- 1Department of Pediatrics, University of Kentucky, Lexington, KY, United States
- 2Department of Biostatistics, College of Public Health, University of Kentucky, Lexington, KY, United States
Background: Brain-derived neurotrophic factor (BDNF) is a type of growth factor that promotes growth and survival of neurons. Fetal exposure to opiates can lead to postnatal withdrawal syndrome, which is referred as neonatal abstinence syndrome (NAS). Preclinical and clinical studies have shown an association between opiates exposure and alteration in BDNF expression in the brain and serum levels in adult. However, to date, there are no data available on the effects of opiate exposure on BDNF levels in infant who are exposed to opiates in utero and whether BDNF level may correlate with the severity of NAS.
Objective: To compare plasma BDNF levels among NAS and non-NAS infants and to determine the correlation of BDNF levels and the severity of NAS.
Methods: This is a prospective cohort study with no intervention involved. Infants ≥35 weeks of gestation were enrolled. BDNF level was measured using enzyme-linked immunosorbent assay technique from blood samples drawn within 48 h of life. The severity of NAS was determined by the length of hospital stay, number of medications required to treat NAS.
Results: 67 infants were enrolled, 34 NAS and 33 non-NAS. Mean gestational age did not differ between the two groups. Mean birth weight of NAS infants was significantly lower than the non-NAS infants (3,070 ± 523 vs. 3,340 ± 459 g, p = 0.028). Mean BDNF level in NAS group was 252.2 ± 91.6 ng/ml, significantly higher than 211.3 ± 66.3 ng/ml in the non-NAS group (p = 0.04). There were no differences in BDNF levels between NAS infants that required one medication vs. more than one medication (254 ± 91 vs. 218 ± 106 ng/ml, p = 0.47). There was no correlation between the BDNF levels and length of hospital stay (p = 0.68) among NAS infants. Overall, there were no significant correlations between BDNF levels and NAS scores except at around 15 h after admission (correlation 0.35, p = 0.045).
Conclusion: Plasma BDNF level was significantly increased in NAS infants during the first 48 h when compared to non-NAS infants. The correlations between plasma BDNF levels and the severity of NAS warrant further study. These results suggest that BDNF may play a neuromodulatory role during withdrawal after in utero opiate exposure.
Introduction
Brain-derived neurotrophic factor (BDNF) is a member of neurotrophin family that is highly expressed in central and peripheral nervous system. The functional maintenance and survival of neurons depends on the availability of BDNF (1, 2). BDNF regulates neuronal survival, promotes neurite outgrowth, and maintains synaptic connectivity in the nervous system (3). BDNF has a neuromodulatory effect on learning and memory (4) and drug addiction (5, 6). BDNF plays significant role in brain synaptic plasticity (7) and locomotor sensitization (8) after opiate withdrawal. Furthermore, several studies demonstrated alterations of serum or plasma BDNF levels in drug abuser (9, 10) and implicated BDNF in the development of addiction (11).
Brain-derived neurotrophic factor is synthesized by neuronal and glial cell populations (12); however, BDNF is also expressed in several non-neuronal tissues such as immune cells and vascular endothelium (13–15). Altered levels and expression of BDNF may lead to abnormal fetal growth and brain development (16). Preclinical study reported increasing peripheral BDNF levels that were positively correlating with the cortical BDNF levels as the animal maturating from early postnatal period to young adults (17). Although there are no normative data for BDNF levels during infancy, study showed that plasma BDNF levels also increased during the early perinatal period in healthy full term infants (18). There was a wide range of peripheral BDNF levels in healthy adult human; however, BDNF levels decreased significantly with increasing age or weight and was affected by gender (19, 20). Expression of BDNF is influenced by many conditions including stress, cigarette smoking (21), alcoholic consumption (22), and depression (23). Decreased serum BDNF levels were found in adults with attention-deficit hyperactive disorder (24) and other neuropsychiatric disorders (23).
In the past decade, neonatal abstinence syndrome (NAS) has been a major health problem. There has been substantial increase in incidence of maternal opiate use and NAS leading to increased health related costs (25). NAS signs include poor sleep, high pitch cry, increased muscle tone, jitteriness, loose stool, poor feeding, etc. Prenatal opiate exposure also results in long-term deleterious consequences including behavioral problems, speech and cognitive deficits, poor social skills, anxiety, aggression, and poor fine and gross motor coordination (26, 27). To date, no reliable biomarker has been identified to predict the severity of NAS and long-term outcomes of children exposed to opiates in utero. However, BDNF may be a good candidate biomarker. Opiate exposure is known to induce apoptosis, downregulate cAMP response element binding expression, and decrease in dendritic branching and spine density, and BDNF protects neurons against these effects (28). Opiate withdrawal can affect serum BDNF level (29) and BDNF expression in the brain (30). Decreased BDNF expression and protein in the brain is associated with behavioral, learning, and memory problems (12, 24, 31). Previous study using a rat model found that opiate exposure compromised memory, increased anxiety levels, and decreased BDNF precursors in the hippocampus (31). Although these studies suggested the likely effects of in utero opiate exposure on the BDNF level and its role in long-term neurobehavioral outcome, to date, there has been no published study on the correlation of NAS/opiate exposure on the levels of BDNF in human infants. Therefore, in the present study, we aimed to assess possible changes in serum BDNF levels in opiates exposed infants compared to unexposed infants and to determine the correlation of BDNF levels with the severity of NAS.
Materials and Methods
This prospective cohort study was performed on 68 infants born at ≥35 weeks of gestation age (GA) and admitted to Kentucky Children’s Hospital Neonatal Intensive Care Unit (NICU) and Newborn Nursery between 2015 and 2016 in Lexington, KY, USA. Inclusion criteria for NAS infants were infants born to mother with history of opiate intake or urine drug screen (UDS) test positive during pregnancy and were admitted in NICU for withdrawal symptoms. These infants were born in University of Kentucky or transferred from outside hospital within 1 week of life. Infants unexposed to opiates by history and/or UDS negative were enrolled in non-NAS group. The exclusion criteria were neonates with major congenital anomalies, infants of mothers <18 years of age, infant transferred from outside hospital after 1 week of life, infant born at GA < 35 weeks, infant who are critically ill, parental refusal to consent, and parents unavailable to consent. The study was approved by the University of Kentucky Institutional Review Board.
Parents of infants who met the inclusion criterion were identified and approached. The informed consent was obtained from the parents. Blood samples, 1.2 ml, were collected from all the subjects at 48 h of life in the non-NAS group and within the first 48 h after the admission to the NICU in the NAS group during the regular blood draw for lab work. Plasma was separated by centrifugation and stored in −80°C till all samples were collected. Measurement of plasma level of BDNF was performed by an enzyme-linked immunosorbent assay (ELISA) method using the human BDNF kit (RayBio Human BDNF ELISA, RayBiotech Inc., GA, USA), according to the manufacturer’s instructions. Plasma samples were diluted 1:100 for BDNF measurement. All BDNF measurement was performed in duplicate. Both NAS and non-NAS were run together in the same plate. The BDNF content was expressed as nanogram (ng) of human recombinant BDNF protein per milliliter of plasma.
We followed the clinical practice guideline for NAS treatment for all NAS infants. NAS scoring using the Finnegan Scale was performed every 3 h after admission, equaled to total of 16 time points in the first 48 h after admission. Opiate replacement therapy with morphine sulfate was started at 0.05 mg/kg, q3 h was started when met the criteria: 3 consecutive scores each ≥9 or 2 consecutive scores ≥13. Morphine dose was increased by 25% of the prior dose if the consecutive scores still met the above criteria and symptoms not captured until reached the maximum dose of 0.12 mg/kg/dose. Second medications were added if the symptoms were still not under controlled with scores met the criteria despite being on the maximum morphine dose based on the history; clonidine for opiate use, phenobarbital for barbiturate use, and diazepam for benzodiazepine use. Morphine weaning was started if the scores remained at desired limits for at least 48 h by 0.02 mg per dose q 24–48 h. The infants were monitored for at least 48 h after morphine was discontinued before discharge. If the infants were also on second medications, they were discharged home on tapering doses with close follow-up at the NICU graduate clinic.
Statistical Analysis
An independent sample t-test was conducted to compare the BDNF levels between NAS group and non-NAS group. A value of p ≤ 0.05 was considered to indicate significance. We also studied the correlation between BDNF levels and the severity of NAS among infants exposed to opiates in utero. The severity of NAS was defined by the length of stay in hospital and whether more than one medicine was needed to treat withdrawal symptoms. Pearson correlation test was performed to study the possible association between BDNF level and length of stay. SAS version 9.4 (SAS Institute, Cary, NC, USA) was used for analyses.
Results
Total of 67 infants were enrolled, 34 in NAS group and 33 in non-NAS group. One infant from NAS group withdrew from study by the parents.
Mean gestational age did not differ between NAS (38.5 ± 1.3 weeks) and non-NAS groups (39 ± 1.2 weeks), p = 0.84 (Table 1). Mean birth weight of the NAS infants was significantly lower than the non-NAS infants, 3,070 ± 523 vs. 3,340 ± 459 g, p = 0.028 (Table 1). Mean BDNF level was significantly higher in NAS infants compared to non-NAS infants (252.2 ± 91.6 vs. 211.3 ± 66.3 ng/ml, difference 41 (2, 80) p = 0.04) (Figure 1).
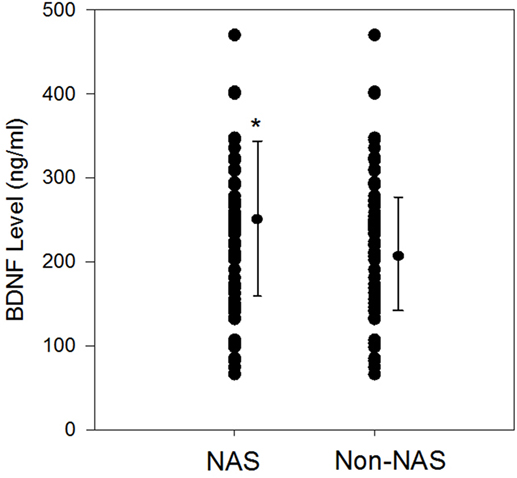
Figure 1. Scatter plots and mean plasma brain-derived neurotrophic factor (BDNF) (±SD) levels (ng/ml) among neonatal abstinence syndrome (NAS) infants during withdrawal phase compared to non-NAS; *p = 0.04.
The area under the receiver operating characteristic curve (ROC) curve is estimated to be 0.641. Based on the minimum Euclidean distance using the ROC curve, the estimated optimal cutoff BDNF level for predicting groups is 240. Using this cutoff value, estimated sensitivity is 61.7%, specificity is 60.6%, positive predictive value is 61.7%, and negative predictive value is 60.6%.
Among the NAS group, 29 infants required one medication whereas 4 infants required two medication for the treatment of NAS, 1 infant did not required any medication for the treatment. There were no differences in BDNF levels between NAS infants who required one medication vs. more than one medication [254 ± 91 vs. 218 ± 106 ng/ml, difference 36 (−64, 137), p = 0.47]. The correlation between the BDNF levels and length of hospital stay among NAS infants was not detected using Pearson Correlation (correlation 0.07, p = 0.68) (Figure 2).
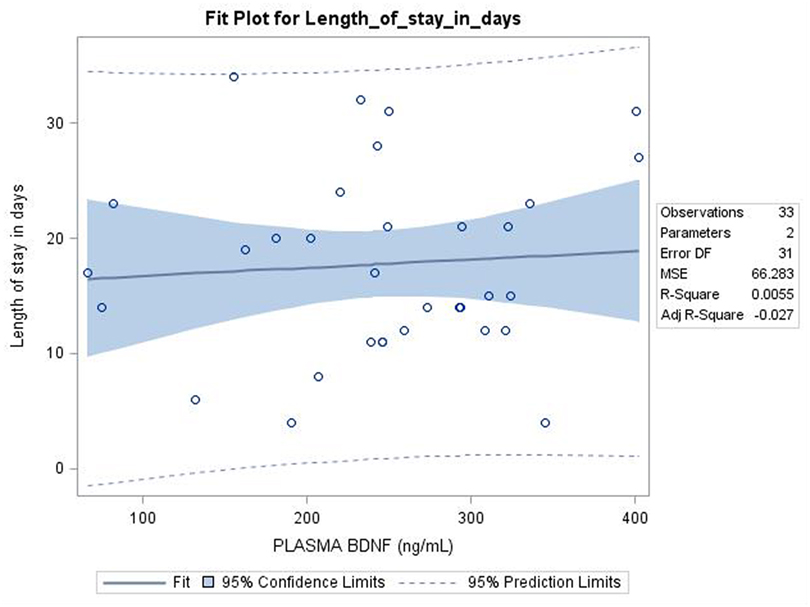
Figure 2. Pearson correlation showed no relation between Plasma Brain derived neurotrophic factor (BDNF) level (ng/ml) and length of stay among neonatal abstinence syndrome infants; p = 0.68.
In the first 48 h after admission for NAS, there were no significant correlations between the BDNF levels and NAS scores at 15 of the 16 time points (Spearman Correlation 0.31 to −0.21, p = 0.07–0.95). However, there was a marginally significant correlation between BDNF levels and NAS scores at time point 5 (around 15 h after admission) (Spearman correlation 0.35, p = 0.045). There were no significant correlations between BDNF levels and maximum scores (p > 0.05), and the means or the total scores in the first 48 h of admission (p = 0.43). The distribution of the NAS scores significantly changed over time based on Friedman’s test (p < 0.0001) as depicted in Figure 3.
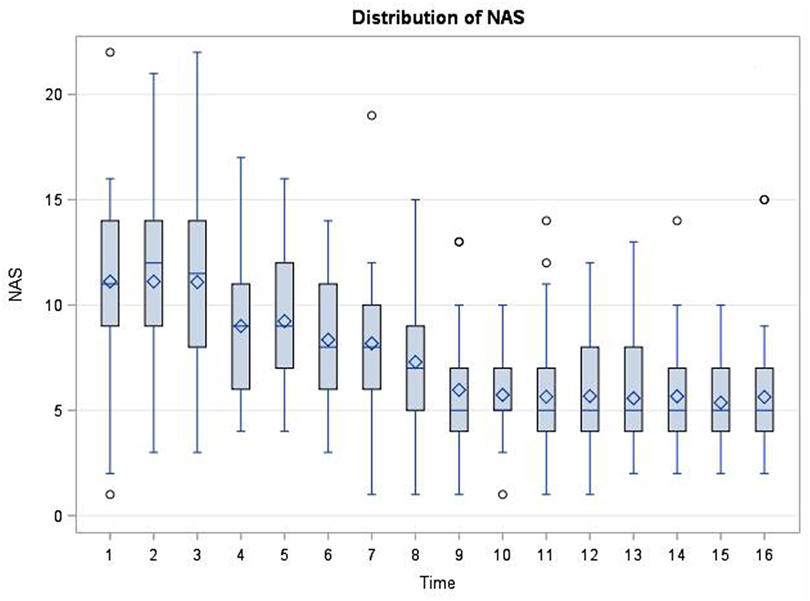
Figure 3. Distribution of neonatal abstinence syndrome (NAS) scores at each time point (every 3 h, total of 16 time points) in the first 48 h after admission for NAS. The scores significantly changed over time based on Friedman Test (p < 0.0001).
Infants in the NAS group were also exposed to other substances, which include cocaine, benzodiazepines, tetrahydrocannabinol (THC), tobacco, amphetamine, methamphetamine, and gabapentin. When BDNF levels among infants with NAS were compared across levels in the given variables, there was no significant difference (Table 2).
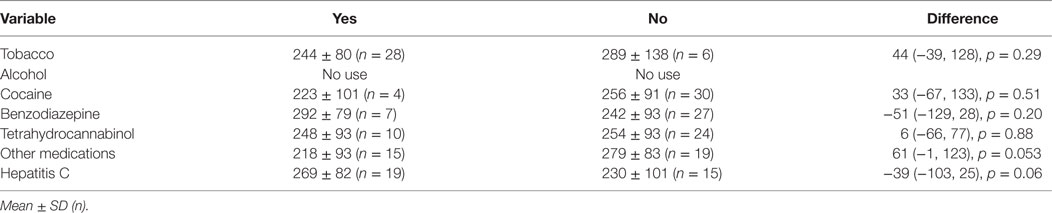
Table 2. Brain-derived neurotrophic factor levels among infants with neonatal abstinence syndrome and comparisons across levels in the given variables.
Discussion
To our knowledge, this is the first study on plasma BDNF level in infants exposed to opiates in utero. Our results showed that plasma BDNF level was significantly increased in NAS infants in early withdrawal phase compared to non-NAS infants. There were no statistically significant correlations between plasma BDNF levels and the severity of NAS based on the length of hospital stay or the number of medications needed for treatment. The BDNF levels did not correlate with NAS scores at any given time point except at around 15 h after admission for NAS, at which point, most of the infants had been started on morphine. The NAS Scores distribution became less dispersed over time after being treated as could be expected. Based on the data, we did not find the cutoff BDNF level that will provide good sensitivity or specificity to predict NAS.
The lower birth weight in NAS infants was consistent with the effect of opiates on the birth weight previously described in the literature (32), but whether this factor may contribute to the higher BDNF levels in this group remains to be further elucidated. The existing data regarding the effects of low birth weight/intrauterine growth restriction on the BDNF levels are still conflicting; at term, the BDNF levels of infants with IUGR were not different from those born appropriate for gestational age (AGA) (33) while the BDNF levels were lower in very preterm infants with severe growth restriction (34).
Our results regarding the increased BDNF levels were in line with previous preclinical and clinical studies. Chronic opiate exposure and acute withdrawal induced upregulation of BDNF genes expression in the nucleus paragigantocellularis in rats (30). Similarly, serum BDNF levels in heroin addicts were higher at the baseline and remained higher than in control subjects after 1 month of heroin cessation (29). Serum BDNF levels correlate well with changes in cortical BDNF levels (17) and measurement of the peripheral BDNF levels may reflect BDNF concentrations in the central nervous system (CNS) and processes in the CNS in opiate-use disorders (35). We, therefore, may postulate that the increase in plasma BDNF level during this early phase of NAS could indicate the upregulation of the BDNF gene expression in the CNS. Together, these support the concept that BDNF might play a critical role in NAS. BDNF plays an important role in the opiate-induced plasticity of noradrenergic locus coeruleus neurons (36), which is implicated in pathogenesis of addiction and withdrawal in adult (37). Additionally, increased BDNF expression may counteract the effect of chronic opiate exposure on the neurons; chronic opiate administration contributed to biochemical and morphological changes in ventral tegmental area (VTA), and some of these changes in VTA were prevented or reversed by the infusion of BDNF into this brain region in rat (38, 39). Taken together, the increased plasma BDNF level in our study is perhaps reflective of the increased BDNF expression in the CNS as a compensatory response to neuronal insult.
Brain-derived neurotrophic factor levels can be influenced by various factors commonly found among substance users such as smoking (21), alcoholic consumption (22), and various neuropsychiatry disorder, which includes depression, anxiety, bipolar disorder, and schizophrenia (23). Recent study by Ghassabian et al. reported that smoking and drinking during pregnancy was associated with lower neonatal BDNF levels (40). In attempt to control for these factors, infants in the control group were not exposed to cigarette smoking during pregnancy. Infants in both groups were not exposed to alcohol during pregnancy. In addition, we compared the BDNF levels among the infants in NAS group with the given variables including maternal smoking, maternal use of other substances including cocaine, benzodiazepine, THC and other neuropsychiatric medications, maternal hepatitis C infection; we found no differences in the BDNF levels in these small subgroups.
Besides the CNS and peripheral nervous system, BDNF is synthesized in other tissues including vascular endothelium and immune cells (13–15). In addition to fetal and neonatal synthesis, maternal passage and placenta synthesis can contribute to the difference in BDNF levels in the newborn (41–43). Thus, it is possible that these factors also contributed to the increased BDNF level in our study.
Our study had certain limitations including maternal polysubstance use as mentioned, inconsistent timing of the blood draw depending on when the infants were admitted for NAS management, and some infants received initiation of treatment with morphine before blood draws; these factors could affect the BDNF levels. Larger sample size is warranted for the study in the future to be able to control for these confounders. Our plasma BDNF levels had a wide distribution consistent with the literature (18); however, the means were higher than previously reported by others. This could be due to different assays used and perhaps the samples had clotted, therefore, became serum samples, which reported to give much higher BDNF levels (44).
In summary, we observed that serum BDNF levels were increased in NAS infants during early withdrawal phase when compared to non-NAS infants. These results suggested that increased serum BDNF levels might be associated with the pathophysiology of opiate exposure and withdrawal in the neonates. Serial measurement of plasma BDNF levels during the withdrawal phase in infants with NAS and during developmental follow-up of these infants would be vital to further understand the role of BDNF in NAS and the outcomes of these infants.
Ethics Statement
This study was carried out in accordance with the recommendations of University of Kentucky, Institutional Review Board with written informed consent from all subjects. All subjects gave written informed consent in accordance with the Declaration of Helsinki. The protocol was approved by the Institutional Review Board.
Author Contributions
LS: designed, conducted the study, analyzed data, and wrote the manuscript. HH: performed the ELISA and helped with analysis of the data. AP: helped collecting data, edited the manuscript. PW: biostatistician, analyzed the data. HB: helped with the design and analysis of the study, edited the manuscript. JB and PG: helped in the design of the study and lab. TS: main adviser for LS, helped with the design, conduction and analysis of the study, and wrote and edited the manuscript.
Conflict of Interest Statement
The authors declare that the research was conducted in the absence of any commercial or financial relationships that could be construed as a potential conflict of interest.
Acknowledgments
We are thankful to the NICU faculty, nurses, research staff, and families.
Funding
This study was supported by Children’s Miracle Network grant, grant number 1012137390 awarded to TS.
Abbreviations
BDNF, brain-derived neurotrophic factor; NAS, neonatal abstinence syndrome; CREB, cAMP response element binding; ADHD, attention-deficit hyperactive disorder; PGi, nucleus paragigantocellularis; LC, locus coeruleus; VTA, ventral tegmental area; THC, tetrahydrocannabinol.
References
1. Leibrock J, Lottspeich F, Hohn A, Hofer M, Hengerer B, Masiakowski P, et al. Molecular cloning and expression of brain-derived neurotrophic factor. Nature (1989) 341:149–52. doi:10.1038/341149a0
2. Hohn A, Leibrock J, Bailey K, Barde YA. Identification and characterization of a novel member of the nerve growth factor/brain-derived neurotrophic factor family. Nature (1990) 344:339–41. doi:10.1038/344339a0
3. Twiss JL, Chang JH, Schanen NC. Pathophysiological mechanisms for actions of the neurotrophins. Brain Pathol (2006) 16:320–32. doi:10.1111/j.1750-3639.2006.00039.x
4. Altar CA, Boylan CB, Jackson C, Hershenson S, Miller J, Wiegand SJ, et al. Brain-derived neurotrophic factor augments rotational behavior and nigrostriatal dopamine turnover in vivo. Proc Natl Acad Sci U S A (1992) 89:11347–51. doi:10.1073/pnas.89.23.11347
5. Grimm JW, Lu L, Hayashi T, Hope BT, Su TP, Shaham Y. Time-dependent increases in brain-derived neurotrophic factor protein levels within the mesolimbic dopamine system after withdrawal from cocaine: implications for incubation of cocaine craving. J Neurosci (2003) 23:742–7.
6. McGough NN, He DY, Logrip ML, Jeanblanc J, Phamluong K, Luong K, et al. RACK1 and brain-derived neurotrophic factor: a homeostatic pathway that regulates alcohol addiction. J Neurosci (2004) 24:10542–52. doi:10.1523/JNEUROSCI.3714-04.2004
7. Li Y, Jia YC, Cui K, Li N, Zheng ZY, Wang YZ, et al. Essential role of TRPC channels in the guidance of nerve growth cones by brain-derived neurotrophic factor. Nature (2005) 434:894–8. doi:10.1038/nature03477
8. Liang J, Zheng X, Chen J, Li Y, Xing X, Bai Y, et al. Roles of BDNF, dopamine D(3) receptors, and their interactions in the expression of morphine-induced context-specific locomotor sensitization. Eur Neuropsychopharmacol (2011) 21:825–34. doi:10.1016/j.euroneuro.2010.12.006
9. Corominas-Roso M, Roncero C, Eiroa-Orosa FJ, Gonzalvo B, Grau-Lopez L, Ribases M, et al. Brain-derived neurotrophic factor serum levels in cocaine-dependent patients during early abstinence. Eur Neuropsychopharmacol (2013) 23:1078–84. doi:10.1016/j.euroneuro.2012.08.016
10. Kim DJ, Roh S, Kim Y, Yoon SJ, Lee HK, Han CS, et al. High concentrations of plasma brain-derived neurotrophic factor in methamphetamine users. Neurosci Lett (2005) 388:112–5. doi:10.1016/j.neulet.2005.06.042
11. Ghitza UE, Zhai H, Wu P, Airavaara M, Shaham Y, Lu L. Role of BDNF and GDNF in drug reward and relapse: a review. Neurosci Biobehav Rev (2010) 35:157–71. doi:10.1016/j.neubiorev.2009.11.009
12. Binder DK, Scharfman HE. Brain-derived neurotrophic factor. Growth Factors (2004) 22:123–31. doi:10.1080/08977190410001723308
13. Karege F, Bondolfi G, Gervasoni N, Schwald M, Aubry JM, Bertschy G. Low brain-derived neurotrophic factor (BDNF) levels in serum of depressed patients probably results from lowered platelet BDNF release unrelated to platelet reactivity. Biol Psychiatry (2005) 57:1068–72. doi:10.1016/j.biopsych.2005.01.008
14. Nakahashi T, Fujimura H, Altar CA, Li J, Kambayashi J, Tandon NN, et al. Vascular endothelial cells synthesize and secrete brain-derived neurotrophic factor. FEBS Lett (2000) 470:113–7. doi:10.1016/S0014-5793(00)01302-8
15. Noga O, Englmann C, Hanf G, Grutzkau A, Seybold J, Kunkel G. The production, storage and release of the neurotrophins nerve growth factor, brain-derived neurotrophic factor and neurotrophin-3 by human peripheral eosinophils in allergics and non-allergics. Clin Exp Allergy (2003) 33:649–54. doi:10.1046/j.1365-2222.2003.01586.x
16. Dhobale M. Neurotrophins: role in adverse pregnancy outcome. Int J Dev Neurosci (2014) 37:8–14. doi:10.1016/j.ijdevneu.2014.06.005
17. Karege F, Schwald M, Cisse M. Postnatal developmental profile of brain-derived neurotrophic factor in rat brain and platelets. Neurosci Lett (2002) 328:261–4. doi:10.1016/S0304-3940(02)00529-3
18. Nikolaou KE, Malamitsi-Puchner A, Boutsikou T, Economou E, Boutsikou M, Puchner KP, et al. The varying patterns of neurotrophin changes in the perinatal period. Ann N Y Acad Sci (2006) 1092:426–33. doi:10.1196/annals.1365.041
19. Lommatzsch M, Zingler D, Schuhbaeck K, Schloetcke K, Zingler C, Schuff-Werner P, et al. The impact of age, weight and gender on BDNF levels in human platelets and plasma. Neurobiol Aging (2005) 26:115–23. doi:10.1016/j.neurobiolaging.2004.03.002
20. Katoh-Semba R, Wakako R, Komori T, Shigemi H, Miyazaki N, Ito H, et al. Age-related changes in BDNF protein levels in human serum: differences between autism cases and normal controls. Int J Dev Neurosci (2007) 25:367–72. doi:10.1016/j.ijdevneu.2007.07.002
21. Bhang SY, Choi SW, Ahn JH. Changes in plasma brain-derived neurotrophic factor levels in smokers after smoking cessation. Neurosci Lett (2010) 468:7–11. doi:10.1016/j.neulet.2009.10.046
22. Joe KH, Kim YK, Kim TS, Roh SW, Choi SW, Kim YB, et al. Decreased plasma brain-derived neurotrophic factor levels in patients with alcohol dependence. Alcohol Clin Exp Res (2007) 31:1833–8. doi:10.1111/j.1530-0277.2007.00507.x
23. Autry AE, Monteggia LM. Brain-derived neurotrophic factor and neuropsychiatric disorders. Pharmacol Rev (2012) 64:238–58. doi:10.1124/pr.111.005108
24. Corominas-Roso M, Ramos-Quiroga JA, Ribases M, Sanchez-Mora C, Palomar G, Valero S, et al. Decreased serum levels of brain-derived neurotrophic factor in adults with attention-deficit hyperactivity disorder. Int J Neuropsychopharmacol (2013) 16:1267–75. doi:10.1017/S1461145712001629
25. Patrick SW, Schumacher RE, Benneyworth BD, Krans EE, Mcallister JM, Davis MM. Neonatal abstinence syndrome and associated health care expenditures: United States, 2000–2009. JAMA (2012) 307:1934–40. doi:10.1001/jama.2012.3951
26. Bauman PS, Levine SA. The development of children of drug addicts. Int J Addict (1986) 21:849–63. doi:10.3109/10826088609027399
27. de Cubas MM, Field T. Children of methadone-dependent women: developmental outcomes. Am J Orthopsychiatry (1993) 63:266–76. doi:10.1037/h0079429
28. Pilakka-Kanthikeel S, Atluri VS, Sagar V, Saxena SK, Nair M. Targeted brain derived neurotropic factors (BDNF) delivery across the blood-brain barrier for neuro-protection using magnetic nano carriers: an in-vitro study. PLoS One (2013) 8:e62241. doi:10.1371/journal.pone.0062241
29. Zhang J, Zhang X, Su H, Tao J, Xie Y, Han B, et al. Increased serum brain-derived neurotrophic factor levels during opiate withdrawal. Neurosci Lett (2014) 571:61–5. doi:10.1016/j.neulet.2014.04.048
30. Hatami H, Oryan S, Semnanian S, Kazemi B, Bandepour M, Ahmadiani A. Alterations of BDNF and NT-3 genes expression in the nucleus paragigantocellularis during morphine dependency and withdrawal. Neuropeptides (2007) 41:321–8. doi:10.1016/j.npep.2007.04.007
31. Schrott LM, Franklin L, Serrano PA. Prenatal opiate exposure impairs radial arm maze performance and reduces levels of BDNF precursor following training. Brain Res (2008) 1198:132–40. doi:10.1016/j.brainres.2008.01.020
32. Oats JN, Beischer NA, Breheny JE, Pepperell RJ. The outcome of pregnancies complicated by narcotic drug addiction. Aust N Z J Obstet Gynaecol (1984) 24:14–6. doi:10.1111/j.1479-828X.1984.tb03314.x
33. Malamitsi-Puchner A, Nikolaou KE, Economou E, Boutsikou M, Boutsikou T, Kyriakakou M, et al. Intrauterine growth restriction and circulating neurotrophin levels at term. Early Hum Dev (2007) 83:465–9. doi:10.1016/j.earlhumdev.2006.09.001
34. Leviton A, Allred EN, Yamamoto H, Fichorova RN, Kuban K, O’shea TM, et al. Antecedents and correlates of blood concentrations of neurotrophic growth factors in very preterm newborns. Cytokine (2017) 94:21–8. doi:10.1016/j.cyto.2017.03.012
35. Palma-Alvarez RF, Ros-Cucurull E, Amaro-Hosey K, Rodriguez-Cintas L, Grau-Lopez L, Corominas-Roso M, et al. Peripheral levels of BDNF and opiate-use disorder: literature review and update. Rev Neurosci (2017) 28:499–508. doi:10.1515/revneuro-2016-0078
36. Akbarian S, Rios M, Liu RJ, Gold SJ, Fong HF, Zeiler S, et al. Brain-derived neurotrophic factor is essential for opiate-induced plasticity of noradrenergic neurons. J Neurosci (2002) 22:4153–62.
37. Nestler EJ, Aghajanian GK. Molecular and cellular basis of addiction. Science (1997) 278:58–63. doi:10.1126/science.278.5335.58
38. Berhow MT, Russell DS, Terwilliger RZ, Beitner-Johnson D, Self DW, Lindsay RM, et al. Influence of neurotrophic factors on morphine- and cocaine-induced biochemical changes in the mesolimbic dopamine system. Neuroscience (1995) 68:969–79. doi:10.1016/0306-4522(95)00207-Y
39. Sklair-Tavron L, Shi WX, Lane SB, Harris HW, Bunney BS, Nestler EJ. Chronic morphine induces visible changes in the morphology of mesolimbic dopamine neurons. Proc Natl Acad Sci U S A (1996) 93:11202–7. doi:10.1073/pnas.93.20.11202
40. Ghassabian A, Sundaram R, Chahal N, Mclain AC, Bell E, Lawrence DA, et al. Determinants of neonatal brain-derived neurotrophic factor and association with child development. Dev Psychopathol (2017) 29:1499–511. doi:10.1017/S0954579417000414
41. Chouthai NS, Sampers J, Desai N, Smith GM. Changes in neurotrophin levels in umbilical cord blood from infants with different gestational ages and clinical conditions. Pediatr Res (2003) 53:965–9. doi:10.1203/01.PDR.0000061588.39652.26
42. Prusa AR, Marton E, Rosner M, Bettelheim D, Lubec G, Pollack A, et al. Neurogenic cells in human amniotic fluid. Am J Obstet Gynecol (2004) 191:309–14. doi:10.1016/j.ajog.2003.12.014
43. Fan CG, Zhang QJ, Tang FW, Han ZB, Wang GS, Han ZC. Human umbilical cord blood cells express neurotrophic factors. Neurosci Lett (2005) 380:322–5. doi:10.1016/j.neulet.2005.01.070
Keywords: intrauterine opiate exposure, effect of opiate exposure, neonatal abstinence syndrome, neurobehavioral outcome, brain derived neurotrophic factor
Citation: Subedi L, Huang H, Pant A, Westgate PM, Bada HS, Bauer JA, Giannone PJ and Sithisarn T (2017) Plasma Brain-Derived Neurotrophic Factor Levels in Newborn Infants with Neonatal Abstinence Syndrome. Front. Pediatr. 5:238. doi: 10.3389/fped.2017.00238
Received: 31 July 2017; Accepted: 20 October 2017;
Published: 03 November 2017
Edited by:
Vineet Bhandari, Drexel University, United StatesReviewed by:
Subrata Sarkar, University of Michigan Health System, United StatesKarel Allegaert, University Hospitals Leuven, Belgium
Copyright: © 2017 Subedi, Huang, Pant, Westgate, Bada, Bauer, Giannone and Sithisarn. This is an open-access article distributed under the terms of the Creative Commons Attribution License (CC BY). The use, distribution or reproduction in other forums is permitted, provided the original author(s) or licensor are credited and that the original publication in this journal is cited, in accordance with accepted academic practice. No use, distribution or reproduction is permitted which does not comply with these terms.
*Correspondence: Thitinart Sithisarn, dHNpdGgyQHVreS5lZHU=