- 1Institute for Sustainable Plant Protection, National Research Council (CNR), Bari, Italy
- 2Department of Agricultural Sciences, University of Naples Federico II, Portici, Italy
- 3Formerly at Institute of Sustainable Plant Protection, CNR, Bari, Italy
- 4Department of Veterinary Medicine and Animal Production, University of Naples Federico II, Naples, Italy
- 5Department of Veterinary Medicine and Animal Production, University of Naples, Regional Centre for Monitoring of Parasitosis (CREMOPAR), Eboli, Italy
FLOTAC Techniques have been widely acknowledged as an effective method for the extraction of human and animal parasites. The present study is the first application of FLOTAC basic technique (FBT) for the extraction of phytoparasitic nematodes from soil and infested plant roots. Eggs and second stage juveniles (J2) of the root-knot nematode Meloidogyne incognita were extracted from infested soil and tomato roots either by FBT and conventional nematode extraction methods, such as centrifugal flotation and root maceration techniques, respectively. The number of M. incognita J2 and eggs extracted from soil by FBT was always significantly higher compared to the extraction with the centrifugal flotation method, averaging 277 vs 35 eggs and J2 mL-1 soil. Conversely, no significant differences were observed between FBT and the root maceration technique in the extraction of eggs and J2 from tomato roots. Results demonstrated that FBT can be highly effective also for the extraction of phytoparasitic nematodes. Due to its accuracy and sensitivity, FBT seems particularly suitable for nematode surveys in wide geographical areas, where an accurate and rapid detection of present phytoparasitic nematofauna is required.
1 Introduction
Plant parasitic nematodes are recognized as a major threat to world agriculture, as causing heavy crop yield losses, estimated as exceeding 157 billion dollars/year at world level (Nicol et al., 2011). Most of these losses are due to root-knot nematodes of the genus Meloidogyne Goeldi, as highly destructive pests of a wide range of herbaceous and woody crops (Jones et al., 2013). These damages are expected to furtherly increase in the future, due to world economic globalization and climate changes, which will favor the introduction of alien species and the intensification of attacks of endemic pests (Gregory et al., 2009). In this scenario, monitoring soil phytoparasitic nematode populations is necessary either to minimize the impact of alien species on agricultural ecosystems, and to prevent a further spread of endemic crop nematode pests (Singh et al., 2013).
A careful assessment of soil phytonematode population densities is needed to predict the potential yield losses and to select appropriate management strategies (Seinhorst, 1986; Sasanelli et al., 2018; Sasanelli et al., 2021). An accurate quanti-qualitative assessment of soil nematophauna is also basic to ecological studies, as soil nematodes are reliable bioindicators of environmental impact of biotic and abiotic stress factors (Bongers and Ferris, 1999; Du Preez et al., 2022). Based on the above considerations, the choice of effective and accurate nematode extraction techniques is a key point for scientific studies as well as for extension services.
Nematode extraction methods vary according to substrates to process (soil or plant tissues), nematode size, feeding habitus (ecto- or endoparasite) and biology (free living, root-knot or cyst-forming species). Extraction techniques include decanting and sieving, incubation and centrifugal flotation procedures (Table 1) (Hooper et al., 2005). These methods are often combined to overcome weaknesses of each individual technique. Cobb’s sieving and decanting method (Cobb, 1918) and the Baermann’s funnel incubation (Baermann, 1917) methods have a general application for nematode extraction from soil samples, while Coolen’s centrifugal flotation method (Coolen, 1979) and the Hussey and Barker’s root maceration technique (Hussey and Barker, 1973) are more frequently used for the extraction of different stages (juveniles, adults and eggs) of root knot nematodes (Meloidogyne species) from soil and roots, respectively. In addition, the Fenwick’s can method (Fenwick, 1940) is specifically addressed to the extraction of egg-containing cysts of cyst-nematode species (Heterodera spp, Globodera spp). All these techniques are efficient but are time and labour consuming, as not allowing routine use in laboratories processing large amounts of samples per day or a direct analysis of samples in field.
In the last two decades, Cringoli et al. (2010) developed and validated a quali-quantitative techniques (FLOTAC basic, dual and double techniques) for a rapid detection and count of parasitic elements (cysts, oocysts, eggs and larvae) in animals and humans. These methods make use of the FLOTAC apparatus, a cylindrical device with two 5-ml flotation chambers (Figure 1). The FLOTAC techniques are based on centrifugal flotation of sample followed by removal of floating suspension and have been repeatedly proven as multivalent, sensitive, specific, accurate, precise and highly reproducible, recently demonstrating an optimal efficiency also for investigating the presence of parasitic elements in fresh salad and fruits (Barlaam et al., 2021; Cringoli et al., 2021; Barlaam et al., 2022). Due to its technical characteristics, FLOTAC could be potentially used also for the extraction of eggs and other life stages of phytoparasitic nematodes both from plant roots and soil. The aim of this study was to evaluate the efficiency of FLOTAC basic technique (FBT) for the extraction of root-knot nematodes from soil and plant roots, in comparison with two methods currently used in nematology labs, i.e. the centrifugal flotation and the root maceration technique, respectively.
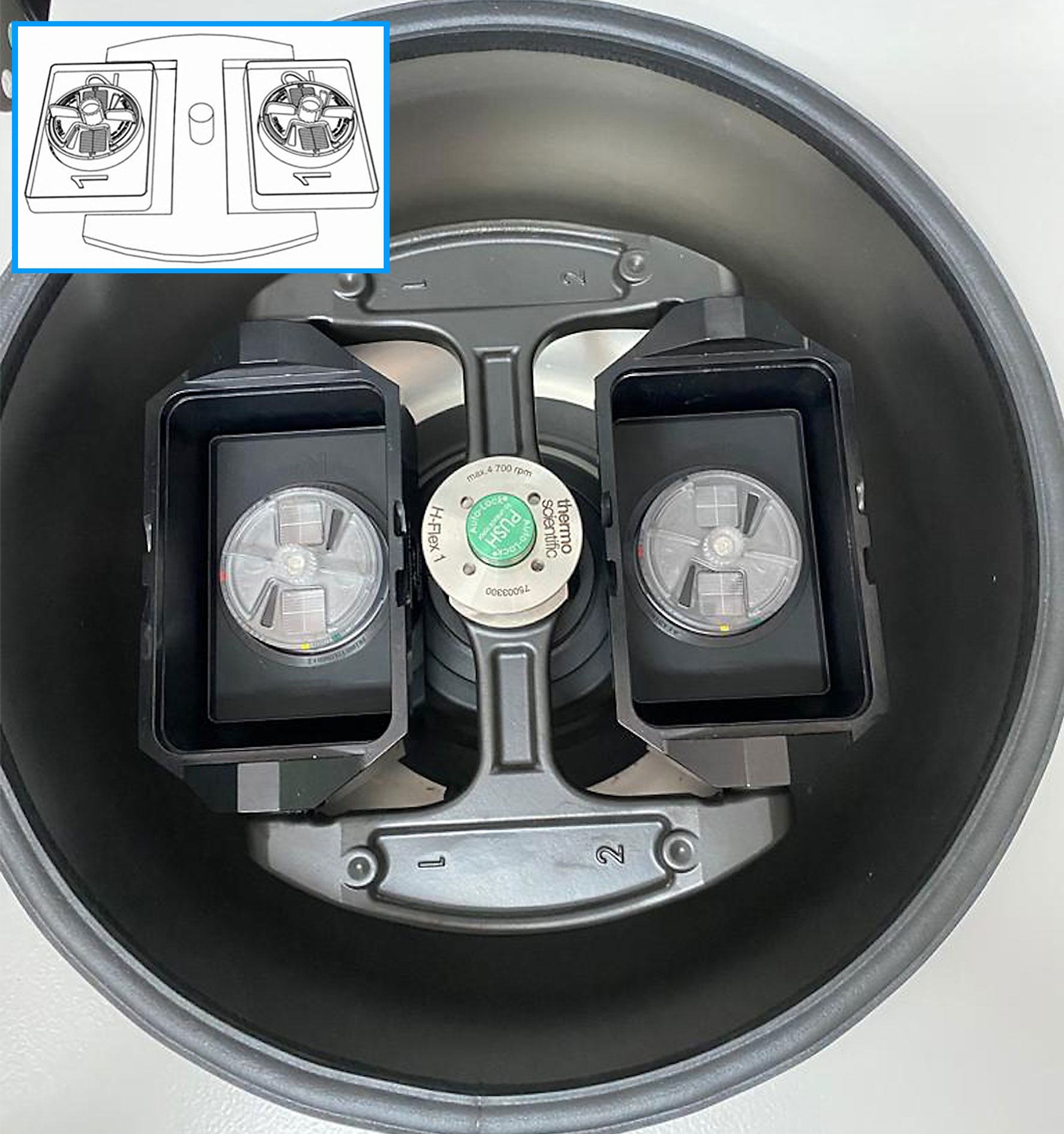
Figure 1 FLOTAC apparatus in a benchtop centrifuge with rotor for microtitre plates. The insert shows a schematic drawing of FLOTAC devise.
2 Materials and methods
2.1 Nematode population
An Italian population of the root-knot nematode Meloidogyne incognita (Kofoid and White) Chitw, previously identified by morphometrical parameters, was reared on tomato (Solanum lycopersicum L.) cv Regina di Fasano for two months in a glasshouse at 25 ± 2°C. The infested tomato roots were minutely comminuted, thoroughly mixed and then used to artificially infest soil or directly addressed to the comparison of root extraction techniques.
2.2 Preparation of soil and root samples
The infested sandy soil (pH 7.2; sand >98%; silt <1%; clay <1%; organic matter = 0.75%) from the glasshouse multiplication of M. incognita was thoroughly mixed in a concrete mixer and enriched with the infested root inoculum. The soil mixture was then divided into twenty-four 500 mL samples used for the comparative evaluation of the two extraction methods (Coolen’s method vs FLOTAC basic technique).
The infested tomato roots were subdivided into 24 samples of about 5 g each used for eggs and juveniles extraction by the two methods in comparison.
2.3 Nematodes extraction from soil
2.3.1 Extraction by the Coolen’s method
Three series of four 500 mL soil samples were thoroughly stirred in four buckets with 1 L of water each and filtered through a 2 mm sieve, recovering each soil suspension in a tube of the Coolen’s apparatus (Figure 2). In the extraction from soil, infested by the root-knot nematode M. incognita, the addition of 100 mL of a 5% sodium hypochlorite water solution and few mL of a wetting agent (silicon antifoam 2%) was also provided, in order to dissolve nematode egg mass gelatinous matrix. Tubes were then filled with water up to a 2.5 L volume and stirred by pressured air for 5 min. A 250 mL volume of the soil suspension was then collected and poured into a metallic centrifuge tube, previously added with 5 mL of kaolin powder, and then filled with water up to a 0.5 L volume and thoroughly stirred. The four metallic tubes were then centrifuged at 2,000 rpm for 5 min, after which the supernatant was discarded. The gelatinous residue retained on the bottom of the tube was re-suspended with 400 mL of a magnesium sulphate solution (1.165 specific gravity), stirred and newly centrifuged at 2,000 rpm for 5 min. The supernatant was then poured into plexiglass 22 μm sieve and eggs and J2 were recovered for microscopical count. Nematodes were counted using a stereo microscope at 20x magnification and their total number was recorded.
2.3.2 Extraction by the FLOTAC basic technique - soil
The 11 operating steps of the FLOTAC basic technique are summarized in Figure 3. Soil sample was sieved with a wire mesh (aperture of 2 mm), then 50 g were weighed. A 6% sodium hypochlorite water solution (450 mL) was added to the 50 g of soil to reach a volume of 500 mL (dilution ratio 1:10). The suspension was thoroughly homogenized (the use of a hand blender is suggested) and filtered through a wire mesh (aperture of 1mm). One aliquot of 11 mL of the filtered suspension was transferred into a conic tube. The tubes were centrifuged for 3 min at 170 g (1,500 rpm) at room temperature and after centrifugation, the supernatant was discarded, leaving only the sediment (pellets) in the tube. The tubes were filled with a magnesium sulphate solution (1.165 specific gravity) (Cringoli et al., 2010) up to the previous 11 mL level. The suspensions were thoroughly homogenized (before and between the filling) and the two flotation chambers of the FLOTAC apparatus (Figure 1) were filled. The FLOTAC apparatus was centrifuged for 5 min at 120 g (1,000 rpm) at room temperature, translated and examined under an optical microscope. The detection limit of this protocol (and multiplication factor) was 1 egg or J2 per gram of soil.
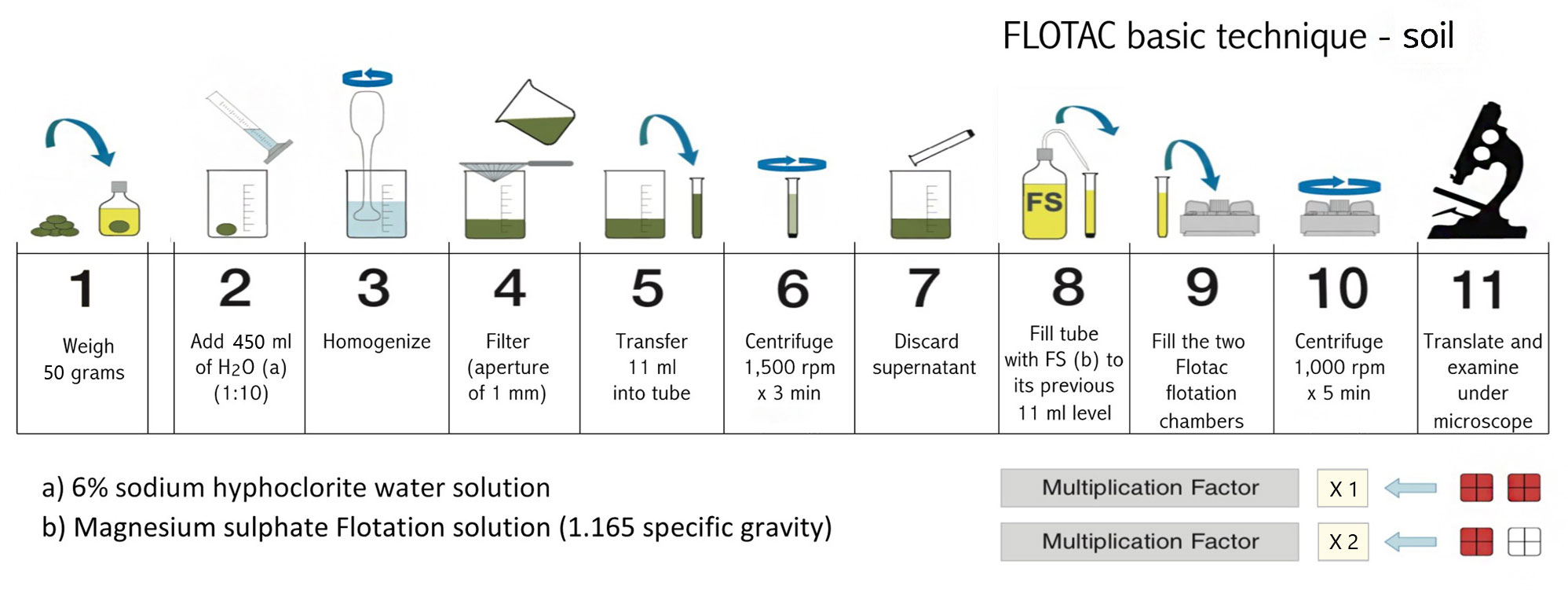
Figure 3 Schematic drawings showing the 11 operating steps of FLOTAC basic technique for the extraction of nematode from soil.
2.4 Nematode extraction from roots
2.4.1 Extraction by the Hussey and Barker’s method
Each root sample was grinded for 30 s in a blender containing 150 mL of a 1% aqueous sodium hypochlorite solution (Figure 4A). The water suspension was then sieved through a 250 μm and a 22 μm pore sieves (Figure 4B). Nematodes and root debris recovered on the 22 μm pore sieve were further centrifuged at 2,000 rpm for 5 min in 400 mL of a magnesium sulphate solution (1.165 specific gravity). The supernatant suspension was then poured onto a 22 μm pore sieve and eggs and J2 were recovered for microscopical count.
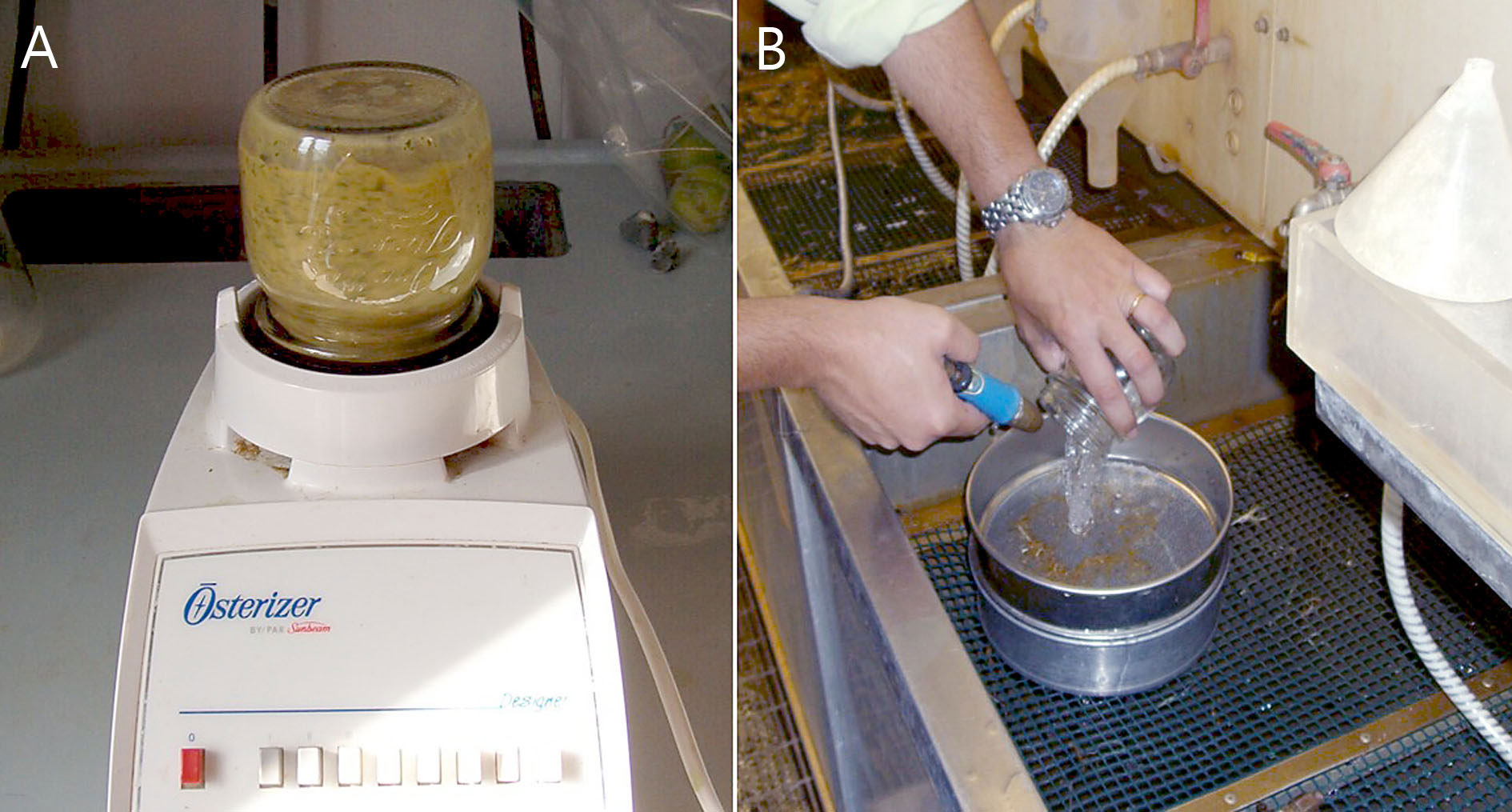
Figure 4 Extraction of eggs and J2 from roots by Hussey and Barker’s method. (A) Blender containing the root suspension; (B) water suspension containing roots poured through the 250 μm (top) and 22 μm (bottom) pore sieves.
2.4.2 Extraction by the FLOTAC basic technique
The 11 operating steps of the FLOTAC technique are summarized in Figure 5. Five grams of root sample were placed in the becker of a blender and a 6% sodium hypochlorite water solution was added up to the volume of 200 ml (a drop of a wetting agent was added) and grinded thoroughly. The suspension was filtered through a wire mesh (aperture of 1 mm) and another part of 6% sodium hypochlorite water solution (800 mL) was added, rinsing the blender and wire mesh, in order to obtain a final dilution ratio of 1:200. One aliquot of 11 mL of the filtered suspension was transferred into a conic tube and the same steps of the protocol previously described for soil samples were applied. Using this protocol, the detection limit (multiplication factor) was 20 eggs or J2 per gram of root.
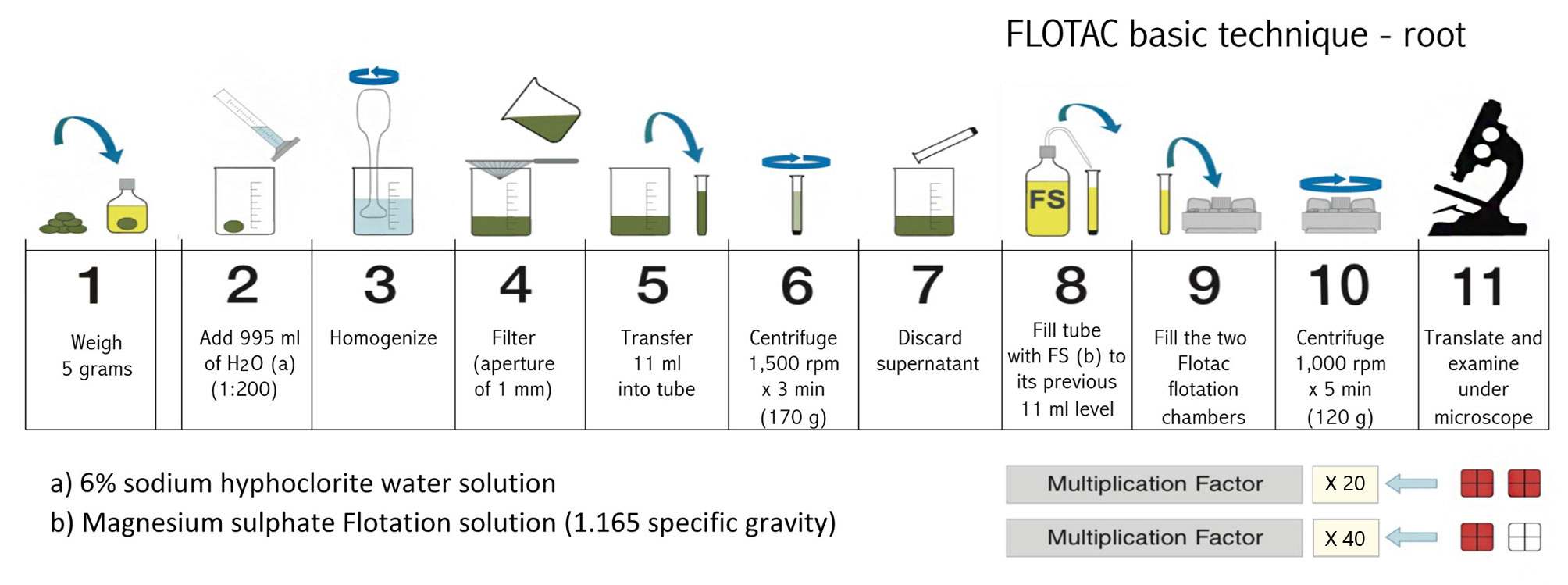
Figure 5 Schematic drawings showing the 11 operating steps of Flotac basic technique for the extraction of nematode from roots.
2.5 Statistical analysis
All the 12-sample soil and root extraction series were repeated twice and data from the two experimental runs were pooled in the absence of a significant experiment per treatment interactions (Finney, 1978). Data from the two extraction methods were statistically analyzed by analysis of variance (ANOVA) and means compared by the Student’s t test (P = 0.01). Statistical analyses were performed using the software Plot IT Vers. 3.2 (Scientific Programming Enterprises, Haslett, Mi, USA).
3 Results
The numbers of M. incognita J2 and eggs extracted from soil by FLOTAC basic technique (FBT) were always significantly higher compared to the extraction with the centrifugal flotation method (Table 2). In particular, FBT extracted a mean of 277 eggs and J2 mL-1 soil versus 35 eggs and J2 mL-1 soil recovered by centrifugal flotation, i.e. an 8-fold higher number. By contrast, no statistical differences occurred between the two methods in the extraction from tomato roots, averaging 20,361 and 20,474 eggs and j2 g-1 root for FBT and maceration-centrifugation, respectively, despite the presence of an aberrant replication in FBT data (9,423 eggs and J2 g-1 root) (Table 2). Values of Standard Deviation (SD) and Coefficient of Variation (CV) indicated a low variability of data among the replications from both methods.
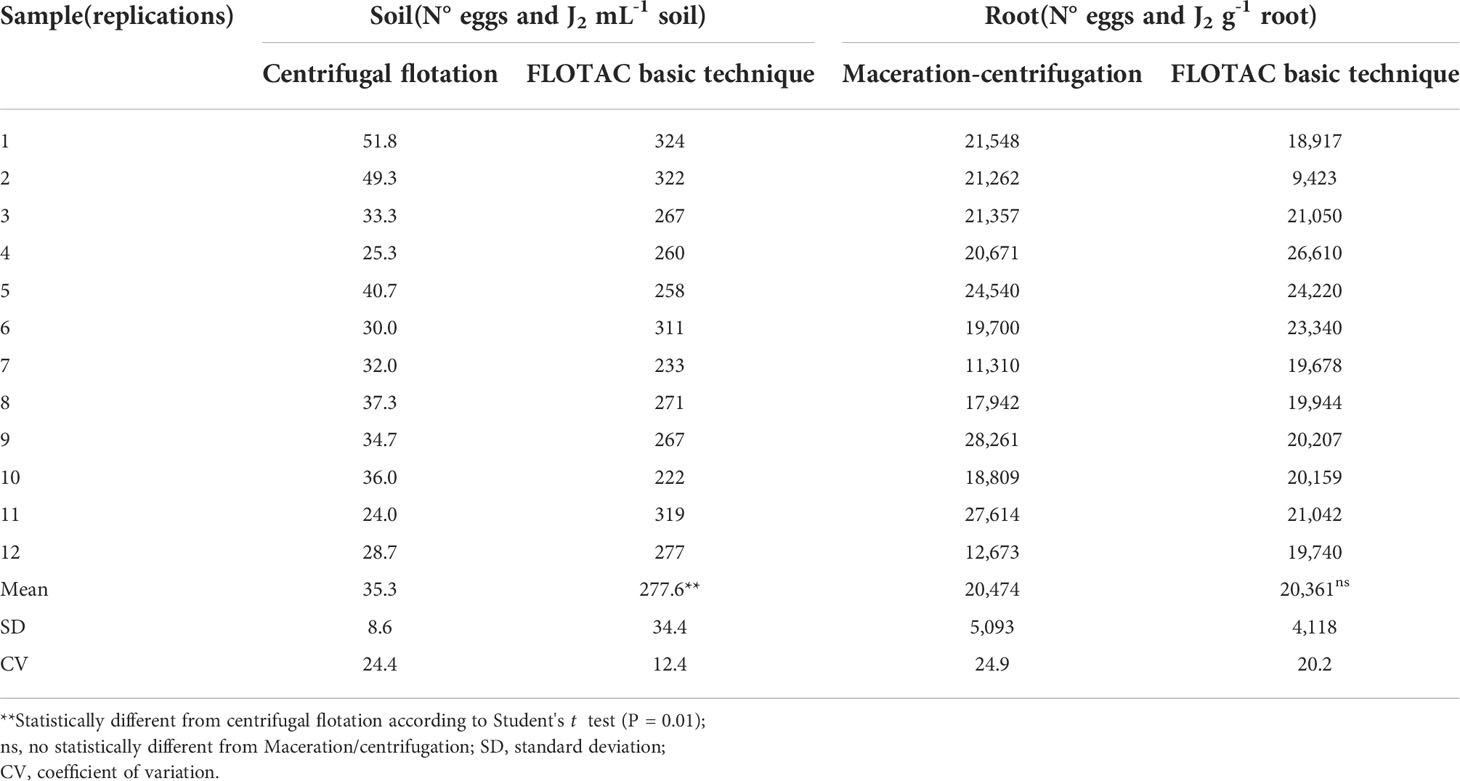
Table 2 Statistical comparison of number of M. incognita eggs and J2 recovered from 12 soil and 12 root samples extracted by the considered methodologies (Coolen’s method for soil; Hussey and Barker for roots and FLOTAC basic technique for soil and roots).
4 Discussion
The efficiency of any technique for nematode extraction from soil and plant materials results from a sum of parameters, such as sensitivity, accuracy and reproducibility of results (McSorley and Walter, 1991). High sensitivity and accuracy of the extraction method is essential to a prompt diagnosis of nematode infestation also in the presence of low population levels. Sensitive and accurate extraction tools are also crucial in ecological studies aimed to detect shift in composition of soil nematophauna related to biotic or abiotic disturbance factors. Reproducibility of extraction yield can ensure the comparability of nematode population data either throughout the time and among different treatments in comparison.
FLOTAC basic technique has been previously acknowledged as an effective method for a rapid and reliable diagnosis of human and veterinary infectious and parasitic diseases, due to its high sensitivity, precision and accuracy (Knopp et al., 2011; Levecke et al., 2012; Steinmann et al., 2012; Ramos et al., 2016).
In our experiments, FBT was demonstrated to be more effective than the centrifugal flotation method for the extraction of M. incognita J2 and eggs from soil, whereas its efficiency was not consistently higher in nematode extraction from roots. Furthermore, nematode suspensions resulting from both soil and root extraction by FBT were clearer and free of debris compared to those obtained with conventional methods, as allowing an easier microscopical observation.
Another plus point of FBT is the need of few laboratory infrastructures, as limited to a benchtop centrifuge and few consumables. Previous parasitological studies reported a rapid and reliable analysis of large numbers of animal samples even in the absence of centrifuges and other basic equipment by an evolution of FLOTAC, namely the mini-FLOTAC (Cringoli et al., 2017; Lozano et al., 2021).
However, extraction efficacy ofFBT is strongly affected by type and density of flotation solution, as largely varying among sucrose, magnesium sulphate and zinc sulphate, i.e. substances most commonly used (Coolen and D’Herde, 1977; Cringoli et al., 2010). In our pivotal experiments (data not showed), zinc sulphate provided a similar extraction efficiency compared to magnesium sulphate, but severely affected J2s morphology and, consequently, their microscopical identification in the presence of saprophytic nematode specimens.
Another factor to be considered is the soil composition. In our study, experiments were performed in sandy soil. However, as recovery of nematodes might show different results in detection based on the type of soil (i.e. clay, silty, silty-loamy, etc.), evaluation of Flotac extraction performance also in soil with different grain size should be assessed.
Based on the above considerations, FBT is strongly suggested for processing large number of soil samples from nematode surveys in wide geographical areas, where all the occurring phytoparasitic nematodes should be accurately detected. Other fields of application of FLOTAC could be represented by ecological studies, as requiring detection of the whole soil nematophauna, as well as by the extraction of entomopathogenic nematodes from insects for their application as biocontrol agents. Conversely, the huge amounts of root and soil samples usually coming from nematode control experiments can be more easily managed with the conventional extraction techniques, which allow to process more samples at the same time.
Data availability statement
The original contributions presented in the study are included in the article/supplementary material. Further inquiries can be directed to the corresponding author.
Author contributions
AT, TD, NS and GC designed the experiments. TD, NS, AB and GC analyzed the data. AT, TD, NS and GC drafted the manuscript with input from all the co-authors. AT, NS, Gd'E, AB and GC performed extraction experiments and microscopy analysis. GC supervised the project and arranged the funding. All authors contributed to the article and approved the submitted version.
Funding
The work was financially supported by the CREMOPAR.
Acknowledgment
Authors thanks Mr. Salatino N. and Mr. Catalano F. of IPSP-CNR for their technical assistance.
Conflict of interest
The authors declare that the research was conducted in the absence of any commercial or financial relationships that could be construed as a potential conflict of interest.
Publisher’s note
All claims expressed in this article are solely those of the authors and do not necessarily represent those of their affiliated organizations, or those of the publisher, the editors and the reviewers. Any product that may be evaluated in this article, or claim that may be made by its manufacturer, is not guaranteed or endorsed by the publisher.
References
Baermann G. (1917). Eine einfache methode zur auffindung von Ankylostomum (nematoden) larven in erdproben. Geneesk Tijdschr Ned-Indië 57, 131–137.
Barlaam A., Sannella A. R., Ferrari N., Temesgen T. T., Rinaldi L., Normanno G., et al. (2022). Ready-to-eat salads and berry fruits purchased in Italy contaminated by cryptosporidium spp., Giardia duodenalis, and Entamoeba histolytica. Int. J. Food Microbiol. 370, 109634. doi: 10.1016/j.ijfoodmicro.2022.109634
Barlaam A., Temesgen T. T., Tysnes K. R., Rinaldi L., Ferrari N., Sannella A. R., et al. (2021). Contamination of fresh produce sold on the Italian market with Cyclospora cayetanensis and Echinococcus multilocularis. Food Microbiol. 98, 103792. doi: 10.1016/j.fm.2021.103792
Bongers T., Ferris H. (1999). Nematode community structure as bioindicator in environmental monitoring. Trends Ecol. Evol. 14, 224–228. doi: 10.1016/s0169-5347(98)01583-3
Cobb N. A. (1918). Estimating the nematode population of the soil. Agric. Tech Circ. US Dept Agriculture. 48.
Coolen W. A. (1979). “Methods for the extraction of meloidogyne spp. and other nematodes from roots and soil,” in Root knot nematodes (Meloidogyne species), systematics, biology and control. Eds. Lamberti F., Taylor C. E. (London, UK: Academic Press), 317–329.
Coolen W. A., D'Herde C. J. (1977). Centrifugal separation of Longidorus and Xiphinema from soil using colloidal silica. Nematol Medit. 5, 195–206.
Cringoli G., Maurelli M. P., Levecke B., Bosco A., Vercruysse J., Utzinger J., et al. (2017). The mini-FLOTAC technique for the diagnosis of helminth and protozoan infections in humans and animals. Nat. Protoc. 12, 1723–1732. doi: 10.1038/nprot.2017.067
Cringoli G., Pepe P., Bosco A., Maurelli M. P., Baldi L., Ciaramella P., et al. (2021). An integrated approach to control cystic Echinococcosis in southern Italy. Vet. Parasitol. 290, 109347. doi: 10.1016/j.vetpar.2021.109347
Cringoli G., Rinaldi L., Maurelli M. P., Utzinger J. (2010). FLOTAC: new multivalent techniques for qualitative and quantitative copromicroscopic diagnosis of parasites in animals and humans. Nat. Protoc. 5, 503–515. doi: 10.1038/nprot.2009.235
Du Preez G., Daneel M., De Goede R., Du Toit M. J., Ferris H., Fourie H., et al. (2022). Nematode-based indices in soil ecology: Application, utility and future directions. Soil Biol. Biochem. 169, 1–14. doi: 10.1016/j.soilbio.2022.108640
Fenwick D. W. (1940). Methods for the recovery and counting of cysts of Heterodera schachtii from soil. J. Helminthol. 18, 155–172.
Finney D. J. (1978). Statistical method in biological assay. 3rd ed (London, UK: Charles Griffin & Company Ltd).
Gregory P. J., Johnson S. N., Newton A. C., Ingram J. S. (2009). Integrating pests and pathogens into the climate change/food security debate. J. Exp. Bot. 60, 2827–2838. doi: 10.1093/jxb/erp080
Hooper D. J., Hallmann J., Subbotin S. A. (2005). “Methods for extraction, processing and detection of plant and soil nematodes,” in Plant parasitic nematodes in subtropical and tropical agriculture, vol. Vol 2 . Eds. Luc M., Sikora R. A., Bridge J. (Wallingford: Cabi Publisher), 53–86.
Hussey R. S., Barker K. R. (1973). A comparison of methods of collecting inocula of Meloidogyne spp. including a new technique. Plant Dis. Reptr. 57, 1025–1028.
Jones J. T., Haegeman A., Danchin E. G. J., Gaur H. S., Helder J., Jones M. G. K., et al. (2013). Top 10 plant-parasitic nematodes in molecular plant pathology. Mol. Plant Pathol. 14, 946–961. doi: 10.1111/mpp.12057
Knopp S., Speich B., Hattendorf J., Rinaldi L., Mohammed K. A., Khamis I. S., et al. (2011). Diagnostic accuracy of kato-Katz and FLOTAC for assessing anthelmintic drug efficacy. PLoS Neg Trop. Dis. 5, e1036. doi: 10.1371/journal.pntd.0001036
Levecke B., Rinaldi L., Charlier J., Maurelli M. P., Bosco A., Vercruysse J., et al. (2012). The bias, accuracy and precision of faecal egg count reduction test results in cattle using McMaster, Cornell-Wisconsin and FLOTAC egg counting methods. Vet. Parasitol. 188, 194–199. doi: 10.1016/j.vetpar.2012.03.017
Lozano J., Almeida C., Victório A. C., Melo P., Rodrigues J. P., Rinaldi L., et al. (2021). Implementation of mini-FLOTAC in routine diagnosis of coccidia and helminth infections in domestic and exotic birds. Vet. Sci. 8, 160. doi: 10.3390/vetsci8080160
McSorley R., Walter D. E. (1991). Comparison of soil extraction methods for nematodes and microarthropods. Agric. Ecosyst. Environ. 34, 201–207. doi: 10.1016/0167-8809(91)90106-8
Nicol J. M., Turner S. J., Coyne D. L., den Nijs L., Hockland S., Maafi Z. T. (2011). “Current nematode threats to world agriculture,” in Genomics and molecular genetics of plant–nematode interactions. Eds. Jones J. T., Gheysen G., Fenoll C. (Heidelberg, Germany: Springer), 21–44. doi: 10.1007/978-94-007-0434-3_2
Ramos R. A. N., Lima V. F. S., Monteiro M. F. M., de Andrade Santana M., Lepold R., Faustino M. A. D. G., et al. (2016). New insights into diagnosis of Platynosomum fastosum (Trematoda: Dicrocoeliidae) in cats. Parasitol. Res. 115, 479–482. doi: 10.1007/s00436-015-4763-x
Sasanelli N., Konrat A., Migunova V., Toderas I., Iurcu-Straistaru E., Rusu S., et al. (2021). Review on control methods against plant parasitic nematodes applied in southern member states (C zone) of the European union. Agriculture 11, 602. doi: 10.3390/agriculture11070602
Sasanelli N., Toderas I., Iurcu-Straistaru E., Rusu S., Migunova V., Konrat A. (2018). “Yield losses caused by plant parasitic nematodes graphical estimation,” in International symposium “Functional ecology of animals” (Chisinau, Moldova: National Book Chamber of R. Moldova, Ed. Institute of Zoology), 319–329.
Seinhorst J. W. (1986). “Effect of nematode attack on the growth and yield of crop plants,” in Cyst nematodes, vol. pp . Eds. Lamberti F., Taylor C. E. (New York (USA: Plenum Press), 191–209. London (UK).
Singh S. K., Hodda M., Ash G. J., Banks N. C. (2013). Plant-parasitic nematode as invasive species: characteristics, uncertainty and biosecurity implications. Ann. App Biol. 163, 223–250. doi: 10.1111/aab.12065
Keywords: phytoparasitic nematodes, extraction methods, centrifugal flotation, FLOTAC, Root maceration
Citation: Troccoli A, d’Errico G, D’Addabbo T, Sasanelli N, Bosco A, Maurelli MP, Rinaldi L and Cringoli G (2022) The FLOTAC basic technique as a new extraction method for root-knot nematodes (Meloidogyne spp.) from soil and roots. Front. Parasitol. 1:1000673. doi: 10.3389/fpara.2022.1000673
Received: 22 July 2022; Accepted: 23 August 2022;
Published: 14 September 2022.
Edited by:
Maria Victoria Periago, Consejo Nacional de Investigaciones Científicas y Técnicas (CONICET), ArgentinaReviewed by:
Sonia Almeria, United States Food and Drug Administration, United StatesShawky Mohamed Aboelhadid, Beni-Suef University, Egypt
Copyright © 2022 Troccoli, d’Errico, D’Addabbo, Sasanelli, Bosco, Maurelli, Rinaldi and Cringoli. This is an open-access article distributed under the terms of the Creative Commons Attribution License (CC BY). The use, distribution or reproduction in other forums is permitted, provided the original author(s) and the copyright owner(s) are credited and that the original publication in this journal is cited, in accordance with accepted academic practice. No use, distribution or reproduction is permitted which does not comply with these terms.
*Correspondence: Alberto Troccoli, alberto.troccoli@ipsp.cnr.it
†These authors have contributed equally to this work