- 1COMSATS Institute of Information Technology, Wah Cantonment, Pakistan
- 2Research Unit, Faculty of Allied Health Sciences, University of Lahore, Lahore, Pakistan
- 3University Institute of Physical Therapy, Faculty of Allied Health Sciences, University of Lahore, Lahore, Pakistan
- 4Department of Computer Sciences, Fatima Jinnah Women University, Rawalpindi, Pakistan
- 5Department of Microbiology and Molecular Genetics, University of the Punjab, Lahore, Pakistan
- 6Medical School, University of Verona, Verona, Italy
- 7Faculty of Health Sciences, University of the Punjab, Lahore, Pakistan
Sudden unexpected perinatal collapse is a major trauma for the parents of victims. Sudden infant death syndrome (SIDS) is unexpected and mysterious death of an apparently healthy neonate from birth till 1 year of age without any known causes, even after thorough postmortem investigations. However, the incidence of sudden intrauterine unexplained death syndrome (SIUDS) is seven times higher as compared with SIDS. This observation is approximated 40–80%. Stillbirth is defined as death of a fetus after 20th week of gestation or just before delivery at full term without a known reason. Pakistan has the highest burden of stillbirth in the world. This basis of SIDS, SIUDS, and stillbirths eludes specialists. The purpose of this study is to investigate factors behind failure in control of these unexplained deaths and how research may go ahead with improved prospects. Animal models and physiological data demonstrate that sleep, arousal, and cardiorespiratory malfunctioning are abnormal mechanisms in SIUDS risk factors or in newborn children who subsequently die from SIDS. This review focuses on insights in neuropathology and mechanisms of SIDS and SIUDS in terms of different receptors involved in this major perinatal demise. Several studies conducted in the past decade have confirmed neuropathological and neurochemical anomalies related to serotonin transporter, substance P, acetylcholine α7 nicotine receptors, etc., in sudden unexplained fetal and infant deaths. There is need to focus more on research in this area to unveil the major curtain to neuroprotection by underlying mechanisms leading to such deaths.
Introduction
In the first year of life, the most frequent type of death is “Crib death,” “Cot death” commonly termed as “sudden infant death syndrome” (SIDS). Among every 1,700–2,000 births approximately, one baby gets affected (1). Numerous inherited abnormalities, such as morphological substrates for SIDS–sudden intrauterine unexplained death syndrome (SIUDS), were detected, mainly represented by variations of cardiac conduction system just like accessory pathway, abnormal resorptive degeneration, and hypoplasia/agenesis of the vital brainstem structures. The National Child Health Institute and Human Development has expressed that SIDS is a developmental issue and it takes its root from the fetal development (2). The neuropathological examination plays a significant role in the death investigation procedure. However, just some limited reviews have sufficiently analyzed the neurological substrates, albeit even subtle anomalies of the autonomic nervous system can measure the dysfunctions in the fundamental functions, prompting sudden and unexpected death (3, 4). In-depth examination results, performed at the University of Milan, Lino Rossi Research Center, have added to recognize the area and the nature of these anomalies, normally observed in both SIUDS and SIDS. External risk factors, for example, alcohol, maternal smoking, and drug abuse are identified to be the potential contributors of SIUDS and SIDS (5) while environmental pollution such as insecticides and pesticides has also been reported recently (6).
Sudden Infant Death Syndrome
In a number of these infants, the cerebrum portion that controls the arousal and breathing from sleep is not yet mature enough to work appropriately. Preterm births and intrauterine growth restrictions can cause repressed cognitive development and chronic infarctions. Low immunological development and postnatal sleeping positions are responsible for major respiratory distress. In this section, we have discussed the risk factors for SIDS. Figure 1 is derived from Filiano and Kinney hypothesis (7) and shows risk factors contributing to SIDS.
Neuropathology of SIDS
The major focus on cerebrum anomalies in SIDS victims for a physiological investigation demonstrates cardiopulmonary abnormalities and sleep arousal dysfunction. A typical pathway of these abnormalities at the level of brainstem, where these control functions including ventilation pathways, cardiac rhythm, and pathways for sleep/arousal. Neuropathological basis of SIDS, as proposed to be the major risk factor and needs more neurochemical investigation (7). Research on the neurochemical abnormalities of SIDS victims was started in the 1980s (8). Some neurotransmitters and their functions in a normal infant or fetus are shown in Figure 2.
For instance, acetylcholine (ACh) and serotonin [5-hydroxytryptamines (5-HT)] were found to facilitate breathing (9) while epinephrine (Epi) and norepinephrine (NE) depressed breathing (10). Moreover, it was demonstrated that Epi, NE, and 5-HT were additionally required in the organization of sleep (11). Dopamine was observed to be required in stimulating breath while the peptide neuromodulator substance P (SP) (12, 13), endogenous opioids (14), and derived brain growth neurotrophic factor (BDNF) (15) were observed to be required in the focal control of breath. The neuropathology abnormalities identified in the SIDS brainstem (16) are summarized in Table 1.
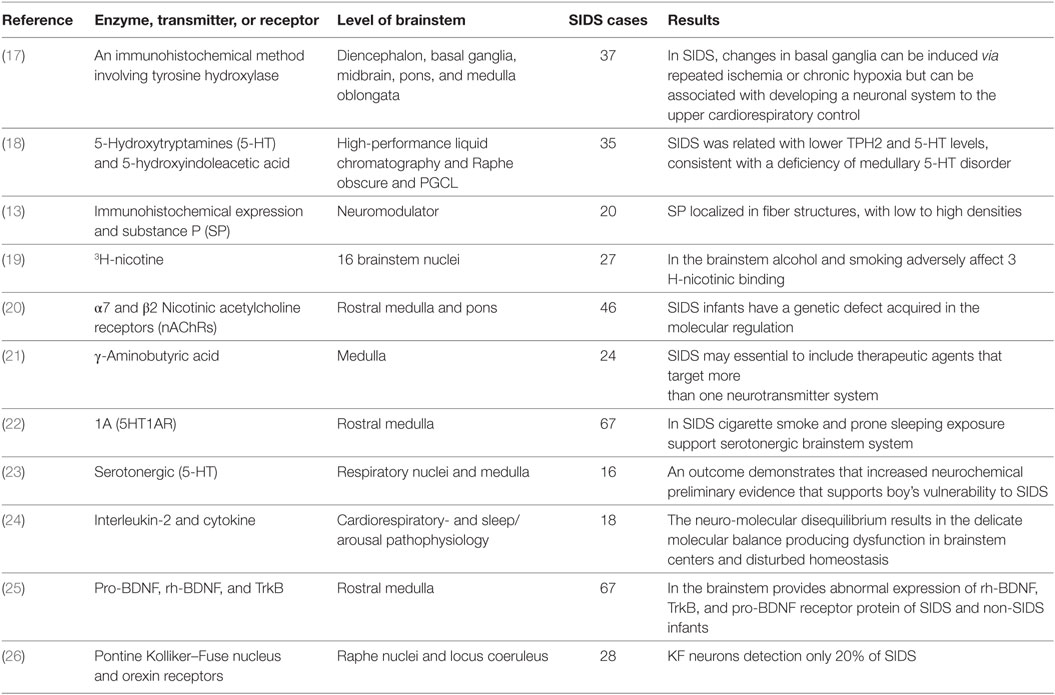
Table 1. Summary for the identification of all neuropathology abnormalities in the sudden infant death syndrome (SIDS) brainstem.
ACh Receptor
Smoking in pregnancy fundamentally increases morbidity and perinatal mortality. It is presently the vital autonomous and modifiable risk factor adding to the sudden newborn child death disorder (SIDS) (27). The more convincing hypothesis for the connection among SIDS and smoking is that nicotine alters the vital breathing patterns and defensive reactions to hypoxia in sleeping (28). A lessened anxiety reaction intensifies hypoxia and apnea (29). The impacts of nicotine are interceded via its activation of very particular nicotinic cholinergic receptors (nAChRs) that are available in the carotid physiques and in the serious brainstem cores, for example, the core of single tract and locus coeruleus (30). At these locales, nAChRs add to the cholinergic adjustment of arousal and breathing. Interference with the nAChRs functions on the presumed basis of negative nicotine reactions (31). Disturbing equilibrium among arousal and ventilatory responses could intensify respiratory failure in sleeping duration. Postnatal exposure to smoke tobacco during early stages is related to increase in the number of sicknesses in repository, pulmonary impaired function, and SIDS events. It is additionally connected through reduced (32) cognitive working and attention deficits in youth. Nicotine, the main neurotoxic segment of tobacco smoke, actuates its activities via binding to nicotinic acetylcholine receptors (nAChR). The immunohistochemical expression of nAChR subunits α2, α3, α4, α5, α7, α9, α1, and α2 in medulla brainstem was analyzed in a piglet model after postnatal nicotine exposure (33). Table 2 describes the ACh receptor abnormalities identified in the SIDS brainstem.
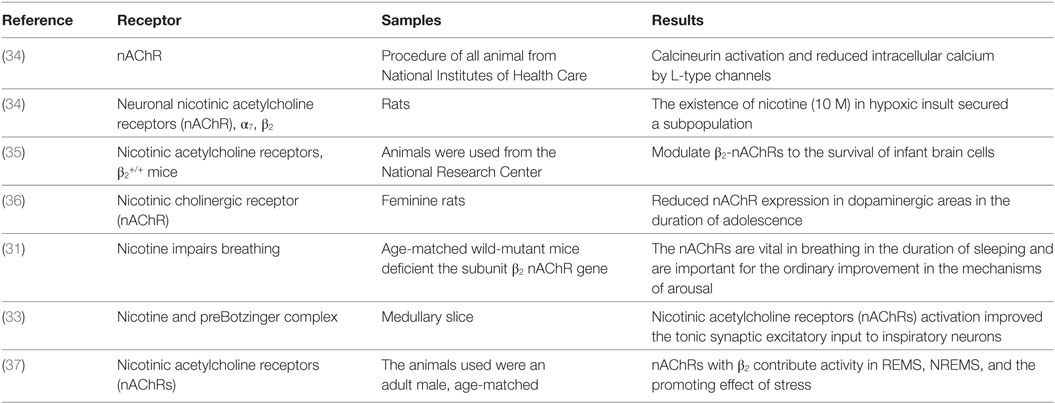
Table 2. Summary for the identification acetylcholine receptor abnormalities in the sudden infant death syndrome brainstem.
Serotonin 5-HT Neurotransmitter
In the brain development, serotonin 5-HT neurotransmitter performs a central role in stress reactivity, mood regulation disorders of psychiatric risk factors and subsequently signaling in 5-HT in the early stage have complicated implications on mental health and behavior over the life span. It takes part in the intercession of cognition, arousal, mood, cerebral blood flow and motor activity. It regulates cardiovascular and cardiorespiratory function, chemosensitivity, thermoregulation, arousal, and pain (38). Figure 3 shows the role of serotonin 5-HT. SIDS victims have been found to have reduced levels of brainstem serotonin (5-HT) and tryptophan hydroxylase 2 (TPH2) but retain producing 5-HT neurons. TPH2 is cerebrum particular enzyme that translates tryptophan into 5-HTP, which is then transformed over into 5-HT via DOPA decarboxylase.
Due to the disturbance in 5-HT medullary levels that outcomes in deadly dysfunction of these dependent sodium-pacemaker neurons regulated via projections of 5-HT from the Raphe and additional Raphe cores (39). We assumed that alteration might be brought about by contrasts in serotonin transporter mRNA expression and 5-HT1A receptor in mind regions included in the control of feelings, memory, and additionally in areas controlling the focal serotonergic tone (40). Table 3 summarizes all the serotonin 5-HT neurotransmitter abnormalities identified in the SIDS brainstem so far.
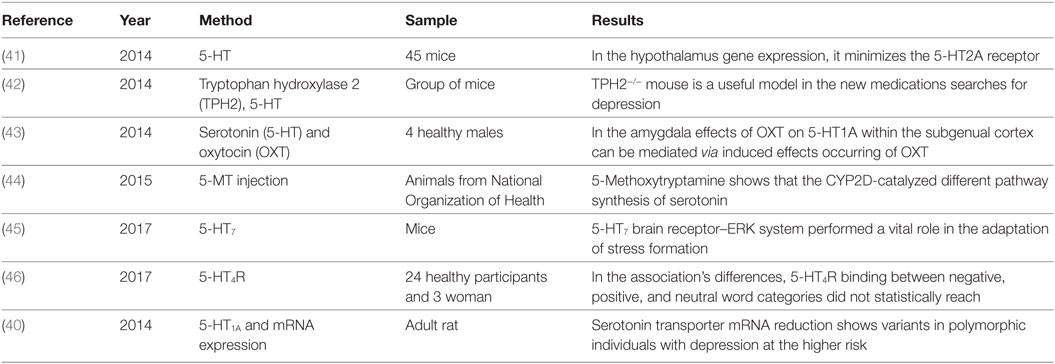
Table 3. Summary for the identification of serotonin 5-hydroxytryptamines (5-HT) neurotransmitter abnormalities in the sudden infant death syndrome.
Low Birthweight and Respiratory Tract Infection
The sudden infant deaths are multifactorial, where low birthweight has been reported major risk factor for SIDS (47). Viral respiratory infections are mainly responsible for the occurrence of sudden death. Mild level of respiratory viral infection was observed by investigators in cases of sudden death infants up to 80% (48).
Sudden Intrauterine Unexplained Death Syndrome
Risk factors for SIUDS are shown in Figure 4. Perinatal brain injuries may occur due to trauma during pregnancy, birth asphyxia, and postnatal accident (48).
Fetal growth restriction (FGR) is a significant difficulty of pregnancy showing a fetus that does not develop to maximum capacity because of pathological compromise. FGR influences 3–9% of pregnancies in high-salary nations and is the main source of perinatal mortality. Placental insufficiency is the key reason for FGR, bringing about chronic fetal hypoxia. This initiates hypoxia of an adaptive fetal reaction of cardiovascular yield redistribution to support indispensable organs, involving the mind and is in result called cerebrum sparing. In spite of this, it is currently apparent that cerebrum sparing does not guarantee normal cerebrum growth in limited development fetuses (49). A brief summary of SIUDS is mentioned in Table 4.
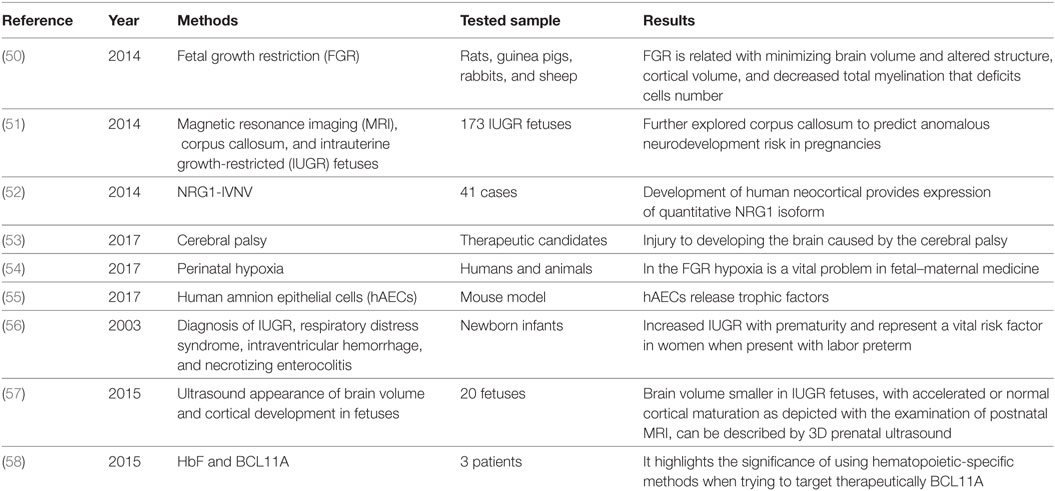
Table 4. Summary for the identification of abnormalities in the sudden intrauterine unexplained death syndrome.
Numerous neurodevelopmental issues of cognitive and motor function have their origins in the antenatal period. Fetal suboptimal growth is probably a key variable underlying altered cerebrum growth. FGR is related with perinatal death, preterm birth and, for survivors, an expanded risk of sensory and motor neurodevelopmental deficits, learning and cognitive impairments, and cerebral palsy. The implementation of the neuroprotective treatments can just happen in light of careful characterization of the abnormalities in brain growth that increases because of FGR, first require the identification of newborn children at most serious risk for the impairment of neurodevelopmental secondary to fetal poor development. Eighty pregnancies end up in termination following detection of an abnormal fetal, neonatal death, or stillbirth, describing no less than eight thousand cases per annum, and there are more than 500 unexplained baby and youth deaths every year. In these circumstances, the posthumous examination is frequently required to decide reason for death, set up implications for relatives, and direct administration of future pregnancies (55).
Fetal growth restriction is generally viewed as a risk for perinatal cerebrum injury with intraventricular hemorrhage (IVH), yet clinical reviews record altered outcomes with elevated, decreased, or unaltered rates of IVH in FGR newborn children contrasted with suitably developed counterparts. Placental insufficiency with anomalous umbilical artery Doppler was connected to the occurrence of IVH. While considering that cerebrum sparing is a characteristic reaction to placental chronic hypoxia, it is not amazing that changes in blood flow to the cerebrum might be both characteristics of the clinical seriousness of FGR, and related with impairments of neurodevelopment. The adaptive reaction of cerebrum sparing requires remodeling of the fetal cerebrum flow that can be diagnosed via Doppler ultrasound as a reduced pulsatility record in the cerebral arteries. At the point when a vast cohort of children was isolated into weekly birth interims, it was found that rates of IVH in FGR were significantly lower versus non-FGR newborn children born at 28 weeks, proposing a defensive impact of development limitation, however, that IVH rates elevated significantly in late-FGR preterm births >34 weeks. This outcome has been confirmed by a recent review demonstrating that IVH was common in late-FGR preterm babies contrasted with suitably developed newborn children. That concern the finding of late preterm births, >34 and <37 weeks, represent most preterm births, and occurrence of preterm births is expanding (59, 60).
Sudden intrauterine unexplained death syndrome is multifactorial and polygenic condition. Although several genetic factors have been reported as cause of SIUDS but defining a specific genetic aberration at this stage is often a challenging issue due to limited phenotype–genotype correlation (61). In addition, genetic anomalies in under developed phenotypes are rarely investigated. Several studies have reported through whole genome sequencing the importance of neurodevelopmental and ion exchange pathway genes (ARHGAP35, BBS7, CASZ1, COL2A1, CRIM1, DHCR7, HADHB, HAPLN3, HSPG2, MYO18B, RYR1, and SRGAP2).
Conclusion
A brainstem abnormality is suggested to be the main underlying etiological factor in SIUDS and SIDS victims. Alterations in certain neurotransmitters such as ACh receptor, serotonin 5-HT neurotransmitter, SP, and brain-derived neurotrophic growth factor (BDNF) are identified in the SIDS and SIUDS, which have vital roles in chemosensation and cardiorespiratory control leading to these sudden deaths. However, further studies are suggested to investigate more into this serious life threatening events.
Author Contributions
All the authors have contributed equally in writing the manuscript.
Conflict of Interest Statement
The authors declare that the research was conducted in the absence of any commercial or financial relationships that could be construed as a potential conflict of interest.
The reviewers BA and ÖÖ and the handling editor declared their shared affiliation.
References
1. Ottaviani G. Defining sudden infant death and sudden intrauterine unexpected death syndromes with regard to anatomo-pathological examination. Front Pediatr (2016) 4:103. doi:10.3389/fped.2016.00103
2. Willinger M, James LS, Catz C. Defining the sudden infant death syndrome (SIDS): deliberations of an expert panel convened by the National Institute of Child Health and Human Development. Pediatr Pathol (1991) 11(5):677–84. doi:10.3109/15513819109065465
3. Rodriguez ML, McMillan K, Crandall LA, Minter ME, Grafe MR, Poduri A, et al. Hippocampal asymmetry and sudden unexpected death in infancy: a case report. Forensic Sci Med Pathol (2012) 8(4):441–6. doi:10.1007/s12024-012-9367-5
4. Sparks DL, Hunsaker JC III. Neuropathology of sudden infant death (syndrome): literature review and evidence of a probable apoptotic degenerative cause. Childs Nerv Syst (2002) 18(11):568–92. doi:10.1007/s00381-002-0629-5
5. Pusiol T, Lavezzi A, Matturri L, Termopoli V, Cappiello A, Piscioli F, et al. Impact assessment of endocrine disruptors on sudden intrauterine and infant death syndromes. Eur J Forensic Sci (2016) 3(2):8/15. doi:10.5455/ejfs.197968
6. Lavezzi AM, Cappiello A, Pusiol T, Corna MF, Termopoli V, Matturri L. Pesticide exposure during pregnancy, like nicotine, affects the brainstem alpha7 nicotinic acetylcholine receptor expression, increasing the risk of sudden unexplained perinatal death. J Neurol Sci (2015) 348(1–2):94–100. doi:10.1016/j.jns.2014.11.014
7. Filiano JJ, Kinney HC. A perspective on neuropathologic findings in victims of the sudden infant death syndrome: the triple-risk model. Biol Neonate (1994) 65(3–4):194–7. doi:10.1159/000244052
8. Myer EC, Morris DL, Adams ML, Brase DA, Dewey WL. Increased cerebrospinal fluid beta-endorphin immunoreactivity in infants with apnea and in siblings of victims of sudden infant death syndrome. J Pediatr (1987) 111(5):660–6. doi:10.1016/S0022-3476(87)80239-1
9. Millhorn DE, Eldridge FL, Waldrop TG. Prolonged stimulation of respiration by a new central neural mechanism. Respir Physiol (1980) 41(1):87–103. doi:10.1016/0034-5687(80)90025-0
10. Machaalani R, Waters KA. Neurochemical abnormalities in the brainstem of the sudden infant death syndrome (SIDS). Paediatr Respir Rev (2014) 15(4):293–300. doi:10.1016/j.prrv.2014.09.008
11. Masserano JM, King C. Effects on sleep of acetylcholine perfusion of the locus coeruleus of cats. Neuropharmacology (1982) 21(11):1163–7. doi:10.1016/0028-3908(82)90174-5
12. Mehboob R. Substance P/neurokinin 1 and trigeminal system: a possible link to the pathogenesis in sudden perinatal deaths. Front Neurol (2017) 8:82. doi:10.3389/fneur.2017.00082
13. Lavezzi AM, Mehboob R, Matturri L. Developmental alterations of the spinal trigeminal nucleus disclosed by substance P immunohistochemistry in fetal and infant sudden unexplained deaths. Neuropathology (2011) 31(4):405–13. doi:10.1111/j.1440-1789.2010.01190.x
14. Moss IR, Denavit-Saubié M, Eldridge FL, Gillis RA, Herkenham M, Lahiri S. Neuromodulators and transmitters in respiratory control. Fed Proc (1986) 45(7):2133–47.
15. Erickson JT, Conover JC, Borday V, Champagnat J, Barbacid M, Yancopoulos G, et al. Mice lacking brain-derived neurotrophic factor exhibit visceral sensory neuron losses distinct from mice lacking NT4 and display a severe developmental deficit in control of breathing. J Neurosci (1996) 16(17):5361–71.
16. Mehboob R, Kabir M, Ahmed N, Ahmad FJ. Towards better understanding of the pathogenesis of neuronal respiratory network in sudden perinatal death. Front Neurol (2017) 8:320. doi:10.3389/fneur.2017.00320
17. Ozawa Y, Obonai T, Itoh M, Aoki Y, Funayama M, Takashima S. Catecholaminergic neurons in the diencephalon and basal ganglia of SIDS. Pediatr Neurol (1999) 21(1):471–5. doi:10.1016/S0887-8994(99)00033-8
18. Duncan JR, Paterson DS, Hoffman JM, Mokler DJ, Borenstein NS, Belliveau RA, et al. Brainstem serotonergic deficiency in sudden infant death syndrome. JAMA (2010) 303(5):430–7. doi:10.1001/jama.2010.45
19. Duncan JR, Randall LL, Belliveau RA, Trachtenberg FL, Randall B, Habbe D, et al. The effect of maternal smoking and drinking during pregnancy upon (3)H-nicotine receptor brainstem binding in infants dying of the sudden infant death syndrome: initial observations in a high risk population. Brain Pathol (2008) 18(1):21–31. doi:10.1111/j.1750-3639.2007.00093.x
20. Machaalani R, Say M, Waters KA. Effects of cigarette smoke exposure on nicotinic acetylcholine receptor subunits alpha7 and beta2 in the sudden infant death syndrome (SIDS) brainstem. Toxicol Appl Pharmacol (2011) 257(3):396–404. doi:10.1016/j.taap.2011.09.023
21. Broadbelt KG, Paterson DS, Belliveau RA, Trachtenberg FL, Haas EA, Stanley C, et al. Decreased GABAA receptor binding in the medullary serotonergic system in the sudden infant death syndrome. J Neuropathol Exp Neurol (2011) 70(9):799–810. doi:10.1097/NEN.0b013e31822c09bc
22. Machaalani R, Say M, Waters KA. Serotoninergic receptor 1A in the sudden infant death syndrome brainstem medulla and associations with clinical risk factors. Acta Neuropathol (2009) 117(3):257–65. doi:10.1007/s00401-008-0468-x
23. Paterson DS, Trachtenberg FL, Thompson EG, Belliveau RA, Beggs AH, Darnall R, et al. Multiple serotonergic brainstem abnormalities in sudden infant death syndrome. JAMA (2006) 296(17):2124–32. doi:10.1001/jama.296.17.2124
24. Kadhim H, Deltenre P, De Prez C, Sébire G. Interleukin-2 as a neuromodulator possibly implicated in the physiopathology of sudden infant death syndrome. Neurosci Lett (2010) 480(2):122–6. doi:10.1016/j.neulet.2010.06.021
25. Tang S, Machaalani R, Waters KA. Expression of brain-derived neurotrophic factor and TrkB receptor in the sudden infant death syndrome brainstem. Respir Physiol Neurobiol (2012) 180(1):25–33. doi:10.1016/j.resp.2011.10.004
26. Lavezzi AM, Ferrero S, Roncati L, Matturri L, Pusiol T. Impaired orexin receptor expression in the Kolliker-Fuse nucleus in sudden infant death syndrome: possible involvement of this nucleus in arousal pathophysiology. Neurol Res (2016) 38(8):706–16. doi:10.1080/01616412.2016.1201632
27. Alm B, Milerad J, Wennergren G, Skjaerven R, Oyen N, Norvenius G, et al. A case-control study of smoking and sudden infant death syndrome in the Scandinavian countries, 1992 to 1995. The Nordic Epidemiological SIDS study. Arch Dis Child (1998) 78(4):329–34. doi:10.1136/adc.78.4.329
28. Lavezzi AM, Ferrero S, Roncati L, Piscioli F, Matturri L, Pusiol T. Nicotinic receptor abnormalities in the cerebellar cortex of sudden unexplained fetal and infant death victims-possible correlation with maternal smoking. ASN Neuro (2017) 9(4):1759091417720582. doi:10.1177/1759091417720582
29. Holgert H, Hökfelt T, Hertzberg T, Lagercrantz H. Functional and developmental studies of the peripheral arterial chemoreceptors in rat: effects of nicotine and possible relation to sudden infant death syndrome. Proc Natl Acad Sci U S A (1995) 92(16):7575–9. doi:10.1073/pnas.92.16.7575
30. Lavezzi AM, Alfonsi G, Matturri L. Pathophysiology of the human locus coeruleus complex in fetal/neonatal sudden unexplained death. Neurol Res (2013) 35(1):44–53. doi:10.1179/1743132812Y.0000000108
31. Cohen G, Han ZY, Grailhe R, Gallego J, Gaultier C, Changeux JP, et al. beta 2 nicotinic acetylcholine receptor subunit modulates protective responses to stress: a receptor basis for sleep-disordered breathing after nicotine exposure. Proc Natl Acad Sci U S A (2002) 99(20):13272–7. doi:10.1073/pnas.192463599
32. Andrew H, Leyan X, Masayoshi N, Heather V, Ayon N, Hiroto K, et al. Increased [18F]ASEM α7-nAChR binding in diffuse traumatic brain injury (TBI) is opposite to that in the focal TBI in rodent models. J Nucl Med (2016) 57(2):347.
33. Shao XM, Feldman JL. Mechanisms underlying regulation of respiratory pattern by nicotine in preBotzinger complex. J Neurophysiol (2001) 85(6):2461–7. doi:10.1152/jn.2001.85.6.2461
34. Hejmadi MV, Dajas-Bailador F, Barns SM, Jones B, Wonnacott S. Neuroprotection by nicotine against hypoxia-induced apoptosis in cortical cultures involves activation of multiple nicotinic acetylcholine receptor subtypes. Mol Cell Neurosci (2003) 24(3):779–86. doi:10.1016/S1044-7431(03)00244-6
35. Mechawar N, Saghatelyan A, Grailhe R, Scoriels L, Gheusi G, Gabellec MM, et al. Nicotinic receptors regulate the survival of newborn neurons in the adult olfactory bulb. Proc Natl Acad Sci U S A (2004) 101(26):9822–6. doi:10.1073/pnas.0403361101
36. Zou D, Luo M, Han Z, Zhan L, Zhu W, Kang S, et al. Activation of alpha-7 nicotinic acetylcholine receptor reduces brain edema in mice with ischemic stroke and bone fracture. Mol Neurobiol (2016) 54(10):8278–86. doi:10.1007/s12035-016-0310-8
37. Léna C, Popa D, Grailhe R, Escourrou P, Changeux JP, Adrien J. Beta2-containing nicotinic receptors contribute to the organization of sleep and regulate putative micro-arousals in mice. J Neurosci (2004) 24(25):5711–8. doi:10.1523/JNEUROSCI.3882-03.2004
38. Brummelte S, Mc Glanaghy E, Bonnin A, Oberlander TF. Developmental changes in serotonin signaling: implications for early brain function, behavior and adaptation. Neuroscience (2017) 342:212–31. doi:10.1016/j.neuroscience.2016.02.037
39. Pena F, Ramirez JM. Endogenous activation of serotonin-2A receptors is required for respiratory rhythm generation in vitro. J Neurosci (2002) 22(24):11055–64.
40. Bravo JA, Dinan TG, Cryan JF. Early-life stress induces persistent alterations in 5-HT1A receptor and serotonin transporter mRNA expression in the adult rat brain. Front Mol Neurosci (2014) 7:24. doi:10.3389/fnmol.2014.00024
41. Popova NK, Kulikov AV, Kondaurova EM, Tsybko AS, Kulikova EA, Krasnov IB, et al. Risk neurogenes for long-term spaceflight: dopamine and serotonin brain system. Mol Neurobiol (2015) 51(3):1443–51. doi:10.1007/s12035-014-8821-7
42. Sampson TR, Mazmanian SK. Control of brain development, function, and behavior by the microbiome. Cell Host Microbe (2015) 17(5):565–76. doi:10.1016/j.chom.2015.04.011
43. Mottolese R, Redouté J, Costes N, Le Bars D, Sirigu A. Switching brain serotonin with oxytocin. Proc Natl Acad Sci U S A (2014) 111(23):8637–42. doi:10.1073/pnas.1319810111
44. Haduch A, Bromek E, Kot M, Kamińska K, Gołembiowska K, Daniel WA. The cytochrome P450 2D-mediated formation of serotonin from 5-methoxytryptamine in the brain in vivo: a microdialysis study. J Neurochem (2015) 133(1):83–92. doi:10.1111/jnc.13031
45. Takeda K. Involvement of brain 5-HT 7 receptors in the formation of stress adaptation in mice. J Explor Res Pharmacol (2017) 2(1):1/23. doi:10.14218/JERP.2016.00035
46. Stenbaek DS, Fisher PM, Ozenne B, Andersen E, Hjordt LV, McMahon B, et al. Brain serotonin 4 receptor binding is inversely associated with verbal memory recall. Brain Behav (2017) 7(4):e00674. doi:10.1002/brb3.674
47. Grether JK, Schulman J. Sudden infant death syndrome and birth weight. J Pediatr (1989) 114(4 Pt 1):561–7. doi:10.1016/S0022-3476(89)80694-8
48. Athanasakis E, Karavasiliadou S, Styliadis I. The factors contributing to the risk of sudden infant death syndrome. Hippokratia (2011) 15(2):127–31.
49. Murray E, Fernandes M, Fazel M, Kennedy SH, Villar J, Stein A. Differential effect of intrauterine growth restriction on childhood neurodevelopment: a systematic review. BJOG (2015) 122(8):1062–72. doi:10.1111/1471-0528.13435
50. Miller SL, Yawno T, Alers NO, Castillo-Melendez M, Supramaniam VG, VanZyl N, et al. Antenatal antioxidant treatment with melatonin to decrease newborn neurodevelopmental deficits and brain injury caused by fetal growth restriction. J Pineal Res (2014) 56(3):283–94. doi:10.1111/jpi.12121
51. Egaña-Ugrinovic G, Sanz-Cortés M, Couve-Pérez C, Figueras F, Gratacós E. Corpus callosum differences assessed by fetal MRI in late-onset intrauterine growth restriction and its association with neurobehavior. Prenat Diagn (2014) 34(9):843–9. doi:10.1002/pd.4381
52. Paterson C, Wang Y, Kleinman JE, Law AJ. Effects of schizophrenia risk variation in the NRG1 gene on NRG1-IV splicing during fetal and early postnatal human neocortical development. Am J Psychiatry (2014) 171(9):979–89. doi:10.1176/appi.ajp.2014.13111518
53. Courtney A, McDonald MCM, Tayla RP, Graham J, Suzanne LM. Umbilical Cord Blood Cells for Perinatal Brain Injury: The Right Cells at the Right Time? Maurício AC, editor. Umbilical Cord Blood Banking for Clinical Application and Regenerative Medicine (2017). Available from: https://www.intechopen.com/books/umbilical-cord-blood-banking-for-clinical-application-and-regenerative-medicine/umbilical-cord-blood-cells-for-perinatal-brain-injury-the-right-cells-at-the-right-time-
54. Paola C, Emilio AH, Bernardo JK. Epigenetic Programming of Cardiovascular Disease by Perinatal Hypoxia and Fetal Growth Restriction, Hypoxia and Human Diseases. Zheng J, editor. (2017). Available from: https://www.intechopen.com/books/hypoxia-and-human-diseases/epigenetic-programming-of-cardiovascular-disease-by-perinatal-hypoxia-and-fetal-growth-restriction
55. Leaw B, Zhu D, Tan J, Muljadi R, Saad MI, Mockler JC, et al. Human amnion epithelial cells rescue cell death via immunomodulation of microglia in a mouse model of perinatal brain injury. Stem Cell Res Ther (2017) 8(1):46. doi:10.1186/s13287-017-0496-3
56. Gilbert WM, Danielsen B. Pregnancy outcomes associated with intrauterine growth restriction. Am J Obstet Gynecol (2003) 188(6):1596–9; discussion 1599–601. doi:10.1067/mob.2003.384
57. Blair EM, Nelson KB. Fetal growth restriction and risk of cerebral palsy in singletons born after at least 35 weeks’ gestation. Am J Obstet Gynecol (2015) 212(4):520.e1–7. doi:10.1016/j.ajog.2014.10.1103
58. Basak A, Hancarova M, Ulirsch JC, Balci TB, Trkova M, Pelisek M, et al. BCL11A deletions result in fetal hemoglobin persistence and neurodevelopmental alterations. J Clin Invest (2015) 125(6):2363–8. doi:10.1172/JCI81163
59. Doğer E, Özdamar Ö, Çakıroğlu Y, Ceylan Y, Çakır Ö, Anık Y, et al. The predictive value of lactate peak detected by the magnetic resonance spectroscopy in the brain of growth-restricted fetuses for adverse perinatal outcomes. J Matern Fetal Neonatal Med (2016) 29(19):3178–82. doi:10.3109/14767058.2015.1118040
60. Lewis AJ, Austin E, Galbally M. Prenatal maternal mental health and fetal growth restriction: a systematic review. J Dev Orig Health Dis (2016) 7(4):416–28. doi:10.1017/S2040174416000076
Keywords: sudden infant death, sudden perinatal death, stillbirth, neuropathology, sudden intrauterine death, neurochemicals
Citation: Muhammad N, Sharif M, Amin J, Mehboob R, Gilani SA, Bibi N, Javed H and Ahmed N (2018) Neurochemical Alterations in Sudden Unexplained Perinatal Deaths—A Review. Front. Pediatr. 6:6. doi: 10.3389/fped.2018.00006
Received: 19 June 2017; Accepted: 09 January 2018;
Published: 25 January 2018
Edited by:
Ilknur Aydin Avci, Ondokuz Mayıs University, TurkeyReviewed by:
Özge Öz, Ondokuz Mayıs University, TurkeySelda Rizalar, Istanbul Medipol University, Turkey
Birsen Altay, Ondokuz Mayıs University, Turkey
Copyright: © 2018 Muhammad, Sharif, Amin, Mehboob, Gilani, Bibi, Javed and Ahmed. This is an open-access article distributed under the terms of the Creative Commons Attribution License (CC BY). The use, distribution or reproduction in other forums is permitted, provided the original author(s) or licensor are credited and that the original publication in this journal is cited, in accordance with accepted academic practice. No use, distribution or reproduction is permitted which does not comply with these terms.
*Correspondence: Naseer Ahmed, ZHIubmFzZWVyOTlAZ21haWwuY29t