- 1University of British Columbia and BC Children’s Hospital, Vancouver, BC, Canada
- 2Department of Pediatrics, Faculty of Medicine, King Abdulaziz University, Jeddah, Saudi Arabia
- 3University of Washington, Seattle, WA, United States
Cytomegalovirus (CMV) is a ubiquitous infection that causes disease in congenitally infected children and immunocompromised patients. Although nearly all CMV infections remain latent and asymptomatic in immunologically normal individuals, numerous studies have found that systemic viral reactivation is common in immunocompetent critically ill adults, as measured by detection of CMV in the blood (viremia). Furthermore, CMV viremia is strongly correlated with adverse outcomes in the adult intensive care unit (ICU), including prolonged stay, duration of mechanical ventilation, and death. Increasing evidence, including from a randomized clinical trial of antiviral treatment, suggests that these effects of CMV may be causal. Therefore, interventions targeting CMV might improve outcomes in adult ICU patients. CMV may have an even greater impact on critically ill children, particularly in low and middle income countries (LMIC), where CMV is regularly acquired in early childhood, and where inpatient morbidity and mortality are inordinately high. However, to date, there are few data regarding the clinical relevance of CMV infection or viremia in immunocompetent critically ill children. We propose that CMV infection should be studied as a potential modifiable cause of disease in critically ill children, and that these studies be conducted in LMIC. Below, we briefly review the role of CMV in immunologically normal critically ill adults and children, outline age-dependent differences in CMV infection that may influence ICU outcomes, and describe an agenda for future research.
Introduction
Cytomegalovirus (CMV) is a double-stranded DNA herpesvirus that infects most of the world’s population (1, 2). Natural transmission is mostly through contact with viral shedding in breast milk, saliva, urine, or genital fluids (3–5). Similar to other herpesviruses, acute CMV infection is associated with high levels of lytic viral replication and dissemination throughout the body, followed by the establishment of viral latency in long-lived cell types that is responsible for lifelong persistence of infection (6).
Cytomegalovirus infection is typically diagnosed by serology (1, 7, 8). In addition to CMV IgG, use of CMV IgM and serial IgG avidity testing may help determine the timing of infection in selected situations (7, 9, 10). However, in infants, CMV serologic testing can be confounded presence of maternal IgG acquired passively in utero (3, 8). Therefore, diagnosis in infants requires either seroconversion (a change from negative to positive serologic testing) or, more often, by the detection of CMV in blood, saliva, or urine (3, 8). Isolation of CMV in culture or detection of CMV DNA or antigen in patient samples reflects lytic viral replication and is termed “active” infection (1, 2). Active CMV infection in a chronically infected person may be due to either reactivation of latent virus or reinfection with an exogenous strain of CMV (1, 2, 11, 12).
Acute CMV infection in healthy children or adults is nearly always asymptomatic or limited to mild non-specific illness. Periodic mucosal CMV reactivation and viral shedding is not uncommon during chronic infection, but systemic replication or viremia (detection of CMV in blood) is rare in immunologically normal individuals (4, 13, 14). By contrast, CMV viremia and end-organ disease is common in congenitally infected children and immunocompromised patients (15–18). More recent findings also suggest that CMV might have pervasive negative impact on health through indirect effects on the immune system (19–21). In particular, CMV has increasingly been recognized as a potential cause of disease in immunologically normal adults with critical illness (22–25). However, despite the fact that young children generally control CMV infection poorly compared to adults, little is known about the role of CMV in immunocompetent critically ill pediatric patients. Below, we review the evidence for CMV causing adverse clinical outcomes in immunocompetent critically ill adults, and provide a rationale for analogous studies to examine the impact of CMV on critical illness in immunocompetent children.
Systemic CMV Reactivation in Immunocompetent Critically Ill Adults
Observational Studies Linking CMV Viremia With Adverse Adult Critical Care Outcomes
There have been more than 30 studies of immunologically normal, critically ill adult patients demonstrating an association between systemic CMV reactivation and worse outcomes in intensive care units (ICUs), as summarized in recent reviews (22–25). In these studies, CMV reactivation was defined as the detection of CMV in blood samples or, when feasible, in bronchoalveolar lavage (BAL) fluid, using CMV DNA PCR, antigen immunocytochemistry, or growth in shell vial culture. CMV viremia occurs in approximately 35% of seropositive adults following admission to the ICU. By contrast, fewer than 1% of adult blood donors with chronic CMV infection have detectable viremia (13, 14). CMV viremia and viral load are positively correlated with worse outcomes, including prolonged ICU stay, length of mechanical ventilation, secondary infections, and death. CMV viremia occurs over a wide spectrum of ICU admission diagnoses, such as infection, trauma, burns, and major surgery (26–35). In particular, patients with bacterial sepsis or pneumonia have been reported to have a higher likelihood of CMV viremia (28, 33, 36). The risk of CMV viremia is not clearly related to disease severity at the time of admission based on prognostic and organ dysfunction scores, and the onset of CMV viremia tends to precede or accompany clinical decline (37, 38), which support the possibility that CMV causes, rather than merely being a marker of, worse ICU outcomes in adults (23–25).
Mechanisms by Which Critical Illness May Cause CMV Viremia
Critical illness may impair immune control of CMV replication normally mediated by cytotoxic T cells and natural killer (NK) cells (39–41). Adults with sepsis show depletion of splenic T cells and reduced T cell responsiveness (42). Functional NK cell differences have also been reported between patients with septic shock or systemic inflammatory response syndrome and healthy controls (43). Furthermore, among patients with septic shock, those with CMV reactivation were found to have reduced NK cell function (44).
Exposure of latently infected cells to a pro-inflammatory environment contributes to CMV reactivation (6). Thus, inflammation, e.g., due to trauma or sepsis, may initiate CMV reactivation in critically ill patients, and CMV replication then might in turn drive more inflammation in a feed-forward manner (Figure 1). CMV replication is associated with production of pro-inflammatory cytokines, including interleukin (IL)-1, IL-6, IL-8, and TNF-α (45–47). In a cohort of young adults, IL-6 levels were significantly higher in the sera of CMV seropositive compared to seronegative subjects (48, 49). Interestingly, CMV-infected adults were more likely to have a pro-inflammatory profile at 3 months after discharge from ICU, even after adjusting for illness severity and underlying conditions (50).
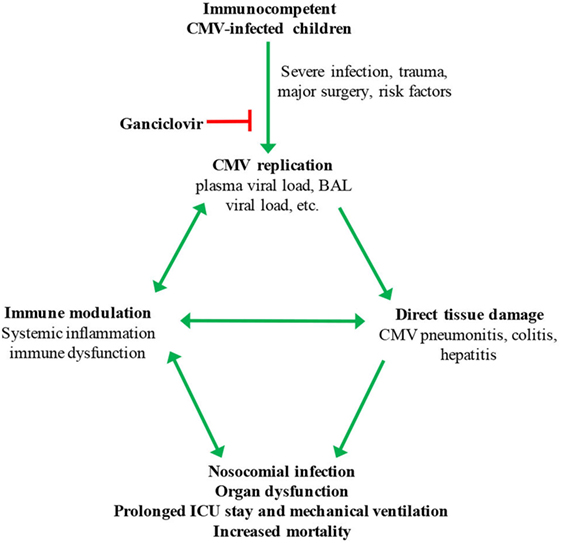
Figure 1. Hypothesized model for cytomegalovirus (CMV) reactivation in critically ill children. Possible risk factors for systemic CMV replication include severity of illness, pneumonia, malnutrition, blood transfusion, corticosteroid treatment, and young age. BAL, bronchoalveolar lavage.
Mechanisms by Which CMV Replication May Contribute to Clinical Decline
Adverse ICU outcomes often result from uncontrolled inflammation and/or impaired immunity (42). CMV infection may play a significant role in both of these processes through both direct and indirect mechanisms.
Cytomegalovirus replication in tissues of immunocompromised individuals is a well-recognized cause of end-organ damage, including pneumonitis, hepatitis, and colitis (11, 16–18). Although direct cytolytic effects of viral replication in tissues are central to the pathogenesis of CMV infection in the immunocompromised host, its role in immunocompetent critically ill individuals is relatively poorly defined. Some studies have reported frequent pulmonary CMV replication based on testing of BAL fluid and lung biopsies (26, 51). Histopathologic evidence of CMV colitis was also found in immunocompetent adult patients admitted to ICU for other causes (52). In addition, CMV has been reported to cause kidney and liver damage in immunocompetent adult ICU patients (53–55). Murine studies add further support to the hypothesis that CMV replication contributes to acute lung injury in adults with sepsis or severe trauma. Sepsis in immunocompetent mice causes murine CMV reactivation in the lungs, as well as pulmonary inflammation and fibrosis that is prevented by treatment with the antiviral drug ganciclovir (56, 57).
In transplant recipients, CMV is associated with the so-called “indirect” effects on the immune system, including increased risk of bacterial and fungal infections (52, 58, 59). Some studies suggest that CMV-induced immunomodulation may increase the risk of nosocomial infections in immunocompetent ICU patients (22, 53). These findings would seem to be consistent with our emerging understanding of the profound impact CMV infection has on the immune system generally (21, 60, 61).
Interventional Studies of CMV Infection in Immunocompetent Critically Ill Adults
Randomized clinical trials exploring the effect of CMV treatment on outcomes in adult ICU patients are ongoing, and have the ability to determine the causality of CMV in these associations (22, 62). A recent open-label randomized trial was conducted among CMV seropositive, immunocompetent mechanically ventilated patients in a single ICU in the UK. Participants were assigned to receive prophylactic low-dose valganciclovir, high-dose valacyclovir, or no treatment (63). The primary outcome was time to CMV viremia by PCR. As expected, the antiviral groups were less likely to develop viremia (8 versus 35% in controls). This study demonstrates the efficacy of prophylactic antiviral use to prevent CMV viremia in ICU patients. However, the study was not powered to measure differences in clinical outcomes. Of note, the valacyclovir arm was discontinued early due to higher mortality, though all deaths were judged to be expected and attributable to underlying disease rather than any adverse antiviral effects.
Subsequently, a phase 2, randomized controlled multicenter trial of prophylactic ganciclovir or valganciclovir versus placebo in immunocompetent CMV seropositive adult ICU patients with respiratory failure and severe sepsis or trauma was published (64). The study failed to detect a difference in the primary outcome, blood IL-6 level, which was chosen based on the reported association of elevated IL-6 with ICU mortality and ventilator-associated lung injury (65, 66). However, in addition to reduced viremia, subjects in the antiviral treatment arm had significantly more ventilation-free days, a predetermined secondary outcome. This effect was particularly marked in the subgroup admitted for sepsis. A composite end point of mortality and increased serum IL-6 by 50% or >7 days ventilation was significantly higher in placebo group, and there was a trend toward increased mortality in the placebo group. Notably, antiviral treatment was not associated with significant side effects. This study provides the strongest evidence to date for a causal association between CMV viremia and worse outcomes in and provides preliminary support for the possibility that antiviral treatment may be beneficial for selected immunocompetent adult ICU patients (62).
The Potential Role of CMV Infection in Immunocompetent Critically Ill Children
Age-Dependent Differences in CMV Pathogenesis
There are qualitative and quantitative age-dependent differences in the immune responses of young children that impair the control of many viral infections, including CMV (67–69). Children with congenital CMV infection are most often asymptomatic, but commonly present with central nervous system disease, hepatitis, hematologic abnormalities, and pneumonitis. Sensorineural hearing loss and neurodevelopmental delay develop in approximately 20% of children with congenital CMV infection (15). Postnatal CMV infection rarely causes serious illness, though extremely premature infants can develop sepsis and severe end-organ disease (70–72). Even in the absence of CMV-related disease, compared with adults primary infection, children have substantially higher levels of viral replication as well as prolonged viremia and viral shedding in saliva or urine (4, 73). Though viral replication gradually declines over time, viral shedding during chronic infection remains substantially greater in children compared to adults (4, 73). Thus, as a result of more recent infection and differences in antiviral immunity, critically ill children might be expected to have worse control of CMV replication than adults.
CMV Infection in Critically Ill Children
There are very few data available about the frequency and effects of active CMV infection in critically ill, immunocompetent children. We performed a pilot study of CMV infection in children admitted to the ICU of BC Children’s Hospital in Vancouver, Canada (unpublished data). Among the 27 children enrolled, the median age was 4 years (range 4 months–17 years). Only one child was CMV seropositive, and none were found to have viremia. This is consistent with the relatively low CMV seroprevalence among children in most high-income countries (74–77). By contrast, a study of children between 3 weeks and 2 years old hospitalized in Zambia found that 34% of HIV-uninfected children had CMV viremia (78). Of note, the prevalence of malnutrition was 36%, and being underweight was significantly associated with CMV viremia. In a retrospective study of Ugandan children 6 months–13 years old treated as outpatients for uncomplicated malaria, 11% had CMV viremia (79). Thus, even in the setting of moderate illness, systemic CMV replication is common in African children. In a prospective study that included 53 HIV-uninfected South African children ≤2 years old admitted to ICU with severe pneumonia, 12 (23%) were defined as having CMV pneumonitis based on DNA detection in BAL fluid and other clinical characteristics (80). Many of these children were treated with ganciclovir, but the published data are insufficient to determine whether disseminated CMV or antiviral treatment in HIV-uninfected children were associated with clinical outcomes (80).
Determining the Impact of CMV Infection on Pediatric ICU Outcomes
General Considerations
Well-designed cohort studies are needed to understand the role of CMV in immunocompetent critically ill children, to estimate the potential benefits, and to inform the design of appropriate pediatric treatment trials. Given the differences between children and adults in the natural history and outcomes of CMV infection, extrapolation from adult studies is inappropriate. As an example, in the adult studies conducted to date, the clinical impact of CMV has been ascribed to reactivation and dissemination of chronic infection (23–25). However, CMV viremia in pediatric ICU patients might often represent systemic viral replication related to recent infection rather than reactivation. To distinguish between reactivation of chronic infection and exacerbation of recent infection would be challenging, but might provide insights into age-dependent differences should they exist. Thus, in pediatrics studies, it would be more appropriate to explicitly evaluate the role of disseminated CMV replication as opposed to reactivation of chronic infection.
Measuring CMV Replication in Pediatric ICU Patients
Quantitative CMV DNA PCR assays can reliably detect ≤50 international units/mL and have largely replaced older methods of detection (81, 82). Because high level CMV replication in saliva and urine is so prevalent in young children (73, 83), these measures are unlikely to be clinically meaningful for critically ill children except for confirming infection in infants with maternal antibody and concern for false-positive serology. As in adult studies, CMV replication in blood is likely to be the best measure of uncontrolled systemic viral infection in critically ill children. Although CMV in BAL fluid may simply represent shedding, high viral loads are predictive of CMV pneumonitis in transplant recipients and, perhaps, in critically ill children (80, 84, 85). Thus, pediatric ICU studies should incorporate CMV testing of BAL fluid, should it be collected for clinical purposes. In addition, detection of CMV in tissue biopsies (e.g., liver, colon), particularly by histopathology indicates invasive disease, and these specimens should be tested if available (Table 1).
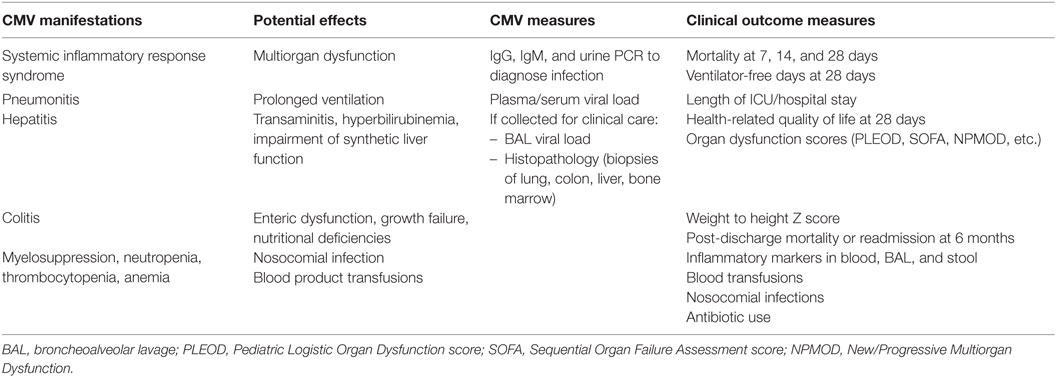
Table 1. Suggested metrics for studies of cytomegalovirus (CMV) viremia in immunocompetent pediatric intensive care unit (ICU) patients.
The risk of CMV disease is correlated to the level of viremia in many clinical scenarios. For example, in HIV-infected individuals and transplant recipients, CMV viral load is proportionally associated with progression of disease (86, 87). However, the threshold of CMV replication that is clinically relevant or that might warrant intervention depends on the patient population. For example, antiviral treatment of newborns with symptomatic congenital CMV infection is indicated, regardless of viral load, to reduce the risk of hearing loss and neurocognitive delay (8, 88–90). Selected transplant patients are monitored using CMV blood PCR in order to trigger preemptive antiviral treatment before the onset of disease, but the viral load threshold used varies based on immunologic status and other clinical criteria (87, 91, 92). The antiviral studies performed so far in adults have randomized all CMV seropositive ICU patients with the intention of preventing, rather than treating, viremia (63, 64). The optimal approach to treating CMV infection in pediatric ICU patients would depend on the associations and dose–response that are found, but might conceivably utilize risk-stratification based on age, underlying illness, viral load, or other factors.
Pediatric Populations of Greatest Interest
Studying the impact of CMV infection on critically ill immunocompetent children is anticipated to be both easier and more clinically relevant in low- and middle-income countries (LMIC), where CMV infection is holoendemic and mortality and morbidity rates from sepsis are often dismal. In LMIC, nearly all children acquire CMV infection within the first few years of life (74). By contrast, the national US survey found that while CMV prevalence increases linearly with age, it reaches only ~50% by 30 years of age (75, 76).
In addition to the relatively low rates of CMV infection in North American and European children, the excellent outcomes of pediatric ICU patients there hinder the ability to detect a clinically important impact of CMV infection on critically ill children (93–96). Furthermore, strategies to reduce mortality in critically ill children in LMIC are urgently needed. For example, in the Kenyatta National Hospital pediatric ICU in Nairobi, Kenya, overall mortality is 42% (unpublished data). Similarly, pediatric ICU mortality rates of 25–50% have been reported in other LMICs in Africa and Asia (97–100). Other outcomes of interest such as length of stay and need for invasive ventilation are also frequently higher in LMICs (99, 100). In Siriraj Hospital, one of the largest hospital in Thailand, median length of ICU stay and rate of mechanical ventilation are 4–5 days and 64%, respectively (Dr. K. Lapphra, personal communication), which are more than double those in the BC Children’s Hospital ICU. Moreover, infections and sepsis account for most of pediatric ICU admission diagnoses in LMIC, which might again predispose to disseminated CMV infection and viremia (97, 98).
Outcome Measure Considerations
Importantly, appropriate studies in children require the incorporation of pediatric-specific illness severity scores and outcome measures (Table 1). Mortality and morbidity in pediatric patients are predicted by progressive or new organ dysfunction, using validated scores (101–103). Evaluation of mechanical ventilation outcomes should include invasive as well as non-invasive modalities, which are now commonly used in children (94). A longer follow-up duration that used in adults studies should also be considered, given the high rate of readmission and post-discharge mortality in children of African and Asian countries (104–106). Higher rates of CMV viremia as well as worse post-discharge outcomes have been observed in underweight children (78, 106). Therefore, including nutritional assessments in pediatric studies is also essential.
Conclusion
Strong evidence supports the association between CMV viremia and adverse outcomes in adult ICU patients (23–25), and early-phase interventional trial results suggest that antivirals in this population might be beneficial (62–64). Unfortunately, there are no studies yet in children that have adequately addressed this question. Not only is there an ethical obligation to include children in research according to the principle of justice (107–109), but we hypothesize that active systemic CMV infection might have an even greater negative impact on ICU outcomes in children than adults. Therefore, we propose that prospective observational studies should be conducted to characterize the association between CMV viremia and clinical outcomes in critically ill children, using metrics appropriate for pediatric populations. Furthermore, we suggest that such studies would best be performed in LMICs settings. Importantly, if CMV treatment were found to be beneficial for immunocompetent critically ill children, resource limitations would not preclude its implementation (110). If successful, studies in LMICs could result in reverse innovation to help targeting specific subpopulations of patients in high-income settings that might also benefit from CMV treatment. Studies in both adults and children should attempt to identify the mechanistic basis for the relationship between CMV and critical illness, and develop novel therapies to improve outcomes of critically ill patients.
Ethics Statement
The pilot study Reactivation of Cytomegalovirus in Pediatrics (ReCIPe) for the prevalence of CMV viremia among pediatric critical care patients in BC Children’s Hospital was approved by BCCHR Ethics Board.
Author Contributions
RA: literature review and writing; SG: reviewing, editing, writing, and knowledge check; JS: reviewing statistics and literature, editing, and writing; SM: checking literature and ICU data.
Conflict of Interest Statement
The authors declare that the research was conducted in the absence of any commercial or financial relationships that could be construed as a potential conflict of interest.
Funding
The pilot study Reactivation of Cytomegalovirus in Pediatrics (ReCIPe) for the prevalence of CMV viremia among pediatric critical care patients in BC Children’s Hospital was generously funded by BC Children’s Hospital Research Institute.
References
2. Mocarski ES, Shenk T, Griffiths P, Pass RF. Cytomegaovirus. 6th ed. In: Knipe DM, Howley PM, editors. Field’s Virology. Philadelphia: Lippincott Williams & Wilkins (2013).
3. Gantt S, Orem J, Krantz EM, Morrow RA, Selke S, Huang ML, et al. Prospective characterization of the risk factors for transmission and symptoms of primary human herpesvirus infections among Ugandan infants. J Infect Dis (2016) 214:36–44. doi:10.1093/infdis/jiw076
4. Cannon MJ, Hyde TB, Schmid DS. Review of cytomegalovirus shedding in bodily fluids and relevance to congenital cytomegalovirus infection. Rev Med Virol (2011) 21:240–55. doi:10.1002/rmv.695
5. Adler SP. Cytomegalovirus transmission among children in day care, their mothers and caretakers. Pediatr Infect Dis J (1988) 7:279–85. doi:10.1097/00006454-198804000-00009
6. Reeves M, Sinclair J. Aspects of human cytomegalovirus latency and reactivation. Curr Top Microbiol Immunol (2008) 325:297–313. doi:10.1007/978-3-540-77349-8
7. Ross SA, Novak Z, Pati S, Boppana SB. Diagnosis of cytomegalovirus Infections. Infect Disord Drug Targets (2011) 11:466–74. doi:10.2174/187152611797636703
8. American Academy of Pediatrics. Cytomegalovirus Infection. In: Kimberlin DW, Brady MT, Jackson MA, Long SS, editors. Red Book® 2015: Report of the Committee on Infectious Diseases. 30th ed. Elk Grove Village, IL: American Academy of Pediatrics (2015). p. 317–22.
9. Dollard SC, Keyserling H, Radford K, Amin MM, Stowell J, Winter J, et al. Cytomegalovirus viral and antibody correlates in young children. BMC Res Notes (2014) 7:776. doi:10.1186/1756-0500-7-776
10. Prince HE, Lape-Nixon M, Novak-Weekley SM. Performance of a cytomegalovirus IgG enzyme immunoassay kit modified to measure avidity. Clin Vaccine Immunol (2014) 21:808–12. doi:10.1128/CVI.00105-14
11. Ljungman P, Griffiths P, Paya C. Definitions of cytomegalovirus infection and disease in transplant recipients. Clin Infect Dis (2002) 34:1094–7. doi:10.1086/339329
12. Boucoiran I, Mayer BT, Krantz E, Marchant A, Pati S, Boppana S, et al. Non-primary maternal CMV infection following viral shedding in infants. Pediatr Infect Dis J (2017). doi:10.1097/INF.0000000000001877
13. Roback JD, Drew WL, Laycock ME, Todd D, Hillyer CD, Busch MP. CMV DNA is rarely detected in healthy blood donors using validated PCR assays. Transfusion (2003) 43:314–21. doi:10.1046/j.1537-2995.2003.00312.x
14. Ziemann M, Thiele T. Transfusion-transmitted CMV infection – current knowledge and future perspectives. Transfus Med (2017) 27:238–48. doi:10.1111/tme.12437
15. Boppana SB, Ross SA, Fowler KB. Congenital cytomegalovirus infection: clinical outcome. Clin Infect Dis (2013) 57(Suppl 4):S178–81. doi:10.1093/cid/cit629
16. Ramanan P, Razonable RR. Cytomegalovirus infections in solid organ transplantation: a review. Infect Chemother (2013) 45:260–71. doi:10.3947/ic.2013.45.3.260
17. Bhat V, Joshi A, Sarode R, Chavan P. Cytomegalovirus infection in the bone marrow transplant patient. World J Transplant (2015) 5:287–91. doi:10.5500/wjt.v5.i4.287
18. Kovacs A, Schluchter M, Easley K, Demmler G, Shearer W, La Russa P, et al. Cytomegalovirus infection and HIV-1 disease progression in infants born to HIV-1-infected women. Pediatric pulmonary and cardiovascular complications of vertically transmitted HIV infection study group. N Engl J Med (1999) 341:77–84. doi:10.1056/NEJM199907083410203
19. Simanek AM, Dowd JB, Pawelec G, Melzer D, Dutta A, Aiello AE. Seropositivity to cytomegalovirus, inflammation, all-cause and cardiovascular disease-related mortality in the United States. PLoS One (2011) 6:e16103. doi:10.1371/journal.pone.0016103
20. Weltevrede M, Eilers R, de Melker HE, van Baarle D. Cytomegalovirus persistence and T-cell immunosenescence in people aged fifty and older: a systematic review. Exp Gerontol (2016) 77:87–95. doi:10.1016/j.exger.2016.02.005
21. Brodin P, Jojic V, Gao T, Bhattacharya S, Angel CJ, Furman D, et al. Variation in the human immune system is largely driven by non-heritable influences. Cell (2015) 160:37–47. doi:10.1016/j.cell.2014.12.020
22. Limaye AP, Boeckh M. CMV in critically ill patients: pathogen or bystander? Rev Med Virol (2010) 20:372–9. doi:10.1002/rmv.664
23. Papazian L, Hraiech S, Lehingue S, Roch A, Chiche L, Wiramus S, et al. Cytomegalovirus reactivation in ICU patients. Intensive Care Med (2016) 42:28–37. doi:10.1007/s00134-015-4066-9
24. Al-Omari A, Aljamaan F, Alhazzani W, Salih S, Arabi Y. Cytomegalovirus infection in immunocompetent critically ill adults: literature review. Ann Intensive Care (2016) 6:110. doi:10.1186/s13613-016-0207-8
25. Lachance P, Chen J, Featherstone R, Sligl WI. Association between cytomegalovirus reactivation and clinical outcomes in immunocompetent critically ill patients: a systematic review and meta-analysis. Open Forum Infect Dis (2017) 4:ofx029. doi:10.1093/ofid/ofx029
26. Chiche L, Forel JM, Roch A, Guervilly C, Pauly V, Allardet-Servent J, et al. Active cytomegalovirus infection is common in mechanically ventilated medical intensive care unit patients. Crit Care Med (2009) 37:1850–7. doi:10.1097/CCM.0b013e31819ffea6
27. Coisel Y, Bousbia S, Forel JM, Hraiech S, Lascola B, Roch A, et al. Cytomegalovirus and herpes simplex virus effect on the prognosis of mechanically ventilated patients suspected to have ventilator-associated pneumonia. PLoS One (2012) 7:e51340. doi:10.1371/journal.pone.0051340
28. Walton AH, Muenzer JT, Rasche D, Boomer JS, Sato B, Brownstein BH, et al. Reactivation of multiple viruses in patients with sepsis. PLoS One (2014) 9:e98819. doi:10.1371/journal.pone.0098819
29. Osawa R, Wagener M, Singh N. Cytomegalovirus infection in patients with sepsis due to bloodstream infections: lower risk and better outcomes in new versus already hospitalised intensive care unit admissions. Anaesth Intensive Care (2016) 44:571–80.
30. Heininger A, Jahn G, Engel C, Notheisen T, Unertl K, Hamprecht K. Human cytomegalovirus infections in nonimmunosuppressed critically ill patients. Crit Care Med (2001) 29:541–7. doi:10.1097/00003246-200103000-00012
31. Bordes J, Maslin J, Prunet B, d’Aranda E, Lacroix G, Goutorbe P, et al. Cytomegalovirus infection in severe burn patients monitoring by real-time polymerase chain reaction: a prospective study. Burns (2011) 37:434–9. doi:10.1016/j.burns.2010.11.006
32. Lopez Roa P, Hill JA, Kirby KA, Leisenring WM, Huang ML, Santo TK, et al. Coreactivation of human herpesvirus 6 and cytomegalovirus is associated with worse clinical outcome in critically ill adults. Crit Care Med (2015) 43:1415–22. doi:10.1097/CCM.0000000000000969
33. Cook CH, Martin LC, Yenchar JK, Lahm MC, McGuinness B, Davies EA, et al. Occult herpes family viral infections are endemic in critically ill surgical patients. Crit Care Med (2003) 31:1923–9. doi:10.1097/01.CCM.0000070222.11325.C4
34. Domart Y, Trouillet JL, Fagon JY, Chastre J, Brun-Vezinet F, Gibert C. Incidence and morbidity of cytomegaloviral infection in patients with mediastinitis following cardiac surgery. Chest (1990) 97:18–22. doi:10.1378/chest.97.1.18
35. Ishioka H, Sanui M, Tsutsumi Y, Yanase F, Shiotsuka J. Low prevalence of active cytomegalovirus infection in a cardiovascular intensive care unit. J Intensive Care (2014) 2:12. doi:10.1186/2052-0492-2-12
36. Heininger A, Haeberle H, Fischer I, Beck R, Riessen R, Rohde F, et al. Cytomegalovirus reactivation and associated outcome of critically ill patients with severe sepsis. Crit Care (2011) 15:R77. doi:10.1186/cc10069
37. Frantzeskaki FG, Karampi ES, Kottaridi C, Alepaki M, Routsi C, Tzanela M, et al. Cytomegalovirus reactivation in a general, nonimmunosuppressed intensive care unit population: incidence, risk factors, associations with organ dysfunction, and inflammatory biomarkers. J Crit Care (2015) 30:276–81. doi:10.1016/j.jcrc.2014.10.002
38. Kalil AC, Florescu DF. Prevalence and mortality associated with cytomegalovirus infection in nonimmunosuppressed patients in the intensive care unit. Crit Care Med (2009) 37:2350–8. doi:10.1097/CCM.0b013e3181a3aa43
39. Orr MT, Murphy WJ, Lanier LL. Unlicensed natural killer cells dominate the response to cytomegalovirus infection. Nat Immunol (2010) 11:321. doi:10.1038/ni.1849
40. La Rosa C, Diamond DJ. The immune response to human CMV. Future Virol (2012) 7:279–93. doi:10.2217/fvl.12.8
41. Klenerman P, Oxenius A. T cell responses to cytomegalovirus. Nat Rev Immunol (2016) 16:367. doi:10.1038/nri.2016.38
42. Boomer JS, To K, Chang KC, Takasu O, Osborne DF, Walton AH, et al. Immunosuppression in patients who die of sepsis and multiple organ failure. JAMA (2011) 306:2594–605. doi:10.1001/jama.2011.1829
43. Forel JM, Chiche L, Thomas G, Mancini J, Farnarier C, Cognet C, et al. Phenotype and functions of natural killer cells in critically-ill septic patients. PLoS One (2012) 7:e50446. doi:10.1371/journal.pone.0050446
44. von Muller L, Klemm A, Durmus N, Weiss M, Suger-Wiedeck H, Schneider M, et al. Cellular immunity and active human cytomegalovirus infection in patients with septic shock. J Infect Dis (2007) 196:1288–95. doi:10.1086/522429
45. Iwamoto GK, Konicek SA. Cytomegalovirus immediate early genes upregulate interleukin-6 gene expression. J Investig Med (1997) 45:175–82.
46. Craigen JL, Yong KL, Jordan NJ, MacCormac LP, Westwick J, Akbar AN, et al. Human cytomegalovirus infection up-regulates interleukin-8 gene expression and stimulates neutrophil transendothelial migration. Immunology (1997) 92:138–45. doi:10.1046/j.1365-2567.1997.00310.x
47. Humar A, St Louis P, Mazzulli T, McGeer A, Lipton J, Messner H, et al. Elevated serum cytokines are associated with cytomegalovirus infection and disease in bone marrow transplant recipients. J Infect Dis (1999) 179:484–8. doi:10.1086/314602
48. Turner JE, Campbell JP, Edwards KM, Howarth LJ, Pawelec G, Aldred S, et al. Rudimentary signs of immunosenescence in cytomegalovirus-seropositive healthy young adults. Age (Dordr) (2014) 36:287–97. doi:10.1007/s11357-013-9557-4
49. Bengner M, Beziat V, Ernerudh J, Nilsson BO, Lofgren S, Wikby A, et al. Independent skewing of the T cell and NK cell compartments associated with cytomegalovirus infection suggests division of labor between innate and adaptive immunity. Age (Dordr) (2014) 36:571–82. doi:10.1007/s11357-013-9587-y
50. Griffith DM, Lewis S, Rossi AG, Rennie J, Salisbury L, Merriweather JL, et al. Systemic inflammation after critical illness: relationship with physical recovery and exploration of potential mechanisms. Thorax (2016) 71:820–9. doi:10.1136/thoraxjnl-2015-208114
51. Papazian L, Fraisse A, Garbe L, Zandotti C, Thomas P, Saux P, et al. Cytomegalovirus. An unexpected cause of ventilator-associated pneumonia. Anesthesiology (1996) 84:280–7. doi:10.1097/00000542-199602000-00005
52. Freeman RB Jr. The ‘indirect’ effects of cytomegalovirus infection. Am J Transplant (2009) 9:2453–8. doi:10.1111/j.1600-6143.2009.02824.x
53. Jaber S, Chanques G, Borry J, Souche B, Verdier R, Perrigault PF, et al. Cytomegalovirus infection in critically ill patients: associated factors and consequences. Chest (2005) 127:233–41. doi:10.1378/chest.127.1.233
54. Squizzato A, Ageno W, Cattaneo A, Brumana N. A case report and literature review of portal vein thrombosis associated with cytomegalovirus infection in immunocompetent patients. Clin Infect Dis (2007) 44:e13–6. doi:10.1086/509641
55. Siciliano RF, Castelli JB, Randi BA, Vieira RD, Strabelli TM. Cytomegalovirus colitis in immunocompetent critically ill patients. Int J Infect Dis (2014) 20:71–3. doi:10.1016/j.ijid.2013.11.008
56. Cook CH, Zhang Y, McGuinness BJ, Lahm MC, Sedmak DD, Ferguson RM. Intra-abdominal bacterial infection reactivates latent pulmonary cytomegalovirus in immunocompetent mice. J Infect Dis (2002) 185:1395–400. doi:10.1086/340508
57. Cook CH, Zhang Y, Sedmak DD, Martin LC, Jewell S, Ferguson RM. Pulmonary cytomegalovirus reactivation causes pathology in immunocompetent mice. Crit Care Med (2006) 34:842–9. doi:10.1097/01.CCM.0000201876.11059.05
58. Prentice HG, Gluckman E, Powles RL, Ljungman P, Milpied N, Fernandez Ranada JM, et al. Impact of long-term acyclovir on cytomegalovirus infection and survival after allogeneic bone marrow transplantation. European Acyclovir for CMV Prophylaxis Study Group. Lancet (1994) 343:749–53. doi:10.1016/S0140-6736(94)91835-X
59. Roman A, Manito N, Campistol JM, Cuervas-Mons V, Almenar L, Arias M, et al. The impact of the prevention strategies on the indirect effects of CMV infection in solid organ transplant recipients. Transplant Rev (Orlando) (2014) 28:84–91. doi:10.1016/j.trre.2014.01.001
60. Beziat V, Liu LL, Malmberg JA, Ivarsson MA, Sohlberg E, Bjorklund AT, et al. NK cell responses to cytomegalovirus infection lead to stable imprints in the human KIR repertoire and involve activating KIRs. Blood (2013) 121:2678–88. doi:10.1182/blood-2012-10-459545
61. Souquette A, Frere J, Smithey M, Sauce D, Thomas PG. A constant companion: immune recognition and response to cytomegalovirus with aging and implications for immune fitness. GeroScience (2017) 39:293–303. doi:10.1007/s11357-017-9982-x
62. Kumar D, Humar A. Time to consider cytomegalovirus prevention in critically ill patients? JAMA (2017) 318:709–10. doi:10.1001/jama.2017.10132
63. Cowley NJ, Owen A, Shiels SC, Millar J, Woolley R, Ives N, et al. Safety and efficacy of antiviral therapy for prevention of cytomegalovirus reactivation in immunocompetent critically ill patients: a randomized clinical trial. JAMA Intern Med (2017) 177:774–83. doi:10.1001/jamainternmed.2017.0895
64. Limaye AP, Stapleton RD, Peng L, Gunn SR, Kimball LE, Hyzy R, et al. Effect of ganciclovir on il-6 levels among cytomegalovirus-seropositive adults with critical illness: a randomized clinical trial. JAMA (2017) 318:731–40. doi:10.1001/jama.2017.10569
65. Parsons PE, Eisner MD, Thompson BT, Matthay MA, Ancukiewicz M, Bernard GR, et al. Lower tidal volume ventilation and plasma cytokine markers of inflammation in patients with acute lung injury. Crit Care Med (2005) 33:1–6; discussion 230–2. doi:10.1097/01.CCM.0000149854.61192.DC
66. Ranieri VM, Suter PM, Tortorella C, De Tullio R, Dayer JM, Brienza A, et al. Effect of mechanical ventilation on inflammatory mediators in patients with acute respiratory distress syndrome: a randomized controlled trial. JAMA (1999) 282:54–61. doi:10.1001/jama.282.1.54
67. Prendergast AJ, Klenerman P, Goulder PJ. The impact of differential antiviral immunity in children and adults. Nat Rev Immunol (2012) 12:636–48. doi:10.1038/nri3277
68. Schleiss MR. Cytomegalovirus in the neonate: immune correlates of infection and protection. Clin Dev Immunol (2013) 2013:501801. doi:10.1155/2013/501801
69. Muller WJ, Jones CA, Koelle DM. Immunobiology of herpes simplex virus and cytomegalovirus infections of the fetus and newborn. Curr Immunol Rev (2010) 6:38–55. doi:10.2174/157339510790231833
70. Lanzieri TM, Dollard SC, Josephson CD, Schmid DS, Bialek SR. Breast milk-acquired cytomegalovirus infection and disease in VLBW and premature infants. Pediatrics (2013) 131:e1937–45. doi:10.1542/peds.2013-0076
71. Capretti MG, Lanari M, Lazzarotto T, Gabrielli L, Pignatelli S, Corvaglia L, et al. Very low birth weight infants born to cytomegalovirus-seropositive mothers fed with their mother’s milk: a prospective study. J Pediatr (2009) 154:842–8. doi:10.1016/j.jpeds.2008.12.046
72. Gunkel J, Wolfs TF, de Vries LS, Nijman J. Predictors of severity for postnatal cytomegalovirus infection in preterm infants and implications for treatment. Expert Rev Anti Infect Ther (2014) 12:1345–55. doi:10.1586/14787210.2014.966080
73. Matrajt L, Gantt S, Mayer BT, Krantz EM, Orem J, Wald A, et al. Virus and host-specific differences in oral human herpesvirus shedding kinetics among Ugandan women and children. Sci Rep (2017) 7:13105. doi:10.1038/s41598-017-12994-0
74. Cannon MJ, Schmid DS, Hyde TB. Review of cytomegalovirus seroprevalence and demographic characteristics associated with infection. Rev Med Virol (2010) 20:202–13. doi:10.1002/rmv.655
75. Staras SA, Dollard SC, Radford KW, Flanders WD, Pass RF, Cannon MJ. Seroprevalence of cytomegalovirus infection in the United States, 1988-1994. Clin Infect Dis (2006) 43:1143–51. doi:10.1086/508173
76. Bate SL, Dollard SC, Cannon MJ. Cytomegalovirus seroprevalence in the United States: the national health and nutrition examination surveys, 1988-2004. Clin Infect Dis (2010) 50:1439–47. doi:10.1086/652438
77. Hyde TB, Schmid DS, Cannon MJ. Cytomegalovirus seroconversion rates and risk factors: implications for congenital CMV. Rev Med Virol (2010) 20:311–26. doi:10.1002/rmv.659
78. Tembo J, Kabwe M, Chilukutu L, Chilufya M, Mwaanza N, Chabala C, et al. Prevalence and risk factors for betaherpesvirus DNAemia in children >3 weeks and <2 years of age admitted to a large referral hospital in sub-Saharan Africa. Clin Infect Dis (2015) 60:423–31. doi:10.1093/cid/ciu853
79. Gantt S, Huang ML, Magaret A, Bunts L, Selke S, Wald A, et al. An artesunate-containing antimalarial treatment regimen did not suppress cytomegalovirus viremia. J Clin Virol (2013) 58:276–8. doi:10.1016/j.jcv.2013.06.008
80. Govender K, Jeena P, Parboosing R. Clinical utility of bronchoalveolar lavage cytomegalovirus viral loads in the diagnosis of cytomegalovirus pneumonitis in infants. J Med Virol (2017) 89:1080–7. doi:10.1002/jmv.24730
81. Boeckh M, Huang M, Ferrenberg J, Stevens-Ayers T, Stensland L, Nichols WG, et al. Optimization of quantitative detection of cytomegalovirus DNA in plasma by real-time PCR. J Clin Microbiol (2004) 42:1142–8. doi:10.1128/JCM.42.3.1142-1148.2004
82. Kraft CS, Armstrong WS, Caliendo AM. Interpreting quantitative cytomegalovirus DNA testing: understanding the laboratory perspective. Clin Infect Dis (2012) 54:1793–7. doi:10.1093/cid/cis212
83. Stowell JD, Mask K, Amin M, Clark R, Levis D, Hendley W, et al. Cross-sectional study of cytomegalovirus shedding and immunological markers among seropositive children and their mothers. BMC Infect Dis (2014) 14:568. doi:10.1186/s12879-014-0568-2
84. Westall GP, Michaelides A, Williams TJ, Snell GI, Kotsimbos TC. Human cytomegalovirus load in plasma and bronchoalveolar lavage fluid: a longitudinal study of lung transplant recipients. J Infect Dis (2004) 190:1076–83. doi:10.1086/422327
85. Boeckh M, Stevens-Ayers T, Travi G, Huang ML, Cheng GS, Xie H, et al. Cytomegalovirus (CMV) DNA quantitation in bronchoalveolar lavage fluid from hematopoietic stem cell transplant recipients with CMV pneumonia. J Infect Dis (2017) 215:1514–22. doi:10.1093/infdis/jix048
86. Deayton JR, Sabin CA, Johnson MA, Emery VC, Wilson P, Griffiths PD. Importance of cytomegalovirus viraemia in risk of disease progression and death in HIV-infected patients receiving highly active antiretroviral therapy. Lancet (2004) 363:2116–21. doi:10.1016/S0140-6736(04)16500-8
87. Griffiths PD, Rothwell E, Raza M, Wilmore S, Doyle T, Harber M, et al. Randomized controlled trials to define viral load thresholds for cytomegalovirus pre-emptive therapy. PLoS One (2016) 11:e0163722. doi:10.1371/journal.pone.0163722
88. Kimberlin DW, Jester PM, Sanchez PJ, Ahmed A, Arav-Boger R, Michaels MG, et al. Valganciclovir for symptomatic congenital cytomegalovirus disease. N Engl J Med (2015) 372:933–43. doi:10.1056/NEJMoa1404599
89. Gantt S, Bitnun A, Renaud C, Kakkar F, Vaudry W. Diagnosis and management of infants with congenital cytomegalovirus infection. Paediatr Child Health (2017) 22:72–4. doi:10.1093/pch/pxx002
90. Rawlinson WD, Boppana SB, Fowler KB, Kimberlin DW, Lazzarotto T, Alain S, et al. Congenital cytomegalovirus infection in pregnancy and the neonate: consensus recommendations for prevention, diagnosis, and therapy. Lancet Infect Dis (2017) 17:e177–88. doi:10.1016/S1473-3099(17)30143-3
91. Solano C, Gimenez E, Pinana JL, Vinuesa V, Poujois S, Zaragoza S, et al. Preemptive antiviral therapy for CMV infection in allogeneic stem cell transplant recipients guided by the viral doubling time in the blood. Bone Marrow Transplant (2016) 51:718–21. doi:10.1038/bmt.2015.303
92. Razonable RR, Hayden RT. Clinical utility of viral load in management of cytomegalovirus infection after solid organ transplantation. Clin Microbiol Rev (2013) 26:703–27. doi:10.1128/CMR.00015-13
93. Jones S, Rantell K, Stevens K, Colwell B, Ratcliffe JR, Holland P, et al. Outcome at 6 months after admission for pediatric intensive care: a report of a national study of pediatric intensive care units in the United kingdom. Pediatrics (2006) 118:2101–8. doi:10.1542/peds.2006-1455
94. Namachivayam P, Shann F, Shekerdemian L, Taylor A, van Sloten I, Delzoppo C, et al. Three decades of pediatric intensive care: who was admitted, what happened in intensive care, and what happened afterward. Pediatr Crit Care Med (2010) 11:549–55. doi:10.1097/PCC.0b013e3181ce7427
95. Visser IH, Hazelzet JA, Albers MJ, Verlaat CW, Hogenbirk K, van Woensel JB, et al. Mortality prediction models for pediatric intensive care: comparison of overall and subgroup specific performance. Intensive Care Med (2013) 39:942–50. doi:10.1007/s00134-013-2857-4
96. Burns JP, Sellers DE, Meyer EC, Lewis-Newby M, Truog RD. Epidemiology of death in the PICU at five U.S. teaching hospitals*. Crit Care Med (2014) 42:2101–8. doi:10.1097/CCM.0000000000000498
97. Nyirasafari R, Corden MH, Karambizi AC, Kabayiza JC, Makuza JD, Wong R, et al. Predictors of mortality in a paediatric intensive care unit in Kigali, Rwanda. Paediatr Int Child Health (2017) 37:109–15. doi:10.1080/20469047.2016.1250031
98. Khanal A, Sharma A, Basnet S. Current state of pediatric intensive care and high dependency care in Nepal. Pediatr Crit Care Med (2016) 17:1032–40. doi:10.1097/PCC.0000000000000938
99. Abebe T, Girmay M, G/Michael G, Tesfaye M. The epidemiological profile of pediatric patients admitted to the general intensive care unit in an Ethiopian university hospital. Int J Gen Med (2015) 8:63–7. doi:10.2147/IJGM.S76378
100. Ballot DE, Davies VA, Cooper PA, Chirwa T, Argent A, Mer M. Retrospective cross-sectional review of survival rates in critically ill children admitted to a combined paediatric/neonatal intensive care unit in Johannesburg, South Africa, 2013–2015. BMJ Open (2016) 6:e010850. doi:10.1136/bmjopen-2015-010850
101. Proulx F, Fayon M, Farrell CA, Lacroix J, Gauthier M. Epidemiology of sepsis and multiple organ dysfunction syndrome in children. Chest (1996) 109:1033–7. doi:10.1378/chest.109.4.1033
102. Leteurtre S, Duhamel A, Grandbastien B, Lacroix J, Leclerc F. Paediatric logistic organ dysfunction (PELOD) score. Lancet (2006) 367:897. doi:10.1016/S0140-6736(06)68371-2
103. Matics TJ, Sanchez-Pinto LN. Adaptation and validation of a pediatric sequential organ failure assessment score and evaluation of the sepsis-3 definitions in critically ill children. JAMA Pediatr (2017) 171:e172352. doi:10.1001/jamapediatrics.2017.2352
104. Wiens MO, Pawluk S, Kissoon N, Kumbakumba E, Ansermino JM, Singer J, et al. Pediatric post-discharge mortality in resource poor countries: a systematic review. PLoS One (2013) 8:e66698. doi:10.1371/journal.pone.0066698
105. Nwankwor OC, Wiens MO, Kissoon N. Post-discharge morbidity and mortality in children with sepsis. In: Vincent JL, editor. Annual Update in Intensive Care and Emergency Medicine 2017. Cham: Springer (2017). p. 431–44.
106. Moisi JC, Gatakaa H, Berkley JA, Maitland K, Mturi N, Newton CR, et al. Excess child mortality after discharge from hospital in Kilifi, Kenya: a retrospective cohort analysis. Bull World Health Organ (2011) 89:725–32, 732A. doi:10.2471/BLT.11.089235
107. Taylor HA. Inclusion of women, minorities, and children in clinical trials: opinions of research ethics board administrators. J Empir Res Hum Res Ethics (2009) 4:65–73. doi:10.1525/jer.2009.4.2.65
108. Fernandez C. Ethical issues in health research in children. Paediatr Child Health (2008) 13:707–12. doi:10.1093/pch/13.8.707
109. Report B. National Commission for the Protection of Human Subjects of Biomedical and Behavioral Research. Ethical Principles and Guidelines for the Protection of Human Subjects of Research. Washington, DC: U.S. Government Printing Office (1978).
Keywords: cytomegalovirus, pediatric critical care, viremia, reactivation, immunocompetent, outcome, ganciclovir
Citation: Alyazidi R, Murthy S, Slyker JA and Gantt S (2018) The Potential Harm of Cytomegalovirus Infection in Immunocompetent Critically Ill Children. Front. Pediatr. 6:96. doi: 10.3389/fped.2018.00096
Received: 15 December 2017; Accepted: 26 March 2018;
Published: 10 April 2018
Edited by:
Utpal S. Bhalala, Baylor College of Medicine, United StatesReviewed by:
Jhuma Sankar, All India Institute of Medical Sciences, IndiaPhuc Huu Phan, Vietnam National Hospital of Pediatrics, Vietnam
Arun Bansal, Post Graduate Institute of Medical Education and Research, India
Copyright: © 2018 Alyazidi, Murthy, Slyker and Gantt. This is an open-access article distributed under the terms of the Creative Commons Attribution License (CC BY). The use, distribution or reproduction in other forums is permitted, provided the original author(s) and the copyright owner are credited and that the original publication in this journal is cited, in accordance with accepted academic practice. No use, distribution or reproduction is permitted which does not comply with these terms.
*Correspondence: Raidan Alyazidi, cmFpZGFuJiN4MDAwNDA7ZGFsLmNh