- 1Department of Pediatrics, Division of Critical Care Medicine, Mattel Children’s Hospital at UCLA, Los Angeles, CA, USA
- 2Departments of Medicine, Division of Pulmonary, Critical Care, and Sleep Medicine, Memphis Veterans Affairs Medical Center, Memphis, TN, USA
Based on molecular mechanisms and physiologic data, a strong association has been established between dysregulated systemic inflammation and progression of acute respiratory distress syndrome (ARDS). In ARDS patients, glucocorticoid receptor-mediated downregulation of systemic inflammation is essential to restore homeostasis, decrease morbidity and improve survival and can be significantly enhanced with prolonged low-to-moderate dose glucocorticoid treatment. A large body of evidence supports a strong association between prolonged glucocorticoid treatment-induced downregulation of the inflammatory response and improvement in pulmonary and extrapulmonary physiology. The balance of the available data from eight controlled trials (n = 622) provides consistent strong level of evidence for improving patient-centered outcomes and hospital survival. The sizable increase in mechanical ventilation-free days (weighted mean difference, 6.48 days; CI 95% 2.57–10.38, p < 0.0001) and intensive care unit-free days (weighted mean difference, 7.7 days; 95% CI, 3.13–12.20, p < 0.0001) by day 28 is superior to any investigated intervention in ARDS. For treatment initiated before day 14 of ARDS, the increased in hospital survival (70 vs. 52%, OR 2.41, CI 95% 1.50–3.87, p = 0.0003) translates into a number needed to treat to save one life of 5.5. Importantly, prolonged glucocorticoid treatment is not associated with increased risk for nosocomial infections (22 vs. 27%, OR 0.61, CI 95% 0.35–1.04, p = 0.07). Treatment decisions involve a tradeoff between benefits and risks, as well as costs. This low-cost, highly effective therapy is familiar to every physician and has a low risk profile when secondary prevention measures are implemented.
Physiologic Considerations for Prolonged Glucocorticoid Therapy in ARDS
Acute respiratory distress syndrome (ARDS) is a secondary disease that follows – usually within 6–48 h – a primary disease of multifactorial etiology (most frequently pneumonia and extrapulmonary sepsis) associated with severe systemic inflammation. Inflammatory mediators released into the systemic circulation (systemic inflammation) from the site of infection reach the broad pulmonary capillary surface producing severe and diffuse inflammatory exudate of the pulmonary lobules resulting in hypoxemic respiratory failure (1, 2). In sepsis and ARDS, systemic inflammation is activated by the nuclear factor-κB (NF-κB) signaling system and downregulated by the activated glucocorticoid receptor-α (GRα) (1) (Figure 1). In these patients, inadequate (endogenous glucocorticoid activated) GRα-mediated downregulation of pro-inflammatory NF-κB in circulating and tissue cells leads to higher initial levels and persistent elevation over time in plasma and bronchoalveolar lavage (BAL) markers of inflammation, hemostasis, and tissue repair (1).
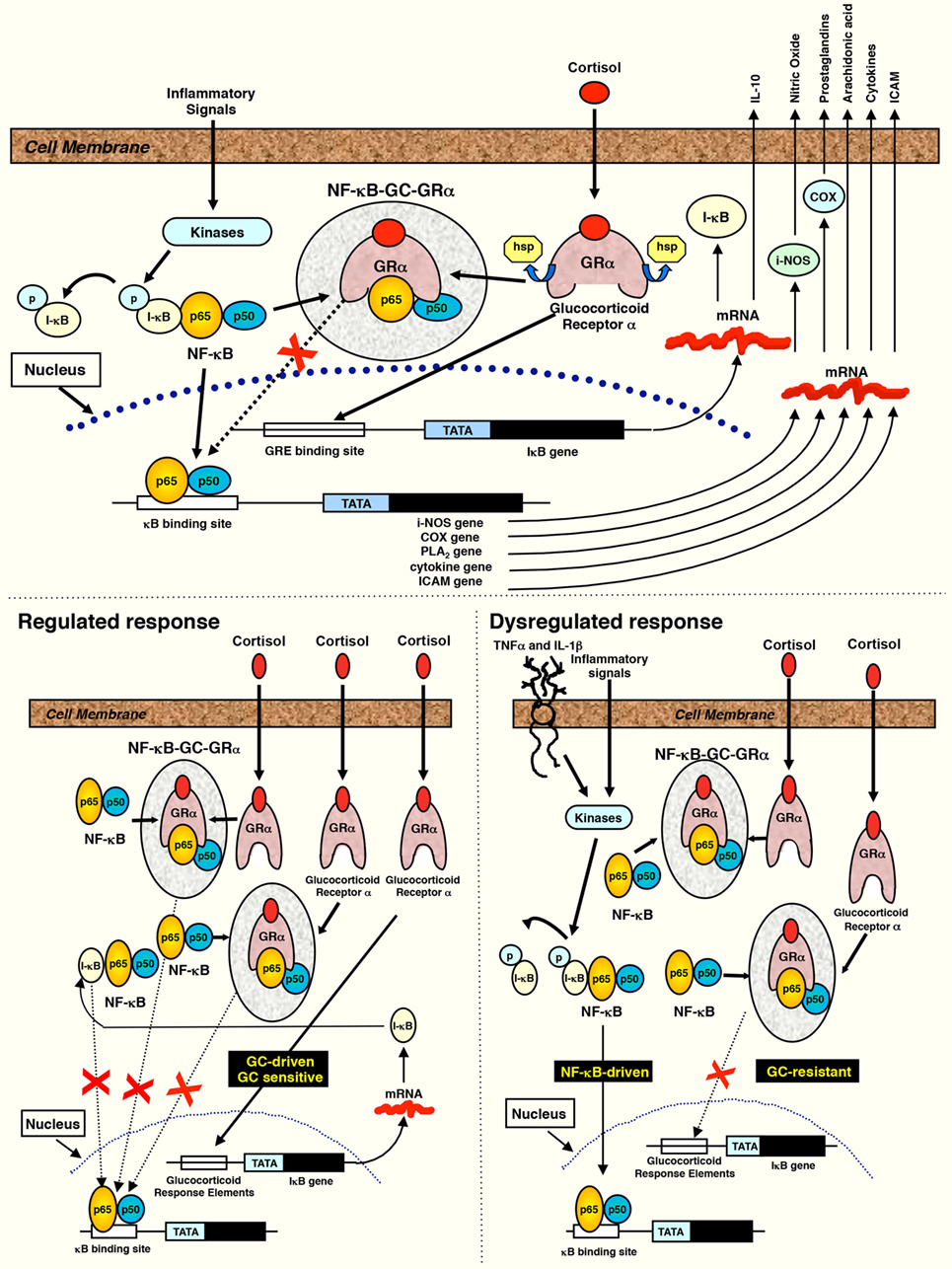
Figure 1. Interaction between NF-κB and the activated glucocorticoid receptor. Reproduced from Ref. (3) with permission from S. Karger AG, Medical and Scientific Publishers (Allschwilerstrasse 10, 4009 Basel, Switzerland). When cells are stimulated by inflammatory signals, specific kinases phosphorylate the inhibitory protein IκB and cause its rapid degradation. The activated form of NF-κB then moves to the nucleus initiating the transcription of mRNA of inflammatory cytokines, chemokines, cell adhesion molecules, and inflammation-associated enzymes (cyclooxygenase, phospholipase A2, and inducible nitric oxide). Cortisol or exogenous glucocorticoids freely cross into the cytoplasm and bind to their specific glucocorticoid receptors (GRα) to form the activated receptor (GC-GRα). GC-GRα complexes may influence NF-κB activity in five major ways: (1) physically interacting with the p65 subunit with formation of an inactive (GC-GRα/NF-κB) complex (4, 5), (2) inducing the synthesis of the inhibitory protein IκBα via interaction with glucocorticoid-responsive element DNA in the promoter of the IκB gene (5–7), (3) blocking degradation of IκBα via enhanced synthesis of IL-10 (8–10), (4) impairing TNF-α-induced degradation of IκBα (11, 12), and (5) competing for limited amounts of GR coactivators, such as CREB-binding protein (CBP) and steroid receptor coactivator-1 (SRC-1) (13). IκBα, in addition to holding NF-κB in an inactive cytoplasmic state (5–7), also translocates into the nucleus, where it binds activated NF-κB complexes to induce their export to the cytoplasm (4). GC-GRα may also decrease the stability of mRNA of several inflammatory cytokines and other molecules (14). Products of the genes that are stimulated by NF-κB activate this transcription factor. Thus, both TNF-α and IL-1β activate and are activated by NF-κB, by forming a positive regulatory loop that amplifies and perpetuates inflammation (15). Regulated response: GC-GRα activation sufficient to maintain NF-κB levels in homeostasis and achieve a reduction in transcription of inflammatory mediators over time is shown. In ARDS improvers, both GC-GRα binding to NF-κB and nuclear GC-GRα binding increased significantly over time, indicating an excess activation of GC-GRα compared to NF-κB (GC-GRα-driven response). Dysregulated response: an excess of NF-κB activation is shown, leading to protracted transcription of inflammatory mediators over time. In ARDS non-improvers, GC-GRα binding to NF-κB was modestly increased while nuclear NF-κB binding increased substantially over time and nuclear GC-GRα and cytoplasmic IκBα levels declined (NF-κB-driven response).
In ARDS, “resolution of inflammation and restoration of tissue homeostasis is an active, continuous, and coordinated process (switch from production of pro-inflammatory toward pro-resolving molecules)” (16) – characterized by non-phlogistic elimination of granulocytes by apoptosis, restoration of the alveolar-capillary membrane’s integrity, resorption of edema, increased surfactant production, decreased clotting, and fibroproliferation with resolution of intra-alveolar and interstitial granulation tissue (1, 2) – that can be accelerated by prolonged glucocorticoid treatment (17). There is a strong scientific foundation in support of prolonged methylprednisolone treatment in ARDS, with evidence showing that this treatment is directed at the core pathogenic mechanism of ARDS and as a result positively affects all “layers” – biology, histology, and physiology – of the disease process (18).
Importantly, in ARDS the inadequate GRα-mediated downregulation of pro-inflammatory transcription factor NF-κB in circulating and tissue cells leads to persistent elevation over time (>4 weeks) in plasma and BAL markers of inflammation, hemostasis, and tissue repair (maladaptive repair) (1). In experimental sepsis, inhibition of endogenous glucocorticoid synthesis aggravates acute lung injury, while glucocorticoid treatment was associated with protection (19). Experimental ARDS is associated with a significant reduction in lung tissue GRα expression (20–22) and increase in GRβ mRNA (21), leading to decreased GRα nuclear translocation (21). In these experiments, low-dose glucocorticoid treatment – contrary to placebo – restored GRα number and function, leading to resolution of pulmonary inflammation (22, 23). Moreover, alveolar macrophages extracted from patients with established ARDS have decreased 11β-HSD oxo-reductase activity with decreased conversion of cortisone to cortisol (24).
In an ex vivo study, prolonged methylprednisolone treatment – contrary to placebo – was associated with upregulation in GRα number and nuclear translocation with reduction in NF-κB–DNA binding and transcription of inflammatory cytokines (25) (Figure 2). ARDS patients randomized to prolonged methylprednisolone treatment, contrary to placebo, demonstrated a sustained reduction in plasma and/or BAL levels of tumor necrosis factor-α (TNF-α), interleukin (IL)-1β, IL-6 (1), IL-8, soluble intercellular adhesion molecule-1 (1), procollagen aminoterminal propeptide type I and III (1), indices of alveolar-capillary membrane permeability (BAL albumin, total protein, and percentage neutrophils) (26), and an increase in IL-10 (1), protein C (27), and surfactant (28).
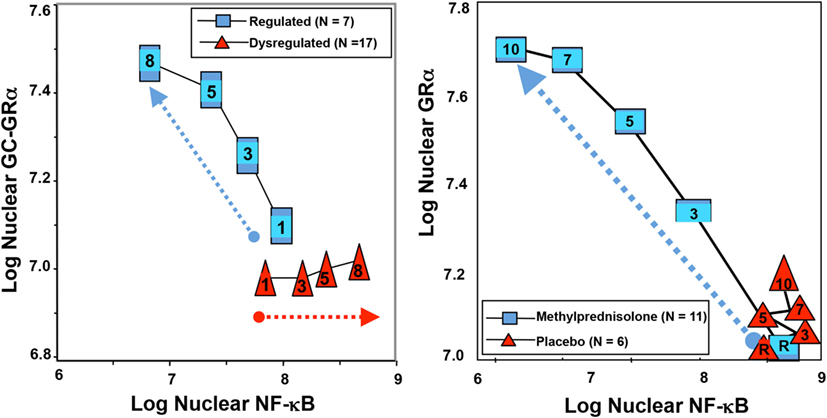
Figure 2. Longitudinal relation on natural logarithmic scales between mean levels of nuclear NF-κB and nuclear GRα: resolving vs. unresolving ARDS (left) and after randomization to methylprednisolone vs. placebo (right). Data shown are from Ref. (3, 25). Left: plasma samples from patients with sustained elevation in cytokine levels over time (triangles) elicited only a modest longitudinal increase in GC-GRα-mediated activity (P = 0.04) and a progressive significant (P = 0.0001) increase in NF-κB nuclear binding over time (dysregulated, NF-κB-driven response). In contrast, in patients with regulated inflammation (squares), an inverse relation was observed between these two transcription factors, with the longitudinal direction of the interaction shifting to the left (decreased NF-κB) and upward (increased GC-GRα). The first interaction is defined as NF-κB-driven response (progressive increase in NF-κB–DNA binding and transcription of TNF-α and IL-1β) and the second interaction as GRα-driven response (progressive increase in GRα-DNA binding and transcription of IL-10 and repression of TNF-α and IL-1β). Right: longitudinal relation on natural logarithmic scales between mean levels of nuclear NF-κB and nuclear GRα observed by exposing naive PBL to plasma samples collected at randomization (rand) and after 3, 5, 7, and 10 days in the methylprednisolone (squares) and placebo (triangles) groups. With methylprednisolone, contrary to placebo, the intracellular relation between the NF-κB and GRα signaling pathways changed from an initial NF-κB-driven and GR-resistant state to a GRα-driven and GR-sensitive one. It is important to compare the two figures to appreciate how methylprednisolone supplementation restored the equilibrium between activation and suppression of inflammation that is distinctive of a regulated inflammatory response.
Prolonged Glucocorticoid Treatment in ARDS is Associated with Improved ARDS Outcomes: Lessons from Adult ARDS Studies
The updated literature on prolonged glucocorticoid treatment in adult patients with ARDS includes eight published randomized controlled trials (RCTs): four investigated methylprednisolone treatment initiated in early (<72 h; n = 118) (29, 30) or late ARDS (day 5–28; n = 204) (31, 32), and four investigated 7 days of hydrocortisone treatment in early ARDS (n = 297) (33–36). Additional publications include, one RCT recently presented in abstract form (n = 197) (37), seven cohort studies with historical or concurrent control (38–44), and eight observational reports (45–52).
As shown in Table 1, glucocorticoid administration, in comparison to placebo, was associated with a significant downregulation of systemic inflammation [reduction in inflammatory cytokines (29, 31, 32, 34), protein C (27, 30), and/or C-reactive protein levels (29–31, 33, 35)]. This biological response was associated with significant improvement in PaO2:FiO2 (29–36), and reduction in duration of mechanical ventilation (29–33, 35), and intensive care unit (ICU)-length of stay (1, 29, 30, 32, 33, 36). These consistently reproducible findings (29–36) “provide additional support for a causal relationship between reduction in systemic inflammation and resolution of ARDS that is further reinforced by experimental (53–56) and clinical (31, 38, 45, 46, 53, 57) data, showing that rebound inflammation following early removal of glucocorticoid treatment leads to recrudescence of ARDS that improves with reinstitution of glucocorticoid therapy” (58).
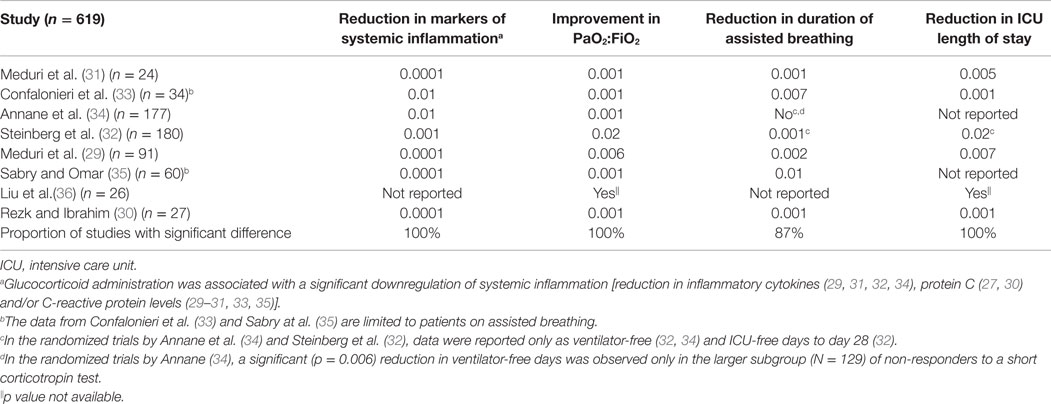
Table 1. Randomized trials investigating prolonged glucocorticoid treatment in early and late ARDS: level of significant difference (p value) – in comparison to control – for anti-inflammatory therapeutic effect and clinical–physiological parameters of disease resolution.
In a recent systematic review (58), we evaluated the effectiveness of prolonged glucocorticoid treatment in ARDS with two sets of intention-to-treat analyses: a primary analysis of individual patients data (IPD) of the four methylprednisolone RCTs (n = 322) (29–32) and a trial-level meta-analysis incorporating methylprednisolone and hydrocortisone RCTs (n = 297) (33–36). In the primary IPD analysis, definitions were standardized to derive outcomes of four RCTs investigating methylprednisolone treatment initiated in early (<72 h; n = 118) (29, 30) and late (after 5–7 days; n = 204) (1, 32) ARDS. In the early (29, 30) and late ARDS trials (31, 32), the initial daily methylprednisolone dosage was 1 and 2 mg/kg/day and duration of treatment extended up to 25 and 32 days, respectively. Since these RCTs differed in timing of treatment initiation [early (29, 30) vs. late (31, 32)] and speed of drug tapering [slow (29–31) vs. rapid (32)] after achieving initial unassisted breathing (UAB), the IPD analysis evaluated the impact of these treatment characteristics on outcomes. As shown in Table 2, glucocorticoid administration, in comparison to placebo, was associated with accelerated resolution of ARDS improving a broad spectrum of interrelated clinical outcomes and decreasing hospital mortality and health-care utilization. “By day 28, the methylprednisolone group achieved initial UAB earlier (12.4 ± 0.61 vs. 19.8 ± 0.78 days; HR 2.59, 95% CI 1.95–3.43, p < 0.001; Figure 3) and the effect was similar after adjusting for pre-specified covariates. Incorporating data past day 28, the methylprednisolone group also had shorter duration of initial AB (12.9 ± 13.4 vs. 23.0 ± 13.9 days, mean difference −10.1, 95% −13.12 to 7.08, p < 0.001; high certainty) (58). In the trial-level meta-analysis, glucocorticoid treatment was associated with a sizable and significant increase in MV-free days (13.3 vs. 7.6 days; mean difference 5.76, 95% CI 3.76–11.52; high certainty), and in ICU-free days (10.8 vs. 6.4 days; mean difference 4.45, 95% CI 2.64–6.26; moderate certainty)” (58).
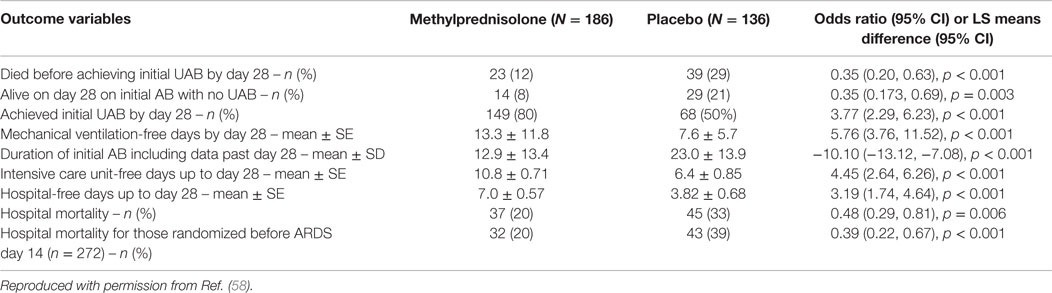
Table 2. Effects of prolonged methylprednisolone treatment based on individual patient data from four randomized clinical trials of acute respiratory distress syndrome.
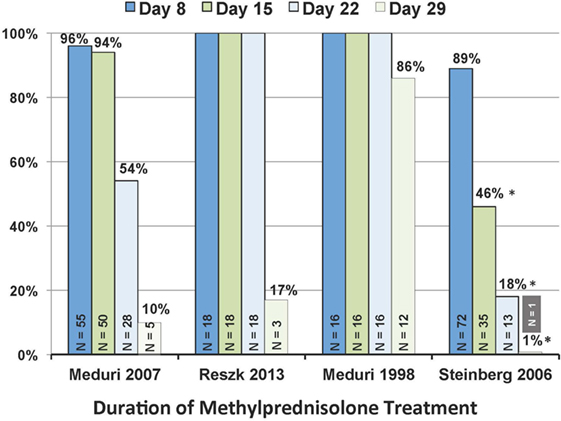
Figure 3. Patients, in each trial, alive on study day 8, 15, 22, and 29 that received at least 7, 14, 21, and 28 days of methylprednisolone treatment. N value within each column reports patients alive on the specified day. *P < 0.001 in comparison to the other three trials. Reproduced with permission (58).
Importantly, continuation of methylprednisolone administration after achieving UAB is essential to preserve the significant improvements achieved under treatment. The ARDS network trial (32), contrary to the other RCTs (29–31), had significantly (p < 0.001) shorter duration of treatment (Figure 4) as a result of rapid tapering soon after extubation (3.1 ± 1.1 days) (59). “The ARDS network trial (32), in comparison to the other trials (29–31), reported more methylprednisolone patients returning to AB (26 vs. 7%; p < 0.008) and to the ICU (21 vs. 2%; p = 0.007) by day 28. Return to AB (without reinstitution of glucocorticoid treatment in patients with adrenal suppression) was associated with higher hospital mortality (21 vs. 4%; p = 0.003), a factor recognized by the authors (32) to be an important reason why early significant physiological and survival (discharged home after initial wean: 62 vs. 49%, p = 0.006) (58) benefits did not translate into a 60-day survival (primary outcome) advantage.”
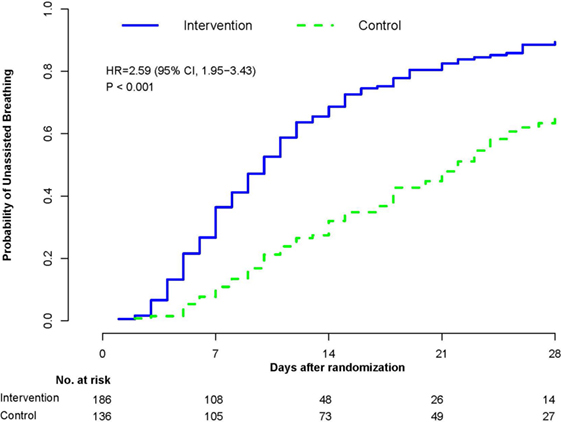
Figure 4. Probability of achieving unassisted breathing from randomization (methylprednisolone vs. placebo) to hospital discharge or day 28. Estimated cumulative incidence of achieving (initial) unassisted breathing by day 28 for patients with ARDS (n = 322) receiving either prolonged methylprednisolone treatment (blue solid line) or usual care (green dashed line). Death before achieving unassisted breathing is considered a competing risk. By day 28, the methylprednisolone group achieved initial UAB earlier (12.4 ± 0.61 vs. 19.8 ± 0.78 days; HR 2.59, 95% CI 1.95–3.43, p < 0.001). Reproduced with permission (58).
The ARDS network original report (32) found increased 60-day mortality for methylprednisolone-treated patients randomized after day 13 (32). “This subgroup (n = 48), however, had an uncharacteristically low mortality (8%) and large differences in baseline characteristics (60). When the analysis was adjusted for the imbalances at baseline, the mortality difference lost significance (25.6 vs. 13.2%; p = 0.325) (61). Irrespective of the interpretation of these data, there is a broad consensus that if glucocorticoid treatment is to be initiated, it should be initiated before day 14 of ARDS (62). For patients randomized before day 14 of ARDS onset, in the methylprednisolone trials, there was a 19% absolute reduction in hospital mortality (20 vs. 39%, p < 0.001) and a hazard ratio of 0.51 in time to death by hospital discharge or day 28 (95% CI 0.32–0.83) after adjusting for SOFA score and age. With treatment initiated before day 14, the number needed to treat (NNT) to save one life is 5.3.”
While the observed mortality benefits – originating from small-to-moderate size trials and investigating different treatment protocols – should be accepted with caution, concordance with the trial-level meta-analysis on hospital mortality (29–36) (36 vs. 49%, risk ratio 0.76, 95% CI 0.59–098; p = 0.035, I2 = 17%; moderate certainty) provides increased confidence for a survival benefit for those randomized before day 14 of ARDS. The benefits persist when the analysis includes a newly presented RCT (n = 197) (37) (29 vs. 44%; RR 0.62, 95% CI 0.44–0.86, p = 0.005; I2 = 46%). Additional support is also provided by the results of seven cohort studies with historical or concurrent control (n = 544) (38–43) reporting a significant mortality reduction (29 vs. 44%; RR 0.68, F-95%, CI 0.57–0.81; p < 0.001, I2 = 46%), and separate meta-analyses showing moderate certainty in a mortality reduction and in prevention of ARDS in patients hospitalized with community-acquired pneumonia (58), and high quality evidence for a mortality reduction for pneumocystis pneumonia (63).
Glucocorticoid Therapy for Pediatric ARDS
Besides low tidal volume ventilation (64) as part of a “lung protective” ventilation strategy, no other therapeutic approach has consistently shown improvement in the outcomes of children with ARDS or has routinely been implemented in our PICUs (65, 66). Unfortunately, even the effects of low tidal volume ventilation in pediatric ARDS (PARDS) are not based on adequately powered, randomized trials but are extrapolated from adult ARDS studies, single center observational studies, case reports, retrospective chart reviews, and pilot studies (67–70). Similarly, when compared to the adult literature on ARDS, our experience with glucocorticoid therapy for PARDS is limited. Nevertheless, the theoretical indications for using glucocorticoid therapy in PARDS are similar to those of adult ARDS. These include (1) a glucocorticoid receptor-mediated downregulation of systemic and pulmonary inflammation, which is essential for the restoration of tissue homeostasis, disease resolution, and prevention of lung fibrosis (71), and (2) an improvement in capillary-alveolar barrier function, with resolution of alveolar flooding and induction of ENaC (epithelial Na channel) expression and function (72–74). Conversely, concerns about glucocorticoid therapy for children with PARDS revolve around (1) the potential for bone growth retardation or growth arrest (75), (2) suppression of the immature immune system, including a potential increase in nosocomial infections or worsening of an existing infection (76), (3) a potential for glucocorticoid-induced hyperglycemia or systemic hypertension, and (4) psychiatric effects, such as insomnia or delirium (77–79).
Multiple reasons account for our lack of knowledge in regard to glucocorticoid-mediated effects in PARDS, including (1) the incidence of PARDS is lower than of adult ARDS [12.8 vs. 78.9 cases per 100,000 person years (65)], (2) mortality rates from PARDS are lower than from adult ARDS [18 vs. 38.5% (80)], (3) most pediatric intensivists consider glucocorticoid therapy an adjunctive therapy for severe, refractory PARDS, and (4) significant concerns persist among pediatricians regarding the potential adverse effects of glucocorticoids particularly on child growth and the immature immune system. Despite these limitations, it is clearly untenable to administer or withhold glucocorticoids from children with PARDS by simply extrapolating data from adult studies since the disease patterns, immunological responses, capacity for lung growth and development, and clinical outcomes of PARDS differ substantially from those of adult patients (81). PARDS caused by sepsis, pneumonia, and aspiration could conceptually respond favorably to glucocorticoid treatment, but we have little information about the susceptibility or risk factors for PARDS resulting from such triggers.
Several recent studies have confirmed that a large proportion of pediatric intensivists use adjunctive treatments in deteriorating PARDS patients, with glucocorticoids being utilized by 30–35% of pediatricians (82, 83). Interestingly, a Scandinavian study from 2015 reported that 83% of Nordic pediatricians administered glucocorticoids “sometimes” for PARDS patients, whereas only 17% admitted to “never” using glucocorticoids for PARDS (84).
Martinot et al. published the first report of glucocorticoids administration to children for refractory PARDS in 1997 (85). Six children (age 3–11 years) with moderate–severe PARDS who showed no improvement by days 4–17 of mechanical ventilation were treated with daily methylprednisolone boluses (30 mg/kg) for 3–6 days depending on the observed response. Glucocorticoid treatment was associated with significant improvements in oxygenation between days 1 and 5, and ultimately four of the six children survived. After the initial glucocorticoid bolus, three of the four survivors received a daily methylprednisolone maintenance dose of 2 mg/kg/day followed by a taper for a total treatment duration of 4–11 weeks. The fourth survivor did not receive maintenance therapy after the initial methylprednisolone bolus since a bacterial superinfection was suspected. Interestingly, none of the children sustained any glucocorticoid-related adverse events, including hypertension, nosocomial infections or gastrointestinal bleeding.
Two years later, Goh et al. reported of a 12-month-old with measles-associated refractory PARDS that was successfully treated with a 42-day course of methylprednisolone, consisting of 2 weeks of maintenance therapy and a 2-week taper (86). This protocol was based on the Meduri et al. (31) randomized trial investigating prolonged methylprednisolone treatment in adult patients with unresolving ARDS. However, Goh used significantly higher doses (5 mg/kg bolus, followed by 8 mg/kg/day maintenance) than those used in the Meduri trial (2 mg/kg bolus, 2 mg/kg/day maintenance). Despite the relatively high glucocorticoid dose, the only adverse effect noted was a fungal urinary tract infection, which responded to antifungal therapy and no other infectious, gastrointestinal, or metabolic adverse effects were observed. In 2006, Guglani et al. reported a 21-month-old child with unresolving PARDS related to malnutrition and eosinophilic gastroenteritis successfully treated with a 32-day course of methylprednisolone therapy (87). In contrast to Goh, Guglani administered a lower dose of methylprednisolone (2 mg/kg bolus, followed by 2 mg/kg/day maintenance), similar to the regimen proposed in the 1998 Meduri trial (31). Importantly, this child also suffered no metabolic, cardiovascular, or infectious adverse effects or muscle weakness from glucocorticoid therapy.
Over the past two decades, it became increasingly evident that surfactant loss is a major contributor to the development and progression of adult ARDS and PARDS. Neonatologists have long been aware of the stimulatory effects of glucocorticoids on lung maturation and surfactant production, an idea that is well supported by animal studies. In fact, in young rats with lipopolysaccharide-induced acute lung injury, early administration of dexamethasone (5 mg/kg) increased surfactant protein D levels (88). It seems plausible from this and other studies that glucocorticoids could have similar surfactant-stimulatory effects in PARDS. Although Willson et al. initially found a survival benefit in PARDS patients treated with surfactant (89), this effect was not confirmed in a later study (90). In a surfactant-deficient acute lung injury model, dexamethasone combined with surfactant administration conveyed no additional benefit over surfactant administration alone, despite decreasing nuclear NF-κB levels in lung homogenates (91). The surfactant-stimulating effects of glucocorticoids are well known in neonates, but a link between glucocorticoid administration and improved surfactant levels in PARDS has never been shown. Of interest, the ARDS network reported that patients randomized to the methylprednisolone vs. placebo group had an increased ratio of functional large surfactant aggregates to dysfunctional small aggregates in their cell-free supernatants (28).
While recognizing the difficulties of performing adequately powered RCTs in children, determining the utility of glucocorticoids in PARDS remains a high priority for pediatric intensivists. In 2015, Drago et al. used for the first time a double-blinded RCT design in a pilot study demonstrating the feasibility of administering prolonged, low-dose glucocorticoids and measuring clinically relevant outcomes in children with early PARDS (59). In the 35 patients randomized to placebo or glucocorticoid groups, no differences occurred in duration of mechanical ventilation, ICU stay, hospital stay, or mortality. However, the glucocorticoid group had higher PaO2/FiO2 ratios on days 8 and 9 and lower CO2 levels on days 2 and 3 of treatment, required fewer racemic epinephrine treatments for post-extubation stridor and less supplemental oxygen at PICU discharge. Importantly, glucocorticoid treatment (a single 2 mg/kg IV methylprednisolone bolus, followed by 7 days of 1 mg/kg/day methylprednisolone maintenance therapy and a 7-day taper) was not associated with any adverse effects, such as hyperglycemia or nosocomial infections. The strengths of this study included the enrollment of a generalizable PICU population, a randomized placebo-controlled study design, masked study drug infusions, a standardized regimen of low-dose methylprednisolone, a priori definition of primary and secondary outcomes and of potential adverse drug effects, as well as an intention-to-treat analysis with complete follow-up of all enrolled subjects. Limitations of this study included the relatively small sample size (17 and 18 patients in the glucocorticoid and placebo groups, respectively) and failure to strictly standardize ventilator management and ventilator weaning strategies. Although a 2-week glucocorticoid course can be considered as “prolonged” treatment and has been used in several adult ARDS trials, the most prominent benefits in the adult studies occurred with a 4-week course of glucocorticoids (58). Furthermore, although this exploratory study demonstrated the feasibility of prolonged glucocorticoid infusions in PARDS patients without any obvious adverse effects, a proper safety analysis could not be performed with this sample size.
Later in 2015, the same authors published a follow-up study describing alterations in plasma biomarkers in PARDS patients and associations of these biomarkers with the changes in clinical outcomes observed earlier (92). Interestingly, the placebo group showed higher IL-15 and basophil levels at study entry, whereas on day 7 this group showed lower IL-1α, IFN-γ, and IL-10 levels. In contrast, the glucocorticoid group showed lower INF-α, IL-6, IL-10, MCP-1, G-CSF, and GM-CSF levels at study entry, but higher IL-17α levels on day 7. Total and differential cell counts remained unchanged within the placebo group between days 0 and 7, whereas in the glucocorticoid group, total WBC and platelets counts were increased on day 7 (92). The authors also proposed a series of positive and negative correlations between cytokine levels, cell counts, coagulation parameters, and clinical parameters of disease severity. Although inflammatory biomarkers can be successfully measured in PARDS patients with commercially available techniques, limitations of this study include again the small sample size (35 patients), the relatively short study period of 7 days, the inability to compare plasma to BAL samples (an intrinsic problem for most pediatric studies of lung disease), and the lack of functional assays to uncover any mechanistic consequences of these alterations in biomarkers.
In the same year, Yehya et al. published a prospective, observational single center study of 283 PARDS patients describing the association between glucocorticoid exposure for >24 h and the clinical outcomes of these patients (93). Interestingly, similar to the Scandinavian survey, the majority (60%) of PARDS patients in this US-based study received glucocorticoids for >24 h and only 23% did not receive any type of glucocorticoid. However, the glucocorticoid most commonly used was hydrocortisone and not methylprednisolone, the most commonly administered glucocorticoid in large-scale, adult ARDS trials showing improvements in outcomes. Yehya et al. found that all-type glucocorticoid exposure for >24 h was associated with increased mortality in PARDS, fewer ventilator-free days at 28 days, and longer duration of mechanical ventilation in survivors, although after multivariate and propensity score adjustments, the increase in mortality was no longer detectable. Strengths of this study include the larger sample size and meticulous documentation of all types of glucocorticoid exposures. The study also revealed that currently pediatricians use multiple types of glucocorticoids for PARDS patients and for a variety of indications, with shock ranking as the most common. However, the outcomes of this study stand in marked contradiction to multiple, large-scale, and highly powered, randomized adult ARDS trials, which consistently show improvements in clinical outcomes and inflammatory biomarkers. This discrepancy may be explained by several limitations of the Yehya study, including (1) not recording the specific indication for glucocorticoid administration, (2) grouping unexposed and short-term (<24 h) glucocorticoid-exposed patients together as a control group, and (3) no implementation of a glucocorticoid weaning protocol to prevent rebound inflammation, which is a key feature of the adult, glucocorticoid-favoring ARDS trials. These flaws create major challenges in the interpretation of the data and in assigning clinical relevance to these findings.
Clearly, much more research is needed before we can make an educated decision about the use of glucocorticoids for all, or even some, PARDS patients. Nevertheless, most recently the expert panel of the 2015 Pediatric Acute Lung Injury Consensus Conference recommended with “strong agreement” against the use of glucocorticoid therapy for PARDS. These experts highlighted the need for future research to identify specific pediatric patient populations most likely to benefit from glucocorticoid therapy and encouraged the development of pediatric dosing and delivery regimens (94). Another expert review proposed that ARDS patients with adrenocortical insufficiency would demonstrate greater benefit with glucocorticoids as compared to patients with an intact hypothalamic–pituitary–adrenal axis (95).
Future Research Priorities
1. The relatively low incidence and mortality rates from PARDS in comparison to adult ARDS mandate the careful design of a large, multicenter RCT to determine the effects of prolonged glucocorticoid therapy on PARDS outcomes, such as mortality, duration of mechanical ventilation, ICU-free days, or hospital stay, since a single center study is unlikely to achieve adequate power. Drago et al. calculated that in order to achieve a 15, 28, or 36% reduction in the duration of mechanical ventilation, we need to enroll 547, 137, or 98 subjects, respectively, in the placebo and the treatment groups (assuming an α-error of 0.01 and a power of 80%) (59). The design of this study should aim at standardizing not only the glucocorticoid protocol but also the mechanical ventilation and ventilator weaning protocols, as well as sedation, fluid management, and nutrition strategies.
2. Although it is safe to assume that patients with different PARDS etiologies will respond differently to prolonged glucocorticoid therapy, an initial trial should enroll all patients who meet PARDS criteria based on the 2015 Pediatric Acute Lung Injury Consensus Conference (94). Stratification of enrollment according to their PARDS etiologies will allow a balanced distribution of PARDS etiologies in the treated and untreated groups, as well as subgroup comparisons of glucocorticoid effectiveness for different subpopulations within these strata.
3. Efforts to define the side effects and safety profile of prolonged, low-dose glucocorticoid administration should receive a high priority. While Drago et al. showed the feasibility of administering prolonged glucocorticoid infusions to children with PARDS using a randomized, double-blinded study design, due to the relatively low number of patients enrolled, a safety analysis could not be performed, which needs to be assessed in futures studies.
4. The molecular and clinical consequences of potential rebound effects after glucocorticoid discontinuation are unknown in children with PARDS. In the adult ARDS trials, a prolonged glucocorticoid weaning protocol was required to sustain improved outcomes. In contrast, rapid discontinuation of glucocorticoids, including in the ARDSnet trial, consistently led to increased readmission rates to the ICU, reinstitution of mechanical ventilation, and increased mortality (31, 96). It is unknown whether clinical deterioration after abrupt glucocorticoid discontinuation holds true for children with PARDS.
5. In both adult and pediatric ARDS trials, prolonged glucocorticoid treatment was not associated with increased risk of nosocomial infections. Other glucocorticoid-induced adverse effects, such as hyperglycemia and hypertension, are rarely reported and easily controlled. Nevertheless, the effects of prolonged glucocorticoid therapy on the immune response of chronically immunosuppressed children (such as solid organ and bone marrow recipients) or children with autoimmune diseases remain unknown. In addition, we need to determine the effects of glucocorticoid therapy for PARDS on children who are chronically exposed to glucocorticoids but not immunosuppressed in the classical sense [e.g., inhaled glucocorticoids for long-term asthma or cystic fibrosis control, or nasal glucocorticoids for allergic rhinitis (97)].
6. Many pediatricians are concerned about the long-term consequences of prolonged glucocorticoid exposure on bone growth and child development. The widespread use of short-term glucocorticoid bursts (e.g., for status asthmaticus or acute adrenal crisis) does not appear to affect long-term growth. On the other hand, exposure to inhaled or oral glucocorticoids for months to years (e.g., asthma control therapy) may decrease the growth potential of children (79). Significant negative effects on bone growth after glucocorticoid exposure for 2 weeks, as proposed in the study design by Drago et al. (59), is unlikely to affect the final height of children, but this needs to confirmed. In contrast, the adult ARDS trials recommend glucocorticoid therapy for 4 weeks (96), potentially carrying greater risk for growth-related long-term effects. Interestingly, critically ill children who suffered burn wounds actually show an increase in lean body mass, bone mineral content, and bone mineral density after prolonged exposure to synthetic glucocorticoids (98). These findings should encourage us to consider different types of glucocorticoids for PARDS therapy in order to minimize potential adverse effects.
7. The consequences of a prolonged glucocorticoid course on the effectiveness of routine vaccinations and vaccination catch-up schedules are unknown and need to be determined.
8. Dysregulated acute and chronic systemic inflammation is associated with long-term systemic morbidity in ARDS survivors, including muscle weakness with impaired physical function (99) and neurocognitive impairment (100). PARDS RCTs must include long-term follow-up evaluating functional status and cognitive function.
In summary, although a robust body of adult literature points toward improved outcomes of ARDS patients treated with prolonged glucocorticoid regimens, including a slow, standardized glucocorticoid wean, the relative lack of pediatric data should encourage us to maintain equipoise toward glucocorticoid therapy for PARDS. We should neither assume that the adult results can be directly applied to a pediatric population, nor should we propagate generalized statements that all exposures to glucocorticoids, including a 4-week treatment course for PARDS, will increase the risk of nosocomial infections, cause hypertension, hyperglycemia, or growth retardation, since this is neither supported by the adult nor pediatric literature on glucocorticoid use in ARDS. Clearly, there is an urgent need for large-scale, well-designed RCTs in Pediatrics to determine the role of prolonged glucocorticoid therapy for PARDS.
Author Contributions
All authors listed, have made substantial, direct and intellectual contribution to the work, and approved it for publication.
Conflict of Interest Statement
The authors declare that the research was conducted in the absence of any commercial or financial relationships that could be construed as a potential conflict of interest.
Funding
This material is the result of work supported with the resources and use of facilities at the Memphis VA Medical Center. The contents of this commentary do not represent the views of the U.S. Department of Veterans Affairs or the United States Government. Funding for the respective trials was disclosed in the original publications. None of the sponsors had any role in the design and conduct of the study, the collection, management, analysis and interpretation of the data, the preparation, review, or approval of the report, or the decision to submit the manuscript for publication. The corresponding author had full access to all the data in the study and had final responsibility for the decision to submit for publication.
References
1. Meduri GU, Annane D, Chrousos GP, Marik PE, Sinclair SE. Activation and regulation of systemic inflammation in ARDS: rationale for prolonged glucocorticoid therapy. Chest (2009) 136(6):1631–43. doi:10.1378/chest.08-2408
2. Meduri GU, Eltorky MA. Understanding ARDS-associated fibroproliferation. Intensive Care Med (2015) 41(3):517–20. doi:10.1007/s00134-014-3613-0
3. Meduri GU, Muthiah MP, Carratu P, Eltorky M, Chrousos GP. Nuclear factor-kappaB- and glucocorticoid receptor alpha-mediated mechanisms in the regulation of systemic and pulmonary inflammation during sepsis and acute respiratory distress syndrome. Evidence for inflammation-induced target tissue resistance to glucocorticoids. Neuroimmunomodulation (2005) 12(6):321–38. doi:10.1159/000091126
4. Didonato JA, Saatcioglu F, Karin M. Molecular mechanisms of immunosuppression and anti-inflammatory activities by glucocorticoids. Am J Respir Crit Care Med (1996) 154(2 Pt 2):S11–5. doi:10.1164/ajrccm/154.2_Pt_2.S11
5. Bamberger CM, Schulte HM, Chrousos GP. Molecular determinants of glucocorticoid receptor function and tissue sensitivity to glucocorticoids. Endocr Rev (1996) 17(3):245–61. doi:10.1210/edrv-17-3-245
6. Scheinman RI, Cogswell PC, Lofquist AK, Baldwin AS Jr. Role of transcriptional activation of I kappa B alpha in mediation of immunosuppression by glucocorticoids. Science (1995) 270(5234):283–6. doi:10.1126/science.270.5234.283
7. Wissink S, van Heerde EC, vand der Burg B, van der Saag PT. A dual mechanism mediates repression of NF-kappaB activity by glucocorticoids. Mol Endocrinol (1998) 12(3):355–63. doi:10.1210/mend.12.3.0081
8. Wang P, Wu P, Siegel MI, Egan RW, Billah MM. Interleukin (IL)-10 inhibits nuclear factor kappa B (NF kappa B) activation in human monocytes. IL-10 and IL-4 suppress cytokine synthesis by different mechanisms. J Biol Chem (1995) 270(16):9558–63. doi:10.1074/jbc.270.16.9558
9. Lentsch AB, Shanley TP, Sarma V, Ward PA. In vivo suppression of NF-kappa B and preservation of I kappa B alpha by interleukin-10 and interleukin-13. J Clin Invest (1997) 100(10):2443–8. doi:10.1172/JCI119786
10. Shames BD, Selzman CH, Meldrum DR, Pulido EJ, Barton HA, Meng X, et al. Interleukin-10 stabilizes inhibitory kappaB-alpha in human monocytes. Shock (1998) 10(6):389–94. doi:10.1097/00024382-199812000-00002
11. Hoffman SL, Punjabi NH, Kumala S, Moechtar MA, Pulungsih SP, Rivai AR, et al. Reduction of mortality in chloramphenicol-treated severe typhoid fever by high-dose dexamethasone. N Engl J Med (1984) 310(2):82–8. doi:10.1056/NEJM198401123100203
12. Poppers DM, Schwenger P, Vilcek J. Persistent tumor necrosis factor signaling in normal human fibroblasts prevents the complete resynthesis of I kappa B-alpha. J Biol Chem (2000) 275(38):29587–93. doi:10.1074/jbc.M002806200
13. Sheppard KA, Phelps KM, Williams AJ, Thanos D, Glass CK, Rosenfeld MG, et al. Nuclear integration of glucocorticoid receptor and nuclear factor-kappaB signaling by CREB-binding protein and steroid receptor coactivator-1. J Biol Chem (1998) 273(45):29291–4. doi:10.1074/jbc.273.45.29291
14. Amano Y, Lee SW, Allison AC. Inhibition by glucocorticoids of the formation of interleukin-1 alpha, interleukin-1 beta, and interleukin-6: mediation by decreased mRNA stability. Mol Pharmacol (1993) 43(2):176–82.
15. Barnes PJ, Karin M. Nuclear factor-kappaB: a pivotal transcription factor in chronic inflammatory diseases. N Engl J Med (1997) 336(15):1066–71. doi:10.1056/NEJM199704103361506
16. Serhan CN, Savill J. Resolution of inflammation: the beginning programs the end. Nat Immunol (2005) 6(12):1191–7. doi:10.1038/ni1276
17. Busillo JM, Cidlowski JA. The five Rs of glucocorticoid action during inflammation: ready, reinforce, repress, resolve, and restore. Trends Endocrinol Metab (2013) 24(3):109–19. doi:10.1016/j.tem.2012.11.005
18. Meduri GU, Yates CR. Systemic inflammation-associated glucocorticoid resistance and outcome of ARDS. Ann N Y Acad Sci (2004) 1024:24–53. doi:10.1196/annals.1321.004
19. Incerpi EK, Oliveira LM, Pereira EM, Soncini R. Inhibition of endogenous glucocorticoid synthesis aggravates lung injury triggered by septic shock in rats. Int J Exp Pathol (2015) 96(3):133–9. doi:10.1111/iep.12113
20. Zhou T, Fan XM, Wang YQ, Qi YJ, Chen H, Qian SY. [Effects of different doses of hydrocortisone on acute lung injury in rats with early septic shock induced by Escherichia coli]. Zhonghua Er Ke Za Zhi (2004) 42(9):644–8.
21. Kamiyama K, Matsuda N, Yamamoto S, Takano K, Takano Y, Yamazaki H, et al. Modulation of glucocorticoid receptor expression, inflammation, and cell apoptosis in septic guinea pig lungs using methylprednisolone. Am J Physiol Lung Cell Mol Physiol (2008) 295(6):L998–1006. doi:10.1152/ajplung.00459.2007
22. Wang XQ, Zhou X, Zhou Y, Rong L, Gao L, Xu W. Low-dose dexamethasone alleviates lipopolysaccharide-induced acute lung injury in rats and upregulates pulmonary glucocorticoid receptors. Respirology (2008) 13(6):772–80. doi:10.1111/j.1440-1843.2008.01344.x
23. Zhang Y, Zuo W, Rong Q, Teng G, Zhang Y. Glucocorticoid receptor expression on acute lung injury induced by endotoxin in rats. World J Emerg Med (2010) 1(1):65–9.
24. Bassford C. 11β-Hydroxysteroid Dehydrogenase Glucocorticoid Metabolism within the Lung and Its Influence on Macrophage Function in the Acute Respiratory Distress Syndrome [Graduation Thesis]. A Thesis Submitted for the Degree of PhD at the University of Warwick. Coventry: University of Warwick (2011).
25. Meduri GU, Tolley EA, Chrousos GP, Stentz F. Prolonged methylprednisolone treatment suppresses systemic inflammation in patients with unresolving acute respiratory distress syndrome: evidence for inadequate endogenous glucocorticoid secretion and inflammation-induced immune cell resistance to glucocorticoids. Am J Respir Crit Care Med (2002) 165(7):983–91. doi:10.1164/ajrccm.165.7.2106014
26. Meduri GU, Kohler G, Headley S, Tolley E, Stentz F, Postlethwaite A. Inflammatory cytokines in the BAL of patients with ARDS. Persistent elevation over time predicts poor outcome. Chest (1995) 108(5):1303–14. doi:10.1378/chest.108.5.1303
27. Seam N, Meduri GU, Wang H, Nylen ES, Sun J, Schultz MJ, et al. Effects of methylprednisolone infusion on markers of inflammation, coagulation, and angiogenesis in early acute respiratory distress syndrome. Crit Care Med (2012) 40(2):495–501. doi:10.1097/CCM.0b013e318232da5e
28. Hite RSM, Grier B, Morris P, Goodman R, Schoenfeld D. Impact of steroid therapy for late ARDS on surfactant recovery in the ARDS Network LASRS Trial. Am J Respir Crit Care Med (2006) 173:A641.
29. Meduri GU, Golden E, Freire AX, Taylor E, Zaman M, Carson SJ, et al. Methylprednisolone infusion in early severe ARDS: results of a randomized controlled trial. Chest (2007) 131(4):954–63. doi:10.1378/chest.06-2100
30. Rezk N, Ibrahim A. Effects of methylprednisolone in early ARDS. Egypt J Chest Dis Tuberc (2013) 62(1):167–72. doi:10.1016/j.ejcdt.2013.02.013
31. Meduri GU, Headley AS, Golden E, Carson SJ, Umberger RA, Kelso T, et al. Effect of prolonged methylprednisolone therapy in unresolving acute respiratory distress syndrome: a randomized controlled trial. JAMA (1998) 280(2):159–65. doi:10.1001/jama.280.2.159
32. Steinberg KP, Hudson LD, Goodman RB, Hough CL, Lanken PN, Hyzy R, et al. Efficacy and safety of corticosteroids for persistent acute respiratory distress syndrome. N Engl J Med (2006) 354(16):1671–84. doi:10.1056/NEJMoa051693
33. Confalonieri M, Urbino R, Potena A, Piattella M, Parigi P, Puccio G, et al. Hydrocortisone infusion for severe community-acquired pneumonia: a preliminary randomized study. Am J Respir Crit Care Med (2005) 171(3):242–8. doi:10.1164/rccm.200406-808OC
34. Annane D, Sebille V, Bellissant E; Ger-Inf-05 Study Group. Effect of low doses of corticosteroids in septic shock patients with or without early acute respiratory distress syndrome. Crit Care Med (2006) 34(1):22–30. doi:10.1097/01.CCM.0000194723.78632.62
35. Sabry NA, Omar EE-D. Corticosteroids and ICU course of community acquired pneumonia in Egyptian settings. Pharmacol Pharm (2011) 2:73–81. doi:10.4236/pp.2011.22009
36. Liu L, Li J, Huang YZ, Liu SQ, Yang CS, Guo FM, et al. [The effect of stress dose glucocorticoid on patients with acute respiratory distress syndrome combined with critical illness-related corticosteroid insufficiency]. Zhonghua Nei Ke Za Zhi (2012) 51(8):599–603.
37. Tongyoo S, Permpikul C, Vattanavanit V, Mongkolpun W. Hydrocortisone in treatment of severe sepsis and septic shock with acute respiratory distress syndrome: a randomised controlled trial. Intensive Care Med Exp (2015) 3(Suppl 1):A808. doi:10.1186/2197-425X-3-S1-A808
38. Keel JB, Hauser M, Stocker R, Baumann PC, Speich R. Established acute respiratory distress syndrome: benefit of corticosteroid rescue therapy. Respiration (1998) 65(4):258–64. doi:10.1159/000029273
39. Varpula T, Pettila V, Rintala E, Takkunen O, Valtonen V. Late steroid therapy in primary acute lung injury. Intensive Care Med (2000) 26(5):526–31. doi:10.1007/s001340051199
40. Huh J, Lim C, Jegal Y, Le S, Kim W, Kim D, et al. The effect of steroid therapy in patients with late ARDS. Tuberc Respir Dis (2002) 52:376–84. doi:10.4046/trd.2002.52.4.376
41. Lee HS, Lee JM, Kim MS, Kim HY, Hwangbo B, Zo JI. Low-dose steroid therapy at an early phase of postoperative acute respiratory distress syndrome. Ann Thorac Surg (2005) 79(2):405–10. doi:10.1016/j.athoracsur.2004.07.079
42. Azoulay E, Canet E, Raffoux E, Lengline E, Lemiale V, Vincent F, et al. Dexamethasone in patients with acute lung injury from acute monocytic leukaemia. Eur Respir J (2012) 39(3):648–53. doi:10.1183/09031936.00057711
43. Taborda L, Barros F, Fonseca V, Irimia M, Carvalho R, Diogo C, et al. Acute respiratory distress syndrome: case series, two years at an intensive care unit. Acta Med Port (2014) 27(2):211–7. doi:10.20344/amp.4266
44. Xiao Z, Zhou J, Chen Z, Wu K, Li X. Effects of glucocorticoids on outcome of patients with acute respiratory distress syndrome. Chin J TCM WM Crit Care (2015) 22(1):83–5.
45. Ashbaugh DG, Maier RV. Idiopathic pulmonary fibrosis in adult respiratory distress syndrome. Diagnosis and treatment. Arch Surg (1985) 120(5):530–5. doi:10.1001/archsurg.1985.01390290012002
46. Hooper RG, Kearl RA. Established ARDS treated with a sustained course of adrenocortical steroids. Chest (1990) 97(1):138–43. doi:10.1378/chest.97.1.138
47. Meduri GU, Chinn AJ, Leeper KV, Wunderink RG, Tolley E, Winer-Muram HT, et al. Corticosteroid rescue treatment of progressive fibroproliferation in late ARDS. Patterns of response and predictors of outcome. Chest (1994) 105(5):1516–27. doi:10.1378/chest.105.5.1516
48. Meduri GU, Headley S, Tolley E, Shelby M, Stentz F, Postlethwaite A. Plasma and BAL cytokine response to corticosteroid rescue treatment in late ARDS. Chest (1995) 108(5):1315–25. doi:10.1378/chest.108.5.1315
49. Biffl WL, Moore FA, Moore EE, Haenel JB, McIntyre RC Jr, Burch JM. Are corticosteroids salvage therapy for refractory acute respiratory distress syndrome? Am J Surg (1995) 170(6):591–5. doi:10.1016/S0002-9610(99)80022-1
50. Song ZF, Guo XH, Wang SY, Xie W, Yin N, Zhang Y, et al. [Evaluation of glucocorticoid in treatment for patients with acute respiratory distress syndrome as a result of serious community-acquired pneumonia]. Zhongguo Wei Zhong Bing Ji Jiu Yi Xue (2003) 15(11):669–74.
51. Gupta L, James B. Prone position and intravenous steroids for the management of severe ARDS. Thorax (2012) 67(Suppl 2):A38. doi:10.1136/thoraxjnl-2012-202678.083
52. Jeon K, Chung CR, Yang JH, Park CM, Suh GY. Predictors of response to corticosteroid treatment in patients with early acute respiratory distress syndrome: results of a pilot study. Yonsei Med J (2015) 56(1):287–91. doi:10.3349/ymj.2015.56.1.287
53. Meduri GU. An historical review of glucocorticoid treatment in sepsis. Disease pathophysiology and the design of treatment investigation. Sepsis (1999) 3:21–38. doi:10.1023/A:1009870524359
54. Hesterberg TW, Last JA. Ozone-induced acute pulmonary fibrosis in rats. Prevention of increased rates of collagen synthesis by methylprednisolone. Am Rev Respir Dis (1981) 123(1):47–52.
55. Hakkinen PJ, Schmoyer RL, Witschi HP. Potentiation of butylated-hydroxytoluene-induced acute lung damage by oxygen. Effects of prednisolone and indomethacin. Am Rev Respir Dis (1983) 128(4):648–51.
56. Kehrer JP, Klein-Szanto AJ, Sorensen EM, Pearlman R, Rosner MH. Enhanced acute lung damage following corticosteroid treatment. Am Rev Respir Dis (1984) 130(2):256–61.
57. Gagnon S, Boota AM, Fischl MA, Baier H, Kirksey OW, La Voie L. Corticosteroids as adjunctive therapy for severe Pneumocystis carinii pneumonia in the acquired immunodeficiency syndrome. A double-blind, placebo-controlled trial. N Engl J Med (1990) 323(21):1444–50. doi:10.1056/NEJM199011223232103
58. Meduri GU, Bridges L, Shih MC, Marik PE, Siemieniuk RA, Kocak M. Prolonged glucocorticoid treatment is associated with improved ARDS outcomes: analysis of individual patients’ data from four randomized trials and trial-level meta-analysis of the updated literature. Intensive Care Med (2016) 42:829–40.
59. Drago BB, Kimura D, Rovnaghi CR, Schwingshackl A, Rayburn M, Meduri GU, et al. Double-blind, placebo-controlled pilot randomized trial of methylprednisolone infusion in pediatric acute respiratory distress syndrome. Pediatr Crit Care Med (2015) 16(3):e74–81. doi:10.1097/PCC.0000000000000349
60. Annane D, Meduri GU, Marik P. Critical illness-related corticosteroid insufficiency and community-acquired pneumonia: back to the future! Eur Respir J (2008) 31(6):1150–2. doi:10.1183/09031936.00040908
61. Thompson BT, Ancukiewicz M, Hudson LD, Steinberg KP, Bernard GR. Steroid treatment for persistent ARDS: a word of caution. Crit Care (2007) 11(6):425. doi:10.1186/cc6186
62. Marik PE, Pastores S, Annane D, Meduri G, Sprung C, Arlt W, et al. Clinical practice guidelines for the diagnosis and management of corticosteroid insufficiency in critical illness: recommendations of an international task force. Crit Care Med (2008) 36:1937–49. doi:10.1097/CCM.0b013e31817603ba
63. Ewald H, Raatz H, Boscacci R, Furrer H, Bucher HC, Briel M. Adjunctive corticosteroids for Pneumocystis jiroveci pneumonia in patients with HIV infection. Cochrane Database Syst Rev (2015) 4:CD006150. doi:10.1002/14651858.CD006150.pub2
64. The Acute Respiratory Distress Syndrome Network. Ventilation with lower tidal volumes as compared with traditional tidal volumes for acute lung injury and the acute respiratory distress syndrome. N Engl J Med (2000) 342(18):1301–8. doi:10.1056/NEJM200005043421801
65. Zimmerman JJ, Akhtar SR, Caldwell E, Rubenfeld GD. Incidence and outcomes of pediatric acute lung injury. Pediatrics (2009) 124(1):87–95. doi:10.1542/peds.2007-2462
66. Slutsky AS, Ranieri VM. Mechanical ventilation: lessons from the ARDSNet trial. Respir Res (2000) 1(2):73–7. doi:10.1186/rr15
67. Sousse LE, Herndon DN, Andersen CR, Ali A, Benjamin NC, Granchi T, et al. High tidal volume decreases adult respiratory distress syndrome, atelectasis, and ventilator days compared with low tidal volume in pediatric burned patients with inhalation injury. J Am Coll Surg (2015) 220(4):570–8. doi:10.1016/j.jamcollsurg.2014.12.028
68. Sarkar D, Sarkar S, Anand S, Kapoor A. Lung protective strategy and prone ventilation resulting in successful outcome in a patient with ARDS due to H1N1. BMJ Case Rep (2011). doi:10.1136/bcr.10.2010.3420
69. Hanson JH, Flori H. Application of the acute respiratory distress syndrome network low-tidal volume strategy to pediatric acute lung injury. Respir Care Clin N Am (2006) 12(3):349–57.
70. Mehta NM, Arnold JH. Mechanical ventilation in children with acute respiratory failure. Curr Opin Crit Care (2004) 10(1):7–12. doi:10.1097/00075198-200402000-00002
71. Meduri GU, Bell W, Sinclair S, Annane D. Pathophysiology of acute respiratory distress syndrome. Glucocorticoid receptor-mediated regulation of inflammation and response to prolonged glucocorticoid treatment. Presse Med (2011) 40(12 Pt 2):e543–60. doi:10.1016/j.lpm.2011.04.023
72. Vaage J. Effects of high-dose corticosteroids on the pulmonary circulation. Acta Chir Scand Suppl (1985) 526:73–82.
73. Davis IC, Matalon S. Epithelial sodium channels in the adult lung – important modulators of pulmonary health and disease. Adv Exp Med Biol (2007) 618:127–40. doi:10.1007/978-0-387-75434-5_10
74. Xinmin D, Yunyou D, Chaosheng P, Huasong F, Pingkun Z, Jiguang M, et al. Dexamethasone treatment attenuates early seawater instillation-induced acute lung injury in rabbits. Pharmacol Res (2006) 53(4):372–9. doi:10.1016/j.phrs.2006.01.006
75. Pruteanu AI, Chauhan BF, Zhang L, Prietsch SO, Ducharme FM. Inhaled corticosteroids in children with persistent asthma: dose-response effects on growth. Evid Based Child Health (2014) 9(4):931–1046. doi:10.1002/ebch.1989
76. Sarwal MM. Out with the old, in with the new: immunosuppression minimization in children. Curr Opin Organ Transplant (2008) 13(5):513–21. doi:10.1097/MOT.0b013e328310b0e0
77. Greaves RF, Jevalikar G, Hewitt JK, Zacharin MR. A guide to understanding the steroid pathway: new insights and diagnostic implications. Clin Biochem (2014) 47(15):5–15. doi:10.1016/j.clinbiochem.2014.07.017
78. Drozdowicz LB, Bostwick JM. Psychiatric adverse effects of pediatric corticosteroid use. Mayo Clin Proc (2014) 89(6):817–34. doi:10.1016/j.mayocp.2014.01.010
79. Kapadia CR, Nebesio TD, Myers SE, Willi S, Miller BS, Allen DB, et al. Endocrine effects of inhaled corticosteroids in children. JAMA Pediatr (2016) 170(2):163–70. doi:10.1001/jamapediatrics.2015.3526
80. Rubenfeld GD, Caldwell E, Peabody E, Weaver J, Martin DP, Neff M, et al. Incidence and outcomes of acute lung injury. N Engl J Med (2005) 353(16):1685–93. doi:10.1056/NEJMoa050333
81. Kneyber MC, Zhang H, Slutsky AS. Ventilator-induced lung injury: similarity and differences between children and adults. Am J Respir Crit Care Med (2014) 190(3):258–65. doi:10.1164/rccm.201401-0168CP
82. Santschi M, Randolph AG, Rimensberger PC, Jouvet P; Pediatric Acute Lung Injury Mechanical Ventilation Investigators; Pediatric Acute Lung Injury and Sepsis Investigators Network, et al. Mechanical ventilation strategies in children with acute lung injury: a survey on stated practice pattern*. Pediatr Crit Care Med (2013) 14(7):e332–7. doi:10.1097/PCC.0b013e31828a89a2
83. Santschi M, Jouvet P, Leclerc F, Gauvin F, Newth CJ, Carroll CL, et al. Acute lung injury in children: therapeutic practice and feasibility of international clinical trials. Pediatr Crit Care Med (2010) 11(6):681–9. doi:10.1097/PCC.0b013e3181d904c0
84. Jensen LL, Baratt-Due A, Englund PN, Harju JA, Sigurethsson TS, Liberg JP. Paediatric ventilation treatment of acute lung injury in Nordic intensive care units. Acta Anaesthesiol Scand (2015) 59(5):568–75. doi:10.1111/aas.12500
85. Martinot A, Fourier C, Cremer R, Hue V, Deschildre A, Leclerc F. Short-course, high-dose corticosteroid treatment in six children with late ARDS. Pediatr Pulmonol (1997) 23(4):314–6.
86. Goh AY, Sekaran D, Roziah M. Corticosteroid rescue in late paediatric acute respiratory distress syndrome. Respirology (1999) 4(3):295–7. doi:10.1046/j.1440-1843.1999.00194.x
87. Guglani L, Jain S, Lodha R. Methylprednisolone therapy in a child with unresolving ARDS. Indian Pediatr (2006) 43(7):639–42.
88. Shu LH, Xue XD, Shu LH, Liu CF, Han XH, Wu HM, et al. Effect of dexamethasone on the content of pulmonary surfactant protein D in young rats with acute lung injury induced by lipopolysaccharide. Zhongguo Dang Dai Er Ke Za Zhi (2007) 9(2):155–8.
89. Willson DF, Thomas NJ, Markovitz BP, Bauman LA, DiCarlo JV, Pon S, et al. Effect of exogenous surfactant (calfactant) in pediatric acute lung injury: a randomized controlled trial. JAMA (2005) 293(4):470–6. doi:10.1001/jama.293.4.470
90. Willson DF, Thomas NJ, Tamburro R, Truemper E, Truwit J, Conaway M, et al. Pediatric calfactant in acute respiratory distress syndrome trial. Pediatr Crit Care Med (2013) 14(7):657–65. doi:10.1097/PCC.0b013e3182917b68
91. von Bismarck P, Klemm K, Garcia Wistadt CF, Winoto-Morbach S, Schutze S, Krause MF. Selective NF-kappaB inhibition, but not dexamethasone, decreases acute lung injury in a newborn piglet airway inflammation model. Pulm Pharmacol Ther (2009) 22(4):297–304. doi:10.1016/j.pupt.2009.02.002
92. Schwingshackl A, Kimura D, Rovnaghi CR, Saravia JS, Cormier SA, Teng B, et al. Regulation of inflammatory biomarkers by intravenous methylprednisolone in pediatric ARDS patients: results from a double-blind, placebo-controlled randomized pilot trial. Cytokine (2016) 77:63–71. doi:10.1016/j.cyto.2015.10.007
93. Yehya N, Servaes S, Thomas NJ, Nadkarni VM, Srinivasan V. Corticosteroid exposure in pediatric acute respiratory distress syndrome. Intensive Care Med (2015) 41(9):1658–66. doi:10.1007/s00134-015-3953-4
94. Jouvet P, Thomas NJ, Wilson DF, Erickson S, Khemani R, Zimmerman J, et al. Pediatric acute respiratory distress syndrome: consensus recommendations from the Pediatric Acute Lung Injury Consensus Conference. Pediatr Crit Care Med (2015) 16(5):428–39. doi:10.1097/PCC.0000000000000350
95. Mok YH, Lee JH, Rehder KJ, Turner DA. Adjunctive treatments in pediatric acute respiratory distress syndrome. Expert Rev Respir Med (2014) 8(6):703–16. doi:10.1586/17476348.2014.948854
96. Meduri GU, Marik PE, Chrousos GP, Pastores SM, Arlt W, Beishuizen A, et al. Steroid treatment in ARDS: a critical appraisal of the ARDS network trial and the recent literature. Intensive Care Med (2008) 34(1):61–9. doi:10.1007/s00134-007-0933-3
97. Hubner M, Hochhaus G, Derendorf H. Comparative pharmacology, bioavailability, pharmacokinetics, and pharmacodynamics of inhaled glucocorticosteroids. Immunol Allergy Clin North Am (2005) 25(3):469–88. doi:10.1016/j.iac.2005.05.004
98. Murphy KD, Thomas S, Mlcak RP, Chinkes DL, Klein GL, Herndon DN. Effects of long-term oxandrolone administration in severely burned children. Surgery (2004) 136(2):219–24. doi:10.1016/j.surg.2004.04.022
99. Fan E, Dowdy DW, Colantuoni E, Mendez-Tellez PA, Sevransky JE, Shanholtz C, et al. Physical complications in acute lung injury survivors: a two-year longitudinal prospective study. Crit Care Med (2014) 42(4):849–59. doi:10.1097/CCM.0000000000000040
Keywords: acute respiratory distress syndrome, glucocorticoid treatment, mechanical ventilation, survival
Citation: Schwingshackl A and Meduri GU (2016) Rationale for Prolonged Glucocorticoid Use in Pediatric ARDS: What the Adults Can Teach Us. Front. Pediatr. 4:58. doi: 10.3389/fped.2016.00058
Received: 16 March 2016; Accepted: 23 May 2016;
Published: 14 June 2016
Edited by:
James Donald Fortenberry, Emory University School of Medicine, USAReviewed by:
Vijay Srinivasan, Children’s Hospital of Philadelphia, USASatoshi Nakagawa, National Center for Child Health and Development, Japan
Copyright: © 2016 Schwingshackl and Meduri. This is an open-access article distributed under the terms of the Creative Commons Attribution License (CC BY). The use, distribution or reproduction in other forums is permitted, provided the original author(s) or licensor are credited and that the original publication in this journal is cited, in accordance with accepted academic practice. No use, distribution or reproduction is permitted which does not comply with these terms.
*Correspondence: Gianfranco Umberto Meduri, Z21lZHVyaUB1dGhzYy5lZHU=