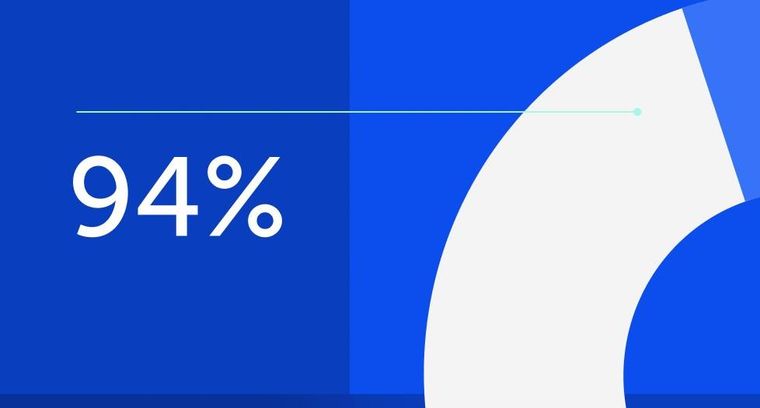
94% of researchers rate our articles as excellent or good
Learn more about the work of our research integrity team to safeguard the quality of each article we publish.
Find out more
MINI REVIEW article
Front. Pediatr., 09 October 2018
Sec. Neonatology
Volume 6 - 2018 | https://doi.org/10.3389/fped.2018.00285
This article is part of the Research TopicSepsis in Neonates and ChildrenView all 23 articles
Sepsis is a leading cause of mortality and morbidity in neonates. Presenting clinical symptoms are unspecific. Sensitivity and positive predictive value of biomarkers at onset of symptoms are suboptimal. Clinical suspicion therefore frequently leads to empirical antibiotic therapy in uninfected infants. The incidence of culture confirmed early-onset sepsis is rather low, around 0.4–0.8/1000 term infants in high-income countries. Six to 16 times more infants receive therapy for culture-negative sepsis in the absence of a positive blood culture. Thus, culture-negative sepsis contributes to high antibiotic consumption in neonatal units. Antibiotics may be life-saving for the few infants who are truly infected. However, overuse of broad-spectrum antibiotics increases colonization with antibiotic resistant bacteria. Antibiotic therapy also induces perturbations of the non-resilient early life microbiota with potentially long lasting negative impact on the individual‘s own health. Currently there is no uniform consensus definition for neonatal sepsis. This leads to variations in management. Two factors may reduce the number of culture-negative sepsis cases. First, obtaining adequate blood cultures (0.5–1 mL) at symptom onset is mandatory. Unless there is a strong clinical or biochemical indication to prolong antibiotics physician need to trust the culture results and to stop antibiotics for suspected sepsis within 36–48 h. Secondly, an international robust and pragmatic neonatal sepsis definition is urgently needed. Neonatal sepsis is a dynamic condition. Rigorous evaluation of clinical symptoms (“organ dysfunction”) over 36–48 h in combination with appropriately selected biomarkers (“dysregulated host response”) may be used to support or refute a sepsis diagnosis.
Neonatal sepsis is a clinical manifestation of a systemic infection during the first 28 days of life, usually classified as early-onset (<48–72 h) and late-onset sepsis (>48–72 h), depending on the age at onset of the sepsis episode (1). The inability to isolate a microbial pathogen does not exclude sepsis (2, 3). The 2016 consensus definition of sepsis in adults (Sepsis-3) states that sepsis is “a life-threatening condition caused by a dysregulated host response to infection and organ dysfunction” (3). There are also ongoing discussions regarding an adapted definition of pediatric sepsis beyond the neonatal period, similar to the Sepsis-3 definition (4, 5). However, there is unfortunately no uniform consensus definition for neonatal sepsis (2, 6, 7). Many neonates are therefore diagnosed with a “probable or possible” sepsis or a “presumed symptomatic infection but no bacterial cause identified” (8); conditions often referred to as “culture-negative sepsis”(9).
Most publications on neonatal sepsis from high-income countries only include culture-confirmed cases. The large number of neonates treated with antibiotics for a culture-negative sepsis are largely ignored in epidemiological studies. Thus, there is a paucity of data on the latter condition. However, neonates with culture-negative sepsis contribute to the high antibiotic consumption seen in neonatal units (10, 11). Moreover, there is a wide variation in antibiotic use between neonatal intensive care units (NICUs) and countries, with no difference in infection-attributable morbidity and mortality (10, 12). This indicates probable overuse of antibiotics in some NICUs, which again may have important adverse short- and long-term consequences for the infant's individual health (13–15). On the other hand, delayed antibiotic therapy in truly infected neonates may result in increased mortality and morbidity (16). Therefore, culture-negative neonatal sepsis is at the crossroad between efficient sepsis care and antimicrobial stewardship programs.
The main objective of this paper is to critically review the controversial term culture-negative neonatal sepsis (9, 17), with a focus on early-onset neonatal sepsis (EONS). We discuss how we can combine efficient sepsis care with antimicrobial stewardship during first days and weeks of life. Finally, we will discuss possible strategies for defining EONS in the absence of a positive blood culture.
In most high-income countries, the incidence of culture-confirmed EONS has decreased or remained relatively stable around 0.4–0.8 cases per 1000 live-born term infants over the last decade (10, 18–21). Although the incidence of EONS is substantially higher in preterm infants, the majority of patients with EONS are delivered at term (22). Robust epidemiological data on culture-negative sepsis are limited. A number of different studies published since 2012 report that infants receiving systemic antibiotic therapy for culture-negative sepsis outnumber infants receiving therapy for culture-confirmed sepsis by a factor of six to 16 (Table 1). The reason for the high number of culture-negative cases is not clear, and diagnostic criteria used in the different publications vary substantially (10, 11, 23, 24, 26, 27). Low levels of bacteremia or only small volumes of blood obtained from sick infants may be one explanation for the high number of culture-negative sepsis cases. Also maternal antibiotic treatment before or during delivery may theoretically in some cases mask detection of bacteremia in the newborn. However, one must also question whether over-diagnosis of sepsis among non-infected infants is an alternative explanation.
Table 1. Reported cases of culture-confirmed vs. culture-negative neonatal sepsis in selected studies published after 2012.
Survivors of neonatal sepsis may suffer short- and long-term consequences (28, 29). Delayed recognition and therapy of sepsis increase the risk of morbidity and mortality and early antibiotic treatment of suspected sepsis is a corner stone of every sepsis care bundle (30). Therefore, clinicians have a low threshold to suspect neonatal EONS and to start empirical antibiotic therapy. Most probably due to a lack of a clear definition and a robust gold standard of the diagnosis neonatal sepsis, started therapy is often continued for 5–7 days, even in the absence of a positive blood culture, to minimize the risk of “partial treatment” of a potential true infection (11).
Maternal group B streptococcal (GBS) colonization in the current pregnancy, GBS bacteriuria, a previous infant with invasive GBS disease, prolonged rupture of membranes (PROM; ≥18 h) and maternal fever (temperature ≥38°C; often interpreted as a sign of chorioamnionitis) are the risk factors most commonly associated with EONS. These risk factors are additive; the presence of more than one factor increases the likelihood of EONS (31, 32). EONS prevention strategies using a risk factor based approach suggest starting empiric antibiotics based on the presence of one or more risk factors. These strategies decrease the incidence of GBS-EONS (33, 34), but have poor predictive ability, leading to high number of mothers and infants being treated with antibiotics. A quantitative model assessing the indication for empiric therapy including degree of maternal fever, duration of PROM and degree of prematurity seem to perform better, and may reduce the number of infants receiving antibiotics (35).
Reports and guidelines differ in their recommendations on how to act on risk factor based strategies (36). The NICE-guidelines do not specify whether the use of intrapartum antibiotic prophylaxis alter the subsequent EONS risk assessment and infant evaluation (8). In contrast, an EONS sepsis calculator developed by Escobar and co-workers incorporates that intrapartum antibiotic given >2–4 h prior to delivery ameliorates the clinical recommendations regarding EONS risk, and subsequent infant management (37). A recent international survey regarding neonatal sepsis showed a broad variability in management of neonates with only risk factors, whereas a newborn with risk factors in combination with clinical signs were uniformly started on antibiotic therapy (36).
EONS generally presents itself with respiratory distress, apnea, lethargy or irritability, temperature instability, and feeding difficulties. These symptoms are unspecific as many non-infected neonates display similar symptoms. During the first days of life, there is a dynamic adaption of different organ systems to extra-uterine life. A single-point, clinical assessment to diagnose EONS therefore seems impossible. Some guidelines suggest that a respiratory rate > 50 or 60/min may be suggestive of an infection (Table 2). However, in healthy infants the 95th percentile for the respiratory rate is 65/min at 2 h of age (41). Moreover, non-infected early term infants (37–38 weeks gestation) born by cesarean section have high rates of transient tachypnea (42). Feeding difficulties are also notoriously difficult to assess during the first 1–2 days of life. A capillary refill time > 2 s is included as a sign of EONS in some sepsis criteria (27, 43), while observational studies indicate that the normal refill time in neonates is at least 3 s (44, 45). These examples clearly show the limitations with single-point assessments of clinical signs. In preterm infants, symptoms from respiratory distress syndrome or clinical instability may be impossible to differentiate from sepsis. However, a number of preterm infants are delivered by cesarean section, before rupture of membranes and with no maternal signs of infection. Among these there is a very low risk for EONS (46). Recognizing a “low-risk situation” may provide the opportunity to limit routine empiric antibiotics for preterm infants even when there is some degree of respiratory distress. Nevertheless, there is a broad consensus to start antibiotic therapy in newborns with clinical signs suggestive of possible EONS (36)—the major challenge is when to diagnose this as sepsis or to discard a sepsis diagnosis and stop therapy!
In this paragraph we will focus on inflammatory markers that are commonly used and commercially available today, namely the complete blood cell (CBC) count, the C-reactive protein (CRP), procalcitonin (PCT), and to some extent interleukins (e.g., interleukin [IL]-6 and IL-8). It is not possible within the scope of this article to review all biomarkers used for diagnostics, and we refer to recent reviews on this topic (47). In the future, rapid bedside point-of care tests using e.g., microarray chips to quantify multiple cytokines and acute phase reactants and other “multi-omic” approaches may become excellent and improved diagnostic tools (47, 48).
The diagnostic value of the CBC-count and differential with regard to neonatal sepsis has been extensively evaluated (7, 49, 50). In general, CBC components are neither very sensitive nor specific for EONS (49, 50). The white blood cell (WBC) and the absolute neutrophil counts (ANC) are most informative when very low (49, 50). There is a very wide range between the lower and upper normal limits for WBC and ANC. Increased band form neutrophils, represented by high immature-to-total neutrophil ratio (IT-ratio), is often suggested to indicate sepsis. However, large intra- and inter-laboratory variation in interpretation limits its use (49, 51). Early onset thrombocytopenia is commonly associated with feto-maternal conditions and not very predictive for an infection (52). In the setting of an EONS evaluation, a low platelet count has a low sensitivity and a low positive predictive value (49, 53). Thus, CBC components used alone are inaccurate to define neonatal sepsis in the absence of a positive blood culture (7).
CRP is the most extensively studied acute-phase reactant in neonates. It is widely available and its determination is simple, fast, and cost-effective (54, 55). The mean CRP level in healthy term infants undergoes a physiological rise from ~1 mg/L at 12 h of age to ~4 mg/L at 48 h of life (55). Preterm infants have a less pronounced CRP response compared to term infants (56). A CRP cut-off value at 10 mg/L is often suggested as the upper normal limit (54). However, the 95th percentile of CRP in healthy infants at 48 h of age is 12–14 mg/L (55, 57, 58). Moreover, non-infected infants may have CRP values up to at least 40–50 mg/L, in particular after vaginal delivery (58, 59). Thus, a substantial number of healthy infants will have CRP values > 10 mg/L. CRP levels at the time of initial EONS-evaluation have a low sensitivity (25), because EONS usually originates during labor or at delivery and it then takes 12–24 h for CRP to rise (54). The positive predictive value of an elevated CRP value > 10 mg/L for possible or proven EONS has also consistently been low. This is mainly because there are multiple non-infectious conditions associated with an inflammatory reaction in the perinatal period, including a complicated labor and delivery, meconium aspiration, intraventricular hemorrhage, and tissue injury (25, 54, 60).
Over the last decade, PCT has emerged as a promising and increasingly used sepsis biomarker in neonates. PCT, like CRP, does not cross the placenta; hence is not affected by maternal fever in labor (61). PCT levels start to rise 2 h after start of a septic insult and peak by 12 h (62). The earlier rise after onset of the infectious stimulus makes PCT an attractive alternative to CRP (63). However, there has been few high-quality studies reporting data on the clinical usefulness of PCT at disease onset in “ruling in” EONS (63). Moreover, the interpretation of PCT values is challenging due to a strong physiological rise and fall during the first 72 h of life. Other perinatal factors, such as chorioamnionitis, hypoxemia, perinatal asphyxia, and maternal preeclampsia, can also cause PCT to increase (64). Dynamic reference values of PCT in neonates with and without EONS have been published (57, 65, 66).
Interleukins, predominantly IL-6 and IL-8, are used routinely in some countries and are considered as sensitive “early warning biomarkers” (47, 67, 68). The advantage of using interleukins is the early spike after an infectious insult that may add in early diagnosis. However, due to their short half-life one may not catch the peak level and this may limit sensitivity. Thus, when used they should always be combined with a “later marker” e.g., CRP (67).
In summary, the positive predictive values of the above-mentioned inflammatory markers are relatively low. The reason for this is mainly due to perinatal inflammatory reactions triggered by factors during delivery or in the early postnatal period. Still, many clinicians use abnormal inflammatory markers at disease onset as one reason to prolong antibiotic therapy in infants with a negative blood culture (36).
The duration of antimicrobial therapy in infants with negative blood cultures is controversial, and there is little evidence to inform practice (8, 31). In the SCOUT-study researchers assessed antibiotic consumption for different conditions among 2500 term and preterm infants (11). Culture-proven infection and necrotizing enterocolitis (NEC) accounted for only 6.9% of antibiotic use. In contrast, prolonged (≥ 5 days) antibiotic therapy for pneumonia or culture-negative sepsis accounted for 26% of total antibiotic use and therapy was continued for median (range) seven (5–14) days for these conditions. The UK NICE-guidelines states that the usual duration of antibiotic treatment for babies with a positive blood culture, and for those with a negative blood culture but in whom there has been strong suspicion of sepsis, should be 7 days (8). In observational studies, infants with culture-negative sepsis are commonly treated for 5–7 days and mortality is very low (10, 11, 23). However, due to variable sepsis definitions it is not possible to assess “safety” vs. a clear possibility of harm due to overtreatment (69). A recent study from India compared the efficacy of 7 vs. 10 days duration of intravenous antibiotics for culture-proven septic neonates and found no difference (70).
There is growing evidence that prolonged antibiotic therapy in preterm infants without proven infection increases the risk of mortality, bronchopulmonary dysplasia, NEC, retinopathy, and periventricular white matter damage (13, 71). The question if antibiotic therapy is a perpetrator or an innocent bystander is still not completely clear, but we must seriously consider that the “administration of antibiotics itself may contribute to mortality and morbidity among preterm infants” (72). It has also been known for decades that overuse of antibiotics paves the way for development of multi-resistant bacteria and there is an urgent call for rational use of antibiotics (73). Unfortunately, the cost of antibiotic resistance for the community compared to the potential risk of delayed or insufficient treated infection is a weak argument for the clinician at the bedside. With the growing knowledge of antibiotic use and the cost on the individual‘s own health via the collateral damage on their microbiota, this may change (74). Recent data indicate that the perturbations of the non-resilient microbiota in early life may influence both the immune system and stem cell populations with potential negative impact on future health (75–77). Therefore, pediatricians and neonatologists need to measure risk and benefits of every dose of antibiotics for culture-negative situations. The hospitals and lead clinicians in neonatal units have an independent responsibility to educate and keep staff updated about current knowledge of the harmful effects of unnecessary antibiotic use in the neonatal period (78). Recent publications reporting results from antimicrobial stewardship programs in NICUs highlight both the potential for improving antibiotic prescription practice, but also challenges when trying to reduce broad-spectrum antibiotic therapy in vulnerable very preterm infants (79, 80).
A positive microbial culture from a normally sterile site (blood) is frequently used as the gold standard to define neonatal sepsis (2). In the UK, around 50% of all blood cultures obtained in the neonatal period were taken on the day of birth, but only 0.8% of these were positive (81). Thus, the majority of blood cultures are negative in neonates who are evaluated with risk factors or clinical signs of EONS. What is the sensitivity of blood cultures in neonates? Schelonka analyzed, in a laboratory-based study, a range of blood volumes containing known concentrations of common neonatal pathogens injected into pediatric blood culture bottles. If organisms were present at very low densities [< 4 colony forming units (cfu)/mL] a blood culture volume of 1.0 mL was needed to detect bacteremia. Otherwise, blood culture volumes down to 0.5 mL were sufficient to detect most bacteremia's. In a similar in-vitro study, placental blood seeded with more than 10 cfu/mL of E. coli or GBS required only 0.25 mL blood to be consistently detected (82). Thus, blood cultures with a volume of 1.0 mL have excellent sensitivity even when the infant has low very levels of bacteremia, and blood culture volumes down to as little as 0.5 mL may be sufficient to detect moderate and high grade bacteremia (83). Some authorities recommend obtaining at least two cultures (aerobic and anaerobic) before commencing antibiotics, but there are no neonatal data to support this, and usual practice is to take only one aerobic blood culture bottle before starting antibiotic treatment (84).
Quantitative blood culture methodology (cfu/mL) is not routinely used. However, blood culture time to positivity (TTP) correlates to level of bacterial density as TTP is inversely proportional to the inoculated bacterial concentration. Using modern continuous monitoring blood culture system, median TTP of blood cultures in neonatal sepsis is 9–18 h for true pathogenic bacteria (85–89). For GBS and E. coli around 96–100% are positive by 36 h (85, 86, 89), whereas coagulase negative staphylococci may take up to 48 h to be detected. Maternal intrapartum antibiotic therapy does not seem to delay TTP (85, 90). Thus, considering TTP aids in clinical interpretation of blood culture results and is the reason why central guidelines suggest stopping antibiotics if culture results are negative at 36–48 h (8, 31). Ensuring a sufficient blood culture volume (minimum 0.5 mL) and adequate routines for 24/7 immediate entry of blood culture bottles into the blood culture system are essential elements in this diagnostic algorithm (84, 87, 91).
Modern molecular methods may potentially represent more rapid, sensitive, and specific ways of identifying bacteria. These methods include conventional and real-time polymerase chain reaction (PCR) assays targeted at universal and specific gene sites. A systematic review from 2017 concluded that molecular assays have the advantage of producing rapid results and may perform well as “add-on” tests, but cannot replace microbial cultures in the diagnosis of neonatal sepsis (92, 93). This may change in the future. A recent publication reports promising results using multiplex real-time PCR in late-onset neonatal sepsis with results potentially available within 4 h of blood sampling and good concordance with blood culture results (94).
In contrast to the low positive predictive value, the negative predictive value of some inflammatory markers like CRP and PCT, and in combination with ILs, are good (26, 54, 67). Available evidence indicates that PCT may have the highest negative predictive value for severe, invasive bacterial infections in neonates (95, 96). Some high-quality intervention studies also show superiority of biomarker-guided duration of antibiotic therapy in neonatal (early- or late-onset) sepsis compared to a standard protocol (26, 67, 68, 97). The NICE-guideline states that if continuing antibiotics for longer than 36 h despite negative blood cultures, one should review the baby at least once every 24 h. Each day one should consider whether it is appropriate to stop antibiotic treatment, taking account of the level of initial clinical suspicion of infection, the baby's clinical progress and current condition, and whether there are “reassuring” levels and trends of CRP (8). One potential weakness with this high-quality guideline is the lack of discussion around which CRP values that can be interpreted as “reassuring.” After implementing the NICE guidelines recommending measuring CRP 18–24 h after commencing antibiotics, one report in fact showed that this led to more investigations and longer duration of antibiotic treatment (98).
A recent large randomized trial showed that PCT-guided decision-making was superior to standard care in reducing antibiotic therapy in neonates with suspected EONS. Clinicians may still “struggle” to become familiar with postnatal age specific PCT cut-off values over the first few 72 h of life which may confound the interpretation of a negative and a positive PCT value for the diagnosis of EONS. Therefore, a web-based guide for PCT-guided decision-making will hopefully be published in 2018-2019 (personal communication). A survey performed in 2011-2012 in Europe, North-America and Australia showed that PCT at that time was not as widely used as CRP to diagnose EONS (36). However, like for CRP, PCT seems to be an excellent additional tool to rule out EONS as long as age specific values are taken into account.
The International Classification of Diseases, 10th Revision (ICD-10), defines different subtypes of bacterial sepsis in the newborn (P36). When specific pathogens are identified in the blood culture, the codes P36.0-8 are applied. For all other cases, e.g., when the clinician “believes” the newborn has sepsis the code P36.9 “unspecified bacterial sepsis” is applied. Regional differences in the use of P36.9 clearly exemplifies the need for a uniform sepsis definition (10). Both the US and the UK EONS-guidelines present the typical clinical symptoms that may indicate EONS, but do not specify clinical criteria defining sepsis in the absence of a positive blood culture (8, 31). Examples of some suggested neonatal sepsis criteria are presented in Table 2.
How can we define sepsis in neonates with a negative blood culture? Current adult sepsis definition is a “dysregulated host response to infection and organ dysfunction.” An adoption of this definition for neonatal sepsis may be possible, but the terms “dysregulated host response,” “infection,” and “organ dysfunction” need to be defined for the neonatal population. Interestingly, there are no risk factors included in the new adult Sepsis-3 definition, although risk factors also exist for sepsis in the adult population. Risk factors for EONS are well anchored in the sepsis framework for pediatricians and neonatologists. Clinical symptoms of sepsis may overlap with non-inflammatory conditions and we suggest that parameters reflecting a host inflammatory response (“dysregulated host response”) need to be included in order to establish a sepsis diagnosis in the absence of growth in a blood culture. Moreover, the biochemical picture of neonatal sepsis changes over the first days after antibiotics has been commenced for a suspected sepsis episode, and the kinetics of inflammatory biomarkers could also be included in a future sepsis definition. Finally, there is an urgent need for an international definition of organ dysfunction in neonates, which for the first days of life require a dynamic evaluation. Wynn and Polin recently called for a consensus definition of neonatal sepsis (5). As a group of European authors on neonatal sepsis we strongly agree with this call for action.
Two important factors may substantially reduce the number of cases coined as culture-negative sepsis.
First, available evidence indicate that blood cultures in neonates are highly sensitive, at least down to volumes of 0.5–1.0 mL. In neonatal units with available equipment and resources for obtaining blood cultures this diagnostic procedure must always be performed prior to commencing antibiotic therapy, with adequate aseptic technique and drawing a sufficient volume, preferably 1 mL and minimum 0.5 mL (9). We suggest to clearly record in the patient chart the blood culture volume taken. Automated 24-h electronic systems for reporting negative or positive (i.e., growth detected) blood culture results at 36–48 h incubation should be implemented in each hospital to support clinical decision-making of stopping antibiotics. Unless there is a strong clinical or biochemical indication to prolong antibiotics physician need to trust the culture results when an adequate volume is obtained and to stop antibiotics for suspected sepsis within 36–48 h. Our recommendations are in line with a recently published perspective article entitled “ending the culture of culture-negative sepsis in the neonatal ICU” where the authors urgently ask clinicians to trust negative cultures (9).
Second, a robust and pragmatic neonatal sepsis definition may reduce subjective variations in antibiotic use and support clinicians providing “appropriate” antibiotic therapy to those infants who need antibiotics. EONS is a dynamic and complex condition. A static definition of clinical symptoms reflecting organ dysfunction at a single point (disease onset) is most likely too unspecific to define EONS and guide antibiotic therapy. A combination of risk factors, symptoms at disease onset combined with development of symptoms upon evaluation after 36–48 h is an alternative approach. This would concomitantly be in line with clinical guidelines strongly suggesting reviewing the infant and deciding whether one should stop treatment of suspected EONS after 36–48 h. Escobar and co-workers developed an electronic sepsis calculator applicable for newborns ≥34 weeks' gestation including both quantitative maternal risk factors and clinical symptoms after birth. Implementation of this calculator in routine clinical care has been associated with a substantial and concomitantly and probably safe reduction in the number of infants commenced on antibiotics for suspected EONS in several countries (35, 99, 100). We believe a similar strategy for diagnosing EONS; including development of clinical symptoms over 36–48 h combined with a biomarker-guided approach may further reduce the unnecessary prolonged duration of antibiotic treatment. CRP and PCT, optionally combined with interleukin 6 or 8, are currently the most attractive biomarkers for this purpose.
In conclusion, there is a need to align the road toward efficient sepsis care with the road toward antimicrobial stewardship in the neonatal unit. Early antibiotic therapy for truly infected newborns is crucial for an optimal outcome. On the other hand, prolonged antibiotic therapy for non-infected newborns is harmful for their microbiota, for their future health and for the community.
CK wrote the first draft of the manuscript. RK, GB, RM, and MS critically revised the manuscript for important intellectual content. All authors read and approved the final manuscript.
The authors declare that the research was conducted in the absence of any commercial or financial relationships that could be construed as a potential conflict of interest.
1. Shane AL, Sanchez PJ, Stoll BJ. Neonatal sepsis. Lancet (2017) 390:1770–80. doi: 10.1016/S0140-6736(17)31002-4
2. Wynn JL, Polin RA. Progress in the management of neonatal sepsis: the importance of a consensus definition. Pediatr Res. (2018) 83:13–5. doi: 10.1038/pr.2017.224
3. Singer M, Deutschman CS, Seymour CW, Shankar-Hari M, Annane D, Bauer M, et al. The third international consensus definitions for sepsis and septic shock (Sepsis-3). JAMA (2016) 315:801–10. doi: 10.1001/jama.2016.0287
4. Schlapbach LJ, Kissoon N. Defining pediatric sepsis. JAMA Pediatr. (2018) 172:312–4. doi: 10.1001/jamapediatrics.2017.5208
5. Schlapbach LJ. Time for sepsis-3 in children? Pediatr Crit Care Med. (2017) 18:805–6. doi: 10.1097/PCC.0000000000001203
6. Haque KN. Definitions of bloodstream infection in the newborn. Pediatr Crit Care Med. (2005) 6(3 Suppl.):S45–9. doi: 10.1097/01.PCC.0000161946.73305.0A
7. Wynn JL, Wong HR, Shanley TP, Bizzarro MJ, Saiman L, Polin RA. Time for a neonatal-specific consensus definition for sepsis. Pediatr Crit Care Med. (2014) 15:523–8. doi: 10.1097/PCC.0000000000000157
8. National Institute for Health and Care Excellence (NICE). Antibiotics for Early-onset Neonatal Infection: Antibiotics for the Prevention and Treatment of Early-Onset Neonatal Infection. London: Clinical guideline [CG149] (2012). Available online at: https://www.nice.org.uk/guidance/cg149
9. Cantey JB, Baird SD. Ending the culture of culture-negative sepsis in the neonatal ICU. Pediatrics (2017) 140:e20170044. doi: 10.1542/peds.2017-0044
10. Fjalstad JW, Stensvold HJ, Bergseng H, Simonsen GS, Salvesen B, Ronnestad AE, et al. Early-onset sepsis and antibiotic exposure in term infants: a nationwide population-based study in Norway. Pediatr Infect Dis J. (2016) 35:1–6. doi: 10.1097/INF.0000000000000906
11. Cantey JB, Wozniak PS, Pruszynski JE, Sanchez PJ. Reducing unnecessary antibiotic use in the neonatal intensive care unit (SCOUT): a prospective interrupted time-series study. Lancet Infect Dis. (2016) 16:1178–84. doi: 10.1016/S1473-3099(16)30205-5
12. Schulman J, Dimand RJ, Lee HC, Duenas GV, Bennett MV, Gould JB. Neonatal intensive care unit antibiotic use. Pediatrics (2015) 135:826–33. doi: 10.1542/peds.2014-3409
13. Esaiassen E, Fjalstad JW, Juvet LK, van den Anker JN, Klingenberg C. Antibiotic exposure in neonates and early adverse outcomes: a systematic review and meta-analysis. J Antimicrob Chemother. (2017) 72:1858–70. doi: 10.1093/jac/dkx088
14. Mitre E, Susi A, Kropp LE, Schwartz DJ, Gorman GH, Nylund CM. Association between use of acid-suppressive medications and antibiotics during infancy and allergic diseases in early childhood. JAMA Pediatr. (2018) 172:e180315. doi: 10.1001/jamapediatrics.2018.2513
15. Rasmussen SH, Shrestha S, Bjerregaard LG, Angquist LH, Baker JL, Jess T, et al. Antibiotic exposure in early life and childhood overweight and obesity: a systematic review and meta-analysis. Diabetes Obes Metab. (2018) 20:1508–14. doi: 10.1111/dom.13230
16. Cordery RJ, Roberts CH, Cooper SJ, Bellinghan G, Shetty N. Evaluation of risk factors for the acquisition of bloodstream infections with extended-spectrum beta-lactamase-producing Escherichia coli and Klebsiella species in the intensive care unit; antibiotic management and clinical outcome. J Hosp Infect. (2008) 68:108–15. doi: 10.1016/j.jhin.2007.10.011
17. Cantey JB, Sanchez PJ. Prolonged antibiotic therapy for “culture-negative” sepsis in preterm infants: it's time to stop! J Pediatr. (2011) 159:707–8. doi: 10.1016/j.jpeds.2011.07.032
18. Stoll BJ, Hansen NI, Sanchez PJ, Faix RG, Poindexter BB, Van Meurs KP, et al. Early onset neonatal sepsis: the burden of group B Streptococcal and E. coli disease continues. Pediatrics (2011) 127:817–26. doi: 10.1542/peds.2010-2217
19. Singh T, Barnes EH, Isaacs D. Early-onset neonatal infections in Australia and New Zealand, 2002-2012. Arch Dis Child Fetal Neonatal Ed. (2018). doi: 10.1136/archdischild-2017-314671. [Epub ahead of print].
20. Schrag SJ, Farley MM, Petit S, Reingold A, Weston EJ, Pondo T, et al. Epidemiology of invasive early-onset neonatal sepsis, 2005 to 2014. Pediatrics (2016) 138:e20162013. doi: 10.1542/peds.2016-2013
21. Bekker V, Bijlsma MW, van de Beek D, Kuijpers TW, van der Ende A. Incidence of invasive group B streptococcal disease and pathogen genotype distribution in newborn babies in the Netherlands over 25 years: a nationwide surveillance study. Lancet Infect Dis. (2014) 14:1083–9. doi: 10.1016/S1473-3099(14)70919-3
22. Cailes B, Kortsalioudaki C, Buttery J, Pattnayak S, Greenough A, Matthes J, et al. Epidemiology of UK neonatal infections: the neonIN infection surveillance network. Arch Dis Child Fetal Neonatal Ed. (2017). doi: 10.1136/archdischild-2017-313203. [Epub ahead of print].
23. Drageset M, Fjalstad JW, Mortensen S, Klingenberg C. Management of early-onset neonatal sepsis differs in the north and south of Scandinavia. Acta Paediatr. (2017) 106:375–81. doi: 10.1111/apa.13698
24. Hofer N, Muller W, Resch B. Neonates presenting with temperature symptoms: role in the diagnosis of early onset sepsis. Pediatr Int. (2012) 54:486–90. doi: 10.1111/j.1442-200X.2012.03570.x
25. Lacaze-Masmonteil T, Rosychuk RJ, Robinson JL. Value of a single C-reactive protein measurement at 18 h of age. Arch Dis Child Fetal Neonatal Ed. (2014) 99:F76–9. doi: 10.1136/archdischild-2013-303984
26. Stocker M, van Herk W, El Helou S, Dutta S, Fontana MS, Schuerman FABA, et al. Procalcitonin-guided decision making for duration of antibiotic therapy in neonates with suspected early-onset sepsis: a multicentre, randomized controlled trial (NeoPIns). Lancet (2017) 390:871–81. doi: 10.1016/S0140-6736(17)31444-7
27. Duvoisin G, Fischer C, Maucort-Boulch D, Giannoni E. Reduction in the use of diagnostic tests in infants with risk factors for early-onset neonatal sepsis does not delay antibiotic treatment. Swiss Med Wkly. (2014) 144:w13981. doi: 10.4414/smw.2014.13981
28. Bakhuizen SE, de Haan TR, Teune MJ, van Wassenaer-Leemhuis AG, van der Heyden JL, van der Ham DP, et al. Meta-analysis shows that infants who have suffered neonatal sepsis face an increased risk of mortality and severe complications. Acta Paediatr. (2014) 103:1211–8. doi: 10.1111/apa.12764
29. Schlapbach LJ, Aebischer M, Adams M, Natalucci G, Bonhoeffer J, Latzin P, et al. Impact of sepsis on neurodevelopmental outcome in a Swiss National Cohort of extremely premature infants. Pediatrics (2011) 128:e348–57. doi: 10.1542/peds.2010-3338
30. Weiss SL, Fitzgerald JC, Balamuth F, Alpern ER, Lavelle J, Chilutti M, et al. Delayed antimicrobial therapy increases mortality and organ dysfunction duration in pediatric sepsis. Crit Care Med. (2014) 42:2409–17. doi: 10.1097/CCM.0000000000000509
31. Polin RA. Management of neonates with suspected or proven early-onset bacterial sepsis. Pediatrics (2012) 129:1006–15. doi: 10.1542/peds.2012-0541
32. Jefferies AL. Management of term infants at increased risk for early-onset bacterial sepsis. Paediatr Child Health (2017) 22:223–8. doi: 10.1093/pch/pxx023
33. Trijbels-Smeulders M, de Jonge GA, Pasker-de Jong PC, Gerards LJ, Adriaanse AH, van Lingen RA, et al. Epidemiology of neonatal group B streptococcal disease in the Netherlands before and after introduction of guidelines for prevention. Arch Dis Child Fetal Neonatal Ed. (2007) 92:F271–6. doi: 10.1136/adc.2005.088799
34. Schrag SJ, Zell ER, Lynfield R, Roome A, Arnold KE, Craig AS, et al. A population-based comparison of strategies to prevent early-onset group B streptococcal disease in neonates. N Engl J Med. (2002) 347:233–9. doi: 10.1056/NEJMoa020205
35. Kuzniewicz MW, Puopolo KM, Fischer A, Walsh EM, Li S, Newman TB, et al. A quantitative, risk-based approach to the management of neonatal early-onset sepsis. JAMA Pediatr. (2017) 171:365–71. doi: 10.1001/jamapediatrics.2016.4678
36. van Herk W, el Helou S, Janota J, Hagmann C, Klingenberg C, Staub E, et al. Variation in current management of term and late-preterm neonates at risk for early-onset sepsis: an international survey and review of guidelines. Pediatr Infect Dis J. (2016) 35:494–500. doi: 10.1097/INF.0000000000001063
37. Puopolo KM, Draper D, Wi S, Newman TB, Zupancic J, Lieberman E, et al. Estimating the probability of neonatal early-onset infection on the basis of maternal risk factors. Pediatrics (2011) 128:e1155–63. doi: 10.1542/peds.2010-3464
38. Goldstein B, Giroir B, Randolph A. International pediatric sepsis consensus conference: definitions for sepsis and organ dysfunction in pediatrics. Pediatr Crit Care Med. (2005) 6:2–8. doi: 10.1097/00130478-200501000-00049
39. Oeser C, Lutsar I, Metsvaht T, Turner MA, Heath PT, Sharland M. Clinical trials in neonatal sepsis. J Antimicrob Chemother. (2013) 68:2733–45. doi: 10.1093/jac/dkt297
40. Hakansson S, Lilja M, Jacobsson B, Kallen K. Reduced incidence of neonatal early-onset group B streptococcal infection after promulgation of guidelines for risk-based intrapartum antibiotic prophylaxis in Sweden: analysis of a national population-based cohort. Acta Obstet Gynecol Scand. (2017) 96:1475–83. doi: 10.1111/aogs.13211
41. Tveiten L, Diep LM, Halvorsen T, Markestad T. Respiratory rate during the first 24 hours of life in healthy term infants. Pediatrics (2016) 137:e20152326. doi: 10.1542/peds.2015-2326
42. Doan E, Gibbons K, Tudehope D. The timing of elective caesarean deliveries and early neonatal outcomes in singleton infants born 37-41 weeks' gestation. Aust N Z J Obstet Gynaecol. (2014) 54:340–7. doi: 10.1111/ajo.12220
43. Koch L, Bosk A, Sasse M, Ruef P, Poeschl J. Managing neonatal severe sepsis in Germany: a preliminary survey of current practices. Klin Padiatr. (2015) 227:23–7. doi: 10.1055/s-0034-1389975
44. Raju NV, Maisels MJ, Kring E, Schwarz-Warner L. Capillary refill time in the hands and feet of normal newborn infants. Clin Pediatr. (1999) 38:139–44. doi: 10.1177/000992289903800303
45. Strozik KS, Pieper CH, Roller J. Capillary refilling time in newborn babies: normal values. Arch Dis Child Fetal Neonatal Ed. (1997) 76:F193–6. doi: 10.1136/fn.76.3.F193
46. Puopolo KM, Mukhopadhyay S, Hansen NI, Cotten CM, Stoll BJ, Sanchez PJ, et al. Identification of extremely premature infants at low risk for early-onset sepsis. Pediatrics (2017) 140:e20170925. doi: 10.1542/peds.2017-0925
47. Ng PC, Ma TP, Lam HS. The use of laboratory biomarkers for surveillance, diagnosis and prediction of clinical outcomes in neonatal sepsis and necrotising enterocolitis. Arch Dis Child Fetal Neonatal Ed. (2015) 100:F448–52. doi: 10.1136/archdischild-2014-307656
48. Ng S, Strunk T, Jiang P, Muk T, Sangild PT, Currie A. Precision medicine for neonatal sepsis. Front Mol Biosci. (2018) 5:70. doi: 10.3389/fmolb.2018.00070
49. Hornik CP, Benjamin DK, Becker KC, Benjamin DK Jr, Li J, Clark RH, et al. Use of the complete blood cell count in early-onset neonatal sepsis. Pediatr Infect Dis J. (2012) 31:799–802. doi: 10.1097/INF.0b013e318256905c
50. Newman TB, Puopolo KM, Wi S, Draper D, Escobar GJ. Interpreting complete blood counts soon after birth in newborns at risk for sepsis. Pediatrics (2010) 126:903–9. doi: 10.1542/peds.2010-0935
51. van der Meer W, van Gelder W, de Keijzer R, Willems H. Does the band cell survive the 21st century? Eur J Haematol. (2006) 76:251–4. doi: 10.1111/j.1600-0609.2005.00597.x
52. Del Vecchio A. Evaluation and management of thrombocytopenic neonates in the intensive care unit. Early Hum Dev. (2014) 90(Suppl. 2):S51–5. doi: 10.1016/S0378-3782(14)50014-X
53. Spector SA, Ticknor W, Grossman M. Study of the usefulness of clinical and hematologic findings in the diagnosis of neonatal bacterial infections. Clin Pediatr. (1981) 20:385–92. doi: 10.1177/000992288102000602
54. Hofer N, Zacharias E, Muller W, Resch B. An update on the use of C-reactive protein in early-onset neonatal sepsis: current insights and new tasks. Neonatology (2012) 102:25–36. doi: 10.1159/000336629
55. Perrone S, Lotti F, Longini M, Rossetti A, Bindi I, Bazzini F, et al. C reactive protein in healthy term newborns during the first 48 hours of life. Arch Dis Child Fetal Neonatal Ed. (2018) 103:F163–6. doi: 10.1136/archdischild-2016-312506
56. Turner MA, Power S, Emmerson AJ. Gestational age and the C reactive protein response. Arch Dis Child Fetal Neonatal Ed. (2004) 89:F272–3. doi: 10.1136/adc.2002.011288
57. Chiesa C, Natale F, Pascone R, Osborn JF, Pacifico L, Bonci E, et al. C reactive protein and procalcitonin: reference intervals for preterm and term newborns during the early neonatal period. Clin Chim Acta (2011) 412:1053–9. doi: 10.1016/j.cca.2011.02.020
58. Chiesa C, Signore F, Assumma M, Buffone E, Tramontozzi P, Osborn JF, et al. Serial measurements of C-reactive protein and interleukin-6 in the immediate postnatal period: reference intervals and analysis of maternal and perinatal confounders. Clin Chem. (2001) 47:1016–22.
59. Ishibashi M, Takemura Y, Ishida H, Watanabe K, Kawai T. C-reactive protein kinetics in newborns: application of a high-sensitivity analytic method in its determination. Clin Chem. (2002) 48:1103–6.
60. Hofer N, Muller W, Resch B. Non-infectious conditions and gestational age influence C-reactive protein values in newborns during the first 3 days of life. Clin Chem Lab Med. (2011) 49:297–302. doi: 10.1515/CCLM.2011.048
61. Robinson P, De SK. How to use. Procalcitonin. Arch Dis Child Educ Pract Ed. (2018) 103:257–62. doi: 10.1136/archdischild-2017-313699
62. Dandona P, Nix D, Wilson MF, Aljada A, Love J, Assicot M, et al. Procalcitonin increase after endotoxin injection in normal subjects. J Clin Endocrinol Metab. (1994) 79:1605–8.
63. Chiesa C, Pacifico L, Osborn JF, Bonci E, Hofer N, Resch B. Early-onset neonatal sepsis: still room for improvement in procalcitonin diagnostic accuracy studies. Medicine (2015) 94:e1230. doi: 10.1097/MD.0000000000001230
64. Chiesa C, Pellegrini G, Panero A, Osborn JF, Signore F, Assumma M, et al. C-reactive protein, interleukin-6, and procalcitonin in the immediate postnatal period: influence of illness severity, risk status, antenatal and perinatal complications, and infection. Clin Chem. (2003) 49:60–8. doi: 10.1373/49.1.60
65. Stocker M, Fontana M, El HS, Wegscheider K, Berger TM. Use of procalcitonin-guided decision-making to shorten antibiotic therapy in suspected neonatal early-onset sepsis: prospective randomized intervention trial. Neonatology (2010) 97:165–74. doi: 10.1159/000241296
66. Chiesa C, Panero A, Rossi N, Stegagno M, De GM, Osborn JF, et al. Reliability of procalcitonin concentrations for the diagnosis of sepsis in critically ill neonates. Clin Infect Dis. (1998) 26:664–72. doi: 10.1086/514576
67. Franz AR, Steinbach G, Kron M, Pohlandt F. Reduction of unnecessary antibiotic therapy in newborn infants using interleukin-8 and C-reactive protein as markers of bacterial infections. Pediatrics (1999) 104(3 Pt 1):447–53.
68. Franz AR, Steinbach G, Kron M, Pohlandt F. Interleukin-8: a valuable tool to restrict antibiotic therapy in newborn infants. Acta Paediatr. (2001) 90:1025–32. doi: 10.1111/j.1651-2227.2001.tb01359.x
69. Fjalstad JW, Esaiassen E, Juvet LK, van den Anker JN, Klingenberg C. Antibiotic therapy in neonates and impact on gut microbiota and antibiotic resistance development: a systematic review. J Antimicrob Chemother. (2017) 73:569–80. doi: 10.1093/jac/dkx426
70. Rohatgi S, Dewan P, Faridi MMA, Kumar A, Malhotra RK, Batra P. Seven versus 10 days antibiotic therapy for culture-proven neonatal sepsis: a randomized controlled trial. J Paediatr Child Health (2017) 53:556–62. doi: 10.1111/jpc.13518
71. Ting JY, Synnes A, Roberts A, Deshpandey A, Dow K, Yoon EW, et al. Association between antibiotic use and neonatal mortality and morbidities in very low-birth-weight infants without culture-proven sepsis or necrotizing enterocolitis. JAMA Pediatr. (2016) 170:1181–7. doi: 10.1001/jamapediatrics.2016.2132
72. Mukhopadhyay S, Puopolo KM. Antibiotic use and mortality among premature infants without confirmed infection-perpetrator or innocent bystander? JAMA Pediatr. (2016) 170:1144–6. doi: 10.1001/jamapediatrics.2016.2836
73. Laxminarayan R, Duse A, Wattal C, Zaidi AK, Wertheim HF, Sumpradit N, et al. Antibiotic resistance-the need for global solutions. Lancet Infect Dis. (2013) 13:1057–98. doi: 10.1016/S1473-3099(13)70318-9
74. Blaser MJ. Antibiotic use and its consequences for the normal microbiome. Science (2016) 352:544–5. doi: 10.1126/science.aad9358
75. Gensollen T, Iyer SS, Kasper DL, Blumberg RS. How colonization by microbiota in early life shapes the immune system. Science (2016) 352:539–44. doi: 10.1126/science.aad9378
76. Gohir W, Ratcliffe EM, Sloboda DM. Of the bugs that shape us: maternal obesity, the gut microbiome, and long-term disease risk. Pediatr Res. (2015) 77:196–204. doi: 10.1038/pr.2014.169
77. Schulfer A, Blaser MJ. Risks of antibiotic exposures early in life on the developing microbiome. PLoS Pathog. (2015) 11:e1004903. doi: 10.1371/journal.ppat.1004903
78. Goff DA, Kullar R, Bauer KA, File TM Jr. Eight habits of highly effective antimicrobial stewardship programs to meet the joint commission standards for hospitals. Clin Infect Dis. (2017) 64:1134–9. doi: 10.1093/cid/cix065
79. Nzegwu NI, Rychalsky MR, Nallu LA, Song X, Deng Y, Natusch AM, et al. Implementation of an antimicrobial stewardship program in a neonatal intensive care unit. Infect Control Hosp Epidemiol. (2017) 38:1137–43. doi: 10.1017/ice.2017.151
80. Ting JY, Paquette V, Ng K, Lisonkova S, Hait V, Shivanada S, et al. Reduction of inappropriate antimicrobial prescriptions in a tertiary neonatal intensive care unit following antimicrobial stewardship care bundle implementation. Pediatr Infect Dis J. (2018). doi: 10.1097/INF.0000000000002039. [Epub ahead of print].
81. Blackburn RM, Muller-Pebody B, Planche T, Johnson A, Hopkins S, Sharland M, et al. Neonatal sepsis–many blood samples, few positive cultures: implications for improving antibiotic prescribing. Arch Dis Child Fetal Neonatal Ed. (2012) 97:F487–8. doi: 10.1136/archdischild-2012-302261
82. Brown DR, Kutler D, Rai B, Chan T, Cohen M. Bacterial concentration and blood volume required for a positive blood culture. J Perinatol. (1995) 15:157–9.
83. Buttery JP. Blood cultures in newborns and children: optimizing an everyday test. Arch Dis Child Fetal Neonatal Ed. (2002) 87:F25–8. doi: 10.1136/fn.87.1.F25
84. Paul SP, Caplan EM, Morgan HA, Turner PC. Barriers to implementing the NICE guidelines for early-onset neonatal infection: cross-sectional survey of neonatal blood culture reporting by laboratories in the UK. J Hosp Infect. (2018) 98:425–8. doi: 10.1016/j.jhin.2017.12.015
85. Garcia-Prats JA, Cooper TR, Schneider VF, Stager CE, Hansen TN. Rapid detection of microorganisms in blood cultures of newborn infants utilizing an automated blood culture system. Pediatrics (2000) 105(3 Pt 1):523–7.
86. Jardine L, Davies MW, Faoagali J. Incubation time required for neonatal blood cultures to become positive. J Paediatr Child Health (2006) 42:797–802. doi: 10.1111/j.1440-1754.2006.00980.x
87. Jardine MA, Kumar Y, Kausalya S, Harigopal S, Wong J, Shivaram A, et al. Reducing antibiotic use on the neonatal unit by improving communication of blood culture results: a completed audit cycle. Arch Dis Child Fetal Neonatal Ed. (2003) 88:F255. doi: 10.1136/fn.88.3.F255-a
88. Vamsi SR, Bhat RY, Lewis LE, Vandana KE. Time to positivity of blood cultures in neonates. Pediatr Infect Dis J. (2014) 33:212–4. doi: 10.1097/INF.0000000000000018
89. Giannoni E, Agyeman PKA, Stocker M, Posfay-Barbe KM, Heininger U, Spycher BD, et al. Neonatal sepsis of early onset, and hospital-acquired and community-acquired late onset: a prospective population-based cohort study. J Pediatr. (2018) 201:106–14.e4. doi: 10.1016/j.jpeds.2018.05.048
90. Sarkar SS, Bhagat I, Bhatt-Mehta V, Sarkar S. Does maternal intrapartum antibiotic treatment prolong the incubation time required for blood cultures to become positive for infants with early-onset sepsis? Am J Perinatol. (2015) 32:357–62. doi: 10.1055/s-0034-1387933
91. Jardine LA, Sturgess BR, Inglis GD, Davies MW. Neonatal blood cultures: effect of delayed entry into the blood culture machine and bacterial concentration on the time to positive growth in a simulated model. J Paediatr Child Health (2009) 45:210–4. doi: 10.1111/j.1440-1754.2008.01455.x
92. Pammi M, Flores A, Leeflang M, Versalovic J. Molecular assays in the diagnosis of neonatal sepsis: a systematic review and meta-analysis. Pediatrics (2011) 128:e973–85. doi: 10.1542/peds.2011-1208
93. Pammi M, Flores A, Versalovic J, Leeflang MM. Molecular assays for the diagnosis of sepsis in neonates. Cochrane Database Syst Rev. (2017) 2:CD011926. doi: 10.1002/14651858.CD011926.pub2
94. van den Brand M, van den Dungen FAM, Bos MP, van Weissenbruch MM, van Furth AM, de LA, et al. Evaluation of a real-time PCR assay for detection and quantification of bacterial DNA directly in blood of preterm neonates with suspected late-onset sepsis. Crit Care (2018) 22:105. doi: 10.1186/s13054-018-2010-4
95. van Rossum AM, Wulkan RW, Oudesluys-Murphy AM. Procalcitonin as an early marker of infection in neonates and children. Lancet Infect Dis. (2004) 4:620–30. doi: 10.1016/S1473-3099(04)01146-6
96. Vouloumanou EK, Plessa E, Karageorgopoulos DE, Mantadakis E, Falagas ME. Serum procalcitonin as a diagnostic marker for neonatal sepsis: a systematic review and meta-analysis. Intensive Care Med. (2011) 37:747–62. doi: 10.1007/s00134-011-2174-8
97. Ehl S, Gering B, Bartmann P, Hogel J, Pohlandt F. C-reactive protein is a useful marker for guiding duration of antibiotic therapy in suspected neonatal bacterial infection. Pediatrics (1997) 99:216–21. doi: 10.1542/peds.99.2.216
98. Mukherjee A, Davidson L, Anguvaa L, Duffy DA, Kennea N. NICE neonatal early onset sepsis guidance: greater consistency, but more investigations, and greater length of stay. Arch Dis Child Fetal Neonatal Ed. (2015) 100:F248–9. doi: 10.1136/archdischild-2014-306349
99. Achten NB, Dorigo-Zetsma JW, van der Linden PD, van BM, Plotz FB. Sepsis calculator implementation reduces empiric antibiotics for suspected early-onset sepsis. Eur J Pediatr. (2018) 177:741–6. doi: 10.1007/s00431-018-3113-2
Keywords: neonate, sepsis, blood culture, C-reactive protein, procalcitonin
Citation: Klingenberg C, Kornelisse RF, Buonocore G, Maier RF and Stocker M (2018) Culture-Negative Early-Onset Neonatal Sepsis — At the Crossroad Between Efficient Sepsis Care and Antimicrobial Stewardship. Front. Pediatr. 6:285. doi: 10.3389/fped.2018.00285
Received: 22 June 2018; Accepted: 17 September 2018;
Published: 09 October 2018.
Edited by:
Charles Christoph Roehr, University of Oxford, United KingdomReviewed by:
Joseph Ting, University of British Columbia, CanadaCopyright © 2018 Klingenberg, Kornelisse, Buonocore, Maier and Stocker. This is an open-access article distributed under the terms of the Creative Commons Attribution License (CC BY). The use, distribution or reproduction in other forums is permitted, provided the original author(s) and the copyright owner(s) are credited and that the original publication in this journal is cited, in accordance with accepted academic practice. No use, distribution or reproduction is permitted which does not comply with these terms.
*Correspondence: Claus Klingenberg, Y2xhdXMua2xpbmdlbmJlcmdAdW5uLm5v
Disclaimer: All claims expressed in this article are solely those of the authors and do not necessarily represent those of their affiliated organizations, or those of the publisher, the editors and the reviewers. Any product that may be evaluated in this article or claim that may be made by its manufacturer is not guaranteed or endorsed by the publisher.
Research integrity at Frontiers
Learn more about the work of our research integrity team to safeguard the quality of each article we publish.