- 1Division of General Internal Medicine, Virginia Commonwealth University, Richmond, VA, United States
- 2Department of Psychology, Virginia Commonwealth University, Richmond, VA, United States
- 3College of Nursing, University of South Florida School of Nursing, Tampa, FL, United States
- 4Department of Anesthesiology, Duke University, Durham, NC, United States
Sickle cell disease (SCD) is a prevalent and complex inherited pain disorder that can manifest as acute vaso-occlusive crises (VOC) and/or chronic pain. Despite their known risks, opioids are often prescribed routinely and indiscriminately in managing SCD pain, because it is so often severe and debilitating. Integrative medicine strategies, particularly non-opioid therapies, hold promise in safe and effective management of SCD pain. However, the lack of evidence-based methods for managing SCD pain hinders the widespread implementation of non-opioid therapies. In this review, we acknowledge that implementing personalized pain treatment strategies in SCD, which is a guideline-recommended strategy, is currently fraught with limitations. The full implementation of pharmacological and biobehavioral pain approaches targeting mechanistic pain pathways faces challenges due to limited knowledge and limited financial and personnel support. We recommend personalized medicine, pharmacogenomics, and integrative medicine as aspirational strategies for improving pain care in SCD. As an organizing model that is a comprehensive framework for classifying pain subphenotypes and mechanisms in SCD, and for guiding selection of specific strategies, we present evidence updating pain research pioneer Richard Melzack’s neuromatrix theory of pain. We advocate for using the updated neuromatrix model to subphenotype individuals with SCD, to better select personalized multimodal treatment strategies, and to identify research gaps fruitful for exploration. We present a fairly complete list of currently used pharmacologic and non-pharmacologic SCD pain therapies, classified by their mechanism of action and by their hypothesized targets in the updated neuromatrix model.
1. Introduction
Most pain, including sickle cell disease (SCD) pain, is managed at home without professional supervision (1–4). And when individuals with pain present to clinicians, they are most often treated with palliative approaches. Drugs such as non-steroidal anti-inflammatory drugs, antidepressants, anticonvulsants, local anesthetics, muscle relaxants, and antianxiety medicines may be used, as may non-pharmacological interventions (5). For SCD pain in western countries, one of the most common palliative therapies used is opioid therapy (6, 7). Though expert guidelines advocate for opioid-sparing approaches, employing multimodal combinations of therapy (8, 9), usually administered by a multidisciplinary team (10), they are based mostly on expert consensus, and often poorly adhered to (11, 12). Treatment can be frustrating for clinicians trained to apply scientific and mechanistic principles to the practice of medicine.
1.1. Precision medicine and pharmacogenomics in SCD
Precision medicine (13) has been defined as “the tailoring of medical treatment to the individual characteristics of each patient…to classify individuals into subpopulations that differ in their susceptibility to a particular disease or their response to a specific treatment.” (14) Applied to pain, precision medicine involves phenotyping pain patterns and operative mechanisms, genetics, and expressions of pain in each individual; then creating or revising an individualized pain management plan for all phases of pain care based on observed phenotypic and mechanistic features. Precision medicine acknowledges individuals’ unique differences in disease susceptibility and drug metabolism, depending on variations in their genetic makeup.
Because of the intricate interplay between genetics and pain expression and analgesic response, pharmacogenomics, a precision medicine modality, can potentially play a crucial role in safe and effective sickle cell disease pain management (15). Pharmacogenomics is the genomic profiling of patients for genetic variants that clinically modify the tolerability and desired effect of specific medications (16). For instance, the cytochrome P450 (CYP) genes variants have an impact on the metabolism of multiple medications across analgesic drug classes. Patients who poorly metabolize certain analgesic drugs may face mild, moderate, or even severe toxicities, requiring acute care admission or even hospitalization (17). Further, severe or even mild adverse events may lower adherence, and genetic variance may be associated with reduced drug efficacy and increased medication wastage.
Pharmacogenomics-guided prescribing guidelines for approximately 80 drugs have been developed by international collaborative groups such as the Clinical Pharmacogenetics Implementation Consortium (CPIC) and the Dutch Pharmacogenetics Working Group (DPWG) (18). Almost two-thirds of these actionable drug-gene associations involve drug-metabolizing enzyme genes, about 80% of which encode cytochrome P450 (CYP) enzymes (19). Preemptive pharmacogenetic testing to predict a patient’s metabolic response can facilitate individualized prescribing (20), but genotype-guided dosing practice is not readily available in SCD care (21).
1.2. Integrative health
Integrative health “includes whole person health, that is, empowering individuals, families, communities, and populations to improve their health in multiple interconnected domains: biological, behavioral, social, and environmental.” Modes of integrative healthcare have received increasing attention and trials among individuals with SCD, especially for pain (22). Integrative approaches come in two subtypes. If a non-mainstream practice is used together with conventional medicine, it is considered “complementary.” If a non-mainstream practice is used in place of conventional medicine, it is considered “alternative.” Complementary approaches are currently the most ones used to treat chronic pain (23).
1.3. Models and mechanisms of pain
Precision medicine and integrative health both evoke a transcendent causal and treatment model of pain to replace the long-held and accepted Cartesian pain model (24). The Cartesian model views pain purely as a biomarker of tissue damage or inflammation that is detected by the peripheral nervous system. The role of = ascending sensory fibers, especially the first-order neurons in the spinothalamic tract, the dorsal root ganglia, and the second-order ascending fibers, is to transmit pain signals unmodulated to the central nervous system (CNS) (25, 26). The role of the CNS is only to receive and process these unmodulated pain signals, leading to passive perception of pain, and some kind of reaction. In contrast, a transcendent pain model consistent with the practice of precision medicine and integrative health must explain the observed pain phenotypes, pain mechanisms, and pain findings which aren’t explained by the Cartesian model. Decades ago, Richard Melzack proposed such a model, the Neuromatrix theory of pain (27, 28). It theorizes that multiple parts of the nervous system– the “body-self neuromatrix”—work together to generate pain, and that pain can be produced independently of peripheral sensory input (29). It is consistent with Engel’s general biopsychosocial model (30–33), because it theorizes environmental as well as internal biological influences on pain. The neuromatrix is genetically predetermined (34), but is biologically responsive to stimuli. Further, pain responses in the neuromatrix may be so altered by the environment that they no longer require a synchronous environmental stimulus, such as in phantom limb pain (35).
Since Melzack’s theory was proposed, animal models, human imaging studies (36) including functional magnetic resonance imaging studies (37), and even invasive brain electrode studies (38) have led to a consensus that a distributed, anatomical neuromatrix is indeed responsible for receipt and processing of a variety of pain signals (Figure 1). For acute pain, this network consists not only of the ascending pain fibers, but also of the primary and secondary somatosensory, insular, anterior cingulate, and prefrontal cortices and the thalamus (36). But more important than these multiple structures is the network of connections between the structures. Connections in this network are constantly shifting (39, 40). We hypothesize, but are still unsure how, shifts in these connections over time can cause a transformation from acute pain to chronic pain. However, evidence is accumulating to show that this same pain neuromatrix, this network, is operative in patients with SCD (41–44).
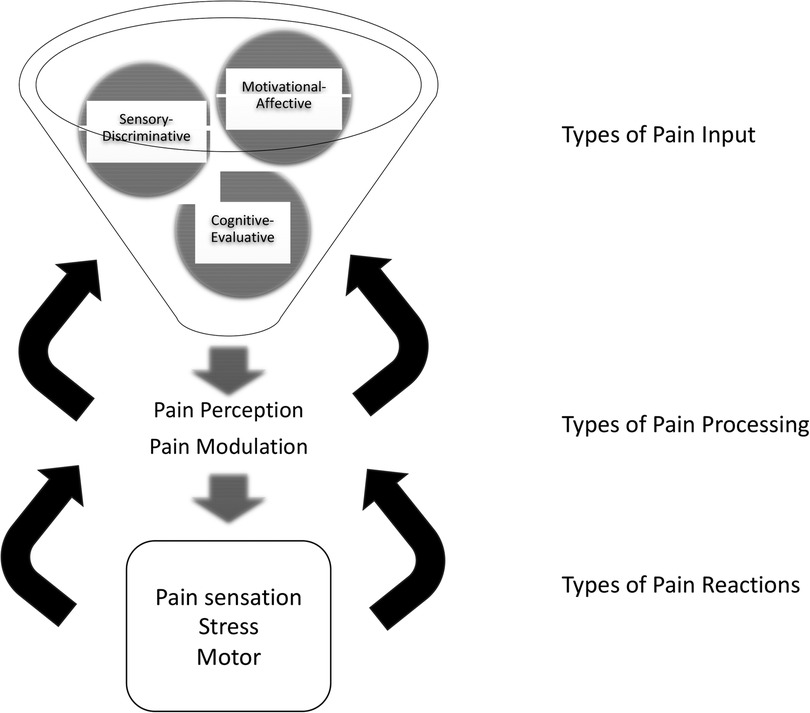
Figure 1. Updated neuromatrix model of pain with components. Three types of input, sensory-discriminative, cognitive-evaluative, and motivational-affective, integrate into pain processors. Processing includes perception in midbrain and thalamic brain structures and a network of connections between them, and modulation by these connections and by ascending and descending fibers. Three types of resulting pain reactions include pain perception/sensation, motor function, and stress. Feedback modulates every stage: reactions modulate processing, and processing modulates input signals. Pain intervention targets are suggested by each component. (Adapted from Melzack and numerous authors).
The updated pain neuromatrix suggests multipe targets for pain therapy for SCD. Interventions may be directed either at three types of inputs, i.e., sensory-discriminative, cognitive-evaluative, and motivational-affective inputs. Or interventions may be directed at pain processing, i.e., interventions may alter how pain signals are integrated, modulated, and/or passed on in the pain neuromatrix.
Regarding inputs, sensory-discriminative inputs consist of cutaneous, musculoskeletal, visceral, visual, and other sensory inputs, which define the location and quality of pain perception. Cognitive-evaluative inputs provide interpretation or meaning for the pain experience. This includes factors, such as memories of past experiences, cultural understanding of pain, pain rumination, pain catastrophizing (45), and somatization of pain. Motivational-affective inputs add an emotion or feeling to the pain experience. They ask, “what should I feel and/or do about my pain?” The limbic system and its associated regulation of autonomic and endocrine responses, including in the gut (46) provides motivational-affective input. Its role in SCD pain requires further exploration (47, 48); however, many studies report comorbid depression and anxiety (49), commonly seen in many pain states.
Regarding pain processing of these types of pain inputs, integration into a summary pain perception, as well as modulation of the inputs, occurs in the network of midbrain and thalamic structures, their connections, and ascending and descending fibers. Last, regarding proposes types of reactions to pain, the model proposes pain perception reactions, motor reactions, and stress reactions. These may vary and may have dramatic effects on subsequent pain trajectories.
Regarding pain mechanisms and phenotypes, pain has traditionally been characterized as either nociceptive or neuropathic. However, the prevalence of the phenotype of unexplained widespread chronic pain, not only in SCD but also in fibromyalgia (50), irritable bowel syndrome (51), and chronic pelvic pain (52), has forced authorities to adopt a third pain mechanism, nociplastic pain (53), distinct from nociceptive pain (i.e., pain due to local inflammation or tissue damage) and from neuropathic pain (i.e., pain due to nerve damage). Research on this mechanism is far less mature. Experts agree that nociplastic pain is not a distinct entity, but rather part of a chronic pain continuum. Nociplastic pain results from neuroplasticity, in this case, an acquired, augmentation of sensory and CNS signal processing, including pain perception and pain modulation. Initially called central sensitization (CS), to emphasize afferent pathways, CS is now known to involve activation or disinhibition of descending pain pathways as well (54), via activation of N-methyl-D-aspartate (NMDA) receptors. Indeed, significant cross talk exists between mu-opioid receptors and NMDA receptors. In SCD, CS is postulated to arise from years of repetitive vaso-occlusive, inflammatory, or other stimulation from SCD, leading to, a reset of the “pain thermostat” in the neuromatrix (55, 56).
Phenotypically, nociplastic pain produces quite variable symptoms, but typically amplified and (over)maintained clinical pain (57) including hyperalgesia—an increased sensitivity to a painful stimulus- or allodynia -pain that is provoked by a stimulus that is normally non-painful. Often, patients have widespread rather than localized pain (58). Widespread, bilateral pain is common in SCD (59). SCD patients with CS have more acute pain, worse sleep, and more psychosocial disturbances than others (60). Anatomically, nociplastic pain is associated with inflammation, not only in central neurons, but also in central glial cells (61, 62). But as stated, the triggers are unknown for transformation of the pain phenotype from intermittent acute, localized pain to widespread chronic pain, the “chronification” of pain.
Framing pain using the neuromatrix justifies treating pain in SCD using precision medicine and integrative health approaches. Already, research guided by this framework has been aimed at investigating the inputs, pain responses, phenotypes, and predictors of pain response in SCD and other pain disorders (63–70). Future research can be aimed at treatment that is more scientific, rather than empiric and atheoretic.
1.4. Article purpose
Thus, this non-systemic topical review is intended to inform the development of precision medicine, integrative healthcare approaches to SCD pain. We have organized the various therapeutic approaches around the updated neuromatrix model. Figure 2 hypothesizes the efficacy of various currently used approaches using the neuromatrix model. The intent of this review is to give practitioners a theoretical basis for multimodal pain therapy to patients with SCD, as well as to suggest a research agenda for improving SCD pain therapy. Figure 2 categorizes all interventions based on their hypothesized neuromatrix operative targets (sensory-discriminative, cognitive-evaluative, motivational-affective inputs, or pain processing). It implies how clinicians might combine standard and alternative/complementary approaches to attack multiple pain targets and perhaps achieve greater pain reductions and/or greater opioid sparing. The review also discusses, for each pharmacotherapeutic approach, whether pharmacogenetics has advanced such that clinicians may more intelligently select or more precisely use that approach for pain in SCD.
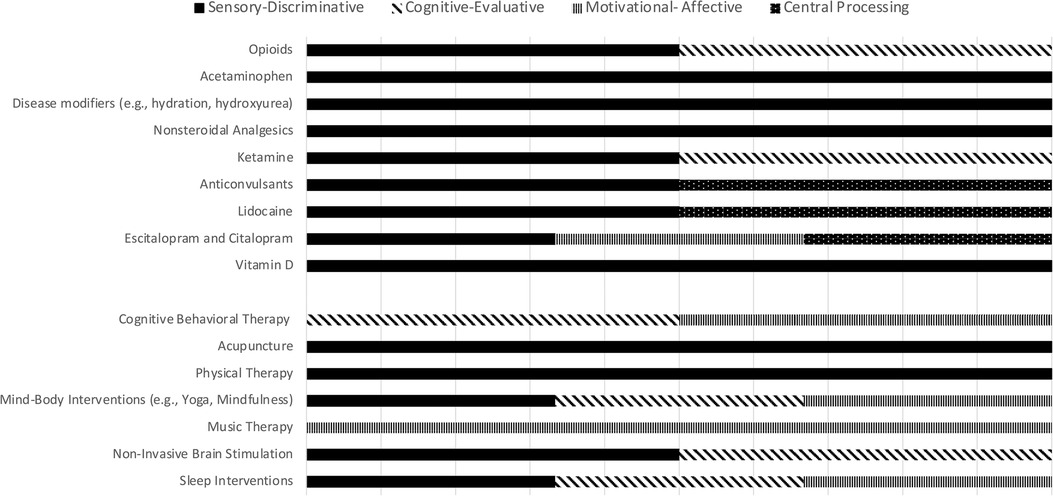
Figure 2. Standard and alternative sickle cell disease pain therapies, and their hypothesized target (s)—sensory-discriminative, cognitive-evaluative, motivational-affective, and pain processing—based on the neuromatrix model of pain. Multiple targets are hypothesized for many therapies.
2. Opioids
Opioid analgesics, especially mu (μ) receptor opioid agonists, have long been the mainstay of acute palliative therapy for SCD pain. (71). The clinical pharmacology of opioids for pain has been extensively reviewed (72). They are active in the peripheral nervous system, at the spinal cord dorsal horn, the brain stem, thalamus, and cortex, modifying sensory neurons both in the ascending pain transmission system and modifying parts of the descending inhibitory system in the spinal cord. Mu opioids, however, are associated with several adverse effects that often discourage their use, including some specific to the SCD population: rapid pharmacodynamic tolerance, the need for a higher mu opioid dose to maintain the analgesic effect (73); increased renal opioid clearance in SCD necessitating higher doses, (74, 75). physical dependence, i.e., opioid withdrawal on removal of opioids or administration of an antagonist; paradoxical pronociceptive effects or opioid-induced hyperalgesia (76); increased mortality (77); broad endocrine effects (78); GI complications, and (79); euphoria and craving leading to opioid use disorder (80, 81) and/or use of heroin (82). For these reasons, enthusiasm for the use of opioids has been limited to their role in treating acute rather than chronic pain (83–88). Further, the evidence of the efficacy of chronic opioid therapy (COT) beyond 12 weeks has been scant (89–91). On the other hand, the harms of opioids in SCD and specifically of COT in SCD compared to the harms in other groups with CNCP are small (92–94).
CDC revised its 2016 published guidelines on COT (95) in 2022 (96), by explicitly excluding chronic pain management and COT related to SCD in 2022. The American Society of Hematology recommended against the use of COT in SCD unless pain was refractory to multiple other treatment modalities. However this ASH recommendation was conditional, and based on very low certainty in the evidence about effects. Implementing these recommendations, including the spirit of the CDC guidelines, is fraught with difficulty. One must select individuals with SCD who should safely be prescribed a trial of COT. One must wean individuals off COT who have been deemed to fail this trial. And one must predict or detect opioid use disorder (OUD) in a population where pain may relent and recur and/or may be acute-on-chronic.
3. Acetaminophen
Acetaminophen (APAP) is a widely used oral analgesic and is primarily believed to exert its analgesic effect centrally via cyclooxygenase (COX) inhibition with no significant peripheral anti-inflammatory effects (97). Oral, suppository, and intravenous forms are available (98). Inter-patient variability in efficacy and hepatotoxicity are the main precision medicine issues associated with APAP treatment (99). The variability is attributed to genetic polymorphisms in the CYP2E1, CYP1A2, CYP3A4, UGT, SULT and GST genes. However, to date, the interaction between these genes has not been well delineated, limiting the role of pharmacogenetic biomarkers in the clinical management of APAP (100, 101).
4. Disease modifiers
Hydration (102), hydroxyurea (103, 104), prescription-grade L-Glutamine (105), voxelotor (106), crizanlizumab (107), red cell (simple or exchange) transfusion (108), and allogeneic bone marrow transplantation or cord blood transplantation (109) are the mainstays of remittive therapy or potentially curative therapy with the possibility of improved pain in SCD. Each are underutilized, and transplantation is still considered experimental by many. When taken regularly, hydroxyurea reduces acute chest syndrome, ED visits, hospitalizations, “strong” opioid use, hospital days, health care costs (110–112), and lengthens life (113), but does not statistically significantly reduce daily pain in most patients (114). Similarly, though they reduce annualized hospitalizations, neither L-glutamine nor crizanlizumab were approved based on improvement in daily pain. Voxelotor was approved based on improvements in total hemoglobin. Transfusions, when chronic, may decrease hospitalization rates (115–117), but are not generally used to reduce pain. Even therapy with curative intent does not uniformly eradicate chronic (likely central) pain in individuals with SCD, but rather aborts acute nociceptive pain (118–120).
5. Non-steroidal analgesics
Nonsteroidal anti-inflammatory drugs (NSAIDs), such as celecoxib, ibuprofen, flurbiprofen, and ketorolac (121), are widely used in management of SCD pain. These medications exert their effects by inhibiting cyclooxygenase (COX) enzymes and suppressing prostaglandin production (122). Notably, NSAIDs function independently of the opioid-mu receptor system, potentially reducing the amount of opioids required for pain (123, 124). However, to date, studies of NSAIDs for SCD pain have shown no significant reduction in the duration of VOCs or pain scores (125–128), nor have they demonstrated opioid sparing (129, 130). Among children, first-line therapy with IV ketorolac and IV fluids resulted in adequate resolution of pain (and avoidance of opioids) in 53% of VOCs (131). NSAIDs’ well-known potential gastrointestinal, cardiovascular, and renal adverse effects restrict their prolonged or chronic use (132).
Cyclooxygenase 2 (COX-2) inhibitors such as R-flurbiprofen and MRS2578 are being studied in SCD (133), and lipoxygenase (LOX) inhibitors are being studied in chronic pain (134), in hopes of achieving analgesic efficacy without the undesired gastrointestinal and renal adverse effects.
NSAIDs are metabolized primarily by the polymorphic CYP2C9 gene. The Clinical Pharmacogenetics Implementation Consortium (135) published the following standardizing terms for clinical pharmacogenetic test results: poor metabolizer, intermediate metabolizer, normal metabolizer, rapid metabolizer, and ultrarapid metabolizer. Using these terms, individuals with CYP2C9 poor or intermediate metabolizer phenotypes could have increased drug exposure, resulting in increased adverse effects from NSAIDs, because metabolism to inactive metabolites is defective in these patients. The CYP2C9*2 allele is associated with greater ibuprofen and diclofenac toxicity. Poor or intermediate CPY2C9 metabolizers need to be started on lower-than-normal starting doses of NSAIDs to avoid potential ADRs, whereas patients who are normal CYP2C9 metabolizers may require higher doses of NSAIDs to achieve effective pain relief (136).
6. Ketamine
Ketamine, a potent analgesic and phencyclidine analogue primarily used as anesthetic, acts as a competitive antagonist of the N-methyl-D-aspartate (NMDA) receptor (137, 138), potentially interfering with central sensitization (139, 140). It is available in various formulations, including intravenous oral, intramuscular, intrarectal or intranasal administrations. Notably, ketamine exhibits opioid sparing effects by interacting with several other binding sites, including mu-opioid receptors and cholinergic and muscarinic receptors (137). Ketamine has also demonstrated efficacy in treatment-resistant depression in recent decades (141). Low doses of ketamine have been used and reported in case studies of VOC and acute and chronic SCD pain (142), especially when pain is refractory to opioids (143), although the supporting evidence remains of low certainty (144). A systematic review of the pharmacogenomics of ketamine found that variants in the CYP450, OPRM, and BDNF genes alter the above-defined metabolizer status of ketamine (poor, intermediate, etc.) and therefore may someday be useful as biomarkers to guide ketamine treatment (141).
7. Anticonvulsants: gabapentin and pregabalin
The gabapentinoids (pregabalin and gabapentin), analogues of the neurotransmitter, gamma-aminobutyric acid (GABA), are considered the cornerstone of the pharmacological management of neuropathic pain. While the precise mechanism of action is uncertain (145), in rats, gabapentin and pregabalin are believed to decrease central sensitization by binding to the α2δ-1 subunit of voltage gated calcium channels in the dorsal root ganglia (146, 147). However, systematic reviews in humans report differing conclusions concerning gabapentinoid effectiveness in preventing chronic pain (148, 149). Adverse effects include dizziness, somnolence, dry mouth, fatigue, ataxia, blurred vision, peripheral edema, and weight gain. Gabapentinoids are substrates of drug transporters OCTN1 and OCT2 encoded by the SLC22A2 and SLC22A4 genes respectively. Polymorphisms in these genes are known to alter the activity of both transporters (150). Dosing should be adjusted in patients with renal disease due to the drugs’ renal excretion.
8. Lidocaine
Lidocaine, a local anesthetic and antiarrhythmic medication, is a well-established non-opioid analgesic for post-operative pain, and used as a second-line agent for neuropathic pain. It is available in systemic and transdermal formulations. It is believed to interact with multiple molecular targets including sodium channels, NMDA receptors and G-protein coupled receptors to exert its therapeutic effects (151–153). The evidence of its efficacy in SCD is sparse (154, 155). It is metabolized by CYP3A4 (156). The clearance of lidocaine does not appear to be affected by pharmacogenomic variability when administered perioperatively as part of multimodal analgesia (157).
9. Escitalopram and citalopram
The selective serotonin reuptake inhibitors (SSRIs), citalopram and escitalopram, are not only widely used for treating major depression and anxiety disorders in SCD, but also anecdotally for SCD pain. The serotonin reuptake site (serotonin transporter), placed presynaptically on serotonergic nerve terminals, is the key regulator of synaptic serotonin levels in the central nervous system (158). Escitalopram has demonstrated effects on neuropathic pain. Citalopram and escitalopram are metabolized primarily by CYP2C19 and, to a lesser extent, by CYP3A4 and CYP2D6 (159). Amitriptyline can provide some pain relief in certain chronic non-cancer pain conditions. However, we found no published evidence either supporting or refuting the use of citalopram in patients with SCD pain. A Cochrane review of antidepressants to treat chronic non-cancer pain found insufficient data for a meta-analysis (160). A review of adult treatment for neuropathic pain included a meta-analysis which was unconvincing for these drugs (161). No predictive algorithm is currently available for dosing these drugs for pain management (162).
10. Vitamin D
Vitamin D use among individuals with SCD who are often Vitamin D deficient has been reported to curb pain but studies did not measure opioid use (163, 164).
11. Other pharmacologic targets and systems
A recent review suggests various targets and systems of pain sensation which may generate therapeutic approaches that may be fruitful (165). These include pain signaling pathways, neurotransmitters, and analgesic receptors such as Ca2+/calmodulin-dependent protein kinase II (166, 167), protein kinase C delta (PKCδ) receptor on GABAergic neurons (168), nociceptin opioid receptor (169), and cannabinoid receptors (170, 171) which have shown promise in relieving pain in preclinical animal models (172, 173). Not yet tried widely in SCD is neuromodulation via direct anatomic or physiologic interruption of signaling pathways known to incite pain and discomfort (174). Another approach being investigated in sickle mice is to target pain prior vaso-occlusive or inflammatory nerve damage believed to lead to chronic pain (171, 175) and attempt to alter or repair it. Examples include targeting the nerve-regeneration associated gene, small proline-rich protein 1A (SPRR1A) (176, 177). In the clinical arena, the term regenerative pain medicine means harnessing the body’s own reparative capacity to treat pain (178).
12. Integrative medicine approaches
12.1. General use
There are few studies of non-pharmacological, complementary pain management approaches to address SCD pain, but most support the promise of these therapies. A recent systematic review of non-pharmacological treatments to reduce SCD pain during childhood found only 10 RCTs and quasi-experimental studies, and found most had significant methodological limitations (e.g., small samples, lack of follow-up data or control groups) (179). This lack of rigorous investigations is in contrast to research indicating the majority of children with SCD use some type of complementary technique to augment their existing treatment regimens (180) and that, when offered integrative approaches that include complementary techniques, over 70% of children with SCD and their families report the approaches to be acceptable and likely effective for treating SCD pain in adolescence.
12.2. Cognitive behavioral therapy for pain
The majority of the studies and most promising evidence is for cognitive behavioral therapy for pain (CBT-P) (179). CBT-P focuses on training people on a suite of cognitive and behavioral skills to alter the affective and cognitive mechanisms of pain. Common components include psychoeducation concerning the biopsychosocial model of pain, cognitive restructuring, relaxation, and distraction. In the previous review, 5 of the 10 identified studies were focused on CBT-P, with overall findings indicating reductions in pain frequency and intensity. Findings also found no evidence that CBT-P reduced health care use due to pain, and most studies were small in size. Notably, there is an ongoing clinical trial examining the efficacy of CBT-P (181).
12.3. Mind-body interventions
Of the other promising treatments in the above review, biofeedback, virtual reality, and yoga each had only 1 study finding reductions in pain. Biofeedback was related to reduced analgesic use in SCD. Mindfulness meditation is being studied currently (182).
12.4. Acupuncture
A review of the use of acupuncture for pain management in pediatric SCD patients (183) found 5 studies with sample sizes ranging from one to 31. Overall, studies indicated that the treatment was feasible, acceptable, and an effective adjunctive pain treatment. These findings are consistent with findings from adult SCD studies indicating acupuncture reduces pain intensity and pain interference, including during acute SCD pain crises (184–187). However, these studies had small sample sizes, ranging from 6 to 47 patients. The primary methods were case reports and retrospective chart reviews, and there are no published RCTs. One ongoing study is testing guided relaxation and acupuncture for SCD pain in adults (188).
12.5. Physical therapy
A pilot RCT of 10 adults with SCD indicated conventional physical therapy and aquatic physical therapy were acceptable and lead to reduced SCD pain (189), and a 2-arm non-randomized clinical trial indicated a home-based therapeutic exercise program led to reductions in SCD pain (190).
12.6. Music therapy, non-invasive brain stimulation, sleep-based approaches
A mixed-method RCT of 24 adults with SCD found that music therapy led to reductions in pain intensity and pain interference (191). We found no published clinical trials of non-invasive brain stimulation or sleep-based interventions, such as CBT for insomnia.
13. Summary
Most of the components available for the assembly of a pain treatment plan for individuals with SCD, even when guideline-recommended, have little evidence for their recommendation. In this way, SCD is similar to other diseases which comprise chronic non-cancer pain. However, precision medicine and integrative health offer hope and a research agenda for a thoughtful multimodal, individualized approach to diagnosis and treatment of acute and chronic pain in SCD. They suggest the use of emerging tools, such as survey instruments, sleep evaluation, pharmacogenomic testing, and functional magnetic resonance imaging, in developing robust individual pain profiles/subphenotypes. They suggest the incorporation of multimodal pharmacologic and non-pharmacologic components into an individualized treatment plan, based on the diagnosed subphenotype of a given patient. And they each demand clinicians identify and avoid drugs or other therapies with an increased risk of causing adverse effects, drugs with a narrow therapeutic index, or drugs or other therapies predicted not to be efficacious (192) for individuals living with SCD.
Author contributions
WS: Conceptualization, Data curation, Investigation, Methodology, Writing – original draft, Writing – review and editing. CV: Data curation, Writing – original draft, Writing – review and editing. CJ: Data curation, Writing – review and editing. MK: Writing – original draft, Writing – review and editing.
Funding
The author(s) declare financial support was received for the research, authorship, and/or publication of this article.
SHIP-HU Study, 1 R18 HL 112737, National Heart, Lung, and Blood Institute, NIH. Pain in Sickle Cell Epidemiology Study, 1 R01 HL 64122, National Heart, Lung, and Blood Institute, NIH. Virginia Basic and Translational Research Program in Sickle Cell Disease. 1U54HL090516, National Heart, Lung, and Blood Institute and Sickle Cell Disease Clinical Research Network, National Heart, Lung, and Blood Institute 1U10HL083732. MOK is supported by a National Heart, Lung, and Blood Institute Career Development Award K01-HL169339-01.
Conflict of interest
WS is a consultant with Pfizer, Agios, Novo-Nordisk, Alexion, and Novartis.
The remaining authors declare that the research was conducted in the absence of any commercial or financial relationships that could be construed as a potential conflict of interest.
The handling editor KG declared a past co-authorship with the author WRS.
Publisher's note
All claims expressed in this article are solely those of the authors and do not necessarily represent those of their affiliated organizations, or those of the publisher, the editors and the reviewers. Any product that may be evaluated in this article, or claim that may be made by its manufacturer, is not guaranteed or endorsed by the publisher.
References
1. National pain strategy report: a comprehensive population health-level strategy for pain—introduction. Available at: https://www.iprcc.nih.gov/node/5/national-pain-strategy-report (Accessed October 17, 2023).
2. Dampier C, Ely E, Brodecki D, O'Neal P. Home management of pain in sickle cell disease: a daily diary study in children and adolescents. J Pediatr Hematol Oncol. (2002) 24(8):643–7. doi: 10.1097/00043426-200211000-00008
3. Dampier C, Ely B, Brodecki D, O'Neal P. Characteristics of pain managed at home in children and adolescents with sickle cell disease by using diary self-reports. J Pain. (2002) 3(6):461–70. doi: 10.1054/jpai.2002.128064
4. Smith WR, Penberthy LT, Bovbjerg VE, Roberts JD, Dahman B, et al. Daily assessment of pain in adults with SCD. Ann Intern Med. (2008) 148:94–101. doi: 10.7326/0003-4819-148-2-200801150-00004
5. Coelho A, Parola V, Cardoso D, Bravo ME, Apóstolo J. Use of non-pharmacological interventions for comforting patients in palliative care: a scoping review. JBI Database System Rev Implement Rep. (2017) 15(7):1867–904. doi: 10.11124/JBISRIR-2016-003204
6. Miller ST, Kim HY, Weiner D, Wager CG, Gallagher D, Styles L, et al. Inpatient management of sickle cell pain: a ’snapshot’ of current practice. Am J Hematol. (2012) 87(3):333–6. doi: 10.1002/ajh.22265
7. Smith WR, McClish DK, Dahman BA, Levenson JL, Aisiku IP, de A Citero V, et al. Daily home opioid use in adults with sickle cell disease: the PiSCES project. J Opioid Manag. (2015) 11(3):243–53. doi: 10.5055/jom.2015.0273
8. Brandow AM, Carroll CP, Creary S, Edwards-Elliott R, Glassberg J, Hurley RW, et al. American society of hematology 2020 guidelines for sickle cell disease: management of acute and chronic pain. Blood Adv. (2020) 4(12):2656–701. doi: 10.1182/bloodadvances.2020001851
9. Kenney MO, Smith WR. Moving toward a multimodal analgesic regimen for acute sickle cell pain with non-opioid analgesic adjuncts: a narrative review. J Pain Res. (2022) 15:879–94. doi: 10.2147/JPR.S343069
10. Interagency Pain Research Coordinating Committee. National pain strategy: a comprehensive population health-level strategy for pain. Available at: https://iprcc.nih.gov/National-Pain-Strategy/Overview (Accessed April 27, 2018).
11. Von Korff MR. Long-term use of opioids for complex chronic pain. Best Pract Res Clin Rheumatol. (2013) 27(5):663–72. doi: 10.1016/j.berh.2013.09.011
12. Tournebize J, Gibaja V, Muszczak A, Kahn JP. Are physicians safely prescribing opioids for chronic noncancer pain? A systematic review of current evidence. Pain Pract. (2016) 16(3):370–83. doi: 10.1111/papr.12289
13. Ginsburg GS, Phillips KA. Precision medicine: from science to value. Health Aff (Millwood). (2018) 37(5):694–701. doi: 10.1377/hlthaff.2017.1624
14. National Research Council (US) Committee on A Framework for Developing a New Taxonomy of Disease. Toward precision medicine: Building a knowledge network for biomedical research and a new taxonomy of disease. Washington (DC): National Academies Press (US) (2011).
15. Evans WE, McLeod HL. Pharmacogenomics—drug disposition, drug targets, and side effects. N. Engl. J. Med. (2003) 348:538–49. doi: 10.1056/NEJMra020526
16. Pirmohamed M, James S, Meakin S, Green C, Scott AK, Walley TJ, et al. Adverse drug reactions as cause of hospital admission: prospective analysis of 18 820 patients. Br Med J. (2004) 329:15–9. doi: 10.1136/bmj.329.7456.15
17. Howard RL, Avery AJ, Slavenburg S, Royal S, Pipe G, Lucassen P, et al. Which drugs cause preventable admissions to hospital? A systematic review. Br. J. Clin. Pharmacol. (2007) 63:136–47. doi: 10.1111/j.1365-2125.2006.02698.x
18. Pharmacogenomics Knowledge Base (PharmGKB). Clinical guideline annotations. Available at: https://www.pharmgkb.org/guidelineAnnotations (Accessed May 19, 2023).
19. Gallaway KA, Sakon C, Ongeri J, Patel KS, Oliver J, Patacca H, et al. Opportunity for pharmacogenetics testing in patients with sickle cell anemia. Pharmacogenomics. (2022) 23:925–31. doi: 10.2217/pgs-2022-0115
20. Rollinson V, Turner R, Pirmohamed M. Pharmacogenomics for primary care: an overview. Genes (Basel). (2020) 11:1337. doi: 10.3390/genes11111337
21. Jaja C, Bowman L, Wells L, Patel N, Xu H, Lyon M, et al. Preemptive genotyping of CYP2C8 and CYP2C9 allelic variants involved in NSAIDs metabolism for sickle cell disease pain management. Clin Transl Sci. (2015) 8(4):272–80. doi: 10.1111/cts.12260
22. Brown M, Anheyer D, Morris CR. Special issue: pediatric pain and sickle cell disease. Complement Ther Med. (2022) 71:102880. doi: 10.1016/j.ctim.2022.102880
23. Health NCfCaI. NCCIH strategic plan FY 2021–2025: mapping the pathway to research on whole person health. (2022). Available at: https://nccih.nih.gov/about/nccih-strategic-plan-2021-2025
24. Trachsel LA, Munakomi S, Cascella M. Pain theory. In: Statpearls. Treasure Island (FL): StatPearls Publishing LLC (2023). Available at: https://www.ncbi.nlm.nih.gov/books/NBK545194/ (Accessed April 17, 2023).
25. Goldberg JS. Revisiting the cartesian model of pain. Med Hypotheses. (2008) 70(5):1029–33. doi: 10.1016/j.mehy.2007.08.014
26. Trachsel LA, Cascella M. Pain theory. In: Statpearls. Treasure Island (FL): StatPearls Publishing LLC (2021). Copyright © 2021.
27. Melzack R. Pain and the neuromatrix in the brain. J Dent Educ. (2001) 65(12):1378–82. doi: 10.1002/j.0022-0337.2001.65.12.tb03497.x
28. Melzack R. Evolution of the neuromatrix theory of pain. The prithvi raj lecture: presented at the third world congress of world institute of pain, Barcelona 2004. Pain Pract. (2005) 5(2):85–94. doi: 10.1111/j.1533-2500.2005.05203
29. Melzack R. From the gate to the neuromatrix. Pain. (1999) 6(Suppl ):S121–s126. doi: 10.1016/s0304-3959(99)00145-1
30. Adler RH. Engel’s biopsychosocial model is still relevant today. J Psychosom Res. (2009) 67(6):607–11. doi: 10.1016/j.jpsychores.2009.08.008
31. Wade DT, Halligan PW. The biopsychosocial model of illness: a model whose time has come. Clin Rehabil. (2017) 31(8):995–1004. doi: 10.1177/0269215517709890
32. Linton SJ. A critical review of behavioural treatments forchronic benign pain other than headache. Br J Clin Psychol. (1982) 21(Pt 4):321–37. doi: 10.1111/j.2044-8260.1982.tb00570.x
33. Guzman J, Esmail R, Karjalainen K, Malmivaara A, Irvin E, Bombardier C. Multidisciplinary bio-psycho-social rehabilitation for chronic low back pain. Cochrane Database SystRev. (2006) 1(2):CD000963. doi: 10.1002/14651858.CD000963
34. Lötsch J, Doehring A, Mogil JS, Arndt T, Geisslinger G, Ultsch A, et al. Functional genomics of pain in analgesic drug development and therapy. Pharmacol Ther. (2013) 139(12013):60–70. doi: 10.1016/j.pharmthera.2013.04.004
35. Melzack R. Phantom limbs and the concept of a neuromatrix. Trends Neurosci. (1990) 13(3):88–92. doi: 10.1016/0166-2236(90)90179-e
36. Apkarian AV, Bushnell MC, Treede RD, Zubieta JK. Human brain mechanisms of pain perception and regulation in health and disease. Eur J Pain. (2005) 9(4):463–84. doi: 10.1016/j.ejpain.2004.11.001
37. Wager TD, Atlas LY, Lindquist MA, Roy M, Woo CW, Kross E. An FMRI-based neurologic signature of physical pain. N Engl J Med. (2013) 368(15):1388–97. doi: 10.1056/NEJMoa1204471
38. Shirvalkar P, Prosky J, Chin G, Ahmadipour P, Sani OG, Desai M, et al. First-in-human prediction of chronic pain state using intracranial neural biomarkers. Nat Neurosci. (2023) 26(6):1090–9. doi: 10.1038/s41593-023-01338-z
39. Fenton BW, Shih E, Zolton J. The neurobiology of pain perception in normal and persistent pain. Pain Manag. (2015) 5(4):297–317. doi: 10.2217/pmt.15.27
40. Necka EA, Lee IS, Kucyi A, Cheng JC, Yu Q, Atlas LY. Applications of dynamic functional connectivity to pain and its modulation. Pain Rep. (2019) 4(4):e752. doi: 10.1097/PR9.0000000000000752
41. Darbari DS, Hampson JP, Ichesco E, Kadom N, Vezina G, Evangelou I, et al. Frequency of hospitalizations for pain and association with altered brain network connectivity in sickle cell disease. J Pain. (2015) 16(11):1077–86. doi: 10.1016/j.jpain.2015.07.005
42. Coates TD, Chalacheva P, Zeltzer L, Khoo MCK. Autonomic nervous system involvement in sickle cell disease. Clin Hemorheol Microcirc. (2018) 68:251–62. doi: 10.3233/ch-189011
43. Wang Y, Hardy SJ, Ichesco E, Zhang P, Harris RE, Darbari DS. Alteration of grey matter volume is associated with pain and quality of life in children with sickle cell disease. Transl Res. (2022) 240:17–25. doi: 10.1016/j.trsl.2021.08.004
44. Santana JERS, Baptista AF, Lucena R, Lopes TDS, do Rosário RS, Xavier MR, et al. Altered dynamic brain connectivity in individuals with sickle cell disease and chronic pain secondary to hip osteonecrosis. Clin EEG Neurosci. (2023) 54(3):333–42. doi: 10.1177/15500594211054297
45. Citero VA, Levenson JL, McClish DK, Bovbjerg VE, Cole PL, Dahman BA, et al. The role of catastrophizing in sickle cell disease—the PiSCES project. Pain. (2007) 133(1-3):39–46. doi: 10.1016/j.pain.2007.02.008
46. Sadler KE, Atkinson SN, Ehlers VL, Waltz TB, Hayward M, Rodríguez García DM, et al. Gut microbiota and metabolites drive chronic sickle cell disease pain. bioRxiv [Preprint]. (2023):538342. doi: 10.1101/2023.04.25.538342
47. el-Hazmi MA, Bahakim HM, al-Fawaz I. Endocrine functions in sickle cell anaemia patients. J Trop Pediatr. (1992) 38(6):307–13. doi: 10.1093/tropej/38.6.307
48. Veluswamy S, Shah P, Denton CC, Chalacheva P, Khoo MCK, Coates TD. Vaso-occlusion in sickle cell disease: is autonomic dysregulation of the microvasculature the trigger? J Clin Med. (2019) 8(10):1690. doi: 10.3390/jcm8101690
49. Levenson JL, McClish DK, Dahman BA, Bovbjerg VE, de A Citero V, Penberthy LT, et al. Depression and anxiety in adults with sickle cell disease: the PiSCES project. Psychosom Med. (2008) 70(2):192–6. doi: 10.1097/PSY.0b013e31815ff5c5
50. Sluka KA, Clauw DJ. Neurobiology of fibromyalgia and chronic widespread pain. Neuroscience. (2016) 338:114–29. doi: 10.1016/j.neuroscience.2016.06.006
51. Ford AC, Sperber AD, Corsetti M, Camilleri M. Irritable bowel syndrome. Lancet. (2020) 396(10263):1675–88. doi: 10.1016/S0140-6736(20)31548-8
52. Lamvu G, Carrillo J, Ouyang C, Rapkin A. Chronic pelvic pain in women: a review. JAMA. (2021) 325(23):2381–91. doi: 10.1001/jama.2021.2631
53. Fitzcharles MA, Cohen SP, Clauw DJ, Littlejohn G, Usui C, Häuser W. Nociplastic pain: towards an understanding of prevalent pain conditions. Lancet. (2021) 397(10289):2098–110. doi: 10.1016/S0140-6736(21)00392-5
54. Ossipov MH, Morimura K, Porreca F. Descending pain modulation and chronification of pain. Curr Opin Support Palliat Care. (2014) 8(2):143–51. doi: 10.1097/SPC.0000000000000055
55. Costigan M, Scholz J, Woolf CJ. Neuropathic pain: a maladaptive response of the nervous system to damage. Annu Rev Neurosci. (2009) 32:1–32. doi: 10.1146/annurev.neuro.051508.135531
56. von Hehn CA, Baron R, Woolf CJ. Deconstructing the neuropathic pain phenotype to reveal neural mechanisms. Neuron. (2012) 73(4):638–52. doi: 10.1016/j.neuron.2012.02.008
57. Brandow AM, Stucky CL, Hillery CA, Hoffmann RG, Panepinto JA. Patients with sickle cell disease have increased sensitivity to cold and heat. Am J Hematol. (2013) 88(1):37–43. doi: 10.1002/ajh.23341
58. Zempsky WT, Wakefield EO, Santanelli JP, New T, Smith-Whitley K, Casella JF, et al. Widespread pain among youth with sickle cell disease hospitalized with vasoocclusive pain: a different clinical phenotype? Clin J Pain. (2017) 33(4):335–9. doi: 10.1097/AJP.0000000000000403
59. McClish DK, Smith WR, Dahman BA, Levenson JL, Roberts JD, Penberthy LT, et al. Pain site frequency and location in sickle cell disease: the PiSCES project. Pain. (2009) 145(1-2):246–51. doi: 10.1016/j.pain.2009.06.029
60. Campbell CM, Moscou-Jackson G, Carroll CP, Kiley K, Jr HC, Lanzkron S, et al. An evaluation of central sensitization in patients with sickle cell disease. J Pain. (2016) 17(5):617–27. doi: 10.1016/j.jpain.2016.01.475
61. Ji RR, Nackley A, Huh Y, Terrando N, Maixner W. Neuroinflammation and central sensitization in chronic and widespread pain. Anesthesiology. (2018) 129(2):343–66. doi: 10.1097/ALN.0000000000002130
62. Donnelly CR, Andriessen AS, Chen G, Wang K, Jiang C, Maixner W, et al. Central nervous system targets: glial cell mechanisms in chronic pain. Neurotherapeutics. (2020) 17(3):846–60. doi: 10.1007/s13311-020-00905-7
63. Arntz A, Claassens L. The meaning of pain influences its experienced intensity. Pain. (2004) 109(1-2):20–5. doi: 10.1016/j.pain.2003.12.030
64. Moseley GL, Arntz A. The context of a noxious stimulus affects the pain it evokes. Pain. (2007) 133(1-3):64–71. doi: 10.1016/j.pain.2007.03.002
65. Price DD. Psychological and neural mechanisms of the affective dimension of pain. Science. (2000) 288(5472):1769–72. doi: 10.1126/science.288.5472.1769
66. Derbyshire SW. Exploring the pain “neuromatrix”. Curr Rev Pain. (2000) 4(6):467–77. doi: 10.1007/s11916-000-0071
67. Eckman JR, Hassell KL, Huggins W, Werner EM, Klings ES, Adams RJ, et al. Standard measures for sickle cell disease research: the PhenX toolkit sickle cell disease collections. Blood Adv. (2017) 1(27):2703–11. doi: 10.1182/bloodadvances.2017010702
68. Hamilton CM, Strader LC, Pratt JG, Maiese D, Hendershot T, Kwok RK, et al. The PhenX toolkit: get the most from your measures. Am J Epidemiol. (2011) 174(3):253–60. doi: 10.1093/aje/kwr193
69. PhenX toolkit release details May 18, 2022, Vver 41.0 (Release includes: 1) Sickle cell disease: pain specialty collection). Available at: https://www.phenxtoolkit.org/about/releases/123
70. Protocol—adult tem (ASCQ-Me). Available at: https://www.phenxtoolkit.org/protocols/view/820201?origin=search
71. Trescot AM, Datta S, Lee M, Hansen H. Opioid pharmacology. Pain Physician. (2008) 11(2 Suppl):S133–53. doi: 10.36076/ppj.2008/11/S133
72. Inturrisi CE. Clinical pharmacology of opioids for pain. Clin J Pain. (2002) 18(4 Suppl):S3–13. doi: 10.1097/00002508-200207001-00002
73. Analgesic Tolerance to Opioids. Pain clinical update. volume IX, no. 5. December 2001, p1. international association for the study of pain. Available at: http://www.iasp-pain.org/AM/AMTemplate.cfm?Section=HOME&CONTENTID=7577&TEMPLATE=/CM/ContentDisplay.cfm&SECTION=HOME (Accessed February 5, 2011).
74. Dampier CD, Setty BN, Logan J, Ioli JG, Dean R. Intravenous morphine pharmacokinetics in pediatric patients with sickle cell disease. J Pediatr. (1995) 126:461–7. doi: 10.1016/S0022-3476(95)70472-8
75. Darbari DS, Minniti CP, Rana S, van den Anker J. Pharmacogenetics of morphine: potential implications in sickle cell disease. Am J Hematol. (2008) 83:233–6. doi: 10.1002/ajh.21027
76. Crofford LJ. Adverse effects of chronic opioid therapy for chronic musculoskeletal pain. Nat Rev Rheumatol. (2010) 6(4):191–7. doi: 10.1038/nrrheum.2010.24
77. Gomes T, Tadrous M, Mamdani MM, Paterson JM, Juurlink DN. The burden of opioid-related mortality in the United States. JAMA Netw Open. (2018) 1(2):e180217. doi: 10.1001/jamanetworkopen.2018.0217
78. Brennan MJ. The effect of opioid therapy on endocrine function. Am J Med. (2013) 126(3 Suppl 1):S12–18. doi: 10.1016/j.amjmed.2012.12.001
79. Nee J, Zakari M, Sugarman MA, Whelan J, Hirsch W, Sultan S, et al. Efficacy of treatments for opioid-induced constipation: systematic review and meta-analysis. Clin Gastroenterol Hepatol. (2018) 16(10):1569–1584.e2. doi: 10.1016/j.cgh.2018.01.021
80. Meehan WJ, Adelman SA, Rehman Z, Khoromi S. Opioid abuse. Available at: http://www.emedicine.com/med/topic1673.htm Last Updated: April 18, 2006. (Accessed August 23, 2006).
81. Han B, Compton WM, Blanco C, Crane E, Lee J, Jones CM. Prescription opioid use, misuse, and use disorders in U.S. adults: 2015 national survey on drug use and health. Ann Intern Med. (2017) 167(5):293–301. doi: 10.7326/M17-0865
82. Jones CM. Heroin use and heroin use risk behaviors among nonmedical users of prescription opioid pain relievers—United States, 2002–2004 and 2008–2010. Drug Alcohol Depend. (2013) 132(1-2):95–100. doi: 10.1016/j.drugalcdep.2013.01.007
83. Jacobson SJ, Kopecky EA, Joshi P, Babul N. Randomised trial of oral morphine for painful episodes of sickle-cell disease in children. Lancet. (1997) 350(9088):1358–61. doi: 10.1016/S0140-6736(97)08462-6
84. Buchanan ID, Woodward M, Reed GW. Opioid selection during sickle cell pain crisis and its impact on the development of acute chest syndrome. Pediatr Blood Cancer. (2005) 45(5):716–24. doi: 10.1002/pbc.20403
85. Tanabe P, Silva S, Bosworth HB, Crawford R, Paice JA, Richardson LD, et al. A randomized controlled trial comparing two vaso-occlusive episode (VOE) protocols in sickle cell disease (SCD). Am J Hematol. (2018) 93(2):159–68. doi: 10.1002/ajh.24948
86. Santos J, Jones S, Wakefield D, Grady J, Andemariam B. Patient controlled analgesia for adults with sickle cell disease awaiting admission from the emergency department. Pain Res Manag. (2016) 2016:3218186. doi: 10.1155/2016/3218186
87. Andemariam B, Odesina V, Owarish-Gross J, Santos J, Grady J, Gorenbeyn A, et al. Timely analgesia, reduced hospitalization rate, and improved economic efficiency following implementation of a new emergency department sickle cell pain management algorithm. J Blood Disord Transfus. (2014) 5:229. doi: 10.4172/2155-9864.1000229
88. -Kavanagh PL, Sprinz PG, Wolfgang TL, Killius K, Champigny M, Sobota A, et al. Improving the management of vaso-occlusive episodes in the pediatric emergency department. Pediatrics. (2015) 136(4):e1016–25. doi: 10.1542/peds.2014-3470
89. Furlan A, Chaparro LE, Irvin E, Mailis-Gagnon A. A comparison between enriched and nonenriched enrollment randomized withdrawal trials of opioids for chronic noncancer pain. Pain Res Manag. (2011) 16:337–51. doi: 10.1155/2011/465281
90. American Pain Society, American Academy of Pain Medicine Opioids Guidelines Panel. Guideline for the use of chronic opioid therapy in chronic noncancer pain: evidence review. Chicago, IL: American Pain Society (2009).
91. Chou R, Deyo R, Devine B, Hansen R, Sullivan S, Jarvik JG, et al. The effectiveness and risks of long-term opioid treatment of chronic pain. Evidence report/technology assessment No. 218. AHRQ publication No. 14-E005-EF. Rockville, MD: Agency for Healthcare Research and Quality (2014). doi: 10.23970/AHRQEPCERTA218
92. Ruta NS, Ballas SK. The opioid drug epidemic and sickle cell disease: guilt by association. Pain Med. (2016) 17(10):1793–8. doi: 10.1093/pm/pnw074
93. Akinboro OA, Nwabudike S, Edwards C, Cirstea D, Addo-Tabiri NO, Voisine M, et al. Opioid Use Is NOT Associated with in-Hospital Mortality Among Patients with Sickle CELL Disease in the United States: findings from the National Inpatient Sample. Blood. (2018):132(Suppl 1):315. doi: 10.1182/blood-2018-99-115573
94. Ballas SK, Kanter J, Agodoa I, Howard R, Wade S, Noxon V, et al. Opioid utilization patterns in United States individuals with sickle cell disease. Am J Hematol. (2018) 93(10):E345–7. doi: 10.1002/ajh.25233
95. Dowell D, Haegerich TM, Chou R. CDC guideline for prescribing opioids for chronic pain–United States, 2016. JAMA. (2016) 315(15):1624–45. doi: 10.1001/jama.2016.1464
96. Dowell D, Ragan KR, Jones CM, Baldwin GT, Chou R. CDC clinical practice guideline for prescribing opioids for pain — united States, 2022. MMWR Recomm Rep. (2022) 71(No. RR-3):1–95. doi: 10.15585/mmwr.rr7103a1
97. Jahr JS, Lee VK. Intravenous acetaminophen. Anesthesiol Clin. (2010) 28(4):619–45. doi: 10.1016/j.anclin.2010.08.006
98. Moller PL, Sindet-Pedersen S, Petersen CT, Juhl GI, Dillenschneider A, Skoglund LA. Onset of acetaminophen analgesia: comparison of oral and intravenous routes after third molar surgery. Br J Anaesth. (2005) 94(5):642–8. doi: 10.1093/bja/aei109
99. Dart RC, Bailey E. Does therapeutic use of acetaminophen cause acute liver failure? Pharmacotherapy. (2007) 27:1219–30. doi: 10.1592/phco.27.9.1219
100. Larson AM. Acetaminophen hepatotoxicity. Clin Liver Dis. (2007) 11:525–48. doi: 10.1016/j.cld.2007.06.006
101. Krasniak AE, Knipp GT, Svensson CK, Liu W. Pharmacogenomics of acetaminophen in pediatric populations: a moving target. Front Genet. (2014) 5:314. doi: 10.3389/fgene.2014.00314
102. Okomo U, Meremikwu MM. Fluid replacement therapy for acute episodes of pain in people with sickle cell disease. Cochrane Database Syst Rev. (2017) 7:CD005406. doi: 10.1002/14651858CD005406.pub5
103. Sins JWR, Mager DJ, Davis SCAT, Biemond BJ, Fijnvandraat K. Pharmacotherapeutical strategies in the prevention of acute, vaso-occlusive pain in sickle cell disease: a systematic review. Blood Adv. (2017) 1(19):1598–616. doi: 10.1182/bloodadvances.2017007211
104. Nevitt SJ, Jones AP, Howard J. Hydroxyurea (hydroxycarbamide) for sickle cell disease. Cochrane Database Syst Rev. (2017) 4:CD002202. doi: 10.1002/14651858.CD002202.pub2
105. L-glutamine (endari) for sickle cell disease. Med Lett Drugs Ther. (2018) 60(1539):21–2.29364198
106. Han J, Saraf SL, Gordeuk VR. Systematic review of voxelotor: a first-in-class sickle hemoglobin polymerization inhibitor for management of sickle cell disease. Pharmacotherapy. (2020) 40(6):525–34. doi: 10.1002/phar.2405
107. Ataga KI, Kutlar A, Kanter J, Liles D, Cancado R, Friedrisch J, et al. Crizanlizumab for the prevention of pain crises in sickle cell disease. N Engl J Med. (2017) 376(5):429–39. doi: 10.1056/NEJMoa1611770
108. Inati A, Mansour AG, Sabbouh T, Amhez G, Hachem A, Abbas HA. Transfusion therapy in children with sickle cell disease. J Pediatr Hematol Oncol. (2017) 39(2):126–32. doi: 10.1097/MPH.0000000000000645
109. Kassim AA, Sharma D. Hematopoietic stem cell transplantation for sickle cell disease: the changing landscape. Hematol Oncol Stem Cell Ther. (2017) 10(4):259–66. doi: 10.1016/j.hemonc.2017.05.008
110. Reeves SL, Dombkowski KJ, Peng HK, Phan H, Kolenic G, Creary SE, et al. Adherence to hydroxyurea and clinical outcomes among children with sickle cell anemia. Pediatr Blood Cancer. (2023) 70(7):e30332. doi: 10.1002/pbc.30332
111. Kang HA, Barner JC. The association between hydroxyurea adherence and opioid utilization among Texas medicaid enrollees with sickle cell disease. J Manag Care Spec Pharm. (2020) 26(11):1412–22. doi: 10.18553/jmcp.2020.26.11.1412
112. Kang HA, Barner JC, Lawson KA, Rascati K, Mignacca RC. Impact of adherence to hydroxyurea on health outcomes among patients with sickle cell disease. Am J Hematol. (2023) 98(1):90–101. doi: 10.1002/ajh.26765
113. Steinberg MH, McCarthy WF, Castro O, Ballas SK, Armstrong FD, Smith W, et al. The risks and benefits of long-term use of hydroxyurea in sickle cell anemia: a 17.5 year follow-up. Am J Hematol. (2010) 85(6):403–8. doi: 10.1002/ajh.21699
114. Smith WR, Ballas SK, McCarthy WF, Bauserman RL, Swerdlow PS, Steinberg MH, et al. The association between hydroxyurea treatment and pain intensity, analgesic use, and utilization in ambulatory sickle cell anemia patients. Pain Med. (2011) 12(5):697–705. doi: 10.1111/j.1526-4637.2011.01096.x
115. Linder GE, Chou ST. Red cell transfusion and alloimmunization in sickle cell disease. Haematologica. (2021) 106(7):1805–15. doi: 10.3324/haematol.2020.270546
116. Dedeken L, Lê PQ, Rozen L. Automated RBC exchange compared to manual exchange transfusion for children with sickle cell disease is cost-effective and reduces iron overload. Transfusion. (2018) 58(6):1356–62. doi: 10.1111/trf.14575|PubMed|GoogleScholar
117. Tsitsikas DA, Ekong A, Berg L. A 5- year cost analysis of automated red cell exchange transfusion for the management of recurrent painful crises in adult patients with sickle cell disease. Transfus Apher Sci. (2017) 56(3):466–9. doi: 10.1016/j.transci.2017.05.020
118. Darbari DS, Liljencrantz J, Ikechi A, Martin S, Roderick MC, Fitzhugh CD, et al. Pain and opioid use after reversal of sickle cell disease following HLA-matched sibling haematopoietic stem cell transplant. Br J Haematol. (2019) 184(4):690–3. doi: 10.1111/bjh.15169
119. Han J, Holden CC, Ahluwalia AY, Molokie RE, Gowhari M, Sweiss K, et al. Chronic opioid use can be reduced or discontinued after haematopoietic stem cell transplantation for sickle cell disease. Br J Haematol. (2020) 191(3):e70–2. doi: 10.1111/bjh.16999
120. Bakshi N, Astles R, Chou E, Hurreh A, Sil S, Sinha CB, et al. Multimodal phenotyping and correlates of pain following hematopoietic cell transplant in children with sickle cell disease. Pediatr Blood Cancer. (2023) 70(1):e30046. doi: 10.1002/pbc.30046
121. Han J, Saraf SL, Lash JP, Gordeuk VR. Use of anti-inflammatory analgesics in sickle-cell disease. J Clin Pharm Ther. (2017) 42(5):656–60. doi: 10.1111/jcpt.12592
122. Brune K, Hinz B. The discovery and development of antiinflammatory drugs. Arthritis Rheum. (2004) 50(8):2391–9. doi: 10.1002/art.20424
123. Elia N, Lysakowski C, Tramèr MR. Does multimodal analgesia with acetaminophen, nonsteroidal antiinflammatory drugs, or selective cyclooxygenase-2 inhibitors and patient-controlled analgesia morphine offer advantages over morphine alone? Meta-analyses of randomized trials. Anesthesiology. (2005) 103(6):1296–304. doi: 10.1097/00000542-200512000-00025
124. Straube S, Derry S, McQuay HJ, Moore RA. Effect of preoperative cox-II-selective NSAIDs (coxibs) on postoperative outcomes: a systematic review of randomized studies. Acta Anaesthesiol Scand. (2005) 49(5):601–13. doi: 10.1111/j.1399-6576.2005.00666
125. Bartolucci P, El Murr T, Roudot-Thoraval F, Habibi A, Santin A, Renaud B, et al. A randomized, controlled clinical trial of ketoprofen for sickle-cell disease vaso-occlusive crises in adults. Article. Blood. (2009) 114(18):3742–7. doi: 10.1182/blood-2009-06-227330
126. Hardwick WE J, Givens TG, Monroe KW, King WD, Lawley D. Effect of ketorolac in pediatric sickle cell vaso-occlusive pain crisis. Pediatr Emerg Care. (1999) 15(3):179–82. doi: 10.1097/00006565-199906000-00004
127. Perlin E, Finke H, Castro O, Rana S, Pittman J, Burt R, et al. Enhancement of pain control with ketorolac tromethamine in patients with sickle cell vaso-occlusive crisis. Am J Hematol. (1994) 46(1):43–7. doi: 10.1002/ajh.2830460108
128. Wright SW, Norris RL, Mitchell TR. Ketorolac for sickle cell vaso-occlusive crisis pain in the emergency department: lack of a narcotic-sparing effect. Ann Emerg Med. (1992) 21(8):925–8. doi: 10.1016/s0196-0644(05)82929-4
129. Wright SW, Norris RL, Mitchell TR. Ketorolac for sickle cell vaso-occlusive crisis pain in the emergency department: lack of a narcotic-sparing effect. Ann Emerg Med. (1992) 21(8):925–8. doi: 10.1016/S0196-0644(05)82929-4
130. de Franceschi L, Finco G, Vassanelli A, Zaia B, Ischia S, Corrocher R. A pilot study on the efficacy of ketorolac plus tramadol infusion combined with erythrocytapheresis in the management of acute severe vaso-occlusive crises and sickle cell pain. Haematologica. (2004) 89(11):1389–91.15531461
131. Beiter JL, Simon HK, Chambliss CR, Adamkiewicz T, Sullivan K. Intravenous ketorolac in the emergency department management of sickle cell pain and predictors of its effectiveness. Arch Pediatr Adolesc Med. (2001) 155(4):496–500. doi: 10.1001/archpedi.155.4.496
132. Baker M, Perazella MA. NSAIDs in CKD: are they safe? Am J Kidney Dis. (2020) 76(4):546–57. doi: 10.1053/j.ajkd.2020.03.023
133. Sadler KE, Stucky CL. Blocking COX-2 for sickle cell pain relief. Review. Blood. (2019) 133(18):1924–5. doi: 10.1182/blood-2019-03-900944
134. Singh J, Sharma D, Bansal R. Pyridazinone: an attractive lead for anti-inflammatory and analgesic drug discovery. Review. Future Med Chem. (2017) 9(1):95–127. doi: 10.4155/fmc-2016-0194
135. Caudle KE, Dunnenberger HM, Freimuth RR, Peterson JF, Burlison JD, Whirl-Carrillo M, et al. Standardizing terms for clinical pharmacogenetic test results: consensus terms from the clinical pharmacogenetics implementation consortium (CPIC). Genet Med. (2017) 19(2):215–23. doi: 10.1038/gim.2016.87
136. Brandl E, Halford Z, Clark MD, Herndon C. Pharmacogenomics in pain management: a review of relevant gene-drug associations and clinical considerations. Ann Pharmacother. (2021) 55:1486–501. doi: 10.1177/10600280211003875
137. Mion G, Villevieille T. Ketamine pharmacology: an update (pharmacodynamics and molecular aspects, recent findings). CNS Neurosci Ther. (2013) 19(6):370–80. doi: 10.1111/cns.12099
138. Koppert W, Sittl R, Scheuber K, Alsheimer M, Schmelz M, Schüttler J. Differential modulation of remifentanil-induced analgesia and postinfusion hyperalgesia by S-ketamine and clonidine in humans. Anesthesiology. (2003) 99(1):152–9. doi: 10.1097/00000542-200307000-00025
139. Raja SN, Sivanesan E, Guan Y. Central sensitization, N-methyl-D-aspartate receptors, and human experimental pain models: bridging the gap between target discovery and drug development. Anesthesiology. (2019) 131(2):233–5. doi: 10.1097/aln.0000000000002808
140. Latremoliere A, Woolf CJ. Central sensitization: a generator of pain hypersensitivity by central neural plasticity. J Pain. (2009) 10(9):895–926. doi: 10.1016/j.jpain.2009.06.012
141. Meshkat S, Rodrigues NB, Di Vincenzo JD, Ceban F, Jaberi S, McIntyre RS, et al. Pharmacogenomics of ketamine: a systematic review. J Psychiatr Res. (2021) 145:27–34. doi: 10.1016/j.jpsychires.2021.11.036
142. Neri CM, Pestieau SR, Darbari DS. Low-dose ketamine as a potential adjuvant therapy for painful vaso-occlusive crises in sickle cell disease. Paediatr Anaesth. (2013) 23(8):684–9. doi: 10.1111/pan.12172
143. Uprety D, Baber A, Foy M. Ketamine infusion for sickle cell pain crisis refractory to opioids: a case report and review of literature. Ann Hematol. (2014) 93(5):769–71. doi: 10.1007/s00277-013-1954-3
144. Schwenk ES, Viscusi ER, Buvanendran A, Hurley RW, Wasan AD, Narouze S, et al. Consensus guidelines on the use of intravenous ketamine infusions for acute pain management from the American society of regional anesthesia and pain medicine, the American academy of pain medicine, and the American society of anesthesiologists. Reg Anesth Pain Med. (2018) 43(5):456–66. doi: 10.1097/AAP.0000000000000806
145. Finnerup NB, Sindrup SH, Jensen TS. The evidence for pharmacological treatment of neuropathic pain. Pain. (2010) 150:573–81. doi: 10.1016/j.pain.2010.06.019
146. Luo ZD, Chaplan SR, Higuera ES, Sorkin LS, Stauderman KA, Williams ME, et al. Upregulation of dorsal root ganglion (alpha)2(delta) calcium channel subunit and its correlation with allodynia in spinal nerve-injured rats. J Neurosci. (2001) 21(6):1868–75. doi: 10.1523/JNEUROSCI.21-06-01868.2001
147. Colloca L, Ludman T, Bouhassira D, Baron R, Dickenson AH, Yarnitsky D, et al. Neuropathic pain. Nat Rev Dis Prim. (2017) 3:17002. doi: 10.1038/nrdp.2017.2
148. Mishriky BM, Waldron NH, Habib AS. Impact of pregabalin on acute and persistent postoperative pain: a systematic review and meta-analysis. Br J Anaesth. (2022) 114:10–31. doi: 10.1093/bja/aeu293
149. Martinez V, Pichard X, Fletcher D. Perioperative pregabalin administration does not prevent chronic postoperative pain. Pain. (2017) 158:775–83. doi: 10.1097/j.pain.0000000000000838
150. Chincholkar M. Analgesic mechanisms of gabapentinoids and effects in experimental pain models: a narrative review. Br J Anaesth. (2018) 120:1315–34. doi: 10.1016/j.bja.2018.02.066
151. Hermanns H, Hollmann MW, Stevens MF, Lirk P, Brandenburger T, Piegeler T, et al. Molecular mechanisms of action of systemic lidocaine in acute and chronic pain: a narrative review. Br J Anaesth. (2019) 123(3):335–49. doi: 10.1016/j.bja.2019.06.014
152. Boas RA, Covino BG, Shahnarian A. Analgesic responses to i.v. lignocaine. Br J Anaesth. (1982) 54(5):501–5. doi: 10.1093/bja/54.5.501
153. Bartlett EE, Hutserani O. Xylocaine for the relief of postoperative pain. Anesth Analg. (1961) 40:296–304. doi: 10.1213/00000539-196105000-00011
154. Rousseau V, Morelle M, Arriuberge C, Darnis S, Chabaud S, Launay V, et al. Efficacy and tolerance of lidocaine 5% patches in neuropathic pain and pain related to vaso-occlusive sickle cell crises in children: a prospective multicenter clinical study. Pain Pract. (2018) 18(6):788–97. doi: 10.1111/papr.12674
155. Nguyen NL, Kome AM, Lowe DK, Coyne P, Hawks KG. Intravenous lidocaine as an adjuvant for pain associated with sickle cell disease. J Pain Palliat Care Pharmacother. (2015) 29(4):359–64. doi: 10.3109/15360288.2015.1082009
156. Beaussier M, Delbos A, Maurice-Szamburski A, Ecoffey C, Mercadal L. Perioperative use of intravenous lidocaine. Drugs. (2018) 78:1229–46. doi: 10.1007/s40265-018-0955-x
157. Cohen M, Sadhasivam S, Vinks AA. Pharmacogenetics in perioperative medicine. Curr Opin Anaesthesiol. (2012) 25:419–27. doi: 10.1097/ACO.0b013e3283556129
158. Blakely RD, De Felice LJ, Hartzell HC. Molecular physiology of norepinephrine and serotonin transporters. J Exp Biol. (1994) 196:263–81. doi: 10.1242/jeb.196.1.263
159. Sangkuhl K, Klein TE, Altman RB. PharmGKB summary: citalopram pharmacokinetics pathway. Pharmacogenet Genomics. (2011) 21:769–72. doi: 10.1097/FPC.0b013e328346063f
160. Cooper TE, Heathcote LC, Clinch J, Gold JI, Howard R, Lord SM, et al. Antidepressants for chronic non-cancer pain in children and adolescents. Cochrane Database Syst Rev. (2017) 8(8):CD012535. doi: 10.1002/14651858.CD012535.pub2
161. Finnerup NB, Attal N, Haroutounian S, McNicol E, Baron R, Dworkin RH, et al. Pharmacotherapy for neuropathic pain in adults: a systematic review and meta-analysis. Lancet Neurol. (2015) 14(2):162–73. doi: 10.1016/S1474-4422(14)70251-0
162. Zobdeh F, Eremenko II, Akan MA, Tarasov VV, Chubarev VN, Schiöth HB, et al. Pharmacogenetics and pain treatment with a focus on non-steroidal anti-inflammatory drugs (NSAIDs) and antidepressants: a systematic review. Pharmaceutics. (2022) 14:1190. doi: 10.3390/pharmaceutics14061190
163. Osunkwo I. Complete resolution of sickle cell chronic pain with high dose vitamin D therapy: a case report and review of the literature. J Pediatr Hematol Oncol. (2011) 33(7):549–51. doi: 10.1097/MPH.0b013e31821ed3ea
164. Soe HH, Abas AB, Than NN, Ni H, Singh J, Said AR, et al. Vitamin D supplementation for sickle cell disease. Cochrane Database Syst Rev. (2017) 1:CD010858. doi: 10.1002/14651858.CD010858.pub2
165. Aich A, Jones MK, Gupta K. Pain and sickle cell disease. Curr Opin Hematol. (2019) 26(3):131–8. doi: 10.1097/MOH.0000000000000491
166. He Y, Chen Y, Tian X, Yang C, Lu J, Xiao C, et al. CaMKIIα underlies spontaneous and evoked pain behaviors in Berkeley sickle cell transgenic mice. Pain. (2016) 157(12):2798–806. doi: 10.1097/j.pain.0000000000000704
167. Molokie RE, Wilkie DJ, Wittert H, Suarez ML, Yao Y, Zhao Z, et al. Mechanism-driven phase I translational study of trifluoperazine in adults with sickle cell disease. Eur J Pharmacol. (2014) 723:419–24. doi: 10.1016/j.ejphar.2013.10.062
168. He Y, Wilkie DJ, Nazari J, Wang R, Messing RO, DeSimone J, et al. PKCδ-targeted intervention relieves chronic pain in a murine sickle cell disease model. J Clin Invest. (2016) 126(8):3053–7. doi: 10.1172/JCI86165
169. Vang D, Paul JA, Nguyen J, Tran H, Vincent L, Yasuda D, et al. Small-molecule nociceptin receptor agonist ameliorates mast cell activation and pain in sickle mice. Haematologica. (2015) 100(12):1517–25. doi: 10.3324/haematol.2015.128736
170. Vincent L, Vang D, Nguyen J, Benson B, Lei J, Gupta K. Cannabinoid receptor-specific mechanisms to alleviate pain in sickle cell anemia inhibition of mast cell activation and neurogenic inflammation. Haematologica. (2016) 101(5):566–77. doi: 10.3324/haematol.2015.136523
171. Kohli DR, Li Y, Khasabov SG, Gupta P, Kehl LJ, Ericson ME, et al. Pain-related behaviors and neurochemical alterations in mice expressing sickle hemoglobin: modulation by cannabinoids. Blood. (2010) 116(3):456–65. doi: 10.1182/blood-2010-01-260372
172. Tran H, Gupta M, Gupta K. Targeting novel mechanisms of pain in sickle cell disease. Blood. (2017) 130:2377–85. doi: 10.1182/blood-2017-05-782003
173. Gupta K, Jahagirdar O, Gupta K. Targeting pain at its source in sickle cell disease. Am J Physiol Integr Comp Physiol. (2018) 315:R104–12. doi: 10.1152/ajpregu.00021.2018
174. Xiong HY, Cao YQ, Du SH, Yang QH, He SY, Wang XQ. Effects of high-definition transcranial direct current stimulation targeting the anterior cingulate cortex on the pain thresholds: a randomized controlled trial. Pain Med. (2023) 24(1):89–98. doi: 10.1093/pm/pnac135
175. Mittal AM, Tran H, Sagi V, Nguyen A, Luk K, Nguyen J, et al. Mast cells contribute to brain microvascular permeability in sickle cell disease. Blood. (2018) 132:269. doi: 10.1182/blood-2018-99-117246
176. Jing X, Wang T, Huang S, Glorioso JC, Albers KM. The transcription factor Sox11 promotes nerve regeneration through activation of the regeneration-associated gene Sprr1a. Exp Neurol. (2012) 233(1):221–32. doi: 10.1016/j.expneurol.2011.10.005
177. Starkey ML, Davies M, Yip PK, Carter LM, Wong DJ, McMahon SB, et al. Expression of the regeneration-associated protein SPRR1A in primary sensory neurons and spinal cord of the adult mouse following peripheral and central injury. J Comp Neurol. (2009) 513(1):51–68. doi: 10.1002/cne.21944
178. Buchheit T, Huh Y, Maixner W, Cheng J, Ji RR. Neuroimmune modulation of pain and regenerative pain medicine. J Clin Invest. (2020) 130(5):2164–76. doi: 10.1172/JCI134439
179. van Veelen S, Vuong C, Gerritsma JJ, Eckhardt CL, Verbeek SEM, Peters M, et al. Efficacy of non-pharmacological interventions to reduce pain in children with sickle cell disease: a systematic review. Pediatr Blood Cancer. (2023) 70(6):e30315. doi: 10.1002/pbc.30315
180. Mahmood LA, Thaniel L, Martin B, Marguiles S, Reece-Stremtan S, Idiokitas R, et al. Integrative holistic approaches for children, adolescents, and young adults with sickle cell disease: a single center experience. Complement Ther Med. (2021) 60:102680. doi: 10.1016/j.ctim.2021.102680
181. Badawy SM, Abebe KZ, Reichman CA, Checo G, Hamm ME, Stinson J, et al. Comparing the effectiveness of education versus digital cognitive behavioral therapy for adults with sickle cell disease: protocol for the cognitive behavioral therapy and real-time pain management intervention for sickle cell via Mobile applications (CaRISMA) study. JMIR Res Protoc. (2021) 10(5):e29014. doi: 10.2196/29014
182. Williams H, Silva S, Simmons LA, Tanabe P. A telephonic mindfulness-based intervention for persons with sickle cell disease: study protocol for a randomized controlled trial. Trials. (2017) 18(1):218. doi: 10.1186/s13063-017-1948-x
183. Plonski KS. Acupuncture for pain management in pediatric patients with sickle cell disease. Children (Basel). (2022) 9(7):1076. doi: 10.3390/children9071076
184. Li H, Patil CL, Molokie RE, Njoku F, Steffen AD, Doorenbos AZ, et al. Acupuncture for chronic pain in adults with sickle cell disease: a mixed-methods pilot study. Acupunct Med. (2021) 39(6):612–8. doi: 10.1177/09645284211017303
185. Lu K, Cheng MC, Ge X, Berger A, Xu D, Kato GJ, et al. A retrospective review of acupuncture use for the treatment of pain in sickle cell disease patients: descriptive analysis from a single institution. Clin J Pain. (2014) 30(9):825–30. doi: 10.1097/AJP.0000000000000036
186. Co LL, Schmitz TH, Havdala H, Reyes A, Westerman MP. Acupuncture: an evaluation in the painful crises of sickle cell anaemia. Pain. (1979) 7(2):181–5. doi: 10.1016/0304-3959(79)90009-5
187. Sodipo J. Acupuncture and blood studies in sickle-cell anemia. Am J Chin Med. (1993) 21(1):85–9. doi: 10.1142/S0192415X9300011X
188. Doorenbos AZ, Schlaeger JM, deMartelly VA, Burke LA, Boyd AD, Knisely MR, et al. Hybrid effectiveness-implementation trial of guided relaxation and acupuncture for chronic sickle cell disease pain (GRACE): a protocol. Contemp Clin Trials Commun. (2023) 32:101076. doi: 10.1016/j.conctc.2023.101076
189. Zanoni CT, Galvão F, Cliquet Junior A, Saad ST. Pilot randomized controlled trial to evaluate the effect of aquatic and land physical therapy on musculoskeletal dysfunction of sickle cell disease patients. Rev Bras Hematol Hemoter. (2015) 37(2):82–9. doi: 10.1016/j.bjhh.2014.11.010
190. Zanoni CT, Galvão F, Maria RM, Zulli R, Ricciardi JB, Junior CA, et al. Effectiveness of a home-based therapeutic exercise program on lower back pain and functionality in sickle cell disease (SCD) patients. Hematol Transfus Cell Ther. (2021) 43(3):268–79. doi: 10.1016/j.htct.2020.05.004
191. Rodgers-Melnick SN, Lin L, Gam K, Souza de Santana Carvalho E, Jenerette C, Rowland DY, et al. Effects of music therapy on quality of life in adults with sickle cell disease (MUSIQOLS): a mixed methods feasibility study. J Pain Res. (2022) 15:71–91. Erratum in: J Pain Res. 2022;15:1123-1124. doi: 10.2147/JPR.S337390
Keywords: pain, sickle cell disease, integrative medicine, precision medicine, pain neuromatrix
Citation: Smith WR, Valrie CR, Jaja C and Kenney MO (2023) Precision, integrative medicine for pain management in sickle cell disease. Front. Pain Res. 4:1279361. doi: 10.3389/fpain.2023.1279361
Received: 17 August 2023; Accepted: 24 October 2023;
Published: 9 November 2023.
Edited by:
Kalpna Gupta, University of California, Irvine, United StatesReviewed by:
Keesha Roach, University of Tennessee Health Science Center (UTHSC), United StatesKofi Anie, London North West University Healthcare NHS Trust, United Kingdom
© 2023 Smith, Valrie, Jaja and Kenney. This is an open-access article distributed under the terms of the Creative Commons Attribution License (CC BY). The use, distribution or reproduction in other forums is permitted, provided the original author(s) and the copyright owner(s) are credited and that the original publication in this journal is cited, in accordance with accepted academic practice. No use, distribution or reproduction is permitted which does not comply with these terms.
*Correspondence: Wally R. Smith V2FsbHkuc21pdGhAdmN1aGVhbHRoLm9yZw==