- Centre for Molecular Therapeutics, Australian Institute of Tropical Health and Medicine (AITHM), James Cook University, Cairns, QLD, Australia
Parasitic helminths secrete and excrete a vast array of molecules known to help skew or suppress the host’s immune response, thereby establishing a niche for sustained parasite maintenance. Indeed, the immunomodulatory potency of helminths is attributed mainly to excretory/secretory products (ESPs). The ESPs of helminths and the identified small molecules (SM) are reported to have diverse biological and pharmacological properties. The available literature reports only limited metabolites, and the identity of many metabolites remains unknown due to limitations in the identification protocols and helminth-specific compound libraries. Many metabolites are known to be involved in host-parasite interactions and pathogenicity. For example, fatty acids (e.g., stearic acid) detected in the infective stages of helminths are known to have a role in host interaction through facilitating successful penetration and migration inside the host. Moreover, excreted/secreted SM detected in helminth species are found to possess various biological properties, including anti-inflammatory activities, suggesting their potential in developing immunomodulatory drugs. For example, helminths-derived somatic tissue extracts and whole crude ESPs showed anti-inflammatory properties by inhibiting the secretion of proinflammatory cytokines from human peripheral blood mononuclear cells and suppressing the pathology in chemically-induced experimental mice model of colitis. Unlike bigger molecules like proteins, SM are ideal candidates for drug development since they are small structures, malleable, and lack immunogenicity. Future studies should strive toward identifying unknown SM and isolating the under-explored niche of helminth metabolites using the latest metabolomics technologies and associated software, which hold potential keys for finding new diagnostics and novel therapeutics.
Introduction
Helminths, the cause of neglected helminthiases, are comprised of two major phyla, namely nematodes (roundworms) and platyhelminths (flatworms) (Hotez et al., 2008). Nematodes include intestinal soil-transmitted helminths (STHs) such as roundworms (e.g., Ascaris lumbricoides), whipworms (e.g., Trichuris trichiura), hookworms (e.g., Ancylostoma spp., and Necator americanus), and tissue or intestinal nematode (e.g., Trichinella spp.); and the filarial worms that cause lymphatic filariasis and onchocerciasis. Platyhelminths include trematodes such as blood-flukes (schistosomes) and cestodes such as tapeworms that cause cysticercosis. These parasites have been associated with humans since they were hominids, with an intimate evolutionary relationship that harks back at least two million years, and they are thus considered “heirloom parasites” (Araujo et al., 2013).
Intestinal nematodes have long been recognized as a public health burden contributing to anaemia, digestive diseases, and stunted growth (Sanchez et al., 2014). The exact global statistics for STH infections and associated morbidity are still elusive due to the inadequacy of up-to-date epidemiological data and the non-specificity of clinical signs of STH infections (De Silva et al., 2003; Brooker, 2010). According to the World Health Organisation (WHO), more than 1.5 billion people (approximately 24% of the world’s population) are infected with STHs alone, mainly affecting sub-Saharan Africa, the Americas, China, and East Asia, with children being the primary victims (WHO, 2020). More than 267 million preschool-age children and 568 million school-age children live in parasite-endemic areas needing treatment and preventive interventions.
As per the Global Burden of Disease study conducted in 2010, chronic parasitic worm infections significantly contribute to disability-adjusted life years (DALYs) lost, causing health and economic losses to billions of people worldwide (Murray et al., 2012; Vos et al., 2012). As of 2010, STHs have caused 5.2 million DALYs, schistosomiasis (3.3 million), lymphatic filariasis (2.8 million), and onchocerciasis (0.5 million) (Murray et al., 2012). However, in 2017, the estimated global burden of STHs dropped to 1.9 million DALYS (Kyu et al., 2018). According to Montresor et al. (2020), more than one billion people worldwide are still affected by STHs (Montresor et al., 2020). Currently, only a few WHO-recommended anthelmintic drugs, such as albendazole, mebendazole, and ivermectin, are used to treat STH infections (WHO, 2020). Despite repeated deworming and mass-drug administration programs in many countries, individuals in endemic areas are susceptible to re-infection. No vaccine is available for any human helminthiasis, and its development has been confounded by the complex life cycles of parasitic worms. Moreover, we do not fully understand the cues that these parasites require from the host to trigger developmental changes during their complicated lifecycles.
It is indisputable that chronic parasitic helminth infections are a major human health burden, but at the same time, epidemiological evidence supports the “Old Friends” hypothesis, notably through the observation that the complete elimination of helminths from defined human populations is associated with an increased incidence of inflammatory diseases and immune dysfunction (Rook, 2012; Rook, 2013). Although this link has not yet established cause and effect, there is clear evidence that helminth infections suppress the development of some inflammatory conditions, which is discussed in later sections. The “Old Friends” hypothesis (Rook, 2007) describes the requirement for early exposure to organisms like STHs, microbes, and environmental factors to prime essential immune development during childhood and ensure the optimal balance between effector and regulatory arms of the immune system. These immunomodulatory strategies mean that individual parasites can colonise a host for many years while preventing the development of sterilising protective immunity and suppressing the onset of inappropriate immune responses to innocuous antigens (McSorley et al., 2011; McSorley et al., 2013). Indeed, hookworms are one of the most adept infectious agents at driving regulatory responses (Chazot et al., 1994). It is well-known that the incidence of inflammatory diseases, including inflammatory bowel disease (IBD), is highest in countries where endemic exposure to helminths is lowest (Weinstock and Elliott, 2009; Rook, 2012; Molodecky et al., 2012). Extensive studies show that as a population urbanises and exposure to helminths is reduced, an increase in the incidence of inflammatory disease is observed (Prideaux et al., 2012; Ahuja and Tandon, 2010). The implication of helminths in this process is documented in mass drug administration programs, where an increase in immune dysfunction disorders soon follows the removal of helminths (Flohr et al., 2006). In a study by Kabeerdoss et al. (2011) (Kabeerdoss et al., 2011) of Crohn’s disease patients in India, individuals depleted of hookworms were more likely to have Crohn’s disease than matched infected subjects. In South Africa, childhood exposure to helminths was shown to be protective against IBD (Chu et al., 2013), and in Papua New Guinea, exposure to intestinal parasites protected infected subjects against the development of allergic rhinitis (Blount et al., 2009). There are also small open-label studies showing disease improvement in adult IBD patients (e.g., Crohn’s disease) when treated with tolerable numbers of live helminths (e.g., human hookworm N. americanus infective larvae) (Croese et al., 2006; Helmby, 2015). Inspired by these beneficial health effects, many patients suffering from autoimmune and metabolic diseases, especially IBD, have resorted to an unregulated and risky self-treatment modality called helminth therapy propagated by the availability of helminths online (Zhang and Gems, 2021).
The key to the helminth’s ability to successfully evade the host immune system’s anti-helminth responses and establish a chronic infection while rendering protection to their host against various inflammatory conditions lies in their excretory/secretory products (ESPs) (Loukas et al., 2016; Eichenberger et al., 2018; Wangchuk et al., 2019). Helminths actively induce tolerance (both local and systemic) by producing ESPs that interact with the host immune system. The ESPs comprise proteins, peptides, organic acids, lipids, carbohydrates, and nucleic acids (Maizels et al., 2018). Some of these molecules are homologous to host molecules and can skew the host’s immune response by mimicking immunologically relevant proteins or miRNAs that target the host’s gene expression (Buck et al., 2014; Smyth et al., 2018). Other helminth-derived molecules are unique to the parasites and help them initiate tissue damage and establish infection (Periago and Bethony, 2012; Flynn et al., 2019). Such helminth-specific biomolecules have also been explored widely as biomarkers for developing diagnostics and targets for developing vaccines and drugs (Hamed et al., 2011; Ryan et al., 2020; Whitman et al., 2021). Numerous reviews (McSorley et al., 2013; Maizels and McSorley, 2016; Maizels et al., 2018; Zakeri et al., 2018; Ryan et al., 2020) have substantially discussed the nature and functions of many helminth-derived macromolecules, including secreted proteins, peptides, and extracellular vesicles. Recently, work on helminth-derived small molecules (SM) has gained traction (Bobardt et al., 2020). However, to our knowledge, there is no comprehensive review article on the SM components of helminths.
Small molecules are chemical compounds whose size/molecular weights are less than one kilodalton (kDa) (Makurvet, 2021). Herein, we have collected published information on SM (identified from helminths) and their immunomodulatory potential from PubMed, Scopus, MEDLINE Ovid, Google Scholar Databases, and journal articles and analysed their contents. To retrieve relevant published information, we used the keywords “small molecules,” “helminths small molecules,” “immunomodulatory,” “helminth-derived small molecules,” “host-parasite interaction,” “helminth metabolites,” “lipidomics,” “helminth therapy,” “metabolomics,” “targeted metabolomics,” “untargeted metabolomics,” “inflammation,” “inflammatory bowel diseases,” “autoimmune disease,” “inflammatory cytokines,” “anti-inflammatory,” “helminthiases,” “clinical trials,” “host-parasite interaction,” “biomarkers,” “down-regulation,” “up-regulation,” “excretory/secretory products,” and names of different helminth species.” We have conducted a narrative review of the available literature and presented SM identified from helminths and their reported biological activities, exploring their potential scope in diagnostics and therapeutics development.
Host-parasite interaction and immunomodulation
Parasitic helminths are believed to have coevolved with humans over millennia, continuously refining and improving their mechanisms to skew or suppress the host’s immune response to establish a sustained niche inside their host. They do so by secreting or excreting a vast array of molecules, including metabolites. Dendritic cells (DCs) respond to ESPs and also microbiome metabolites, consequently promoting the development of regulatory T cells (Treg), regulatory B cells (Breg), and T helper type 2 (Th2) cells and inhibiting Th1 and Th17 cell responses (Figure 1). For instance, Trichinella spiralis adult and muscle larva-derived ESPs activated bone marrow-derived dendritic cells enabled naïve mice to acquire Treg cells differentiation (Sun et al., 2019), suggesting that T. spiralis ESPs might stimulate differentiation of the host’s Treg cells via dendritic cells activation favoring parasite survival inside the host. Helminths residing in the human gut induce tolerogenic DCs, alternatively activated macrophages, and Treg cells, which produce suppressor cytokines that help keep inflammatory T cells and their effector molecules in check (Loukas et al., 2016). Helminths-induced type 2 immune response causes profound changes in the host tissue physiology, hyperplasia of goblet cells (Miller and Nawa, 1979), and hypercontractility of smooth muscles (Castro et al., 1976). Additionally, the innate lymphoid cell (ILC) family and T and B lymphocytes are involved in host defence, barrier integrity, and tissue homeostasis and repair (Artis and Spits, 2015). Alarmins such as thymic stromal lymphopoietin (TSLP), IL-33, and IL-25 released by tuft cells (Figure 1) and other epithelial cells following tissue damage act on granulocytes (mast cells, basophils, and eosinophils) and tissue-resident group 2 ILCs (ILCs-2), consequently enhancing the production of type 2 cytokines, including IL-13 (Grencis, 2015; von Moltke et al., 2016).
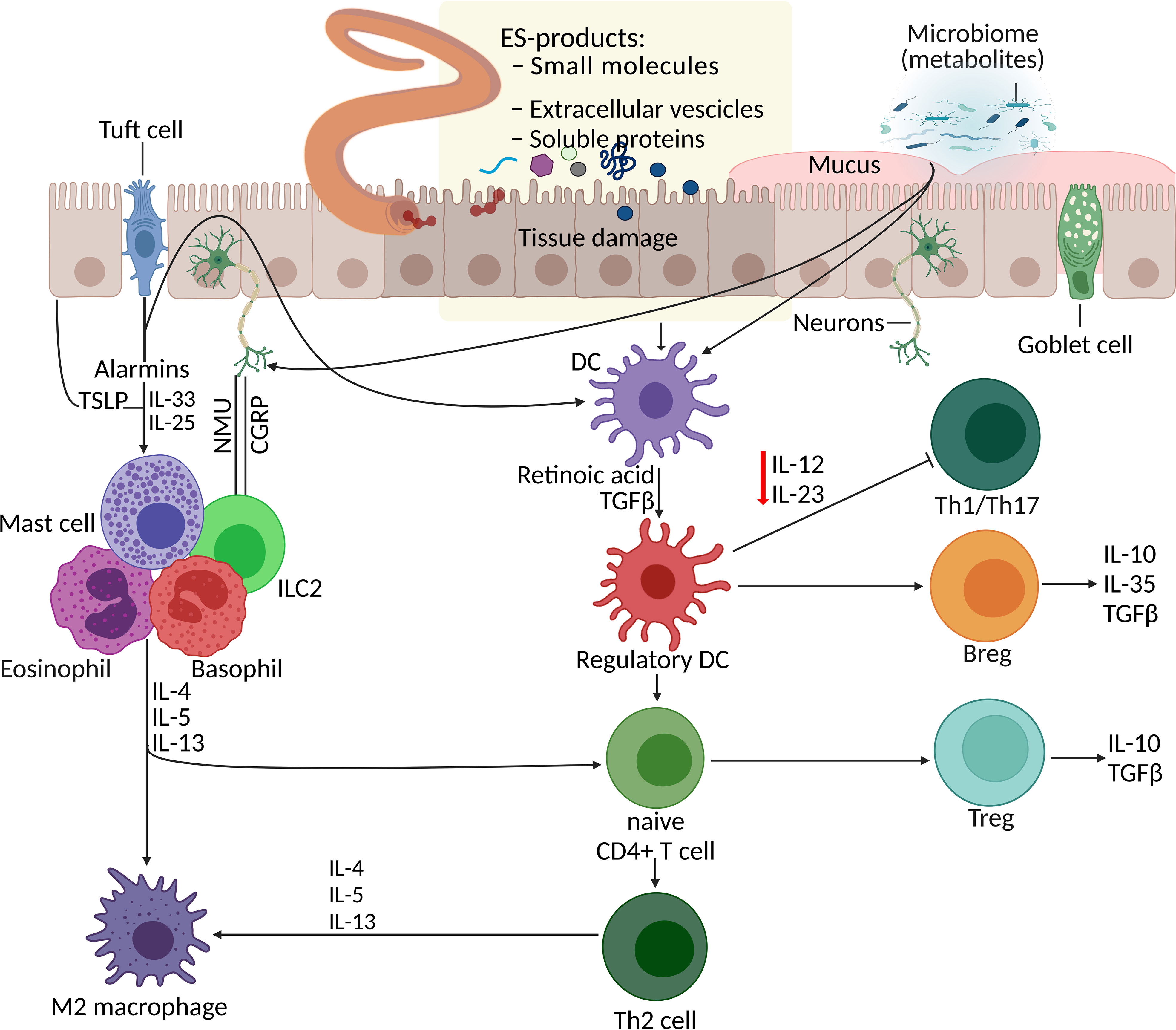
Figure 1 Parasite–host interaction: manipulation of the host immune system by helminth excretory/secretory products (ESPs). Helminth infection together with additional events and signals (such as worm ESPs, microbiota metabolites, and alarmins from tissue damage) initiate processes including the differentiation of Th2 cells, Treg expansion and responses, macrophage polarisation, and mucus production. DCs respond to various stimuli (alarmins, ESPs, and metabolites) and promote development of Treg, Breg, and Th2 cells, and inhibit Th1 and Th17 cell responses. Additionally, alarmins (TSLP, IL-33, IL-25) released by tuft cells and other epithelial cells following tissue damage, act on granulocytes (mast cells, basophils, and eosinophils) and ILC2s, consequently enhancing the production of type 2 cytokines. Gut sensory neurons also influence this immune network upon receiving signals from helminths and microbes by releasing neuropeptides (NMU- neuropeptide neuromedin U and CGRP-calcitonin gene-related peptide) which directly effects the ILC2 responses. APR, aspartic protease; Breg, regulatory B cell; CGRP, calcitonin gene-related protein; DC, dendritic cell; ESP, excretory/secretory product; GST, glutathione-S-transferase; ILC2, type 2 innate lymphoid cell; NMU, neuromedin U; serpin, serine protease inhibitor; Th2, T helper type 2, TLSP, thymic stromal lymphopoietin; Treg, regulatory T cell.
As helminths (both nematodes and platyhelminths) transition from their free-living stage to parasitism, they require long-lasting niches, including intermediate hosts, to complete their life cycle (Hotez et al., 2008). In the course of their journey, they either secrete or excrete many molecules that help them enter their host, invade tissues, shield them from the host’s immune assault and thus, establish chronic infections (reviewed elsewhere (Maizels et al., 1993; Maizels and Yazdanbakhsh, 2003; Dzik, 2006; Maizels and McSorley, 2016; Ryan et al., 2020)). For instance, skin is the first immune barrier Schistosoma mansoni has to pass through. Proteolytic factors, including various serine proteases, are reported to be responsible for skin penetration (Marikovsky et al., 1988; Chavez-Olortegu et al., 1992). Among many serine proteases, the 28/30kDa variant called SmCE (S. mansoni cercarial Elastase) is considered most crucial as inhibition (by inhibitor succinyl-alanyl-alanyl-prolyl-phenylalanine chloromethyl ketone, AAPF-CMK) of this protease reduces skin penetration up to 80% (Salter et al., 2000). After successful skin penetration, one of the mechanisms that favor the survival of lung stage schistosomula is using their surface-associated peroxiredoxin 1 to overcome oxidative stress scavenging surrounding hydrogen peroxide (Kumagai et al., 2009). Surface-bound enolases of schistosomula in both S. japonicum and S. mansoni are associated with a fibrinolytic activity which prevents clot formation in their vicinity (Figueiredo et al., 2015). Glycoproteins such as alpha-1 (also known as IL-4 inducing principle of S. mansoni eggs, IPSE) and omega-1 are known to involve in skewing T cell response in granulomas (Th2 type response) while migrating through the intestine towards the lumen (Everts et al., 2009; Haeberlein et al., 2017). The interaction mechanism with host tissues is complex and hard to dissect at the molecular level, partly due to the lack of suitable animal models (Loukas and Prociv, 2001), and the roles of many bigger molecules, particularly proteins, are yet to be understood. This review highlights known or potential roles of SM either secreted and/or excreted by various helminths in their life cycle stages, including while interacting with their respective hosts (Table 1). Octadecanoic acid secreted by the infective third-stage larvae (L3) of Nippostrongylus brasiliensis (a model organism for human hookworms) is known to help to lyse the host’s red blood cells, and similarly, in Haemonchus contortus (Ward, 1982; Yeshi et al., 2020). Another small molecule, succinic acid, identified from the ESP of adult N. brasiliensis, can induce intestinal tuft cells to initiate Th2 responses (Wangchuk et al., 2019). Succinic acid is thought to be energy source for helminths during adaptations toward anaerobiosis (Saz, 1981). Succinic acid is also detected as a dominant metabolite from the ESPs of adult-stage Trichuris muris (a model organism for the human-dwelling T. trichiura) (Wangchuk et al., 2019).
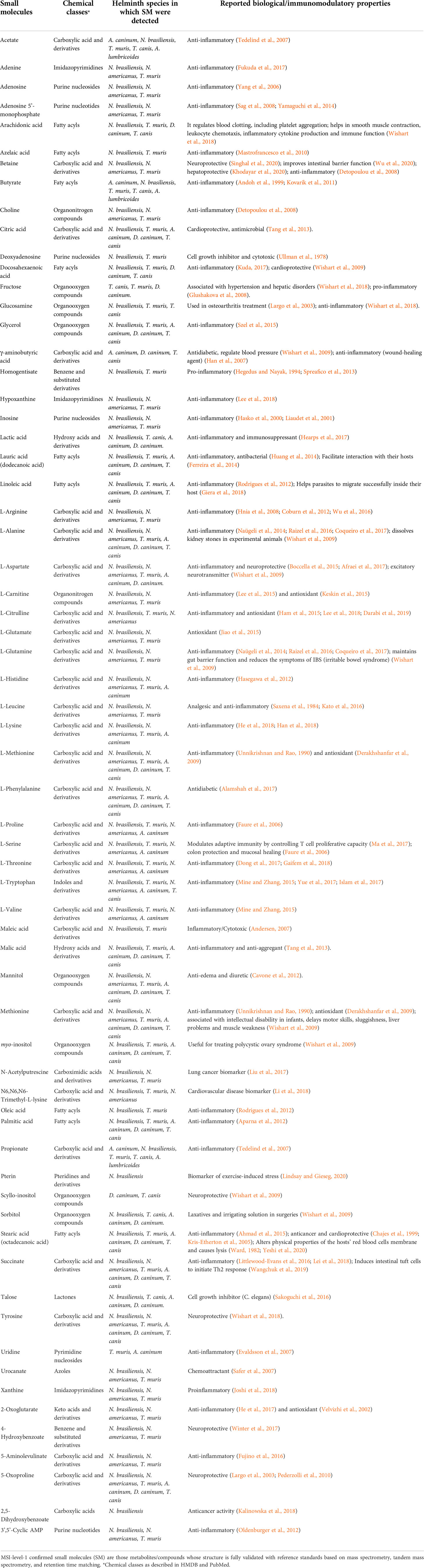
TABLE 1 Reported biological/immunomodulatory properties of MSI level 1 confirmed small molecules from helminths.
Old friends/hygiene hypothesis: evidence from helminth therapy
From an evolutionary viewpoint, the coevolution of humans and helminths may have shaped our metabolism and organ functions (Stilling et al., 2014). Infection with our ‘old friends’ – helminths – is believed to have shaped our immune system to facilitate co-existence (Rook et al., 2014; Versini et al., 2015; Longman and Littman, 2015; Filyk and Osborne, 2016), and there is evidence that helminths can protect us from a broad spectrum of immune-mediated inflammatory diseases (IMIDs). These changes to the immune system have often benefited hosts by reducing overall inflammation. Moreover, as per the hygiene hypothesis (first proposed by Strachan) (Strachan, 1989), reduced contact with infectious agents (particularly those that co-evolved with us) due to improved sanitation, lifestyle changes (including dietary habits) are attributed to the alarming rise in IMIDs, including metabolic syndrome (e.g., obesity, insulin resistance, hypertension, and atherogenic dyslipidemia) (Grundy et al., 2005; Huang, 2009; Rook et al., 2014). Several studies have reported that helminths promote the host’s gut health/influence microbiome composition (evident from their fecal microbiome) (Lee et al., 2014; Jenkins et al., 2017; Jenkins et al., 2018; Rosa et al., 2018; Schneeberger et al., 2018; Gordon et al., 2020; Mejia et al., 2020), including increased alpha diversity (multispecies-helminth infected individuals with greater diversity than single species-infected individuals). The influence on microbiome composition is found to be specific to helminth species and the host’s anatomical niche (e.g., observed strong influence by helminths in the large intestine such as Enterobius vermicularis and Trichuris trichiura). Studies have also shown that infection with various helminth species can reduce the severity of diseases, including chemically-induced colitis (Fox et al., 2000; Khan et al., 2002; Elliott et al., 2004; Moreels et al., 2004; Hunter et al., 2005) and high fat diet-induced obesity (Su et al., 2018; Su et al., 2020), and such protection is partially attributed to Th2-dependent, M2 macrophage-mediated change in the gut microbiome (Durack and Lynch, 2019; Su et al., 2020). For instance, targeted removal of the bacterial family Enterobacteriaceae reduced inflammation in mice (Zhu et al., 2018). Reviews done by Brussow (2019) (Brussow, 2020), Kupritz et al. (2021) (Kupritz et al., 2021), and Durack and Lynch (2019) have more detailed updates on the helminth-induced human gut microbiome composition and their benefits and therapeutic potential. The human gut microbiome is also said to be influenced by deworming (the clearance effect) and anthelmintic drugs (the treatment effect) (Kupritz et al., 2021). For instance, a study conducted in a rural Kenyan village observed that the clearance of STHs (e.g., A. lumbricoides and N. americanus) using albendazole therapy led to a significant increase in Clostridials and reduction in Enterobacteriales in the gut microbiome of participants when compared with their age-matched controls (Easton et al., 2019). A similar finding was also reported from the cross-sectional studies between Indonesia and Liberia, where both countries showed a significant association of 12 bacterial taxa with STH infections, including Olsenella (associated with reduced gut inflammation), which decreases in numbers after deworming (Rosa et al., 2018). It is difficult to discriminate the influence between the clearance and treatment effect, and further, the change in microbiome composition is mostly significant only in treated subjects who remain infected after the treatment (Martin et al., 2018; Martin et al., 2019). Perturbations in the gut microbiome (e.g., the imbalance between harmful and protective bacteria) or dysbiosis characterised by, for instance, reduced diversity of short-chain fatty acids (SCFAs) producers and enriched proinflammatory microbes (e.g., H2S producers) is said to be responsible for the pathogenesis of IBD, and it is reviewed elsewhere (Kaur et al., 2011; Sultan et al., 2021). For example, the lack of certain symbiotic species (e.g., Faecalibacterium prausnitzii) is said to be responsible for the recurrence of Crohn’s disease as mice supplemented with this organism experienced reduced inflammation after inducing colitis chemically (Sokol et al., 2008). A reduced amount of F. prausnitzii is also linked to relapse of Ulcerative colitis (Werkman et al., 2018). However, the causal effect of dysbiosis on IBD and other diseases is still inconclusive, as dysbiosis is even observed in the fecal samples from healthy relatives of IBD patients (Van de Merwe et al., 1988). There is no cure for IMIDs, and some patients, in desperation and inspired by the above theories, have turned to alternative treatments such as helminth therapy by deliberately self-infecting with live helminths. Only two helminth species, pig whipworm Trichuris suis ova (TSO) and the human hookworm N. americanus are used for human helminth therapy.
In recent years, helminth therapy has been assessed in early phase clinical trials using TSO and N. americanus infective larvae against inflammatory, autoimmune, and allergic diseases (Summers, 2003; Croese et al., 2006; Blount et al., 2009), yielding both positive and negative outcomes. IBD patients (both Crohn’s disease and ulcerative colitis), when given TSO (single dose) in open-label phase I trials, not only tolerated the treatment but also achieved remission, and benefits were maintained through repeated doses (Summers, 2003; Summers et al., 2005). However, in a double-blind, placebo-controlled phase II trial of TSO in adults with active Crohn’s disease (n = 252), ingestion of 250-7500 TSO fortnightly over 12 weeks was observed to be safe without any adverse effects, but the treatment did not have an advantage over the placebo in inducing clinical remission and other immunological responses (Scholmerich et al., 2017). Moreover, the study failed to reach its clinical endpoint for both active Crohn’s disease (Scholmerich et al., 2017) and multiple sclerosis (Voldsgaard et al., 2015; Fleming et al., 2019). A meta-analysis on TSO therapy trials (Huang et al., 2018) found that the treatment did not show statistically significant benefits for IBD and suggested the need for further studies with larger sample sizes. One of the challenges of using TSO is that frequent dosing is required for human trials as T. suis is not adapted to develop in humans and is rapidly expelled. On the contrary, N. americanus is a human hookworm, and it can survive within the human body for years. Crohn’s disease patients (a few already in remission before the trial started) tolerated a low dose of N. americanus larvae during a phase I open label trial, where larvae were administered percutaneously (Croese et al., 2006). Even at a lower dose (initial 10 larvae, followed by a booster dose of 5 larvae at week 12), N. americanus larvae was partially effective over placebo when tested in 20 HLA-DQ2+ celiac disease patients (autoimmune response to dietary gluten) followed by incremental wheat challenge after 20 weeks. Patients not only tolerated the treatment, but their duodenal biopsies showed reduced inflammatory cytokines such as IFN-γ and IL-17 (McSorley et al., 2011); however, gluten challenge did not yield any difference in the clinical parameters between the treated and placebo groups (Daveson et al., 2011). In a recent larger randomised controlled trial with the increasing exposure of celiac disease patients to gluten (Croese et al., 2020), N. americanus larvae could not restore tolerance to sustained moderate gluten consumption (2 g/day), but hookworm-infected patients did experience reduced symptoms when gluten was given intermittently at a lower dose. In summary, there are numerous clinical trials involving live helminths, but they remain inconclusive (Elliott and Weinstock, 2017; Ryan et al., 2020). Such drawbacks could be due to difficulty controlling the quality and innocuity of the infectious agent, low doses of worms that are insufficient to achieve required immunomodulation, logistical issues with scale-up, patient compliance to the treatments, and inability to define good manufacturing standards. Moreover, persistent infection can cause various health problems, including anaemia, malnutrition, and cognitive retardation (Albonico et al., 2008), and can sometimes be fatal. For instance, Kradin et al. (2006) found sexually mature male trichurid in the cecum of pediatric Crohn’s disease patients treated with T. suis ova (Kradin et al., 2006). Furthermore, the pathogenicity of Campylobacter jejuni may increase in patients receiving T. suis ova treatment (Shin et al., 2004). These complications and challenges necessitate the investigation of safer molecules to develop alternative therapeutics.
In pursuit of a more palatable treatment approach, recent focus has been on ESPs of helminths, constantly secreted by adult worms into the tissues of infected hosts in their various final niches (Loukas et al., 2016; Eichenberger et al., 2018; Wangchuk et al., 2019). Studies have identified several recombinant ESP proteins that can protect mice against inflammation and weight loss in two different mouse models of colitis – the T cell-dependent TNBS (2,4,6-Trinitrobenzene sulfonic acid) model (Ruyssers et al., 2008; Buitrago et al., 2021) and the T cell-independent DSS (dextran sodium sulfate) model (Ferreira et al., 2013; Ryan et al., 2020; Wang et al., 2020). Trichinella spiralis 53 kDa glycoprotein (Tsp53) is anti-inflammatory (induces production of IL-4 and IL-10 in T cells) (Cvetkovic et al., 2016), and its recombinant form rTsp53 confer protection in TNBS-induced colitis in mice (Du et al., 2011). Moreover, rTsp53 can downregulate proinflammatory cytokines and increase IL-10 and TGF-β1 levels via inducing macrophage polarisation (M1 to M2) (Chen et al., 2016). Other T. spiralis-derived molecules, including cystatin-like protein (Ts-CLP) (Liu et al., 2014; Tang et al., 2015), calreticulin (Ts-CRT) (Zhao et al., 2017), cystatin (TsCstN) (Kobpornchai et al., 2020), glutathione-S-transferase (TsGST) (Jin et al., 2019), and paramyosin (Ts-Pmy) (Sun et al., 2015; Guo et al., 2016) are known to possess anti-inflammatory and immunomodulatory properties. The main immunomodulatory mechanism shown by these proteins is upregulating Treg cells to produce more IL-10 and TGF-β, thus inhibiting Th1 and Th17-mediated inflammation.
Reported biological/immunomodulatory properties of helminth-derived small molecules
Due to their pathogenic and infectious nature, parasitic helminths can produce a wide array of immunomodulatory molecules, including proteins, glycans, nucleic acids, lipids, and SM (Maizels et al., 2018; Kahl et al., 2018). Wangchuk et al. (Wangchuk et al., 2019) reported that crude ESPs from A. caninum could suppress colitis pathology in a mouse model and further inhibit the secretion of inflammatory cytokines in primary human leukocytes. Ferreira et al. (Ferreira et al., 2013) also reported a similar reduced inflammatory response after administration of A. caninum crude ESPs in a murine colitis model by inducing a type 2 cytokine response. Therefore, we have reviewed bioactivities associated with metabolites (both polar and non-polar) identified/isolated from helminths, including literature describing metabolites’ role in the development of diagnostics/biomarkers and therapeutics. Polar metabolites, including carbohydrates, amino acids, nucleic acids, and organic acids, are hydrophilic. While non-polar metabolites, mainly lipids, are hydrophobic.
From the literature review of helminth-derived SM reported so far, about 65 were associated with various biological activities such as anti-inflammatory and antioxidant, anti-microbial, anticancer, anti-diabetic, cardio-, hepato-, and neuro-protective, and maintaining gut-barrier integrity (Table 1). These 65 helminth-derived compounds were identified using metabolomics standard initiative level I identification protocols, the highest identification level achieved using standards. Most of these compounds (45 metabolites) are associated with anti-inflammatory and antioxidant properties, suggesting roles in active immune regulation (Figure 2). For example, major metabolites detected in the infective stages of T. muris and N. brasiliensis, such as adenine, adenosine, betaine, and choline, possess anti-inflammatory properties (see Table 1). Betaine and other amino acids such as glutamine and serine are known to help maintain colon homeostasis (Faure et al., 2006; Wishart et al., 2009; Wu et al., 2020). The amino acid L-serine produced by N. americanus, N. brasiliensis, A. caninum, and T. muris has been shown to modulate adaptive immunity by controlling T cell proliferative capacity (Ma et al., 2017) and is involved in colon protection and mucosal healing (Faure et al., 2006). L-arginine in intestinal ischemia animal models aids mucosal healing by reducing tissue damage (Fotiadis et al., 2016), and a similar protective effect of L-arginine was also reported in LPS (bacteria-derived lipopolysaccharide)-challenged weaned pigs (Zhu et al., 2013). Moreover, this amino acid was reported from infective stages of N. americanus, N. brasiliensis, and T. muris (Yeshi et al., 2020; Wangchuk et al., 2021).
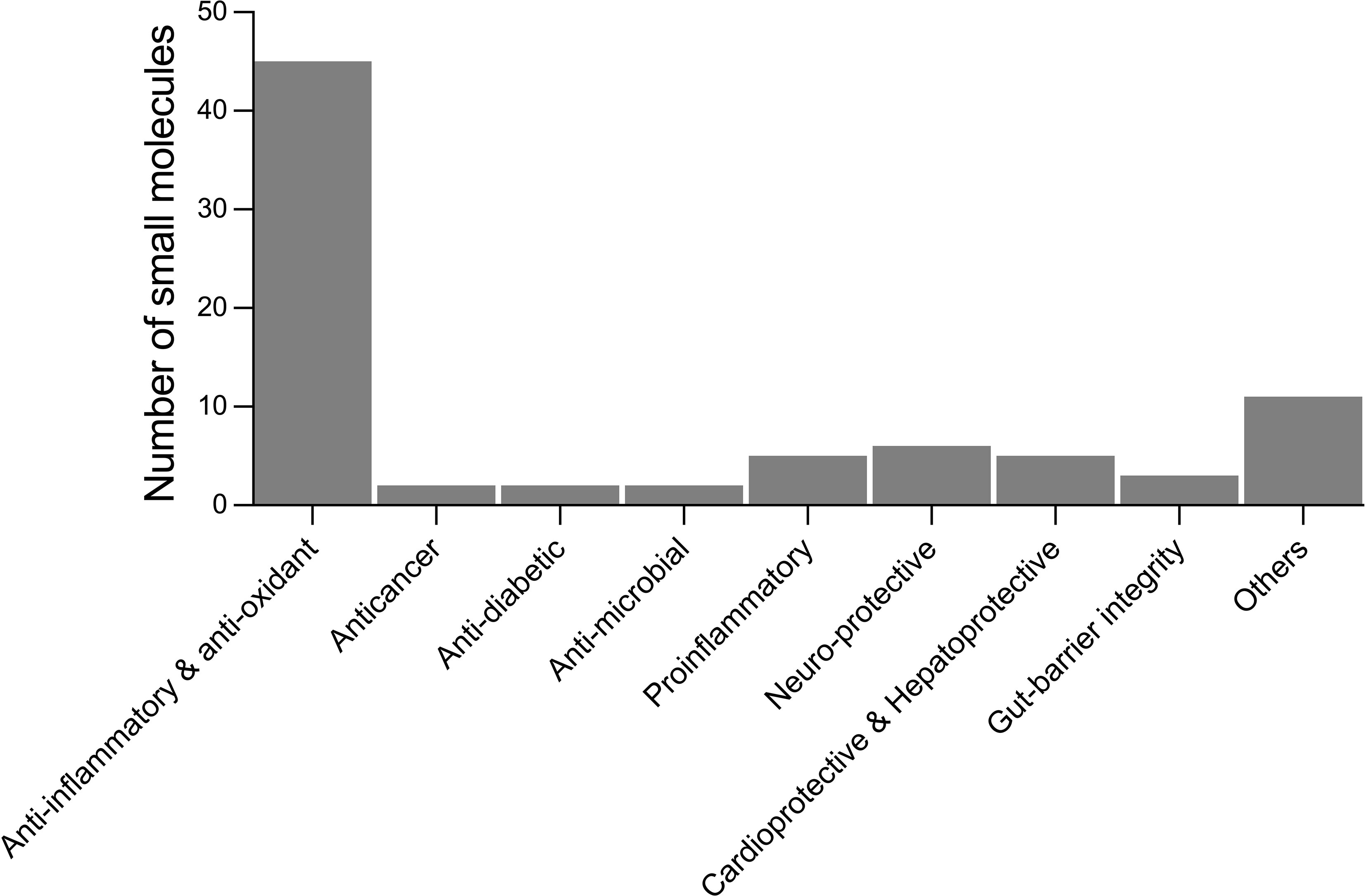
Figure 2 Number of small molecules identified in helminths with reported biological activities from the literature. Others include analgesics, laxatives, anti-aggregant, immuno-modulatory, cell-growth inhibitors, regulate blood clotting, treatment of osteoarthritis, polycystic ovary syndrome, and disease biomarkers.
Lipid utilization by helminths varies as they develop from free-living to the parasitic stage, and considerable changes occur, including lipid abundance and composition (Wang et al., 2020) (e.g., decreased synthesis of triradylglycerols but increased glycerophospholipids (Phosphatidylcholine, PC and phosphatidylethanolamine, PE in Haemonchus contortus)) (Wang et al., 2018), energy metabolism (Harder, 2016; Mangmee et al., 2020) and membrane fatty acids (FAs) composition (Proudfoot et al., 1990). For instance, the free-living stage requires more unsaturated FAs in the cell membrane to protect against the effects of low environmental temperatures, but the demand for FAs would be less as they develop to the parasitic stage (Hazel and Williams, 1990; Wang et al., 2018; Mangmee et al., 2020; Wang et al., 2020). Moreover, unlike the free-living infective stage, adults (parasitic stage) metabolise exogenous substrate, relying less on endogenous food stores (Barrett, 1968). In that way, adults use only a few specific FAs and fat-soluble vitamins as energy (Tielens, 1997; Dalton et al., 2003), and many lipids are eventually stored and excreted (Cheng, 1986) or become food for gametes (Barrett, 1968). We assumed that SCFAs such as propionate and acetate in the ESPs of adult A. caninum, N. brasiliensis, and T. muris might be stored as food reserves for their gametes. Fatty acids also play an essential role in diverse biological processes, and most importantly, FAs such as the cis-form of octadecanoic acid (stearic acid), monoenoic acids (oleic acid and vaccenic acid), and other branched-chain acids are known to help in breaking through the host cell membrane (Ward, 1982). Probably, the presence of stearic acid and oleic acid in the ESPs or somatic tissues of various helminth species, including Brugia malayi, Dipylidium caninum, Dictyocaulus viviparus, N. brasiliensis, Strongyloides ratti, Toxocara canis, and T. muris (Smith et al., 1996; Ferreira et al., 2014; Becker et al., 2017; Wangchuk et al., 2019; Wangchuk et al., 2019; Wangchuk et al., 2020; Yeshi et al., 2020) suggest that these worms might be producing compounds that could help them in invasion and establishing infection in their respective host(s) (Yeshi et al., 2020). Lipids are also known to have important functions in signalling transition. Endogenous steroidal hormones, such as dafachronic acid, have been demonstrated to play essential roles in regulating larval development in parasitic nematodes (e.g., in Ancylostoma ceylanicum, Strongyloides stercolis, and Haemonchus contortus) (Wang et al., 2009; Stoltzfus et al., 2012; Zhi et al., 2012; Albarqi et al., 2016; Ma et al., 2019). Other key nematode species, including Ascaris suum and Toxocara canis also produce dafachronic acid (Ma et al., 2019).
Zaiss et al. (2015) reported the immunoregulatory role of an increase in the concentration of bacterial-derived SCFAs induced by the Heligmosomoides polygyrus bakeri infection in mice with allergic inflammation (Zaiss et al., 2015). The same study also observed the increased SCFAs in the host-parasite setting by analysing colon contents of pigs infected with Ascaris suum eggs and stools from human volunteers infected with N. americanus infective larvae (Zaiss et al., 2015). Thus, the presence of SCFAs like propionate and acetate in A. caninum, N. brasiliensis, T. muris, T. canis, and A. lumbricoides might have immuno-modulatory roles in their host. Short-chain fatty acids derived from other species (not worms) also possess anti-inflammatory properties (Andoh et al., 1999; Tedelind et al., 2007; Kovarik et al., 2011), and they are known to promote gut health. Phosphatidylcholines (PCs), reported as major lipids in S. mansoni (Ferreira et al., 2015), are also said to have similar functions. For instance, a glycoprotein ES-62 secreted by Acanthocheilonema viteae can protect against rheumatoid arthritis, systemic lupus erythematosus, and airway hyper-responsiveness is mainly attributed to the covalently-bonded phosphorylcholine moieties (Pineda et al., 2014; Pineda et al., 2015). However, ES-62 did not protect against autoimmune diseases like type I diabetes, multiple sclerosis, and IBD (Doonan et al., 2018). Some schistosome-derived PCs are also responsible for pathogenesis. For instance, when toll-like receptor-2 knockout (TLR2−/−) mice were given S. mansoni-derived lysophosphatidylcholine (lyso-PC), it induced eosinophil recruitment and cytokine production in a TLR2-dependent manner, suggesting that this mechanism might contribute to the pathogenesis and lethality, particularly during the chronic phase of schistosome infection (Magalhaes et al., 2010). Nadjsombati et al. (Nadjsombati et al., 2018) showed that succinate (one of the metabolites secreted/excreted by a few helminth species) given ad libitum in the drinking water could induce a Th2 immune response in the mouse intestine through the tuft cell-ILC2 circuit, which could activate its receptor SUCNR1 to initiate succinate-induced IL-13 production, which is responsible for initiating Th2 responses.
When Giera et al. (Giera et al., 2018) analyzed the lipid composition of different life cycle stages of S. mansoni, eggs were found enriched with prostaglandins, while the cercaria stage contains mainly resolvins. However, Salafsky et al. (Salafsky et al., 1984) proposed that prostaglandins released by cercariae into the skin after initiating penetration upon stimulation by host skin essential fatty acids might further promote penetration via releasing histamine. Resolvins are well known for their anti-inflammatory properties (reviewed by Moro et al. (Moro et al., 2016)) as resolvin E1 protects liver fibrosis in mice (pathogen-free Kunming mice) infected with S. japonicum (Qiu et al., 2014). These findings suggest that prostaglandins and resolvins might be involved in the host immune adjustment.
Potential application of small biomarker molecules in diagnostics
Currently, there is no 100% sensitive diagnostic tool for detecting STHs infections. The WHO-recommended gold standard microscopy-based techniques (including Kato-Katz thick smear, McMaster, and flotation, which enable to count/detect parasite eggs in a fecal sample) (Khurana et al., 2021) are not adequately sensitive in low-endemic and near-elimination areas (McCarthy et al., 2012; Werkman et al., 2018). Moreover, diagnostic assays commonly used for detecting many STHs infections are considered invasive and risky as they involve collecting samples such as body fluids (e.g., stool and blood) and tissues through biopsy, requiring both special equipment and specialist, which is often a big challenge in developing countries. There has been continued effort toward developing more sensitive, low-cost, and easy-to-operate molecular diagnostics, which can be used in both endemic and low-endemicity settings, and this topic is reviewed elsewhere (Ullah et al., 2020; Khurana et al., 2021). Recent findings about the immunomodulatory roles of helminth-derived ESPs, including proteins, microRNAs, and extracellular vesicles (Hoy et al., 2014; Kammoun-Rebai et al., 2016; Eichenberger et al., 2018; Bi et al., 2021), is a paradigm shift in diagnosis/prognosis of helminthiasis and more about proteins/protein microarrays, microRNAs, and extracellular vesicles are available in these reviews (Sotillo et al., 2020; Li et al., 2021; Mu et al., 2021). An alternative potential area of diagnostic development is small biomarker molecules. Recent advances in metabolomics techniques and technologies have enabled the identification of small biomarker molecules of infections caused by helminths. Comparative analysis of metabolites profiles in samples obtained from infected and non-infected individuals/animals can be used to identify diagnostic biomarkers. For instance, Denery et al. (2010) (Denery et al., 2010) identified 14 potential candidate metabolites biomarkers, including long-chain fatty acids hexacosenoic acid and pentacosenoic acid in the plasma and serum obtained from onchocerciasis positive human volunteers from African countries. Another example of a urine biomarker is 2-methyl pentanoyl carnitine (2-MPC), and 2-MPC could be used as A. lumbricoides-specific urine biomarker (Table 2) since it gives high accuracy for detecting both infection and infection intensity (Lagatie et al., 2020). Moreover, the 2-MPC level in urine decreased significantly when infected individuals were given a single albendazole dose (400 mg) (Lagatie et al., 2020).
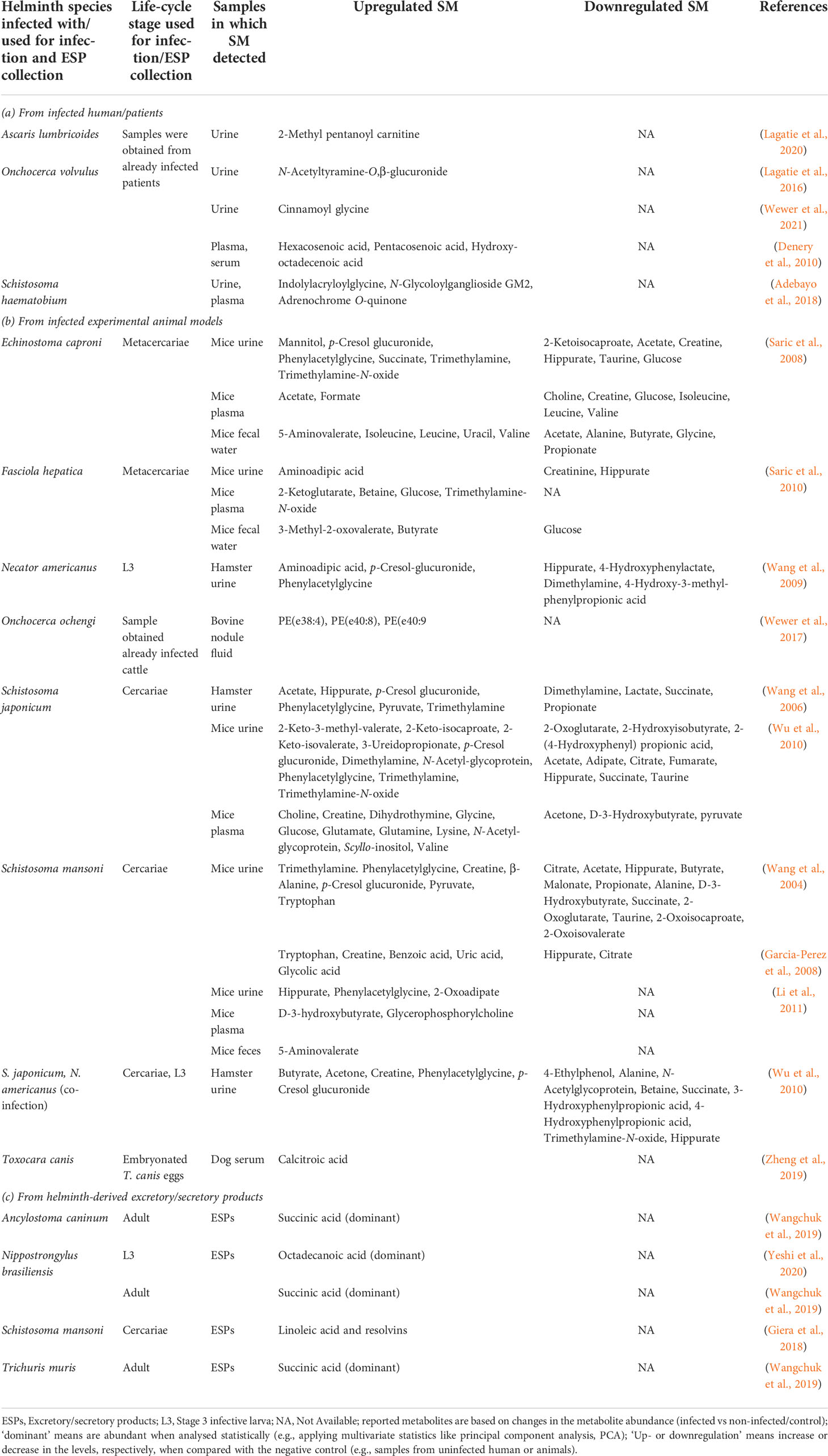
Table 2 Reported small molecules as potential diagnostic biomarkers in samples obtained from human and animals.
Due to challenges in biomarkers from human host-parasite interactions, focus has been diverted to study this phenomenon in animal models. There are variations in the metabolite profiles in the samples obtained from animals infected with different helminth species (Table 2). For example, urine samples from S. mansoni-infected mice showed decreased levels of SCFAs such as acetate, butyrate, and propionate (Wang et al., 2004) but urine samples from S. japonicum-infected and S. japonicum and N. americanus co-infected hamster had increased levels of acetate and butyrate, and reduction in propionate (Wang et al., 2006; Wu et al., 2010) (Table 2). Such fluctuations in the SM profile could be due to the type of helminth species used for infection or duration of infection and animal models used. Some of the commonly detected increased levels of SM in samples from infected animals are microbial-related metabolites such as p-cresol glucuronide, trimethylamine, and phenylacetylglycine (Table 2). The metabolic product of Clostridium difficile (opportunistic mild pathogenic anaerobe), p-cresol, is known to be the source of p-cresol glucuronide (Selmer and Andrei, 2001). Thus, elevation in such metabolites/SM could be related to the impact of infection on gut-microbial homeostasis. Another secretory product of O. volvulus as a potential urine biomarker for river blindness is N-acetyltyramine-O,β-glucuronide (NATOG) (Globisch et al., 2013). However, NATOG alone is inadequate to discriminate between infected and control (Lagatie et al., 2016; Hotterbeekx et al., 2020), whereby considering the level of additional urine metabolites like cinnamoyl glycine can enhance the onchocerciasis assessment (Wewer et al., 2021).
Wewer et al. (2017) (Wewer et al., 2017) reported ether-linked PC and PE in Onchocerca volvulus, O. ochengi, and Litomosoides sigmodontis through targeted shotgun lipidomics. Their study could not detect parasite-specific lipids in the infected bovine’s (host) serum, but the fluid taken from O. ochengi nodules from the host contained nematode-derived ether-linked PE species [e.g., PE(e38:4), PE(e40:8), and PE(e40:9)], which could be potentially used to develop diagnostic biomarkers for river blindness (Wewer et al., 2017). Adebayo et al. (2018) (Adebayo et al., 2018) reported few putative urine metabolites (e.g., N-Glycoloylganglioside, adrenochrome, 3-succinoylpyridine, 1-Nitro-5,6-dihydroxy-dihydronaphthalene) from individuals with urogenital schistosomiasis (caused by S. haematobium) associated with bladder pathology and are worth further analysis (including MSI level 1 identification) to be used as potential biomarkers. Oxidized pterins (e.g., neopterin) are used as urine markers (e.g., for hyperphenylalaninemia) (Longo, 2009). Pterin was detected in the ESPs of the infective L3 of N. brasiliensis (Yeshi et al., 2020). Considering these examples of potential small biomarker molecules and those mentioned in Table 2, investigating other neglected helminthiases may lead to discovering more unique biomarkers to better understand the helminth infections.
Potential application of small molecules in immunomodulatory drugs
Several small molecule immunomodulatory drugs (IMiDs) (e.g., aminosalicylates, azathioprine, 6-mercaptopurine, and methotrexate) have been historically used for the management of inflammatory conditions, including IBD (Yeshi et al., 2020). Recent studies demonstrated that hookworm-derived SM extract could suppress pathology in TNBS-induced colitis and inhibit the secretion of key inflammatory cytokines from human peripheral blood mononuclear cells (Wangchuk et al., 2019; Wangchuk et al., 2019). Excretory/secretory products from other helminth species also showed similar protection in various experimental colitis models (Ruyssers et al., 2008; Varyani et al., 2017; Bobardt et al., 2020; Wang et al., 2020). Small molecule analogs of Acanthocheilonema viteae secreted immunomodulatory ESP ES-62, namely ES-62-SMAs 11a and 12b, protected ovalbumin-induced Th2 response-mediated airway inflammation and lungs eosinophil infiltration in mice suggesting potential drug leads to allergies (Rzepecka et al., 2014). Dafachronic acid detected in a few species of parasitic helminths (A. ceylanicum, A. suum, S. stercolis, H. contortus, and T. canis) is another example of a small molecule with therapeutic potential. When methylprednisolone acetated-treated NSG mice undergoing hyperinfection with S. stercolis were administered 10 μM dafachronic acid orally with drinking water, it significantly reduced the worm burden in mice (Patton et al., 2018; Wang et al., 2021). Although saturated fatty acids such as palmitic acid and stearic acid were detected in all bioactive extracts, SCFAs such as acetate, propionate, and butyrate could be responsible for the observed protection (Wangchuk et al., 2019) as Tedelind et al. (2007) have reported anti-inflammatory properties associated to these SCFAs (Tedelind et al., 2007). Dodecanoic acid produced by the miracidium of S. mansoni is involved in host-parasite interactions, including penetration into its intermediate host (snail) (Ferreira et al., 2014; Wangchuk et al., 2021). Linoleic acid produced by cercariae of S. mansoni, and prostaglandins (PGE2) enriched in their eggs helps cercariae to migrate successfully inside their human hosts (Ramaswamy et al., 2000; Giera et al., 2018). Thus, with many helminth-derived SM showing various immunomodulatory/biological properties, including anti-inflammatory (Table 1), it has the potential to spur novel anti-inflammatory drug and IMiDs development.
Unlike bigger molecules like proteins (biologics), SM are considered ideal candidates for drug development since they are small structures, are stable, cheaper to scale up, and lack immunogenicity (Yeshi et al., 2020). Moreover, unlike biologics that typically require parenteral delivery, SM can be developed into oral dosage forms, boosting patient satisfaction and compliance (Olivera et al., 2017). A search for SM in the innovative therapeutic design and development is still in the picture, and as of 2012, the Chemical Universe Database GDB-17 has recorded 166 billion SM (Ruddigkeit et al., 2012). Moreover, recently, a Sweden-based biopharmaceutical company, Nuevolution, has revolutionized drug discovery by creating a DNA-encoded library of 40 trillion unique SM (Makurvet, 2021). Such a library with DNA-encoded SM has enabled rapid evaluation and identification of bioactive drug-like compounds (Kleiner et al., 2011; Kunig et al., 2018). For example, a benzoxazepinone inhibitor discovered from a DNA-encoded library has become a clinical candidate that can potentially treat multiple inflammatory conditions (Harris et al., 2017). Therefore, there is an urgent need to enhance efforts to analyse helminth ESPs for bioactive molecules and establishing a helminth-specific DNA-encoded SM library would help revolutionize the development of novel therapeutics for neglected helminthiases.
Conclusion
Recent studies have reported the protective role of small molecule crude extract obtained from helminth ES products against allergic and various autoimmune diseases, including chemically-induced colitis experimental mice models. Focusing on isolating compounds from such bioactive crude ES products of helminths would likely result in discovering novel drug leads, especially against inflammatory diseases. Limited known SM (e.g., amino acids and SCFAs) were reported from many helminths, and these molecules are associated with various biological and pharmacological activities. The available literature reports only limited known metabolites present in the crude extracts, and the identity of many metabolites remain unknown due to limitations in the identification protocols and helminth-specific compound libraries. Many of these unknown metabolites, particularly lipids that remain largely unstudied, may likely have interesting biological and pharmacological properties, which could facilitate the development of novel diagnostic and therapeutic products.
There is a real need to pursue extensive and comprehensive metabolomics studies to identify such unknown compounds. With the rapid improvement in metabolomics platforms such as high-resolution mass spectrometry (HRMS) and other associated software tools and growing databases, it is expected to accelerate the identification of many novel immunomodulatory SM produced by helminths. For example, a new pulsed mass-spectrometry ion generation technique called triboelectric nanogenerator inductive nano-electrospray ionization (TENGi nanoESI) mass spectrometry, introduced in 2020 could analyse volume-limited samples even at nanolitre scale. Such technological advancement is likely to help popularise immuno-metabolomics studies of helminths, where obtaining even modest quantities of ESPs is challenging without a good rodent model. Furthermore, future studies should prioritize those parasitic helminths not studied so far and develop a small molecule database (i.e., helminth-specific) to allow researchers to dive deep into the treasure trove of helminth metabolites.
Author contributions
All authors contributed to the article and approved the submitted version.
Funding
This work was funded by a James Cook University Postgraduate Research Scholarship (JCUPRS) to KY, the National Health and Medical Research Council (NHMRC) Ideas Grant (APP1183323) to PW, and NHMRC Program Grant (APP1132975) and a Senior Principal Research Fellowship (APP1117504) to AL.
Conflict of interest
The authors declare that the research was conducted in the absence of any commercial or financial relationships that could be construed as a potential conflict of interest.
Publisher’s note
All claims expressed in this article are solely those of the authors and do not necessarily represent those of their affiliated organizations, or those of the publisher, the editors and the reviewers. Any product that may be evaluated in this article, or claim that may be made by its manufacturer, is not guaranteed or endorsed by the publisher.
References
Adebayo A. S., Mundhe S. D., Awobode H. O., Onile O. S., Agunloye A. M., Isokpehi R. D., et al. (2018). Metabolite profiling for biomarkers in Schistosoma haematobium infection and associated bladder pathologies. PloS Negl. Trop. Dis. 12 (4), 1–17. doi: 10.1371/journal.pntd.0006452
Afraei S., D'Aniello A., Sedaghat R., Ekhtiari P., Azizi G., Tabrizian N., et al. (2017). Therapeutic effects of d-aspartate in a mouse model of multiple sclerosis. J. Food Drug Anal. 25 (3), 699–708. doi: 10.1016/j.jfda.2016.10.025
Ahmad M., Baba W. N., Gani A., Wani T. A., Gani A., Masoodi F. A., et al. (2015). Effect of extraction time on antioxidants and bioactive volatile components of green tea (Camellia sinensis), using GC/MS. Cogent Food Agric. 1 (1), 1–11. doi: 10.1080/23311932.2015.1106387
Ahuja V., Tandon R. K. (2010). Inflammatory bowel disease in the Asia-pacific area: a comparison with developed countries and regional differences. J. Dig Dis. 11 (3), 134–147. doi: 10.1111/j.1751-2980.2010.00429.x
Alamshah A., Spreckley E., Norton M., Kinsey-Jones J. S., Amin A., Ramgulam A., et al. (2017). L-phenylalanine modulates gut hormone release and glucose tolerance, and suppresses food intake through the calcium-sensing receptor in rodents. Int. J. Obes. 41 (11), 1693–1701. doi: 10.1038/ijo.2017.164
Albarqi M. M., Stoltzfus J. D., Pilgrim A. A., Nolan T. J., Wang Z., Kliewer S. A., et al. (2016). Regulation of life cycle checkpoints and developmental activation of infective larvae in Strongyloides stercoralis by dafachronic acid. PloS Pathog. 12 (1), 1–20. doi: 10.1371/journal.ppat.1005358
Albonico M., Allen H., Chitsulo L., Engels D., Gabrielli A. F., Savioli L. (2008). Controlling soil-transmitted helminthiasis in pre-school-age children through preventive chemotherapy. PloS Negl. Trop. Dis. 2 (3), 1–11. doi: 10.1371/journal.pntd.0000126
Andersen F. A. (2007). Final report on the safety assessment of maleic acid. Int. J. Toxicol. 26, 125–130. doi: 10.1080/10915810701351251
Andoh A., Bamba T., Sasaki M. (1999). Physiological and anti-inflammatory roles of dietary fiber and butyrate in intestinal functions. J. Parenter Enteral Nutr. 23 (5), S70–SS3. doi: 10.1177/014860719902300518
Aparna V., Dileep K. V., Mandal P. K., Karthe P., Sadasivan C., Haridas M. (2012). Anti-inflammatory property of n-hexadecanoic acid: Structural evidence and kinetic assessment. Chem. Biol. Drug Des. 80 (3), 434–439. doi: 10.1111/j.1747-0285.2012.01418.x
Araujo A., Reinhard K., Ferreira L. F., Pucu E., Chieffi P. P. (2013). Paleoparasitology: The origin of human parasites. Arq Neuropsiquiatr 71 (9B), 722–726. doi: 10.1590/0004-282X20130159
Artis D., Spits H. (2015). The biology of innate lymphoid cells. Nature. 517 (7534), 293–301. doi: 10.1038/nature14189
Barrett J. (1968). Lipids of the infective and parasitic stages of some nematodes. Nature. 218, 1267–1268. doi: 10.1038/2181267a0
Becker A.-C., Willenberg I., Springer A., Schebb N. H., Steinberg P., Strube C. (2017). Fatty acid composition of free-living and parasitic stages of the bovine lungworm dictyocaulus viviparus. Mol. Biochem. Parasitol. 216, 39–44. doi: 10.1016/j.molbiopara.2017.06.008
Bi N. N., Zhao S., Zhang J. F., Cheng Y., Zuo C. Y., Yang G. L., et al. (2021). Proteomics investigations of potential protein biomarkers in sera of rabbits infected with schistosoma japonicum. Front. Cell Infect. Microbiol. 11, 1–13. doi: 10.3389/fcimb.2021.784279
Blount D., Hooi D., Feary J., Venn A., Telford G., Brown A., et al. (2009). Immunologic profiles of persons recruited for a randomized, placebo-controlled clinical trial of hookworm infection. Am. J. Trop. Med. Hyg. 81 (5), 911–916. doi: 10.4269/ajtmh.2009.09-0237
Bobardt S. D., Dillman A. R., Nair M. G. (2020). The two faces of nematode infection: Virulence and immunomodulatory molecules from nematode parasites of mammals, insects and plants. Front. Microbiol. 11, 1–13. doi: 10.3389/fmicb.2020.577846
Boccella S., Vacca V., Errico F., Marinelli S., Squillace M., Guida F., et al. (2015). D-aspartate modulates nociceptive-specific neuron activity and pain threshold in inflammatory and neuropathic pain condition in mice. BioMed. Res. Int. 2015, 1–10. doi: 10.1155/2015/905906
Brooker S. (2010). Estimating the global distribution and disease burden of intestinal nematode infections: Adding up the numbers–a review. Int. J. Parasitol. 40, 1137–1144. doi: 10.1016/j.ijpara.2010.04.004
Brussow H. (2020). Problems with the concept of gut microbiota dysbiosis. Microb. Biotechnol. 13 (2), 423–434. doi: 10.1111/1751-7915.13479
Buck A. H., Coakley G., Simbari F., McSorley H. J., Quintana J. F., Le Bihan T., et al. (2014). Exosomes secreted by nematode parasites transfer small RNAs to mammalian cells and modulate innate immunity. Nat. Commun. 5, 1–11. doi: 10.1038/ncomms6488
Buitrago G., Pickering D., Ruscher R., Cobos Caceres C., Jones L., Cooper M., et al. (2021). A netrin domain-containing protein secreted by the human hookworm Necator americanus protects against CD4 T cell transfer colitis. Transl. Res. 232, 88–102. doi: 10.1016/j.trsl.2021.02.012
Castro G. A., Badial-Aceves F., Smith J. W., Dudrick S. J., Weisbrodt N. W. (1976). Altered small bowel propulsion associated with parasitism. Gastroenterology. 71, 620–625. doi: 10.1016/S0016-5085(76)80553-7
Cavone L., Calosi L., Cinci L., Moroni F., Chiarugi A. (2012). Topical mannitol reduces inflammatory edema in a rat model of arthritis. Pharmacology 89 (1-2), 18–21. doi: 10.1159/000335094
Chajes V., Hulten K., Van Kappel A.-L., Winkvist A., Kaaks R., Hallmans G., et al. (1999). Fatty-acid composition in serum phospholipids and risk of breast cancer: an incident case-control study in Sweden. Int. J. Cancer. 83, 585–590. doi: 10.1002/(SICI)1097-0215(19991126)83:5<585::AID-IJC2>3.0.CO;2-Z
Chavez-Olortegu C., Tavares C. A. P., Resende M. (1992). Purification and characterization of a 47 kDa protease from Schistosoma mansoni cerearial secretion. Parasitology. 105 (2), 211–218. doi: 10.1017/S0031182000074138
Chazot P. L., Coleman S. K., Cik M., Stephenson F. A. (1994). Molecular characterization of n-methyl-D-aspartate receptors expressed in mammalian cells yields evidence for the coexistence of three subunit types within a discrete receptor molecule. J. Biol. Chem. 269 (39), 24403–24409. doi: 10.1016/S0021-9258(19)51098-5
Chen Z. B., Tang H., Liang Y. B., Yang W., Wu J. G., Hu X. C., et al. (2016). Recombinant Trichinella spiralis 53-kDa protein activates M2 macrophages and attenuates the LPS-induced damage of endotoxemia. Innate Immun. 22, 419–432. doi: 10.1177/1753425916651984
Chu K. M., Watermeyer G., Shelly L., Janssen J., May T. D., Brink K., et al. (2013). Childhood helminth exposure is protective against inflammatory bowel disease: a case control study in south Africa. Inflammation Bowel Dis. 19 (3), 614–620. doi: 10.1097/MIB.0b013e31827f27f4
Coburn L. A., Gong X., Singh K., Asim M., Scull B. P., Allaman M. M., et al. (2012). L-arginine supplementation improves responses to injury and inflammation in dextran sulfate sodium colitis. PloS One 7 (3), 1–18. doi: 10.1371/journal.pone.0033546
Coqueiro A. Y., Raizel R., Hypólito T. M., Tirapegui J. (2017). Effects of supplementation with l-glutamine and l-alanine in the body composition of rats submitted to resistance exercise. Rev. Bras. Ciências do Esporte. 39 (4), 417–423. doi: 10.1016/j.rbce.2017.06.003
Croese J., Miller G. C., Marquart L., Llewellyn S., Gupta R., Becker L., et al. (2020). Randomized, placebo controlled trial of experimental hookworm infection for improving gluten tolerance in celiac disease. Clin. Transl. Gastroenterol. 11 (12), 1–11. doi: 10.14309/ctg.0000000000000274
Croese J., O'Neil J., Masson J., Cooke S., Melrose W., Pritchard D., et al. (2006). A proof of concept study establishing Necator americanus in crohn's patients and reservoir donors. Gut. 55 (1), 136–137. doi: 10.1136/gut.2005.079129
Cvetkovic J., Sofronic-Milosavljevic L., Ilic N., Gnjatovic M., Nagano I., Gruden-Movsesijan A. (2016). Immunomodulatory potential of particular Trichinella spiralis muscle larvae excretory-secretory components. Int. J. Parasitol. 46, 833–842. doi: 10.1016/j.ijpara.2016.07.008
Dalton J. P., Skelly P., Halton D. W. (2003). Role of the tegument and gut in nutrient uptake by parasitic platyhelminthes. Can. J. Zool. 82, 211–232. doi: 10.1139/z03-213
Darabi Z., Darand M., Yari Z., Hedayati M., Faghihi A., Agah S., et al. (2019). Inflammatory markers response to citrulline supplementation in patients with non-alcoholic fatty liver disease: A randomized, double blind, placebo-controlled, clinical trial. BMC Res. Notes. 12 (1), 1–5. doi: 10.1186/s13104-019-4130-6
Daveson A. J., Jones D. M., Gaze S., McSorley H., Clouston A., Pascoe A., et al. (2011). Effect of hookworm infection on wheat challenge in celiac disease-a randomised double-blinded placebo controlled trial. PloS One 6 (3), 1–9. doi: 10.1371/journal.pone.0017366
Denery J. R., Nunes A. A., Hixon M. S., Dickerson T. J., Janda K. D. (2010). Metabolomics-based discovery of diagnostic biomarkers for onchocerciasis. PloS Negl. Trop. Dis. 4 (10), 1–9. doi: 10.1371/journal.pntd.0000834
Derakhshanfar A., Bidadkosh A., Hashempour Sadeghian M. (2009). L-methionine attenuates gentamicin nephrotoxicity in male wistar rat: pathological and biochemical findings. Iran J. Vet. Res. 10, 323–328. doi: 10.22099/IJVR.2009.1715
De Silva N. R., Brooker S., Hotez P. J., Montresor A., Engels D., Savioli L. (2003). Soil-transmitted helminth infections: Updating the global picture. Trends Parasitol. 19, 547–551. doi: 10.1016/j.pt.2003.10.002
Detopoulou P., Panagiotakos D. B., Antonopoulou S., Pitsavos C., Stefanadis C. (2008). Dietary choline and betaine intakes in relation to concentrations of inflammatory markers in healthy adults: The ATTICA study. Am. J. Clin. Nutr. 87, 424–430. doi: 10.1093/ajcn/87.2.424
Dong Y. W., Jiang W. D., Liu Y., Wu P., Jiang J., Kuang S. Y., et al. (2017). Threonine deficiency decreased intestinal immunity and aggravated inflammation associated with NF-kappaB and target of rapamycin signalling pathways in juvenile grass carp (Ctenopharyngodon idella) after infection with aeromonas hydrophila. Br. J. Nutr. 118 (2), 92–108. doi: 10.1017/S0007114517001830
Doonan J., Thomas D., Wong M. H., Ramage H. J., Al-Riyami L., Lumb F. E., et al. (2018). Failure of the anti-inflammatory parasitic worm product ES-62 to provide protection in mouse models of type I diabetes, multiple sclerosis, and inflammatory bowel disease. Molecules. 23 (10), 1–14. doi: 10.3390/molecules23102669
Durack J., Lynch S. V. (2019). The gut microbiome: Relationships with disease and opportunities for therapy. J. Exp. Med. 216 (1), 20–40. doi: 10.1084/jem.20180448
Du L., Tang H., Ma Z., Xu J., Gao W., Chen J., et al. (2011). The protective effect of the recombinant 53-kDa protein of Trichinella spiralis on experimental colitis in mice. Dig Dis. Sci. 56 (10), 2810–2817. doi: 10.1007/s10620-011-1689-8
Dzik J. M. (2006). Molecules released by helminth parasites involved in host colonization. Acta Biochim. Pol. 53 (1), 33–64. doi: 10.18388/abp.2006_3361
Easton A. V., Quinones M., Vujkovic-Cvijin I., Oliveira R. G., Kepha S., Odiere M. R., et al. (2019). The impact of anthelmintic treatment on human gut microbiota based on cross-sectional and pre- and postdeworming comparisons in Western Kenya. mBio. 10 (2), 1–14. doi: 10.1128/mBio.00519-19
Eichenberger R. M., Ryan S., Jones L., Buitrago G., Polster R., Montes de Oca M., et al. (2018). Hookworm secreted extracellular vesicles interact with host cells and prevent inducible colitis in mice. Front. Immunol. 9, 1–14. doi: 10.3389/fimmu.2018.00850
Eichenberger R. M., Talukder M. H., Field M. A., Wangchuk P., Giacomin P., Loukas A., et al. (2018). Characterization of Trichuris muris secreted proteins and extracellular vesicles provides new insights into host-parasite communication. J. Extracell Vesicles. 7 (1), 1–16. doi: 10.1080/20013078.2018.1428004
Elliott D. E., Setiawan T., Metwali A., Blum A., Urban J. F. Jr., Weinstock J. V. (2004). Heligmosomoides polygyrus inhibits established colitis in IL-10-deficient mice. Eur. J. Immunol. 34 (10), 2690–2698. doi: 10.1002/eji.200324833
Elliott D. E., Weinstock J. V. (2017). Nematodes and human therapeutic trials for inflammatory disease. Parasite Immunol. 39 (5), 1–22. doi: 10.1111/pim.12407
Evaldsson C., Rydén I., Uppugunduri S. (2007). Anti-inflammatory effects of exogenous uridine in an animal model of lung inflammation. Int. Immunopharmacol. 7 (8), 1025–1032. doi: 10.1016/j.intimp.2007.03.008
Everts B., Perona-Wright G., Smits H. H., Hokke C. H., van der Ham A. J., Fitzsimmons C. M., et al. (2009). Omega-1, a glycoprotein secreted by Schistosoma mansoni eggs, drives Th2 responses. J. Exp. Med. 206 (8), 1673–1680. doi: 10.1084/jem.20082460
Faure M., Mettraux C., Moennoz D., Godin J.-P., Vuichoud J., Rochat F., et al. (2006). Specific amino acids increase mucin synthesis and microbiota in dextran sulfate sodium–treated rats. J. Nutr. 136, 1558–1564. doi: 10.1093/jn/136.6.1558
Ferreira M. S., de Oliveira D. N., de Oliveira R. N., Allegretti S. M., Catharino R. R. (2014). Screening the life cycle of Schistosoma mansoni using high-resolution mass spectrometry. Anal. Chim. Acta 845, 62–69. doi: 10.1016/j.aca.2014.06.013
Ferreira M. S., de Oliveira D. N., de Oliveira R. N., Allegretti S. M., Vercesi A. E., Catharino R. R. (2014). Mass spectrometry imaging: a new vision in differentiating Schistosoma mansoni strains. J. Mass Spectrom. 49 (1), 86–92. doi: 10.1002/jms.3308
Ferreira M. S., de Oliveira R. N., de Oliveira D. N., Esteves C. Z., Allegretti S. M., Catharino R. R. (2015). Revealing praziquantel molecular targets using mass spectrometry imaging: An expeditious approach applied to schistosoma mansoni. Int. J. Parasitol. 45 (6), 385–391. doi: 10.1016/j.ijpara.2014.12.008
Ferreira I., Smyth D., Gaze S., Aziz A., Giacomin P., Ruyssers N., et al. (2013). Hookworm excretory/secretory products induce interleukin-4 (il-4)+ il-10+ cd4+ t cell responses and suppress pathology in a mouse model of colitis. Infect. Immun. 81 (6), 2104–2111. doi: 10.1128/IAI.00563-12
Figueiredo B. C., Da’dara A. A., Oliveira S. C., Skelly P. J. (2015). Schistosomes enhance plasminogen activation: The role of tegumental enolase. PloS Pathog. 11 (12), 1–22. doi: 10.1371/journal.ppat.1005335
Filyk H. A., Osborne L. C. (2016). The multibiome: the intestinal ecosystem's influence on immune homeostasis, health, and disease. EBioMedicine. 13, 46–54. doi: 10.1016/j.ebiom.2016.10.007
Fleming J., Hernandez G., Hartman L., Maksimovic J., Nace S., Lawler B., et al. (2019). Safety and efficacy of helminth treatment in relapsing-remitting multiple sclerosis: Results of the HINT 2 clinical trial. Mult Scler. 25 (1), 81–91. doi: 10.1177/1352458517736377
Flohr C., Tuyen L. N., Lewis S., Quinnell R., Minh T. T., Liem H. T., et al. (2006). Poor sanitation and helminth infection protect against skin sensitization in Vietnamese children: A cross-sectional study. J. Allergy Clin. Immunol. 118 (6), 1305–1311. doi: 10.1016/j.jaci.2006.08.035
Flynn A. F., Joyce M. G., Taylor R. T., Bennuru S., Lindrose A. R., Sterling S. L., et al. (2019). Intestinal UDP-glucuronosyltransferase as a potential target for the treatment and prevention of lymphatic filariasis. PloS Negl. Trop. Dis. 13 (9), 1–25. doi: 10.1371/journal.pntd.0007687
Fotiadis C., Adamis S., Misiakos E. P., Genetzakis M., Antonakis P. T., Tsekouras D. K., et al. (2016). The prophylactic effect of l-arginine in acute ischaemic colitis in a rat model of ischaemia/reperfusion injury. Acta Chirurgica Belgica. 107 (2), 192–200. doi: 10.1080/00015458.2007.11680039
Fox J. G., Beck P., Dangler C. A., Whary M. T., Wang T. C., Shi H. N., et al. (2000). Concurrent enteric helminth infection modulates inflammation and gastric immune responses and reduces helicobacter-induced gastric atrophy. Nat. Med. 6, 536–542. doi: 10.1038/75015
Fujino M., Nishio Y., Ito H., Tanaka T., Li X. K. (2016). 5-aminolevulinic acid regulates the inflammatory response and alloimmune reaction. Int. Immunopharmacol. 37, 71–78. doi: 10.1016/j.intimp.2015.11.034
Fukuda T., Majumder K., Zhang H., Matsui T., Mine Y. (2017). Adenine has an anti-inflammatory effect through the activation of adenine receptor signaling in mouse macrophage. J. Funct. Foods. 28, 235–239. doi: 10.1016/j.jff.2016.11.013
Gaifem J., Goncalves L. G., Dinis-Oliveira R. J., Cunha C., Carvalho A., Torrado E., et al. (2018). L-threonine supplementation during colitis onset delays disease recovery. Front. Physiol. 9, 1–7. doi: 10.3389/fphys.2018.01247
Garcia-Perez I., Whitfield P., Bartlett A., Angulo S., Legido-Quigley C., Hanna-Brown M., et al. (2008). Metabolic fingerprinting of Schistosoma mansoni infection in mice urine with capillary electrophoresis. Electrophoresis. 29 (15), 3201–3206. doi: 10.1002/elps.200800031
Giera M., Kaisar M. M. M., Derks R. J. E., Steenvoorden E., Kruize Y. C. M., Hokke C. H., et al. (2018). The Schistosoma mansoni lipidome: Leads for immunomodulation. Anal. Chim. Acta 1037, 107–118. doi: 10.1016/j.aca.2017.11.058
Globisch D., Moreno A. Y., Hixon M. S., Nunes A. A., Denery J. R., Specht S., et al. (2013). Onchocerca volvulus-neurotransmitter tyramine is a biomarker for river blindness. Proc. Natl. Acad. Sci. 110 (11), 4218–4223. doi: 10.1073/pnas.1221969110
Glushakova O., Kosugi T., Roncal C., Mu W., Heinig M., Cirillo P., et al. (2008). Fructose induces the inflammatory molecule ICAM-1 in endothelial cells. J. Am. Soc. Nephrol. 19, 1712–1720. doi: 10.1681/ASN.2007121304
Gordon C. A., Krause L., McManus D. P., Morrison M., Weerakoon K. G., Connor M. C., et al. (2020). Helminths, polyparasitism, and the gut microbiome in the Philippines. Int. J. Parasitol. 50 (3), 217–225. doi: 10.1016/j.ijpara.2019.12.008
Grencis R. K. (2015). Immunity to helminths: Resistance, regulation, and susceptibility to gastrointestinal nematodes. Annu. Rev. Immunol. 33, 201–225. doi: 10.1146/annurev-immunol-032713-120218
Grundy S. M., Cleeman J. I., Daniels S. R., Donato K. A., Eckel R. H., Franklin B. A., et al. (2005). Diagnosis and management of the metabolic syndrome: An American heart Association/National heart, lung, and blood institute scientific statement. Circulation. 112 (17), 2735–2752. doi: 10.1161/CIRCULATIONAHA.105.169404
Guo K., Sun X., Gu Y., Wang Z., Huang J., Zhu X. (2016). Trichinella spiralis paramyosin activates mouse bone marrow-derived dendritic cells and induces regulatory T cells. Parasit Vectors. 9 (1), 1–11. doi: 10.1186/s13071-016-1857-y
Haeberlein S., Obieglo K., Ozir-Fazalalikhan A., Chayé M. A. M., Veninga H., van der Vlugt L. E. P. M., et al. (2017). Schistosome egg antigens, including the glycoprotein IPSE/alpha-1, trigger the development of regulatory b cells. PloS Pathog. 13 (7), 1–28. doi: 10.1371/journal.ppat.1006539
Hamed M. A. A., Ahmed S. A. A., Khaled H. M. (2011). Efficiency of diagnostic biomarkers among colonic schistosomiasis Egyptian patients. Mem Inst Oswaldo Cruz. 106 (3), 322–329. doi: 10.1590/S0074-02762011000300011
Ham D. J., Gleeson B. G., Chee A., Baum D. M., Caldow M. K., Lynch G. S., et al. (2015). L-citrulline protects skeletal muscle cells from cachectic stimuli through an iNOS-dependent mechanism. PloS One 10 (10), 1–17. doi: 10.1371/journal.pone.0141572
Han D., Kim H. Y., Lee H. J., Shim I., Hahm D. H. (2007). Wound healing activity of gamma-aminobutyric acid (GABA) in rats. J. Microbiol. Biotechnol. 17, 1661–1669.
Han H., Yin J., Wang B., Huang X., Yao J., Zheng J., et al. (2018). Effects of dietary lysine restriction on inflammatory responses in piglets. Sci. Rep. 8 (1), 1–8. doi: 10.1038/s41598-018-20689-3
Harder A. (2016). The biochemistry of Haemonchus contortus and other parasitic nematodes. Adv. Parasitol. 93, 69–94. doi: 10.1016/bs.apar.2016.02.010
Harris P. A., Berger S. B., Jeong J. U., Nagilla R., Bandyopadhyay D., Campobasso N., et al. (2017). Discovery of a first-in-class receptor interacting protein 1 (RIP1) kinase specific clinical candidate (GSK2982772) for the treatment of inflammatory diseases. J. Med. Chem. 60 (4), 1247–1261. doi: 10.1021/acs.jmedchem.6b01751
Hasegawa S., Ichiyama T., Sonaka I., Ohsaki A., Okada S., Wakiguchi H., et al. (2012). Cysteine, histidine and glycine exhibit anti-inflammatory effects in human coronary arterial endothelial cells. Clin. Exp. Immunol. 167 (2), 269–274. doi: 10.1111/j.1365-2249.2011.04519.x
Hasko G., Kuhel D. G., Nemeth Z. H., Mabley J. G., Stachlewitz R. F., Virag L., et al. (2000). Inosine inhibits inflammatory cytokine production by a posttranscriptional mechanism and protects against endotoxin-induced shock. J. Immunol. 164 (2), 1013–1019. doi: 10.4049/jimmunol.164.2.1013
Hazel J. R., Williams E. E. (1990). The role of alterations in membrane lipid composition in enabling physiological adaptation of organisms to their physical environment. Prog. Lipid Res. 29, 167–227. doi: 10.1016/0163-7827(90)90002-3
Hearps A. C., Tyssen D., Srbinovski D., Bayigga L., Diaz D. J. D., Aldunate M., et al. (2017). Vaginal lactic acid elicits an anti-inflammatory response from human cervicovaginal epithelial cells and inhibits production of pro-inflammatory mediators associated with HIV acquisition. Mucosal Immunol. 10, 1480–1490. doi: 10.1038/mi.2017.27
Hegedus Z. L., Nayak U. (1994). Homogentisic acid and structurally related compounds as intermediates in plasma soluble melanin formation and in tissue toxicities. Arch. Internationalesde Physiologic Biochimie Biophysique 102, 175–181. doi: 10.3109/13813459409007534
He L., Li H., Huang N., Zhou X., Tian J., Li T., et al. (2017). Alpha-ketoglutarate suppresses the NF-κB-mediated inflammatory pathway and enhances the PXR-regulated detoxification pathway. Oncotarget. 8, 102974–102988. doi: 10.18632/oncotarget.16875
Helmby H. (2015). Human helminth therapy to treat inflammatory disorders - where do we stand? BMC Immunol. 16, 12. doi: 10.1186/s12865-015-0074-3
He F., Wu C., Li P., Li N., Zhang D., Zhu Q., et al. (2018). Functions and signaling pathways of amino acids in intestinal inflammation. BioMed. Res. Int. 2018, 1–13. doi: 10.1155/2018/9171905
Hnia K., Gayraud J., Hugon G., Ramonatxo M., de la Porte S., Matecki S., et al. (2008). L-arginine decreases inflammation and modulates the nuclear factor-kappaB/matrix metalloproteinase cascade in mdx muscle fibers. Am. J. Pathol. 172 (6), 1509–1519. doi: 10.2353/ajpath.2008.071009
Hotez P. J., Brindley P. J., Bethony J. M., King C. H., Pearce E. J., Jacobson J. (2008). Helminth infections: the great neglected tropical diseases. J. Clin. Invest. 118, 1311–1321. doi: 10.1172/JCI34261
Hotterbeekx A., Dusabimana A., Mandro M., Abhafule G. M., Deogratias W., Siewe Fodjo J. N., et al. (2020). Urinary n-acetyltyramine-O,beta-glucuronide in persons with onchocerciasis-associated epilepsy. Pathogens. 9 (3), 1–10. doi: 10.3390/pathogens9030191
Hoy A. M., Lundie R. J., Ivens A., Quintana J. F., Nausch N., Forster T., et al. (2014). Parasite-derived microRNAs in host serum as novel biomarkers of helminth infection. PloS Negl. Trop. Dis. 8 (2), 1–14. doi: 10.1371/journal.pntd.0002701
Huang P. L. (2009). A comprehensive definition for metabolic syndrome. Dis. Model. Mech. 2 (5-6), 231–237. doi: 10.1242/dmm.001180
Huang W. C., Tsai T. H., Chuang L. T., Li Y. Y., Zouboulis C. C., Tsai P. J. (2014). Antibacterial and anti-inflammatory properties of capric acid against propionibacterium acnes: A comparative study with lauric acid. J. Dermatol. Sci. 73, 232–240. doi: 10.1016/j.jdermsci.2013.10.010
Huang X., Zeng L. R., Chen F. S., Zhu J. P., Zhu M. H. (2018). Trichuris suis ova therapy in inflammatory bowel disease: A meta-analysis. Med. (Baltimore). 97 (34), 1–9. doi: 10.1097/MD.0000000000012087
Hunter M. M., Wang A., Hirota C. L., McKay D. M. (2005). Neutralizing anti-IL-10 antibody blocks the protective effect of tapeworm infection in a murine model of chemically induced colitis. J. Immunol. 174 (11), 7368–7375. doi: 10.4049/jimmunol.174.11.7368
Islam J., Sato S., Watanabe K., Watanabe T., Ardiansyah, Hirahara K., et al. (2017). Dietary tryptophan alleviates dextran sodium sulfate-induced colitis through aryl hydrocarbon receptor in mice. J. Nutr. Biochem. 42, 43–50. doi: 10.1016/j.jnutbio.2016.12.019
Jenkins T. P., Formenti F., Castro C., Piubelli C., Perandin F., Buonfrate D., et al. (2018). A comprehensive analysis of the faecal microbiome and metabolome of Strongyloides stercoralis infected volunteers from a non-endemic area. Sci. Rep. 8 (1), 1–13. doi: 10.1038/s41598-018-33937-3
Jenkins T. P., Rathnayaka Y., Perera P. K., Peachey L. E., Nolan M. J., Krause L., et al. (2017). Infections by human gastrointestinal helminths are associated with changes in faecal microbiota diversity and composition. PloS One 12 (9), 1–18. doi: 10.1371/journal.pone.0184719
Jiao N., Wu Z., Ji Y., Wang B., Dai Z., Wu G. (2015). L-glutamate enhances barrier and antioxidative functions in intestinal porcine epithelial cells. J. Nutr. 145 (10), 2258–2264. doi: 10.3945/jn.115.217661
Jin X., Yang Y., Liu X., Shi H., Cai X., Luo X., et al. (2019). Glutathione-s-transferase of Trichinella spiralis regulates maturation and function of dendritic cells. Parasitology. 146 (14), 1725–1732. doi: 10.1017/S003118201900115X
Joshi A., Kimball A., Schaller M., denDekker A., Burant C., Kunkel S. L., et al. (2018). Modulation of xanthine metabolism ameliorates inflammation and accelerates diabetic wound healing. J. Immunol. 200 (1):170.13 13.
Kabeerdoss J., Pugazhendhi S., Subramanian V., Binder H. J., Ramakrishna B. S. (2011). Exposure to hookworms in patients with crohn's disease: a case-control study. Aliment Pharmacol. Ther. 34 (8), 923–930. doi: 10.1111/j.1365-2036.2011.04824.x
Kahl J., Brattig N., Liebau E. (2018). The untapped pharmacopeic potential of helminths. Trends Parasitol. 34, 828–842. doi: 10.1016/j.pt.2018.05.011
Kalinowska M., Mazur L., Jabłońska-Trypuć A., Lewandowski W. (2018). A new calcium 2,5-dihydroxybenzoate: synthesis, characterization and antioxidant studies and stress mediated cytotoxity in MCF-7 cells. J. Saudi Chem. Soc 22 (6), 742–756. doi: 10.1016/j.jscs.2017.12.006
Kammoun-Rebai W., Naouar I., Libri V., Albert M., Louzir H., Meddeb-Garnaoui A., et al. (2016). Protein biomarkers discriminate Leishmania major-infected and non-infected individuals in areas endemic for cutaneous leishmaniasis. BMC Infect. Dis. 16, 1–10. doi: 10.1186/s12879-016-1458-6
Kato H., Miura K., Nakano S., Suzuki K., Bannai M., Inoue Y. (2016). Leucine-enriched essential amino acids attenuate inflammation in rat muscle and enhance muscle repair after eccentric contraction. Amino Acids 48 (9), 2145–2155. doi: 10.1007/s00726-016-2240-1
Kaur N., Chen C. C., Luther J., Kao J. Y. (2011). Intestinal dysbiosis in inflammatory bowel disease. Gut Microbes 2 (4), 211–216. doi: 10.4161/gmic.2.4.17863
Keskin E., Uluisik D., Altin M. (2015). Antioxidant effect of l-carnitine in rats fed cholesterol rich diet. Anim. Vet. Sci. 3 (4), 113–116. doi: 10.11648/j.avs.20150304.13
Khan W. I., Blennerhasset P. A., Varghese A. K., Chowdhury S. K., Omsted P., Deng Y., et al. (2002). Intestinal nematode infection ameliorates experimental colitis in mice. Infect. Immun. 70 (11), 5931–5937. doi: 10.1128/IAI.70.11.5931-5937.2002
Khodayar M. J., Kalantari H., Khorsandi L., Rashno M., Zeidooni L. (2020). Upregulation of Nrf2- related cytoprotective genes expression by acetaminophen-induced acute hepatotoxicity in mice and the protective role of betaine. Hum. Exp. Toxicol., 39(7), 948–959. doi: 10.1177/0960327120905962
Khurana S., Singh S., Mewara A. (2021). Diagnostic techniques for soil-transmitted helminths-recent advances. Res. Rep. Trop. Med. 12, 181–196. doi: 10.2147/RRTM.S278140
Kleiner R. E., Dumelin C. E., Liu D. R. (2011). Small-molecule discovery from DNA-encoded chemical libraries. Chem. Soc. Rev. 40 (12), 5707–5717. doi: 10.1039/c1cs15076f
Kobpornchai P., Flynn R. J., Reamtong O., Rittisoonthorn N., Kosoltanapiwat N., Boonnak K., et al. (2020). A novel cystatin derived from Trichinella spiralis suppresses macrophage-mediated inflammatory responses. PloS Negl. Trop. Dis. 14 (4), 1–24. doi: 10.1371/journal.pntd.0008192
Kovarik J. J., Tillinger W., Hofer J., Holzl M. A., Heinzl H., Saemann M. D., et al. (2011). Impaired anti-inflammatory efficacy of n-butyrate in patients with IBD. Eur. J. Clin. Invest. 41 (3), 291–298. doi: 10.1111/j.1365-2362.2010.02407.x
Kradin R. L., Badizadegan K., Auluck P., Korzenik J., Lauwers G. Y. (2006). Iatrogenic Trichuris suis infection in a patient with crohn disease. Arch. Pathol. Lab. Med. 130 (5), 718–720. doi: 10.5858/2006-130-718-ITSIIA
Kris-Etherton P. M., Griel A. E., Psota T. L., Gebaur S. K., Zhang J., Etherton T. D. (2005). Dietary stearic acid and risk of cardiovascular disease: intake, sources, digestion, and absorption. Lipids. 40, 1193–1200. doi: 10.1007/s11745-005-1485-y
Kuda O. (2017). Bioactive metabolites of docosahexaenoic acid. Biochimie. 136, 12–20. doi: 10.1016/j.biochi.2017.01.002
Kumagai T., Osada Y., Ohta N., Kanazawa T. (2009). Peroxiredoxin-1 from Schistosoma japonicum functions as a scavenger against hydrogen peroxide but not nitric oxide. Mol. Biochem. Parasitol. 164 (1), 26–31. doi: 10.1016/j.molbiopara.2008.11.002
Kunig V., Potowski M., Gohla A., Brunschweiger A. (2018). DNA-Encoded libraries - an efficient small molecule discovery technology for the biomedical sciences. Biol. Chem. 399 (7), 691–710. doi: 10.1515/hsz-2018-0119
Kupritz J., Angelova A., Nutman T. B., Gazzinelli-Guimaraesa P. H. (2021). Helminth-induced human gastrointestinal dysbiosis: A systematic review and meta-analysis reveals insights into altered taxon diversity and microbial gradient collapse. mBio. 12, 1–17. doi: 10.1128/mBio.02890-21
Kyu H. H., Abate D., Abate K. H., Abay S. M., Abbafati C., Abbasi N., et al. (2018). Global, regional, and national disability-adjusted life-years (DALYs) for 359 diseases and injuries and healthy life expectancy (HALE) for 195 countries and territories, 1990–2017: A systematic analysis for the global burden of disease study 2017. Lancet 392 (10159), 1859–1922. doi: 10.1016/S0140-6736(18)32335-3
Lagatie O., Njumbe Ediage E., Batsa Debrah L., Diels L., Nolten C., Vinken P., et al. (2016). Evaluation of the diagnostic potential of urinary n-Acetyltyramine-O,beta-glucuronide (NATOG) as diagnostic biomarker for Onchocerca volvulus infection. Parasit Vectors. 9 (1), 1–10. doi: 10.1186/s13071-016-1582-6
Lagatie O., Verheyen A., Van Asten S., Odiere M. R., Djuardi Y., Levecke B., et al. (2020). 2-methyl-pentanoyl-carnitine (2-MPC): A urine biomarker for patent Ascaris lumbricoides infection. Sci. Rep. 10 (1), 1–13. doi: 10.1038/s41598-020-72804-y
Largo R., Alvarez-Soria M. A., Diez-Ortego I., Calvo E., Sánchez-Pernaute O., Egido J., et al. (2003). Glucosamine inhibits IL-1-induced NF-kB activation in human osteoarthritic chondrocytes. Osteoarthr Cartil. 11, 290–298. doi: 10.1016/S1063-4584(03)00028-1
Lee B. J., Lin J. S., Lin Y. C., Lin P. T. (2015). Antiinflammatory effects of l-carnitine supplementation (1000 mg/d) in coronary artery disease patients. Nutr. 31 (3), 475–479. doi: 10.1016/j.nut.2014.10.001
Lee Y. C., Su Y. T., Liu T. Y., Tsai C. M., Chang C. H., Yu H. R. (2018). L-arginine and l-citrulline supplementation have different programming effect on regulatory T-cells function of infantile rats. Front. Immunol. 9, 1–11. doi: 10.3389/fimmu.2018.02911
Lee S. C., Tang M. S., Lim Y. A., Choy S. H., Kurtz Z. D., Cox L. M., et al. (2014). Helminth colonization is associated with increased diversity of the gut microbiota. PloS Negl. Trop. Dis. 8 (5), 1–15. doi: 10.1371/journal.pntd.0002880
Lee J. S., Wang R. X., Alexeev E. E., Lanis J. M., Battista K. D., Glover L. E., et al. (2018). Hypoxanthine is a checkpoint stress metabolite in colonic epithelial energy modulation and barrier function. J. Biol. Chem. 293 (16), 6039–6051. doi: 10.1074/jbc.RA117.000269
Lei W., Ren W., Ohmoto M., Urban J. F. Jr., Matsumoto I., Margolskee R. F., et al. (2018). Activation of intestinal tuft cell-expressed Sucnr1 triggers type 2 immunity in the mouse small intestine. Proc. Natl. Acad. Sci. 115 (21), 5552–5557. doi: 10.1073/pnas.1720758115
Liaudet L., Mabley J. G., Soriano F. G., Pacher P., Marton A., Hasko G., et al. (2001). Inosine reduces systemic inflammation and improves survival in septic shock induced by cecal ligation and puncture. Am. J. Respir. Crit. Care Med. 164, 1213–1220. doi: 10.1164/ajrccm.164.7.2101013
Lindsay A., Gieseg S. P. (2020). Pterins as diagnostic markers of exercise-induced stress: a systematic review. J. Sci. Med. Sport. 23 (1), 53–62. doi: 10.1016/j.jsams.2019.08.018
Li J. V., Saric J., Wang Y., Keiser J., Utzinger J., Holmes E. (2011). Chemometric analysis of biofluids from mice experimentally infected with schistosoma mansoni. Parasit Vectors 4, 179:1–17916. doi: 10.1186/1756-3305-4-179
Li S., Song G., Bai Y., Song N., Zhao J., Liu J., et al. (2021). Applications of protein microarrays in biomarker discovery for autoimmune diseases. Front. Immunol. 12, 1–13. doi: 10.3389/fimmu.2021.645632
Littlewood-Evans A., Sarret S., Apfel V., Loesle P., Dawson J., Zhang J., et al. (2016). GPR91 senses extracellular succinate released from inflammatory macrophages and exacerbates rheumatoid arthritis. J. Exp. Med. 213 (9), 1655–1662. doi: 10.1084/jem.20160061
Liu R., Li P., Bi W. C., Ma R., Yin Y., Bi K., et al. (2017). Plasma n-acetylputrescine, cadaverine and 1,3-diaminopropane: Potential biomarkers of lung cancer used to evaluate the ef cacy of anticancer drugs. Oncotarget. 8, 88575–88585. doi: 10.18632/oncotarget.19304
Liu X. D., Wang X. L., Bai X., Liu X. L., Wu X. P., Zhao Y., et al. (2014). Oral administration with attenuated salmonella encoding a Trichinella cystatin-like protein elicited host immunity. Exp. Parasitol. 141, 1–11. doi: 10.1016/j.exppara.2014.03.015
Li X. S., Wang Z., Cajka T., Buffa J. A., Nemet I., Hurd A. G., et al. (2018). Untargeted metabolomics identifies trimethyllysine, a TMAO-producing nutrient precursor, as a predictor of incident cardiovascular disease risk. JCI Insight 3 (6), 1–18. doi: 10.1172/jci.insight.99096
Longman R. S., Littman D. R. (2015). The functional impact of the intestinal microbiome on mucosal immunity and systemic autoimmunity. Curr. Opin. Rheumatol. 27, 381–387. doi: 10.1097/BOR.0000000000000190
Longo N. (2009). Disorders of biopterin metabolism. J. Inherit Metab. Dis. 32, 333–342. doi: 10.1007/s10545-009-1067-2
Loukas A., Hotez P. J., Diemert D., Yazdanbakhsh M., McCarthy J. S., Correa-Oliveira R., et al. (2016). Hookworm infection. Nat. Rev. Dis. Primers. 2, 1–18. doi: 10.1038/nrdp.2016.88
Loukas A., Prociv P. (2001). Immune responses in hookworm infections. Clin. Microbiol. Rev. 14 (4), 689–703. doi: 10.1128/CMR.14.4.689-703.2001
Ma E. H., Bantug G., Griss T., Condotta S., Johnson R. M., Samborska B., et al. (2017). Serine is an essential metabolite for effector T cell expansion. Cell Metab. 25 (2), 345–357. doi: 10.1016/j.cmet.2016.12.011
Magalhaes K., Almeida P. E., Atella G., Maya-Monteiro C. M., Castro-Faria-Neto H., Pelajo-Machado M., et al. (2010). Schistosomal-derived lysophosphatidylcholine are involved in eosinophil activation and recruitment through toll-like receptor-2-dependent mechanisms. J. Infect. Dis. 202 (9), 1369–1379. doi: 10.1086/656477
Maizels R. M., Bundy D. A. P., Selkirk M. E., Smith D. F., Anderson R. M. (1993). Immunological modulation and evasion by helminth parasites in human populations. Nature. 365, 797–805. doi: 10.1038/365797a0
Maizels R. M., McSorley H. J. (2016). Regulation of the host immune system by helminth parasites. J. Allergy Clin. Immunol. 138, 666–675. doi: 10.1016/j.jaci.2016.07.007
Maizels R. M., Smits H. H., McSorley H. J. (2018). Modulation of host immunity by helminths: the expanding repertoire of parasite effector molecules. Immunity. 49, 801–818. doi: 10.1016/j.immuni.2018.10.016
Maizels R. M., Yazdanbakhsh M. (2003). Immune regulation by helminth parasites: cellular and molecular mechanisms. Nat. Rev. Immunol. 3 (9), 733–744. doi: 10.1038/nri1183
Makurvet F. D. (2021). Biologics vs. small molecules: Drug costs and patient access. Med. Drug Discovery 9, 1–8. doi: 10.1016/j.medidd.2020.100075
Mangmee S., Adisakwattana P., Tipthara P., Simanon N., Sonthayanon P., Reamtong O. (2020). Lipid profile of Trichinella papuae muscle-stage larvae. Sci. Rep. 10 (1), 1–11. doi: 10.1038/s41598-020-67297-8
Marikovsky M., Fishelson Z., Arnon R. (1988). Purification and characterization of proteases secreted by transforming schistosomula of schistosoma mansoni. Mol. Biochem. Parasitol. 30 (1), 45–54. doi: 10.1016/0166-6851(88)90131-4
Martin I., Djuardi Y., Sartono E., Rosa B. A., Supali T., Mitreva M., et al. (2018). Dynamic changes in human-gut microbiome in relation to a placebo-controlled anthelminthic trial in Indonesia. PloS Negl. Trop. Dis. 12 (8), 1–19. doi: 10.1371/journal.pntd.0006620
Martin I., Kaisar M. M. M., Wiria A. E., Hamid F., Djuardi Y., Sartono E., et al. (2019). The effect of gut microbiome composition on human immune responses: An exploration of interference by helminth infections. Front. Genet. 10, 1–10. doi: 10.3389/fgene.2019.01028
Mastrofrancesco A., Ottaviani M., Aspite N., Cardinali G., Izzo E., Graupe K., et al. (2010). Azelaic acid modulates the inflammatory response in normal human keratinocytes through PPARgamma activation. Exp. Dermatol. 19 (9), 813–820. doi: 10.1111/j.1600-0625.2010.01107.x
Ma G., Wang T., Korhonen P. K., Nie S., Reid G. E., Stroehlein A. J., et al. (2019). Comparative bioinformatic analysis suggests that specific dauer-like signalling pathway components regulate Toxocara canis development and migration in the mammalian host. Parasit Vectors. 12 (1), 1–10. doi: 10.1186/s13071-018-3265-y
Ma G., Wang T., Korhonen P. K., Young N. D., Nie S., Ang C. S., et al. (2019). Dafachronic acid promotes larval development in Haemonchus contortus by modulating dauer signalling and lipid metabolism. PloS Pathog. 15 (7), 1–20. doi: 10.1371/journal.ppat.1007960
McCarthy J. S., Lustigman S., Yang G. J., Barakat R. M., Garcia H. H., Sripa B., et al. (2012). A research agenda for helminth diseases of humans: diagnostics for control and elimination programmes. PloS Negl. Trop. Dis. 6 (4), 1–13. doi: 10.1371/journal.pntd.0001601
McSorley H. J., Gaze S., Daveson J., Jones D., Anderson R. P., Clouston A., et al. (2011). Suppression of inflammatory immune responses in celiac disease by experimental hookworm infection. PloS One 6 (9), 1–7. doi: 10.1371/journal.pone.0024092
McSorley H. J., Hewitson J. P., Maizels R. M. (2013). Immunomodulation by helminth parasites: Defining mechanisms and mediators. Int. J. Parasitol. 43, 301–310. doi: 10.1016/j.ijpara.2012.11.011
Mejia R., Damania A., Jeun R., Bryan P. E., Vargas P., Juarez M., et al. (2020). Impact of intestinal parasites on microbiota and cobalamin gene sequences: A pilot study. Parasit Vectors. 13 (1), 1–9. doi: 10.1186/s13071-020-04073-7
Miller H. R., Nawa Y. (1979). Immune regulation of intestinal goblet cell differentiation. specific induction of nonspecific protection against helminths? Nouv Rev. Fr Hematol. 21 (1), 31–45.
Mine Y., Zhang H. (2015). Calcium-sensing receptor (CaSR)-mediated anti-inflammatory effects of l-amino acids in intestinal epithelial cells. J. Agric. Food Chem. 63 (45), 9987–9995. doi: 10.1021/acs.jafc.5b03749
Molodecky N. A., Soon I. S., Rabi D. M., Ghali W. A., Ferris M., Chernoff G., et al. (2012). Increasing incidence and prevalence of the inflammatory bowel diseases with time, based on systematic review. Gastroenterology. 142 (1), 46–54.e42. doi: 10.1053/j.gastro.2011.10.001
Montresor A., Mupfasoni D., Mikhailov A., Mwinzi P., Lucianez A., Jamsheed M., et al. (2020). The global progress of soil-transmitted helminthiases control in 2020 and world health organization targets for 2030. PloS Negl. Trop. Dis. 14 (8), 1–17. doi: 10.1371/journal.pntd.0008505
Moreels T. G., Nieuwendijk R. J., De Man J. G., De Winter B. Y., Herman A. G., Van Marck E. A., et al. (2004). Concurrent infection with Schistosoma mansoni attenuates inflammation induced changes in colonic morphology, cytokine levels, and smooth muscle contractility of trinitrobenzene sulphonic acid induced colitis in rats. Gut. 53, 99–107. doi: 10.1136/gut.53.1.99
Moro K., Nagahashi M., Ramanathan R., Takabe K., Wakai T. (2016). Resolvins and omega three polyunsaturated fatty acids: Clinical implications in inflammatory diseases and cancer. World J. Clin. Cases. 4 (7), 155–164. doi: 10.12998/wjcc.v4.i7.155
Mu Y., McManus D. P., Gordon C. A., Cai P. (2021). Parasitic helminth-derived microRNAs and extracellular vesicle cargos as biomarkers for helminthic infections. Front. Cell Infect. Microbiol. 11, 1–8. doi: 10.3389/fcimb.2021.708952
Murray C. J. L., Vos T., Lozano R., Naghavi M., Flaxman A. D., Michaud C., et al. (2012). Disability-adjusted life years (DALYs) for 291 diseases and injuries in 21 regions, 1990–2010: a systematic analysis for the global burden of disease study 2010. Lancet 380 (9859), 2197–2223. doi: 10.1016/S0140-6736(12)61689-4
Naügeli M., Fasshauer M., Sommerfeld J., Fendel A., Brandi G., Stover J. F. (2014). Prolonged continuous intravenous infusion of the dipeptide l-alanine- l-glutamine significantly increases plasma glutamine and alanine without elevating brain glutamate in patients with severe traumatic brain injury. Crit. Care 18, R139:1–R13912. doi: 10.1186/cc13962.
Nadjsombati M. S., McGinty J. W., Lyons-Cohen M. R., Jaffe J. B., DiPeso L., Schneider C., et al. (2018). Detection of succinate by intestinal tuft cells triggers a type 2 innate immune circuit. Immunity. 49 (1), 33–41.e7. doi: 10.1016/j.immuni.2018.06.016
Oldenburger A., Roscioni S. S., Jansen E., Menzen M. H., Halayko A. J., Timens W., et al. (2012). Anti-inflammatory role of the cAMP effectors epac and PKA: implications in chronic obstructive pulmonary disease. PloS One 7 (2), 1–12. doi: 10.1371/journal.pone.0031574
Olivera P., Danese S., Peyrin-Biroulet L. (2017). Next generation of small molecules in inflammatory bowel disease. Gut. 66 (2), 199–209. doi: 10.1136/gutjnl-2016-312912
Patton J. B., Bonne-Annee S., Deckman J., Hess J. A., Torigian A., Nolan T. J., et al. (2018). Methylprednisolone acetate induces, and Delta7-dafachronic acid suppresses, Strongyloides stercoralis hyperinfection in NSG mice. Proc. Natl. Acad. Sci. 115 (1), 204–209. doi: 10.1073/pnas.1712235114
Pederzolli C. D., Mescka C. P., Zandona B. R., de Moura Coelho D., Sgaravatti A. M., Sgarbi M. B., et al. (2010). Acute administration of 5-oxoproline induces oxidative damage to lipids and proteins and impairs antioxidant defenses in cerebral cortex and cerebellum of young rats. Metab. Brain Dis. 25 (2), 145–154. doi: 10.1007/s11011-010-9190-1
Periago M. V., Bethony J. M. (2012). Hookworm virulence factors: making the most of the host. Microbes Infect. 14, 1451–1464. doi: 10.1016/j.micinf.2012.09.002
Pineda M. A., Eason R. J., Harnett M. M., Harnett W. (2015). From the worm to the pill, the parasitic worm product ES-62 raises new horizons in the treatment of rheumatoid arthritis. Lupus 24 (4-5), 400–411. doi: 10.1177/0961203314560004
Pineda M. A., Lumb F., Harnett M. M., Harnett W. (2014). ES-62, a therapeutic anti-inflammatory agent evolved by the filarial nematode Acanthocheilonema viteae. Mol. Biochem. Parasitol. 194 (1-2), 1–8. doi: 10.1016/j.molbiopara.2014.03.003
Prideaux L., Kamm M. A., De Cruz P. P., Chan F. K., Ng S. C. (2012). Inflammatory bowel disease in Asia: a systematic review. J. Gastroenterol. Hepatol. 27 (8), 1266–1280. doi: 10.1111/j.1440-1746.2012.07150.x
Proudfoot L., Kusel J. R., Smith H. V., Harnett W., Worms M. J., Kennedy M. W. (1990). The surface lipid of parasitic nematodes: Organization, and modifications during transition to the mammalian host environment. Acta Trop. 47, 323–330. doi: 10.1016/0001-706X(90)90033-V
Qiu W., Guo K., Yi L., Gong Y., Huang L., Zhong W. (2014). Resolvin E1 reduces hepatic fibrosis in mice with Schistosoma japonicum infection. Exp. Ther. Med. 7 (6), 1481–1485. doi: 10.3892/etm.2014.1641
Raizel R., Leite J. S., Hypolito T. M., Coqueiro A. Y., Newsholme P., Cruzat V. F., et al. (2016). Determination of the anti-inflammatory and cytoprotective effects of l-glutamine and l-alanine, or dipeptide, supplementation in rats submitted to resistance exercise. Br. J. Nutr. 116 (3), 470–479. doi: 10.1017/S0007114516001999
Ramaswamy K., Kumar P., He Y. X. (2000). A role for parasite-induced PGE2 in IL-10-mediated host immunoregulation by skin stage schistosomula of schistosoma mansoni. J. Immunol. 165 (8), 4567–4574. doi: 10.4049/jimmunol.165.8.4567
Rodrigues H. G., Vinolo M. A. R., Magdalon J., Vitzel K., Nachbar R. T., Pessoa A. F. M., et al. (2012). Oral administration of oleic or linoleic acid accelerates the inflammatory phase of wound healing. J. Invest. Dermatol. 132 (1), 208–215. doi: 10.1038/jid.2011.265
Rook G. A. (2007). The hygiene hypothesis and the increasing prevalence of chronic inflammatory disorders. Trans. R Soc. Trop. Med. Hyg. 101 (11), 1072–1074. doi: 10.1016/j.trstmh.2007.05.014
Rook G. A. (2012). Hygiene hypothesis and autoimmune diseases. Clin. Rev. Allergy Immunol. 42 (1), 5–15. doi: 10.1007/s12016-011-8285-8
Rook G. A. (2013). Regulation of the immune system by biodiversity from the natural environment: an ecosystem service essential to health. Proc. Natl. Acad. Sci. 110 (46), 18360–18367. doi: 10.1073/pnas.1313731110
Rook G. A., Raison C. L., Lowry C. A. (2014). Microbial 'old friends', immunoregulation and socioeconomic status. Clin. Exp. Immunol. 177 (1), 1–12. doi: 10.1111/cei.12269
Rosa B. A., Supali T., Gankpala L., Djuardi Y., Sartono E., Zhou Y., et al. (2018). Differential human gut microbiome assemblages during soil-transmitted helminth infections in Indonesia and Liberia. Microbiome. 6 (1), 1–19. doi: 10.1186/s40168-018-0416-5
Ruddigkeit L., van Deursen R., Blum L. C., Reymond J. L. (2012). Enumeration of 166 billion organic small molecules in the chemical universe database GDB-17. J. Chem. Inf Model. 52 (11), 2864–2875. doi: 10.1021/ci300415d
Ruyssers N. E., De Winter B. Y., De Man J. G., Loukas A., Herman A. G., Pelckmans P. A., et al. (2008). Worms and the treatment of inflammatory bowel disease: are molecules the answer? Clin. Dev. Immunol. 2008, 1–7. doi: 10.1155/2008/567314
Ryan S. M., Eichenberger R. M., Ruscher R., Giacomin P. R., Loukas A. (2020). Harnessing helminth-driven immunoregulation in the search for novel therapeutic modalities. PloS Pathog. 16 (5), 1–20. doi: 10.1371/journal.ppat.1008508
Rzepecka J., Coates M. L., Saggar M., Al-Riyami L., Coltherd J., Tay H. K., et al. (2014). Small molecule analogues of the immunomodulatory parasitic helminth product ES-62 have anti-allergy properties. Int. J. Parasitol. 44 (9), 669–674. doi: 10.1016/j.ijpara.2014.05.001
Safer D., Brenes M., Dunipace S., Schad S. (2007). Urocanic acid is a major chemoattractant for the skin-penetrating parasitic nematode strongyloides stercoralis. Proc. Natl. Acad. Sci. 104, 1627–1630. doi: 10.1073/pnas.0610193104
Sag D., Carling D., Stout R. D., Suttles J. (2008). Adenosine 5'-monophosphate-activated protein kinase promotes macrophage polarization to an anti-inflammatory functional phenotype. J. Immunol. 181 (12), 8633–8641. doi: 10.4049/jimmunol.181.12.8633
Sakoguchi H., Yoshihara A., Izumori K., Sato M. (2016). Screening of biologically active monosaccharides: Growth inhibitory effects of d-allose, d-talose, and l-idose against the nematode caenorhabditis elegans. Biosci. Biotechnol. Biochem. 80, 1058–1061. doi: 10.1080/09168451.2016.1146069
Salafsky B., Wang Y.-S., Kevin M. B., Hill H., Fusco A. C. (1984). The role of prostaglandins in cercarial (Schistosoma mansoni) response to free fatty acids. J. Parasitol. 70 (4), 584–591. doi: 10.2307/3281410
Salter J. P., Lim K. C., Hansell E., Hsieh I., McKerrow J. H. (2000). Schistosome invasion of human skin and degradation of dermal elastin are mediated by a single serine protease. J. Biol. Chem. 275 (49), 38667–38673. doi: 10.1074/jbc.M006997200
Sanchez A. L., Gabrie J. A., Rueda M. M., Mejia R. E., Bottazzi M. E., Canales M. (2014). A scoping review and prevalence analysis of soil-transmitted helminth infections in Honduras. PloS Negl. Trop. Dis. 8 (1), 1–15. doi: 10.1371/journal.pntd.0002653
Saric J., Li J. V., Utzinger J., Wang Y., Keiser J., Dirnhofer S., et al. (2010). Systems parasitology: effects of Fasciola hepatica on the neurochemical profile in the rat brain. Mol. Syst. Biol. 6, 1–10. doi: 10.1038/msb.2010.49
Saric J., Li J. V., Wang Y., Keiser J., Bundy J. G., Holmes E., et al. (2008). Metabolic profiling of an Echinostoma caproni infection in the mouse for biomarker discovery. PloS Negl. Trop. Dis. 2 (7), 1–15. doi: 10.1371/journal.pntd.0000254
Saxena R. N., Pendse V. K., Khanna N. K. (1984). Anti-inflammatory and analgesic properties of four amino-acids. Indian J. Physiol. Pharmacol. 28, 299–305.
Saz H. J. (1981). Energy metabolisms of parasitic helminths: adaptations to parasitism. Annu. Rev. Physiol. 43, 323–341. doi: 10.1146/annurev.ph.43.030181.001543
Schneeberger P. H. H., Coulibaly J. T., Panic G., Daubenberger C., Gueuning M., Frey J. E., et al. (2018). Investigations on the interplays between Schistosoma mansoni, praziquantel and the gut microbiome. Parasit Vectors. 11 (1), 1–12. doi: 10.1186/s13071-018-2739-2
Scholmerich J., Fellermann K., Seibold F. W., Rogler G., Langhorst J., Howaldt S., et al. (2017). A randomised, double-blind, placebo-controlled trial of Trichuris suis ova in active crohn's disease. J. Crohns Colitis. 11(4), 390–399. doi: 10.1093/ecco-jcc/jjw184
Selmer T., Andrei P. I. (2001). P-hydroxyphenylacetate decarboxylase from Clostridium difficile–a novel glycyl radical enzyme catalysing the formation of p-cresol. Eur. J. Biochem. 268, 1363–1372. doi: 10.1046/j.1432-1327.2001.02001.x
Shin J. L., Gardiner G. W., Deitel W., Kandel G. (2004). Does whipworm increase the pathogenicity of Campylobacter jejuni? Can. J. Gastroenterol. Hepatol. 18(3), 175–177. doi: 10.1155/2004/298064
Singhal N. K., Sternbach S., Fleming S., Alkhayer K., Shelestak J., Popescu D., et al. (2020). Betaine restores epigenetic control and supports neuronal mitochondria in the cuprizone mouse model of multiple sclerosis. Epigenetics, 15(8), 871–886. doi: 10.1080/15592294.2020.1735075
Smith V. P., Selkirk M. E., Gounaris K. (1996). Identification and composition of lipid classes in surface and somatic preparationss of adult brugia malayi. Mol. Biochem. Parasitol. 78, 105–116. doi: 10.1016/S0166-6851(96)02615-1
Smyth D. J., Harcus Y., White M. P. J., Gregory W. F., Nahler J., Stephens I., et al. (2018). TGF-beta mimic proteins form an extended gene family in the murine parasite heligmosomoides polygyrus. Int. J. Parasitol. 48 (5), 379–385. doi: 10.1016/j.ijpara.2017.12.004
Sokol H., Pigneur B., Watterlot L., Lakhdari O., Bermudez-Humaran L. G., Gratadoux J. J., et al. (2008). Faecalibacterium prausnitzii is an anti-inflammatory commensal bacterium identified by gut microbiota analysis of crohn disease patients. Proc. Natl. Acad. Sci. 105 (43), 16731–16736. doi: 10.1073/pnas.0804812105
Sotillo J., Robinson M. W., Kimber M. J., Cucher M., Ancarola M. E., Nejsum P., et al. (2020). The protein and microRNA cargo of extracellular vesicles from parasitic helminths - current status and research priorities. Int. J. Parasitol. 50 (9), 635–645. doi: 10.1016/j.ijpara.2020.04.010
Spreafico A., Millucci L., Ghezzi L., Geminiani M., Braconi D., Amato L., et al. (2013). Antioxidants inhibit SAA formation and pro-inflammatory cytokine release in a human cell model of alkaptonuria. Rheumatology. 52 (9), 1667–1673. doi: 10.1093/rheumatology/ket185
Stilling R. M., Bordenstein S. R., Dinan T. G., Cryan J. F. (2014). Friends with social benefits: host-microbe interactions as a driver of brain evolution and development? Front. Cell Infect. Microbiol. 4, 1–17. doi: 10.3389/fcimb.2014.00147
Stoltzfus J. D., Minot S., Berriman M., Nolan T. J., Lok J. B. (2012). RNAseq analysis of the parasitic nematode Strongyloides stercoralis reveals divergent regulation of canonical dauer pathways. PloS Negl. Trop. Dis. 6 (10), 1–24. doi: 10.1371/journal.pntd.0001854
Strachan D. P. (1989). Hay fever, hygiene, and household size. Br. Med. J. 299, 1259–1260. doi: 10.1136/bmj.299.6710.1259
Su C. W., Chen C. Y., Jiao L., Long S. R., Mao T., Ji Q., et al. (2020). Helminth-induced and Th2-dependent alterations of the gut microbiota attenuate obesity caused by high-fat diet. Cell Mol. Gastroenterol. Hepatol. 10 (4), 763–778. doi: 10.1016/j.jcmgh.2020.06.010
Su C. W., Chen C. Y., Li Y., Long S. R., Massey W., Kumar D. V., et al. (2018). Helminth infection protects against high fat diet-induced obesity via induction of alternatively activated macrophages. Sci. Rep. 8 (1), 1–14. doi: 10.1038/s41598-018-22920-7
Sultan S., El-Mowafy M., Elgaml A., Ahmed T. A. E., Hassan H., Mottawea W. (2021). Metabolic influences of gut microbiota dysbiosis on inflammatory bowel disease. Front. Physiol. 12, 1–27. doi: 10.3389/fphys.2021.715506
Summers R. (2003). Trichuris suis seems to be safe and possibly effective in the treatment of inflammatory bowel disease. Am. J. Gastroenterol. 98 (9), 2034–2041. doi: 10.1111/j.1572-0241.2003.07660.x
Summers R. W., Elliott D. E., Urban J. F. Jr., Thompson R., Weinstock J. V. (2005). Trichuris suis therapy in crohn's disease. Gut. 54 (1), 87–90. doi: 10.1136/gut.2004.041749
Sun X. M., Guo K., Hao C. Y., Zhan B., Huang J. J., Zhu X. (2019). Trichinella spiralis excretory-secretory products stimulate host regulatory T cell differentiation through activating dendritic cells. Cells. 8 (11), 1–19. doi: 10.3390/cells8111404
Sun R., Zhao X., Wang Z., Yang J., Zhao L., Zhan B., et al. (2015). Trichinella spiralis paramyosin binds human complement C1q and inhibits classical complement activation. PloS Negl. Trop. Dis. 9 (12), 1–14. doi: 10.1371/journal.pntd.0004310
Szel E., Polyanka H., Szabo K., Hartmann P., Degovics D., Balazs B., et al. (2015). Anti-irritant and anti-inflammatory effects of glycerol and xylitol in sodium lauryl sulphate-induced acute irritation. J. Eur. Acad. Dermatol. Venereol. 29 (12), 2333–2341. doi: 10.1111/jdv.13225
Tang X., Liu J., Dong W., Li P., Li L., Lin C., et al. (2013). The cardioprotective effects of citric acid and l-malic acid on myocardial ischemia/reperfusion injury. Evid Based Complement Altern. Med. 2013, 1–11. doi: 10.1155/2013/820695
Tang B., Liu M., Wang L., Yu S., Shi H., Boireau P., et al. (2015). Characterisation of a high-frequency gene encoding a strongly antigenic cystatin-like protein from Trichinella spiralis at its early invasion stage. Parasit Vectors. 8, 1–12. doi: 10.1186/s13071-015-0689-5
Tedelind S., Westberg F., Kjerrulf M., Vidal A. (2007). Anti-inflammatory properties of the short-chain fatty acids acetate and propionate: A study with relevance to inflammatory bowel disease. World J. Gastroenterol. 13 (20), 2826–2832. doi: 10.3748/wjg.v13.i20.2826
Ullah H., Qadeer A., Rashid M., Rashid M. I., Cheng G. (2020). Recent advances in nucleic acid-based methods for detection of helminth infections and the perspective of biosensors for future development. Parasitology. 147 (4), 383–392. doi: 10.1017/S0031182019001665
Ullman B., Gudas L. J., Cohen A., Martin D. W. (1978). Deoxyadenosine metabolism and cytotoxicity in cultured mouse T lymphoma cells: A model for immunodeficiency disease. Cell. 14, 365–375. doi: 10.1016/0092-8674(78)90122-8
Unnikrishnan M. K., Rao M. N. A. (1990). Anti-inflammatory activity of methionine, methionine sulfoxide and methionine sulfone. Agents Actions. 31, 110–112. doi: 10.1007/BF02003229
Van de Merwe J. P., Schroder A. M., Wensinck F., Hazenberg M. P. (1988). The obligate anaerobic faecal flora of patients with crohn’s disease and their first-degree relatives. Scand. J. Gastroenterol. 23, 1125–1131. doi: 10.3109/00365528809090179
Varyani F., Fleming J. O., Maizels R. M. (2017). Helminths in the gastrointestinal tract as modulators of immunity and pathology. Am. J. Physiol. Gastrointest Liver Physiol. 312 (6), G537–GG49. doi: 10.1152/ajpgi.00024.2017
Velvizhi S., Nagalashmi T., Essa M. M., Dakshayani K. B., Subramanian P. (2002). Effects of alpha-ketoglutarate on lipid peroxidation and antioxidant status during chronic ethnol administration in wistar rats. Pol. J. Pharmacol. 54, 231–236.
Versini M., Jeandel P. Y., Bashi T., Bizzaro G., Blank M., Shoenfeld Y. (2015). Unraveling the hygiene hypothesis of helminthes and autoimmunity: origins, pathophysiology, and clinical applications. BMC Med. 13, 1–16. doi: 10.1186/s12916-015-0306-7
Voldsgaard A., Bager P., Garde E., Akeson P., Leffers A. M., Madsen C. G., et al. (2015). Trichuris suis ova therapy in relapsing multiple sclerosis is safe but without signals of beneficial effect. Mult Scler. 21 (13), 1723–1729. doi: 10.1177/1352458514568173
von Moltke J., Ji M., Liang H. E., Locksley R. M. (2016). Tuft-cell-derived IL-25 regulates an intestinal ILC2-epithelial response circuit. Nature. 529 (7585), 221–225. doi: 10.1038/nature16161
Vos T., Flaxman A. D., Naghavi M., Lozano R., Michaud C., Ezzati M., et al. (2012). Years lived with disability (YLDs) for 1160 sequelae of 289 diseases and injuries 1990–2010: a systematic analysis for the global burden of disease study 2010. Lancet 380 (9859), 2163–2196. doi: 10.1016/S0140-6736(12)61729-2
Wang Z., Cheong M. C., Tsien J., Deng H., Qin T., Stoltzfus J. D., et al. (2021). Characterization of the endogenous DAF-12 ligand and its use as an anthelmintic agent in strongyloides stercoralis. Elife 10, 1–25. doi: 10.7554/eLife.73535
Wangchuk P., Anderson D., Yeshi K., Loukas A. (2021). Identification of small molecules of the infective stage of human hookworm using LCMS-based metabolomics and lipidomics protocols. ACS Infect. Dis. 7 (12), 3264–3276. doi: 10.1021/acsinfecdis.1c00428
Wangchuk P., Constantinoiu C., Eichenberger R. M., Field M., Loukas A. (2019). Characterization of tapeworm metabolites and their reported biological activities. Molecules. 24 (8), 1–13. doi: 10.3390/molecules24081480
Wangchuk P., Kouremenos K., Eichenberger R. M., Pearson M., Susianto A., Wishart D. S., et al. (2019). Metabolomic profiling of the excretory-secretory products of hookworm and whipworm. Metabolomics. 15 (7), 1–15. doi: 10.1007/s11306-019-1561-y
Wangchuk P., Lavers O., Wishart D. S., Loukas A. (2020). Excretory/secretory metabolome of the zoonotic roundworm parasite toxocara canis. Biomolecules 10, 1–18. doi: 10.3390/biom10081157
Wangchuk P., Shepherd C., Constantinoiu C., Ryan R. Y. M., Kouremenos K. A., Becker L., et al. (2019). Hookworm-derived metabolites suppress pathology in a mouse model of colitis and inhibit secretion of key inflammatory cytokines in primary human leukocytes. Infect. Immun. 87 (4), 1–19. doi: 10.1128/IAI.00851-18
Wang Z., Hao C., Zhuang Q., Zhan B., Sun X., Huang J., et al. (2020). Excretory/secretory products from Trichinella spiralis adult worms attenuated DSS-induced colitis in mice by driving PD-1-mediated M2 acrophage polarization. Front. Immunol. 11, 1–12. doi: 10.3389/fimmu.2020.563784
Wang Y., Holmes E., Nicholson J. K., Cloarec O., Chollet J., Tanner M., et al. (2004). Metabonomic investigations in mice infected with Schistosoma mansoni: an approach for biomarker identification. Proc. Natl. Acad. Sci. 101 (34), 12676–12681. doi: 10.1073/pnas.0404878101
Wang T., Nie S., Ma G., Korhonen P. K., Koehler A. V., Ang C. S., et al. (2018). The developmental lipidome of haemonchus contortus. Int. J. Parasitol. 48 (12), 887–895. doi: 10.1016/j.ijpara.2018.06.002
Wang T., Nie S., Ma G., Vlaminck J., Geldhof P., Williamson N. A., et al. (2020). Quantitative lipidomic analysis of ascaris suum. PloS Negl. Trop. Dis. 14 (12), 1–19. doi: 10.1371/journal.pntd.0008848
Wang Y., Utzinger J., Xiao S. H., Xue J., Nicholson J. K., Tanner M., et al. (2006). System level metabolic effects of a Schistosoma japonicum infection in the Syrian hamster. Mol. Biochem. Parasitol. 146 (1), 1–9. doi: 10.1016/j.molbiopara.2005.10.010
Wang Y., Xiao S.-H., Xue J., Singer B. H., Utzinger J., Holmes E. (2009). Systems metabolic effects of a Necator americanus infection in Syrian hamster. J. Proteome Res. 8, 5442–5450. doi: 10.1021/pr900711j
Wang Z., Zhou X. E., Motola D. L., Gao X., Suino-Powell K., Conneely A., et al. (2009). Identification of the nuclear receptor DAF-12 as a therapeutic target in parasitic nematodes. Proc. Natl. Acad. Sci. 106 (23), 9138–9143. doi: 10.1073/pnas.0904064106
Ward P. F. (1982). Aspects of helminth metabolism. Parasitology. 84, 177–194. doi: 10.1017/S0031182000051775
Weinstock J. V., Elliott D. E. (2009). Helminths and the IBD hygiene hypothesis. Inflammation Bowel Dis. 15 (1), 128–133. doi: 10.1002/ibd.20633
Werkman M., Wright J. E., Truscott J. E., Easton A. V., Oliveira R. G., Toor J., et al. (2018). Testing for soil-transmitted helminth transmission elimination: Analysing the impact of the sensitivity of different diagnostic tools. PloS Negl. Trop. Dis. 12 (1), 1–20. doi: 10.1371/journal.pntd.0006114
Wewer V., Makepeace B. L., Tanya V. N., Peisker H., Pfarr K., Hoerauf A., et al. (2017). Lipid profiling of the filarial nematodes Onchocerca volvulus, Onchocerca ochengi and Litomosoides sigmodontis reveals the accumulation of nematode-specific ether phospholipids in the host. Int. J. Parasitol. 47 (14), 903–912. doi: 10.1016/j.ijpara.2017.06.001
Wewer V., Peisker H., Gutbrod K., Al-Bahra M., Menche D., Amambo N. G., et al. (2021). Urine metabolites for the identification of Onchocerca volvulus infections in patients from Cameroon. Parasit Vectors. 14 (1), 1–11. doi: 10.1186/s13071-021-04893-1
Whitman J. D., Sakanari J. A., Mitreva M. (2021). Areas of metabolomic exploration for helminth infections. ACS Infect. Dis. 7 (2), 206–214. doi: 10.1021/acsinfecdis.0c00658
WHO (2020). Available at: https://www.who.int/news-room/fact-sheets/detail/soil-transmitted-helminth-infections.
Winter A. N., Brenner M. C., Punessen N., Snodgrass M., Byars C., Arora Y., et al. (2017). Comparison of the neuroprotective and anti-inflammatory effects of the anthocyanin metabolites, protocatechuic acid and 4-hydroxybenzoic acid. Oxid. Med. Cell Longev. 2017, 1–13. doi: 10.1155/2017/6297080
Wishart D. S., Feunang Y. D., Marcu A., Guo A. C., Liang K., Vazquez-Fresno R., et al. (2018). HMDB 4.0: The human metabolome database for 2018. Nucleic Acids Res. 46 (D1), D608–DD17. doi: 10.1093/nar/gkx1089
Wishart D. S., Knox C., Guo A. C., Eisner R., Young N., Gautam B., et al. (2009). HMDB: a knowledgebase for the human metabolome. Nucleic Acids Res. 37 (Database issue), D603–D610. doi: 10.1093/nar/gkn810
Wu J., He C., Bu J., Luo Y., Yang S., Ye C., et al. (2020). Betaine attenuates LPS-induced downregulation of occludin and claudin-1 and restores intestinal barrier function. BMC Vet. Res. 16 (1), 1–8. doi: 10.1186/s12917-020-02298-3
Wu J. F., Holmes E., Xue J., Xiao S. H., Singer B. H., Tang H. R., et al. (2010). Metabolic alterations in the hamster co-infected with Schistosoma japonicum and necator americanus. Int. J. Parasitol. 40 (6), 695–703. doi: 10.1016/j.ijpara.2009.11.003
Wu T., Wang C., Ding L., Shen Y., Cui H., Wang M., et al. (2016). Arginine relieves the inflammatory response and enhances the casein expression in bovine mammary epithelial cells induced by lipopolysaccharide. Mediators Inflamm. 2016, 1–10. doi: 10.1155/2016/8696481
Wu J., Xu W., Ming Z., Dong H., Tang H., Wang Y. (2010). Metabolic changes reveal the development of schistosomiasis in mice. PloS Negl. Trop. Dis. 4 (8), 1–11. doi: 10.1371/journal.pntd.0000807
Yamaguchi H., Maruyama T., Urade Y., Nagata S. (2014). Immunosuppression via F adenosine receptor activation by adenosine monophosphate released from apoptotic cells. elife. 3, 1–15. doi: 10.7554/eLife.02172
Yang D., Zhang Y., Nguyen H. G., Koupenova M., Chauhan A. K., Makitalo M., et al. (2006). The A2B adenosine receptor protects against inflammation and excessive vascular adhesion. J. Clin. Invest. 116 (7), 1913–1923. doi: 10.1172/JCI27933
Yeshi K., Creek D. J., Anderson D., Ritmejerytė E., Becker L., Loukas A., et al. (2020). Metabolomes and lipidomes of the infective stages of the gastrointestinal nematodes, Nippostrongylus brasiliensis and trichuris muris. Metabolites 10 (11), 1–39. doi: 10.3390/jcm9051273
Yeshi K., Ruscher R., Hunter L., Daly N. L., Loukas A., Wangchuk P. (2020). Revisiting inflammatory bowel disease: Pathology, treatments, challenges and emerging therapeutics including drug leads from natural products. J. Clin. Med. 9 (5), 1–39. doi: 10.3390/jcm9051273
Yue Y., Guo Y., Yang Y. (2017). Effects of dietary l-tryptophan supplementation on intestinal response to chronic unpredictable stress in broilers. Amino Acids 49 (7), 1227–1236. doi: 10.1007/s00726-017-2424-3
Zaiss M. M., Rapin A., Lebon L., Dubey L. K., Mosconi I., Sarter K., et al. (2015). The intestinal microbiota contributes to the ability of helminths to modulate allergic inflammation. Immunity. 43 (5), 998–1010. doi: 10.1016/j.immuni.2015.09.012
Zakeri A., Hansen E. P., Andersen S. D., Williams A. R., Nejsum P. (2018). Immunomodulation by helminths: Intracellular pathways and extracellular vesicles. Front. Immunol. 9, 1–21. doi: 10.3389/fimmu.2018.02349
Zhang B., Gems D. (2021). Gross ways to live long: Parasitic worms as an anti-inflammaging therapy? Elife 10, 1–12. doi: 10.7554/eLife.65180
Zhao L., Shao S., Chen Y., Sun X., Sun R., Huang J., et al. (2017). Trichinella spiralis calreticulin binds human complement C1q as an immune evasion strategy. Front. Immunol. 8, 1–15. doi: 10.3389/fimmu.2017.00636
Zheng W. B., Zou Y., Elsheikha H. M., Liu G. H., Hu M. H., Wang S. L., et al. (2019). Serum metabolomic alterations in beagle dogs experimentally infected with toxocara canis. Parasit Vectors. 12 (1), 1–10. doi: 10.1186/s13071-019-3703-5
Zhi X., Zhou X. E., Melcher K., Motola D. L., Gelmedin V., Hawdon J., et al. (2012). Structural conservation of ligand binding reveals a bile acid-like signaling pathway in nematodes. J. Biol. Chem. 287 (7), 4894–4903. doi: 10.1074/jbc.M111.315242
Zhu H. L., Liu Y. L., Xie X. L., Huang J. J., Hou Y. Q. (2013). Effect of l-arginine on intestinal mucosal immune barrier function in weaned pigs after Escherichia coli LPS challenge. Innate Immun. 19, 242–252. doi: 10.1177/1753425912456223
Keywords: helminths, immunomodulatory, small molecules, excretory-secretory products, biological activities, pharmacological properties, metabolomics
Citation: Yeshi K, Ruscher R, Loukas A and Wangchuk P (2022) Immunomodulatory and biological properties of helminth-derived small molecules: Potential applications in diagnostics and therapeutics. Front. Parasitol. 1:984152. doi: 10.3389/fpara.2022.984152
Received: 01 July 2022; Accepted: 23 August 2022;
Published: 09 September 2022.
Edited by:
Javier Sotillo, Instituto de Salud Carlos III (ISCIII), SpainReviewed by:
Tao Wang, The University of Melbourne, AustraliaDalia Ashour, Tanta University, Egypt
Alba Cortés, University of Valencia, Spain
Copyright © 2022 Yeshi, Ruscher, Loukas and Wangchuk. This is an open-access article distributed under the terms of the Creative Commons Attribution License (CC BY). The use, distribution or reproduction in other forums is permitted, provided the original author(s) and the copyright owner(s) are credited and that the original publication in this journal is cited, in accordance with accepted academic practice. No use, distribution or reproduction is permitted which does not comply with these terms.
*Correspondence: Karma Yeshi, karma.yeshi@my.jcu.edu.au