/j.jpeds.2006.01.047
66. Marshall DD, Kotelchuck M, Young TE, Bose CL, Kruyer L, O'Shea TM. Risk factors for chronic lung disease in the surfactant era: a North Carolina population-based study of very low birth weight infants. Pediatrics (1999) 104:1345–50. doi: 10.1542/peds.104.6.1345
67. Askenazi D, Patil NR, Ambalavanan N, Balena-Borneman J, Lozano DJ, Ramani M, et al. Acute kidney injury is associated with bronchopulmonary dysplasia/mortality in premature infants. Pediatr Nephrol. (2015) 30:1511–8. doi: 10.1007/s00467-015-3087-5
68. Swaniker F, Kolla S, Moler F, Custer J, Grams R, Bartlett R, et al. Extracorporeal life support outcome for 128 pediatric patients with respiratory failure. J Pediatric Surg. (2000) 35:197–202. doi: 10.1016/S0022-3468(00)90009-5
69. Lee ST, Cho H. Fluid overload and outcomes in neonates receiving continuous renal replacement therapy. Pediatric Nephrol. (2016) 31:2145–52. doi: 10.1007/s00467-016-3363-z
70. Askenazi DJ, Koralkar R, Hundley HE, Montesanti A, Patil N, Ambalavanan N. Fluid overload and mortality are associated with acute kidney injury in sick near-term/term neonate. Pediatr Nephrol. (2012) 28:661–6. doi: 10.1007/s00467-012-2369-4
71. Ingelse SA, Wiegers MG, Calis JC, van Woensel JB, Bem RA. Early fluid overload prolongs mechanical ventilation in children with viral-lower respiratory tract disease*. Pediatr Crit Care Med. (2017) 18:e106–11. doi: 10.1097/PCC.0000000000001060
72. Sinitsky L, Walls D, Nadel S, Inwald DP. Fluid overload at 48 hours is associated with respiratory morbidity but not mortality in a general PICU. Pediatr Crit Care Med. (2015) 16:205–9. doi: 10.1097/PCC.0000000000000318
73. Valentine SL, Sapru A, Higgerson RA, Spinella C, Flori HR, Graham DA, et al. Fluid balance in critically ill children with acute lung injury*. Crit Care Med. (2012) 40:2883–9. doi: 10.1097/CCM.0b013e31825bc54d
74. Flori HR, Church G, Liu KD, Gildengorin G, Matthay MA. Positive fluid balance is associated with higher mortality and prolonged mechanical ventilation in pediatric patients with acute lung injury. Crit Care Res Pract. (2011) 2011:1–5. doi: 10.1155/2011/854142
75. Chen J, Li X, Bai Z, Fang F, Hua J, Li Y, et al. Association of fluid accumulation with clinical outcomes in critically ill children with severe sepsis. PLOS ONE (2016) 11:e0160093. doi: 10.1371/journal.pone.0160093
76. Bhaskar P, Dhar AV, Thompson M, Quigley R, Modem V. Early fluid accumulation in children with shock and ICU mortality: a matched case–control study. Intens Care Med. (2015) 41:1445–53. doi: 10.1007/s00134-015-3851-9
77. Hoover NG, Heard M, Reid C, Wagoner S, Rogers K, Foland J, et al. Enhanced fluid management with continuous venovenous hemofiltration in pediatric respiratory failure patients receiving extracorporeal membrane oxygenation support. Intens Care Med. (2008) 34:2241–7. doi: 10.1007/s00134-008-1200-y
78. Blijdorp K, Cransberg K, Wildschut ED, Gischler SJ, Jan Houmes R, Wolff ED, et al. Haemofiltration in newborns treated with extracorporeal membrane oxygenation: a case-comparison study. Crit Care (2009) 13:R48. doi: 10.1186/cc7771
79. Maitland K, Kiguli S, Opoka RO, Engoru C, Olupot-Olupot P, Akech SO, et al. Mortality after Fluid Bolus in African Children with Severe Infection. N Engl J Med. (2011) 364:2483–95. doi: 10.1056/NEJMoa1101549
80. McCann EM, Lewis K, Deming DD, Donovan MJ, Brady JP. Controlled trial of furosemide therapy in infants with chronic lung disease. J Pediatr. (1985) 106:957–62. doi: 10.1016/S0022-3476(85)80252-3
81. Barrington KJ, Fortin-Pellerin E, Pennaforte T. Fluid restriction for treatment of preterm infants with chronic lung disease. Cochr Database Syst Rev. (2017) 2:Cd005389. doi: 10.1002/14651858.CD005389.pub2
82. Grissom CK, Hirshberg EL, Dickerson JB, Brown SM, Lanspa MJ, Liu KD, et al. Fluid management with a simplified conservative protocol for the acute respiratory distress syndrome*. Crit Care Med. (2015) 43:288–95. doi: 10.1097/CCM.0000000000000715
83. Rivers E, Nguyen B, Havstad S, Ressler J, Muzzin A, Knoblich B, et al. Early goal-directed therapy in the treatment of severe sepsis and septic shock. N Engl J Med. (2001) 345:1368–77. doi: 10.1056/NEJMoa010307
84. Akcan Arikan A, Williams EA, Graf JM, Kennedy CE, Patel B, Cruz AT. Resuscitation bundle in pediatric shock decreases acute kidney injury and improves outcomes. J Pediatr. (2015) 167:1301–5 e1. doi: 10.1016/j.jpeds.2015.08.044
85. Han YY, Carcillo JA, Dragotta MA, Bills DM, Watson RS, Westerman ME, et al. Early reversal of pediatric-neonatal septic shock by community physicians is associated with improved outcome. Pediatrics (2003) 112:793–9. doi: 10.1542/peds.112.4.793
86. Kissoon N, Orr RA, Carcillo JA. Updated American College of Critical Care Medicine-pediatric advanced life support guidelines for management of pediatric and neonatal septic shock. Pediatr Emerg Care (2010) 26:867–9. doi: 10.1097/PEC.0b013e3181fb0dc0
87. Oliveira CF, Nogueira de Sá FR, Oliveira SF, Gottschald FC, Moura DG, Shibata RO, et al. Time- and fluid-sensitive resuscitation for hemodynamic support of children in septic shock. Pediatr Emerg Care (2008) 24:810–5. doi: 10.1097/PEC.0b013e31818e9f3a
88. de Oliveira CFD, de Oliveira SF, Gottschald FC, Moura DG, Costa GA, Ventura AC, et al. ACCM/PALS haemodynamic support guidelines for paediatric septic shock: an outcomes comparison with and without monitoring central venous oxygen saturation. Intens Care Med. (2008) 34:1065–75. doi: 10.1007/s00134-008-1085-9
89. Peake SL, Delaney A, Bailey M, Bellomo R, ARISE Investigators. Goal-directed resuscitation for patients with early septic shock. N Engl J Med. (2014) 371:1496–506. doi: 10.1056/NEJMoa1404380
90. Mouncey PR, Osborn TM, Power GS, Harrison DA, Sadique MZ, Grieve RD, et al. Trial of early, goal-directed resuscitation for septic shock. N Engl J Med. (2015) 372:1301–11. doi: 10.1056/NEJMoa1500896
91. Yealy DM, Kellum JA, Huang DT, Barnato AE, Weissfeld LA, Pike F, et al. A randomized trial of protocol-based care for early septic shock. N Engl J Med. (2014) 370:1683–93. doi: 10.1056/NEJMoa1401602
92. Dellinger RP, Levy MM, Rhodes A, Annane D, Gerlach H, Opal SM, et al. Surviving sepsis campaign: international guidelines for management of severe sepsis and septic shock 2012. Intens Care Med. (2013) 39:165–228. doi: 10.1007/s00134-012-2769-8
93. Yu H, Chi D, Wang S, Liu B. Effect of early goal-directed therapy on mortality in patients with severe sepsis or septic shock: a meta-analysis of randomised controlled trials. BMJ Open (2016) 6:e008330. doi: 10.1136/bmjopen-2015-008330
94. Zhang L, Zhu G, Han L, Fu P. Early goal-directed therapy in the management of severe sepsis or septic shock in adults: a meta-analysis of randomized controlled trials. BMC Med. (2015) 13:71. doi: 10.1186/s12916-015-0312-9
95. Workman JK, Ames SG, Reeder RW, Korgenski EK, Masotti SM, Bratton SL, et al. Treatment of pediatric septic shock with the surviving sepsis campaign guidelines and PICU patient outcomes*. Pediatr Crit Care Med. (2016) 17:e451–8. doi: 10.1097/PCC.0000000000000906
96. Carcillo JA. Intravenous fluid choices in critically ill children. Curr Opin Crit Care (2014) 20:396–401. doi: 10.1097/MCC.0000000000000119
97. Pediatric Acute Respiratory Distress Syndrome: Consensus Recommendations From the Pediatric Acute Lung Injury Consensus Conference. Pediatr Crit Care Med. (2015) 16: 428–39. doi: 10.1097/PCC.0000000000000350
98. Rhodes A, Evans LE, Alhazzani W, Levy MM, Antonelli M, Ferrer R, et al. Surviving sepsis campaign: international guidelines for management of sepsis and septic shock: 2016. Intens Care Med. (2017) 43:304–77. doi: 10.1007/s00134-017-4683-6
99. Booy R. Reduction in case fatality rate from meningococcal disease associated with improved healthcare delivery. Arch Dis Child. (2001) 85:386–90. doi: 10.1136/adc.85.5.386
100. Maitland K, Pamba A, English M, Peshu N, Marsh K, Newton C, et al. Randomized Trial of volume expansion with albumin or saline in children with severe malaria: preliminary evidence of albumin benefit. Clin Infect Dis. (2005) 40:538–45. doi: 10.1086/427505
101. Weiss SL, Keele L, Balamuth F, Vendetti N, Ross R, Fitzgerald JC, et al. Crystalloid fluid choice and clinical outcomes in pediatric sepsis: a matched retrospective cohort study. J Pediatr. (2017) 182:304–10.e10. doi: 10.1016/j.jpeds.2016.11.075
102. Sutherland A, Jemmett E, Playfor S. The impact of fixed concentrations sedation infusions on fluid overload in critically ill children. Arch Dis Child. (2016) 101:e2.43–e2. doi: 10.1136/archdischild-2016-311535.48
103. Oh GJ, Sutherland SM. Perioperative fluid management and postoperative hyponatremia in children. Pediatr Nephrol. (2015) 31:53–60. doi: 10.1007/s00467-015-3081-y
104. Wang J, Xu E, Xiao Y. Isotonic versus hypotonic maintenance IV fluids in hospitalized children: a meta-analysis. Pediatrics (2013) 133:105–13. doi: 10.1542/peds.2013-2041
105. Goldstein S, Bagshaw S, Cecconi M, Okusa M, Wang H, Kellum J, et al. Pharmacological management of fluid overload. Br J Anaesthes. (2014) 113:756–63. doi: 10.1093/bja/aeu299
106. Wald EL, Finer G, McBride ME, Nguyen N, Costello JM, Epting CL. Fluid management. Pediatr Crit Care Med. (2016) 17:S257–65. doi: 10.1097/PCC.0000000000000781
107. van der Voort PHJ, Boerma EC, Koopmans M, Zandberg M, de Ruiter J, Gerritsen RT, et al. Furosemide does not improve renal recovery after hemofiltration for acute renal failure in critically ill patients: a double blind randomized controlled trial*. Crit Care Med. (2009) 37:533–8. doi: 10.1097/CCM.0b013e318195424d
108. Chawla LS, Davison DL, Brasha-Mitchell E, Koyner JL, Arthur JM, Shaw AD, et al. Development and standardization of a furosemide stress test to predict the severity of acute kidney injury. Crit Care (2013) 17:R207. doi: 10.1186/cc13015
109. Kidney Disease: Improving Global Outcomes (KDIGO) CKD-MBD Update Work GrouKDIGO 2017 Clinical Practice Guideline Update for the Diagnosis, Evaluation Prevention, and Treatment of Chronic Kidney Disease–Mineral and Bone Disorder (CKD-MBD). Kidney International Supplements (2017) 7:e1.
110. Axelrod DM, Anglemyer AT, Sherman-Levine SF, Zhu A, Grimm C, Roth SJ, et al. Initial Experience using aminophylline to improve renal dysfunction in the pediatric cardiovascular ICU. Pediatr Crit Care Med. (2014) 15:21–7. doi: 10.1097/01.pcc.0000436473.12082.2f
111. Moffett BS, Mott AR, Nelson DP, Goldstein SL, Jefferies JL. Renal effects of fenoldopam in critically ill pediatric patients: a retrospective review. Pediatr Crit Care Med. (2008) 9:403–6. doi: 10.1097/PCC.0b013e3181728c25
112. Gan H, Cannesson M, Chandler JR, Ansermino JM. Predicting fluid responsiveness in children. Anesthes Analges. (2013) 117:1380–92. doi: 10.1213/ANE.0b013e3182a9557e
113. Saxena R, Durward A, Steeley S, Murdoch IA, Tibby SM. Predicting fluid responsiveness in 100 critically ill children: the effect of baseline contractility. Intens Care Med. (2015) 41:2161–9. doi: 10.1007/s00134-015-4075-8
Keywords: fluid overload, acute kidney injury, critical care, pediatric nephrology, intensive care
Citation: Raina R, Sethi SK, Wadhwani N, Vemuganti M, Krishnappa V and Bansal SB (2018) Fluid Overload in Critically Ill Children. Front. Pediatr. 6:306. doi: 10.3389/fped.2018.00306
Received: 14 June 2018; Accepted: 28 September 2018;
Published: 29 October 2018.
Edited by:
Ibrahim F. Shatat, Sidra Medical and Research Center, QatarReviewed by:
Brian Christopher Bridges, Vanderbilt University, United StatesMichael L. Moritz, University of Pittsburgh School of Medicine, United States
Copyright © 2018 Raina, Sethi, Wadhwani, Vemuganti, Krishnappa and Bansal. This is an open-access article distributed under the terms of the Creative Commons Attribution License (CC BY). The use, distribution or reproduction in other forums is permitted, provided the original author(s) and the copyright owner(s) are credited and that the original publication in this journal is cited, in accordance with accepted academic practice. No use, distribution or reproduction is permitted which does not comply with these terms.
*Correspondence: Rupesh Raina, rraina@akronchildrens.org; raina@akronnephrology.com
†These authors share first authorship