- 1Center for Adaptive Systems of Brain Body Interactions, George Mason University, Fairfax, VA, United States
- 2Department of Bioengineering, George Mason University, Fairfax, VA, United States
- 3Department of Human Health and Nutritional Sciences, University of Guelph, Guelph, CA, United States
- 4Rehabilitation Medicine Department, National Institutes of Health Clinical Center, Bethesda, MD, United States
- 5Department of Rehabilitation Medicine, New York University Grossman School of Medicine, New York City, NY, United States
- 6Optimal Motion Inc, Herndon, VA, United States
- 7Faculty of Medicine, University of São Paolo, São Paulo, Brazil
- 8Department of Medicine, INOVA Health System, Fairfax, VA, United States
Low back pain (LBP) is the leading cause of disability worldwide. Most LBP is non-specific or idiopathic, which is defined as symptoms of unknown origin without a clear specific cause or pathology. Current guidelines for clinical evaluation are based on ruling out underlying serious medical conditions, but not on addressing underlying potential contributors to pain. Although efforts have been made to identify subgroups within this population based on response to treatment, a comprehensive framework to guide assessment is still lacking. In this paper, we propose a model for a personalized mechanism-based assessment based on the available evidence that seeks to identify the underlying pathologies that may initiate and perpetuate central sensitization associated with chronic non-specific low back pain (nsLBP). We propose that central sensitization can have downstream effects on the “myofascial unit”, defined as an integrated anatomical and functional structure that includes muscle fibers, fascia (including endomysium, perimysium and epimysium) and its associated innervations (free nerve endings, muscle spindles), lymphatics, and blood vessels. The tissue-level abnormalities can be perpetuated through a vicious cycle of neurogenic inflammation, impaired fascial gliding, and interstitial inflammatory stasis that manifest as the clinical findings for nsLBP. We postulate that our proposed model offers biological plausibility for the complex spectrum of clinical findings, including tissue-level abnormalities, biomechanical dysfunction and postural asymmetry, ecological and psychosocial factors, associated with nsLBP. The model suggests a multi-domain evaluation that is personalized, feasible and helps rule out specific causes for back pain guiding clinically relevant management. It may also provide a roadmap for future research to elucidate mechanisms underlying this ubiquitous and complex problem.
Introduction
Low back pain (LBP) is the leading cause of disability globally with an average prevalence of 39% in the United States (1–3). LBP presents significant financial burden to society (4). Low back and neck pain are the highest contributors to healthcare spending in the US, exceeding $135 billion in 2016 (5). It has been estimated that up to 90% of LBP is non-specific (6, 7), meaning symptoms of unknown origin without a clear, identifiable and specific cause or pathology. This lack of clarity presents a major challenge with diagnosis and appropriate treatment. Consequently, current clinical practice guidelines (8, 9) typically recommend diagnostic triage through physical examination and history taking to rule out underlying “red flags” (e.g., malignancy, fracture or infection), neurological testing to identify radiculopathy, and assessment of psychosocial factors. These guidelines do not offer mechanism-based management strategies targeted to individual patients with LBP who do not present with red flags or radiculopathy, without which poor clinical outcomes are more likely in this population (10). Given the rapidly growing physical and financial burden of LBP, there is an urgent need to identify phenotypes among patients with non-specific LBP (nsLBP) that have the potential for personalized management, and can lead to testable hypotheses about underlying mechanisms.
It is recognized that nsLBP is a complex multifaceted pain condition encompassing biological and psychosocial domains (11). However, the underlying pathophysiologic mechanisms are still poorly understood. A substantial body of literature exists on the association of biomechanical (12–14) and psychosocial factors (15–17) in nsLBP. More recently, the term nociplastic pain has been proposed in the literature (18) to explain the manifestation of neuroplastic maladaptations commonly observed within the nociceptive pathways of nsLBP patients in the absence of verifiable pathology. Significant gaps in our understanding still exist surrounding the complexity of tissue-level interactions among the sensory, motor, vascular, lymphatic, immune and sympathetic nervous systems, biomechanical and postural factors, and their relationship to central mechanisms, psychosocial contributors and even environmental factors. Emerging research suggests that all these components merit consideration if we are to be successful in properly diagnosing and treating this multi-factorial problem (19, 20).
In this paper, we describe a new model that seeks to provide a linkage between the tissue-level biological and physiological abnormalities, biomechanical and postural abnormalities, and abnormalities in the central nervous system. Central to this model is the concept of the myofascial unit (19), defined as an integrated anatomical and functional structure that includes muscle fibers, fascia (including endomysium, perimysium and epimysium) and its associated innervations (free nerve endings, muscle spindles), lymphatics, and blood vessels. Our model describes how central mechanisms of segmental sensitization, perpetuated by persistent nociceptive bombardment from somatic or visceral pathologies, can cause downstream dysfunction at the myofascial unit in the low back, and in turn lead to abnormal biomechanics and posture. Segmental sensitization in the spinal cord can be modulated through descending mechanisms, providing a plausible link between psychosocial factors and tissue-level abnormalities.
Our model is informed by clinical observations about nsLBP (including deep, achy and diffuse regional pain, palpable physical findings in affected muscles, altered range of motion, and absence of a nociceptive withdrawal reflex), as well as another non-specific musculoskeletal pain condition: myofascial pain syndrome (MPS) (21–24), whose clinical findings overlap with nsLBP (25). A hallmark of MPS is a localized MTrP, which many clinicians define as a “discrete palpable hyperirritable locus within a taut band of a skeletal muscle that elicits a referral sensation with pressure application” (26). MTrPs are prevalent in the quadratus lumborum muscle of nsLBP patients (25, 27–29). Emerging research is beginning to highlight the key foundational role of central sensitization (CS) and neurogenic inflammation, along with tissue-level interactions in MPS, that include muscle, lymphatics, blood vessels, nerves and fascia, collectively referred to as the “myofascial unit” (19, 30). This conceptual shift away from MTrPs and towards the more comprehensive and integrated idea of the myofascial unit (30) is a step forward in paving the way for a more comprehensive assessment and management strategy informed by multidimensional, patient-specific and mechanism-based diagnostic and treatment criteria. The biological mechanisms at the myofascial unit may offer important mechanistic insight into the clinical manifestation of nsLBP. Our model is also informed by new experimental data that helps link mechanisms of central sensitization, a common component of all chronic pain syndromes, to dysfunction at the myofascial unit through the mechanism of neurogenic inflammation (31–34). Finally, our model is informed by a new understanding of the critical role played by the fascia and loose connective tissue in biomechanical force transmission and postural control (30, 35, 36), and abnormal fascial gliding associated with myofascial unit dysfunction (37).
Hypothesis: unified model linking the myofascial unit dysfunction to central sensitization and neurogenic inflammation
Several studies have reported soft tissue findings associated with non-musculoskeletal conditions of visceral hypersensitivity such as irritable bowel syndrome and chronic pelvic pain (38–40). This raises the possibility that one contributor to myofascial unit dysfunction may be from primary pathology residing within somatic or visceral tissues (31), such as facet joints, irritable bowel, endometriosis, etc. Although the causal mechanisms are still being investigated, a plausible mechanism is that persistent nociceptive bombardment from these underlying somatic or visceral pathologies can lead to sensitization in the spinal segment, that can subsequently activate dorsal root reflexes that trigger antidromic release of proinflammatory neuropeptides into peripheral tissues to produce neurogenic inflammation and, if allowed to persist (i.e., chronic), can enable complex tissue-level interactions that contribute to the clinical manifestation of myofascial unit dysfunction.
The recently proposed Neurogenic Hypothesis (31, 32, 41) postulates the biological foundation of this mechanism. This hypothesis suggests that persistent nociceptive input from a primary pathology of somatic or visceral origin leads to the induction of central sensitization within the dorsal horn of segment(s) innervating the pathologic tissue. Central sensitization is a maladaptive state of hyperexcitability within the central nervous system (42, 43). Central sensitization can trigger primary afferent depolarization and dorsal root reflexes within the dorsal horn, leading to the antidromic release of proinflammatory neuropeptides (e.g., substance P, CGRP) peripherally into segmentally-linked tissues (both somatic and/or visceral) via small unmyelinated fibers (31–33). These neuropeptides trigger a cascade of events that include degranulation of mast cells, local vasodilation, plasma extravasation, and the formation of a sensitizing pro-inflammatory biochemical milieu (26). The Neurogenic Hypothesis further describes increased efferent activity in both intermediate and ventral horns subsequent to sensitization, mediating enhanced sympathetic and motor efferent activity, respectively. These coexisting pathways create the characteristic proinflammatory biochemical milieu, enhanced sympathetic outflow and motor unit excitability (26).
The Neurogenic Hypothesis provides a novel neurobiological framework that explains many of the clinical observations of non-noxious, deep, achy and diffuse pain associated with nsLBP (41). Importantly, it provides the biological plausibility for the commonly observed comorbidity of MPS and nsLBP with pathologies of both visceral and/or somatic origin, in the absence of local injury within the muscle. These mechanisms have been previously reported in a series of studies conducted by Srbely et al. which demonstrate segmentally arranged responses to pressure sensitivity (31) and sympathetic activity (33) in humans using an experimental sensitization model. They have also demonstrated antidromic release of substance P and neurogenic inflammation in segmentally-linked muscles after experimentally-induced osteoarthritis using animal models (32, 34). Support for this notion exists in the literature that has already linked MPS to common injuries and/or somatic pathologies including chronic muscle or ligament strain, intervertebral disc degeneration (44) and/or degenerative joints (45, 46) in addition to chronic non-musculoskeletal pathologies such as metabolic or cancerous conditions (47–49), which are also relevant for nsLBP.
Downstream effects of persistent central sensitization and neurogenic inflammation
A corollary of the Neurogenic Hypothesis suggests that dorsal horn sensitization may influence the activity of intermediate and ventral horns through the release of pro-inflammatory cytokines via activated glial cells (50, 51). This provides the biological rationale for the increased sympathetic and motor activity associated with MPS. Furthermore, persistent neurogenic inflammation within peripheral tissues may lead to pain and dysfunction via impaired fascial gliding (30) and interstitial inflammatory stasis (52) within the affected myotome (Figure 1). This cascade of events can result in a vicious cycle of pain and inflammation that can persist even after the initial pathology has been resolved, a state that is consistent with the definition of chronic pain.
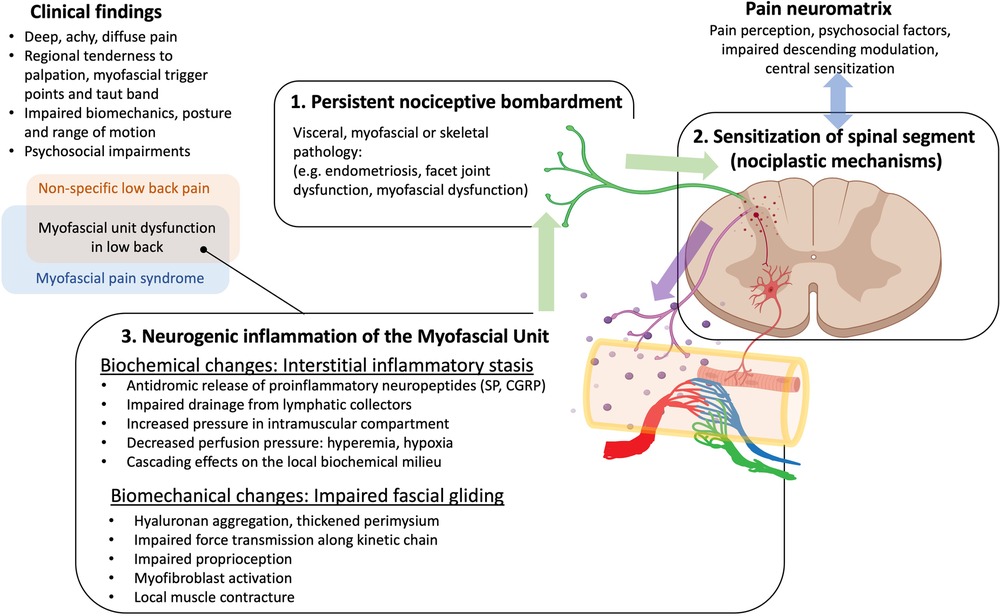
Figure 1. Overarching hypothesis and rationale for our research. Neurogenic inflammation, involving the antidromic release of sensitizing neuropeptides in response to persistent nociceptive bombardment to the dorsal horn provides a link between mechanisms of central sensitization and myofascial unit dysfunction, and can explain many of the clinical findings in myofascial pain and non-specific low back pain. We postulate that neurogenic inflammation as well as other factors can lead to hyaluronan aggregation and/or activation of myofibroblasts that thicken the perimuscular fascia and perimysium and impair fascial gliding, and nociception. Fascial thickening and accumulating cytokines can impair the lymphatic drainage leading to inflammatory stasis that elevates hydrostatic pressure and impairs blood flow, perpetuating the cycle.
Biomechanical dysfunction and the role of impaired fascial gliding
The fascia is a significant source of pain and dysfunction in MPS. Deep muscular fascia and aponeuroses are richly innervated by small-diameter nociceptive afferent fibers (19, 53, 54), predisposing them to neurogenic inflammatory mechanisms. This feature provides the anatomic rationale for how the fascia may act a potential source of ongoing nociception.
Fascia also plays a significant biomechanical role by providing a continuous anatomical bridge from tissues to organ structures that acts as a scaffold for routing nerves, blood vessels, and lymphatics throughout the body (36, 55). Fascia is integral to force transmission and proprioception. Its rich innervation with free nerve endings and proprioceptors (spindles, Golgi tendon organs) underscores its important role in proprioception (53, 54, 56). It is comprised of both dense and loose connective tissues. The dense portions are mainly formed from collagen types I and III, facilitating the transmission of force from muscle fibers to joints and assisting in the synergistic coordination of complex human movements (57). The loose connective tissue, predominantly consisting of adipose cells, glycosaminoglycans, and hyaluronan, enables the gliding between layers of dense connective tissue, essential for smooth muscle and joint operations. Densification within the fascial compartments has been identified as a significant factor in impaired fascial gliding and dysfunction (35, 58–60). This phenomenon occurs due to the aggregation of glycosaminoglycans, particularly hyaluronan, within the loose connective tissue. As a consequence of this aggregation, the viscosity of the loose connective tissue increases, leading to a reduced range of motion in the myotendinous structures (61). Trauma, overuse, disuse and inflammation (eg., neurogenic inflammation) are primary mechanisms by which the viscosity of hyaluronan can be increased, leading to altered fascial gliding and irritation of unmyelinated nerve fibers (61). This densification was normalized following treatment of spastic muscles in stroke patients with hyaluronidase, an FDA-approved enzyme that breaks down hyaluronan, resulting in increased range of motion (62, 63).
Altered functional gliding of the fascial layers may also lead to irritation of the free nerve endings innervating the fascia, further contributing to pain and discomfort (18). Novel imaging techniques like T1-rho imaging have been used recently in detecting this densification, offering insights into its implications for musculoskeletal health.
Biochemical changes and the role of interstitial inflammatory stasis in the clinical manifestation of chronic pain and fascial dysfunction
Neurogenic inflammation can lead to the accumulation of a proinflammatory milieu within the affected tissues to promote pain. Shah et al. (64, 65) were the first to document an abnormal biochemical milieu in active MTrPs using a microdialysis needle technique. The studies revealed that the concentrations of neuropeptides (SP, CGRP, BK), catecholamines (5-HT, NE), and pro-inflammatory cytokines (TNF-α, and IL-1β) were higher in muscle regions demonstrating active MTrPs than latent MTrPs or normal. In addition, pH levels were significantly lower in the active group compared to the latent and normal groups, the latter two of which did not differ.
Accumulating cytokines including TNF-a, IL-1b and IL-6 have been shown to also disable the lymphatic pump as well as stimulating the differentiation of fibroblasts to myofibroblasts to promote fascial contraction. In addition, these cytokines have also been shown to increase the rate of extracellular matrix production and remodeling (fibrotic thickening) of the perimysium over time that lead to impairment of fascial gliding with subsequent stiffness and pain (35, 66). Collectively, these mechanisms if allowed to persist may lead to impaired lymphatic drainage, perimysium thickening and impaired fascial gliding that promotes interstitial stasis of proinflammatory fluids released by neurogenic inflammation (52). This is a potential source of persistent nociception that can facilitate ongoing sensitization and promote chronic pain as exhibited with MPS and nsLBP. Imaging studies using ultrasound (67–69) and MRI (70, 71) have provided additional evidence for increased interstitial fluid near MTrPs.
Discussion
Strengths of new model
The primary strength of our proposed model lies in its ability to rationalize clinical observations that highlights important parallels in the clinical manifestation of MPS and nsLBP that suggest common underlying physiologic mechanisms between the two conditions. We propose a novel model for these conditions that draws on the emerging concept of the myofascial unit. This unit is defined as an integrated anatomical and functional structure that includes muscle fibers, fascia and its associated innervation, lymphatics, and blood vessels. The myofascial unit framework offers a more comprehensive perspective of the complex interplay between the different mechanistic pathways that have been linked to the clinical manifestation of MPS and nsLBP. In this way, we offer biological plausibility for the complex overlapping spectrum of clinical findings associated with MPS and nsLBP that have been a persistent source of confusion to clinicians, researchers, and educators and provides rationale for the well-documented comorbidity of MPS and nsLBP with other somatic and/or visceral conditions of pain hypersensitivity that are currently unexplained using existing hypotheses. Our model suggests that structures like facet joints, intervertebral discal structures, and ligaments could be the originators of a vicious cycle of soft tissue pain associated with nsLBP, that can persist even after the underlying pathology is resolved or treated.
The model postulates a vicious cycle between neurogenic inflammation and inflammatory stasis leading to dysfunctional mechanics of the myofascial unit that further exacerbates, and is exacerbated by, biomechanical and postural factors leading to further pain and inflammation. Additional psychosocial factors such as stress, anxiety, depression, sleep and mood disturbances, and pain catastrophizing further contribute to the affective component of chronic pain perception in the pain neuromatrix, and can also modulate descending inhibitory mechanisms that can impact tissue-level abnormalities (Figure 1).
The proposed model enables the integration of central and peripheral mechanisms by drawing on the mechanisms of central sensitization, a well-established pathophysiological mechanism observed in all chronic pain syndromes. It prioritizes patient individuality and proposes the use of evaluation tools and imaging methods to investigate the dynamics of fascia gliding, mechanical changes at the myofascial unit level, local vascular changes, local fluid content of tissue, motor unit excitability, and the local biochemical milieu. This approach enhances the ability to identify patient phenotypes, leading to sensitivity and specificity of diagnostic criteria and appropriate mechanism-based treatments that result in improved clinical outcomes.
Finally, our model explains why many different therapeutic modalities, including manual therapies, needling with and without analgesics, acupuncture and complementary and alternative medicine approaches, all have been documented to provide relief yet none of the modalities alone has been shown to have consistent and reproducible evidence of efficacy at Level 1. We postulate that the complex interplay between the different mechanistic pathways between patients may be the primary explanation, further supporting the need for a patient-specific multidimensional assessment protocol that is sensitive to the unique clinical phenotype of each individual subject.
Transforming current clinical guidelines into proposed multidimensional assessment in the clinic
Current evidence-based clinical practice guidelines for assessment of the patient with LBP (8, 9) emphasize the use of history taking and physical exam to identify “red flags” and rule out serious medical conditions, neurological testing for radicular syndrome, and psychosocial assessment. We advocate that this approach, while a good starting point, is inadequate alone, since it does not provide a systematic approach to assessing other more common forms (i.e., non-specific) of low back pain. Additionally, this approach, diagnosis of exclusion for nsLBP does not provide insight into causal explanations for pain in the absence of obvious tissue pathology. We propose a systematic multidimensional assessment for nsLBP, allowing for more individualized patient assessment driven by underlying mechanisms, assessment of activity limitations, and use of validated patient-reported outcome measures. There have been attempts to classify chronic LBP patients into groups based on clinical characteristics, then further subgrouping into management strategies and interventions (10, 13). However, to date, no single classification system has proven more effective than another for reducing pain and disability in patients with chronic LBP (72). Most of the more successful classification systems integrate individualization and motor control components (13, 14) but are still insufficient.
Our proposed systematic multi-dimensional assessment takes the foundational work to the next level by providing additional insight into the underlying mechanisms, considering patient individuality, and attempting to maintain objective continuity based on a neurobiological framework. To fully capture the amalgamation of neuroscience and biomechanics effecting nsLBP as a system, we are suggesting an expansion of the understanding of pain generators to encompass the idea of an integrated myofascial unit (muscle, fascia, blood vessels, nerve and lymphatics) so as to broaden the clinical evaluation of the MPS patient to include each of these structures.
Based on the mechanistic framework presented above, we propose a structured and comprehensive clinical assessment aligned with the guiding principle that CS is a foundational driving mechanism in the pathophysiology and clinical manifestations of nsLBP. While maladaptive neuroplastic changes in the nervous system, like CS, are evoked by persistent nociception arising from active primary pathologies, these maladaptive changes, once established, may persist long after the resolution of the primary pathology.
The primary goals of the comprehensive nsLBP assessment are to (1) assess for the clinical signs of CS and identify any active or resolved primary pathologies that could have contributed to the manifestation of CS; (2) understand biomechanical factors and assessment of activity limitations that can guide management to achieve patient-specific functional goals; and (3) assessment of psychosocial factors (20) that can play a role in descending inhibition, and stressors and protective factors that might indicate maladaptive changes (73).
We recognize that clinical time is limited and often a large number of tests using detailed physical assessment is not practical. Nonetheless, we support the value of learning about the various components that may be assessed to positively identify nsLBP, use it to appropriately plan treatment and possibly identify a need for additional diagnostic testing. The comprehensive assessment includes the following, which may be considered a menu of options to serve as a guide to practicing professions (Table 1):
1. Assessment of pain characteristics:
a. Systems review to identify underlying active primary pathologies (of visceral and/or somatic origin), serious medical conditions as described in current clinical practice guidelines for nsLBP, and additional pathologies that can contribute to CS, such as osteoarthritis, disc degeneration, and visceral conditions. History and physical examination starts with self-reports of pain, its location and its characteristics. Patient-reported outcomes such as the Oswestry Disability Index and the Roland Morris Questionnaire, Patient-Specific Functional Scale and SF-36 are supported in the literature (74).
b. Regional assessment that focuses on the identification of chief complaints or patient subjective experience. This involves identification of any involved organs and/or tissues and their neurosegmental connections by assessing dermatomes, myotomes, viscerotomes and sclerotomes for signs of inflammation, injury and/or pathology. While an association between nsLBP and MPS has been shown in the literature, assessment of myofascial tissues is typically not part of the routine evaluation for nsLBP. Many, but not all (75–78), clinicians and investigators agree that an essential characteristic of MPS is the presence of a MTrP, a “discrete palpable hyperirritable locus within a taut band of a skeletal muscle that elicits a referral sensation with pressure application” (78). MTrPs can be active or latent. Active MTrPs are spontaneously painful, and their palpation reproduces the symptomatic pain with a characteristic referral pattern. Latent MTrPs are not spontaneously painful and elicit pain only when palpated or disturbed (26). An extensive review (79) documented that “trigger point, muscle and pain” were evaluations used in 90% of published studies. Pain pressure threshold, range of motion, and manual muscle testing were the most frequent functional objective measures reported. Palpation of muscles in the region with particular attention to the presence/absence of hyper-irritable nodules MTrPs and the presence of taut-muscular bands were common.
c. Pain phenotyping that aims to determine where on the pain spectrum the patient falls, from acute to chronic, including episodic to persistent, and non-spontaneous to spontaneous (12). A thorough clinical history will enable the clinician to characterize the quality, intensity, duration, frequency, and radiation (if present) of pain. Furthermore, the character of the pain and response to perturbation should be assessed through physical exam. For example, chronic non-specific pain is typically described as a deep achy pain that is poorly localized and diffuse, in contrast to the well-demarcated sharp presentation of acute pain. Furthermore, in contrast to local acute pain, the muscular/myofascial pain associated with chronic nsLBP does not typically induce a nociceptive withdrawal reflex when the tissue is perturbed or challenged via digital pressure during a physical examination.
d. Quantitative Sensory Testing (QST) techniques to assess for/quantify the presence of windup, a neurophysiologic mechanism commonly associated with CS. Windup shares common mechanisms with CS and is the physiologic manifestation of enhanced temporal summation to repetitive stimuli. A recent systematic review (80) demonstrated significant increases in temporal summation in nsLBP (81, 82). Enhanced temporal summation has also been reported with other chronic musculoskeletal conditions including osteoarthritis, fibromyalgia (83), and MPS. The goal of this stage is to identify affected spinal segment(s) demonstrating enhanced temporal summation, suggesting the presence of either an active nociceptive source or a previously resolved pathology which as evoked maladaptive changes within its neuromeric field. Identifying and resolving an active primary pathology is essential to the long-term resolution of the musculoskeletal component of nsLBP. In addition, treating the spinal segmental sensitization after a resolved primary pathology is also essential for nsLBP. Other QST measures can be used to assess the integrity of the somatosensory system and phenotype pain, including heat and mechanical pain and/or detection thresholds, vibration detection thresholds and Pain Pressure Thresholds (84, 85) using algometry. Use of assessments of CS and autonomic dysfunction as potential estimation of blood flow abnormalities should be considered. This is based on the established segmental somato-sympathetic mechanisms commonly observed in regional pain syndromes.
2. Assessment of body movement:
a. Movement assessment to determine where on the movement spectrum the patient is (86) and if there is a decrease in optimization of movement, including asymmetry, decreased AROM, hypermobility with or without control, and altered timing of firing. Perform important functional tasks with high interrater reliability or individualized to patient specificity (87) as identified with a patient reported outcome such as the Patient Specific Functional Scale. Active straight leg raise, overhead squat, five time sit to stand, picking up an object from the floor, partial abdominal curl up (strength), side bridge and trunk flexor tests (endurance), Beighton and Brighton (hypermobility), single-legged hop, and lateral step-down, unilateral stance test with eyes open may identify suboptimal tissue loading (14), threat interference, altered movement patterns, loss of balance, asymmetry, altered posture or kinematics, and/or avoidance. Since human movement is highly adaptable to be task specific, patient movement may uncover contributing factors to the clinical manifestations of CS. Follow up with manual muscle testing to confirm or deny observed lack of optimization for weakness and/or neurosegmental involvement.
b. Range of motion (ROM) assessment. Limitations in range of motion, as well as asymmetry in range of motion and compensatory movements could indicate impaired fascial gliding and densification, or regional pain sensitivity, neurogenic inflammation and/or interstitial inflammatory stasis due to lack of ROM or hypermobility. Most evaluations include range of motion and strength measures of the anatomical segments involved, including range of motion, strength and assessment of triplanar motion of the low back. These are sometimes assessed while performing functional activities of reaching, walking and stooping.
3. Assessment of psychosocial factors:
a. Current clinical guidelines recommend analysis of psychosocial factors in the evaluation of nonspecific LBP. Psychosocial factors should be evaluated using clinical history and validated PROs to identify potential impact on descending modulation of pain (88). Metrics such as the history of previous episodes (89–91), psychological distress or depression (32–34), fear of pain or movement, and reinjury or low expectations of recovery (92, 93) and a passive coping style (8, 16, 94) and fear avoidance are also often assessed in the comprehensive evaluation of the MPS patient.
b. In addition to descending modulation, an imbalance of stressors and protective factors (17, 73) may lead to contributory maladaptive changes (allostatic load) that may perpetuate pain perception (95, 96). Evaluation has often included measures of function and quality of life as well as symptoms of fatigue, depression/dysphoria and anxiety. However, the sensitivity of the self-reports and the reliability of the physical examinations were not reported and are inconsistent and do not appear to be based on individualized patient needs.
Implications for future research to improve personalised medicine for back pain
Testing of the conceptual framework to validate the interaction of the three proposed mechanisms, including neurogenic inflammation, impaired fascial gliding and interstitial inflammatory stasis, associated with the clinical manifestation of MPS and nsLBP, will help to establish the mechanistic contributions to these pain syndromes.
Firstly, the proper selection of evaluation tools, assessing their feasibility and ease of use in the clinical settings is critical to developing accurate and reliable databases for the syndrome. Treatment effectiveness can only be assessed if the measurements are acknowledged to be consistent, reliable, sensitive and specific for clinical use. Table 1 proposes a starting point.
The individual elements of our hypothesis at the tissue level are testable in clinical settings using available technologies, and some of these investigations are currently ongoing in our research group. Imaging methods, such as ultrasound and shear wave elastography (37, 67–69), can be used to investigate the dynamics of fascia gliding and mechanical changes at the myofascial unit; photoacoustic imaging and microvascular Doppler can be used to image local vascular changes (97–99); bioimpedance spectroscopy can be a useful technique to investigate local fluid content of tissue (100, 101); high density surface electromyography can be utilized to investigate motor unit excitability (102); and microdialysis can be utilized to investigate the local biochemical milieu (64, 65).
The objectification of pain measures creates opportunities for exploration of multimodal treatments including pharmacological, non-pharmacological, behavioral, and even environmental adaptive approaches. A key limiting factor in the advancement of research in this field is the capacity to quantify changes in central sensitization. While there are current psychophysical approaches to assessing temporal summation, the biosignature, based on the conceptual framework laid out in this paper, should aim to further advance quantitative measures of CS and neurogenic inflammation, levels of Substance P, CGRP, motor unit activity at the endplate and quantitative sensory testing.
Ongoing research studies are developing objective methods to understand the physiology of the myofascial unit. Methods being explored include measures of fascial physical properties including ultrasound imaging and physical examination of movement along with measures of interstitial inflammatory stasis using Doppler imaging of blood flow, and bioimpedance spectroscopy. However, abnormal findings on imaging may not be meaningful for clinical management or prognosis without additional research indicating their utility. Additional research is also needed to better understand the biochemical milieu of the myofascial unit using microanalytic measures of pro-inflammatory cytokines.
The proposed model will have heuristic value if we can show that the evaluation of the different components of the myofascial unit enable us to develop (1) more sensitive and specific diagnostic criteria for nsLBP and (2) appropriate mechanism-based treatments that lead to improvement of clinical outcomes. High quality randomized studies are needed in the future to evaluate if the proposed diagnostic approach leads to improved outcomes compared to the standard of care. If future studies do not support the postulated mechanisms proposed in this model, the model would need to be revised accordingly.
Data availability statement
The original contributions presented in the study are included in the article/Supplementary Material, further inquiries can be directed to the corresponding author.
Author contributions
All authors contributed to the development of the proposed framework in this paper, and contributed to the writing and editing of the manuscript. All authors contributed to the article and approved the submitted version.
Funding
This work is funded in part by National Institutes of Health grant 1R61AT012286-01.
Conflict of interest
SD was employed by Optimal Motion Inc.
The remaining authors declare that the research was conducted in the absence of any commercial or financial relationships that could be construed as a potential conflict of interest.
Publisher's note
All claims expressed in this article are solely those of the authors and do not necessarily represent those of their affiliated organizations, or those of the publisher, the editors and the reviewers. Any product that may be evaluated in this article, or claim that may be made by its manufacturer, is not guaranteed or endorsed by the publisher.
References
1. Chen S, Chen M, Wu X, Lin S, Tao C, Cao H, et al. Global, regional and national burden of low back pain 1990–2019: a systematic analysis of the global burden of disease study 2019. J Orthop Translat. (2022) 32:49–58. doi: 10.1016/j.jot.2021.07.005
2. Wu A, March L, Zheng X, Huang J, Wang X, Zhao J, et al. Global low back pain prevalence and years lived with disability from 1990 to 2017: estimates from the global burden of disease study 2017. Ann Transl Med. (2020) 8(6):299. doi: 10.21037/atm.2020.02.175
3. Lucas J, Connor E, Bose J. Back, Lower Limb, and Upper Limb Pain Among U.S. Adults, 2019. National Center for Health Statistics (U.S.); 2021. Available at: https://stacks.cdc.gov/view/cdc/107894 (Cited March 4, 2023).
4. Gore M, Sadosky A, Stacey BR, Tai KS, Leslie D. The burden of chronic low back pain: clinical comorbidities, treatment patterns, and health care costs in usual care settings. Spine. (2012) 37(11):E668–77. doi: 10.1097/BRS.0b013e318241e5de
5. Dieleman JL, Cao J, Chapin A, Chen C, Li Z, Liu A, et al. US Health care spending by payer and health condition, 1996–2016. JAMA. (2020) 323(9):863–84. doi: 10.1001/jama.2020.0734
6. Maher C, Underwood M, Buchbinder R. Non-specific low back pain. Lancet. (2017) 389(10070):736–47. doi: 10.1016/S0140-6736(16)30970-9
7. Balagué F, Mannion AF, Pellisé F, Cedraschi C. Non-specific low back pain. Lancet. (2012) 379(9814):482–91. doi: 10.1016/S0140-6736(11)60610-7
8. Delitto A, George SZ, Van Dillen L, Whitman JM, Sowa G, Shekelle P, et al. Low back pain: clinical practice guidelines linked to the international classification of functioning, disability, and health from the orthopaedic section of the American physical therapy association. J Orthop Sports Phys Ther. (2012) 42(4):A1–57. doi: 10.2519/jospt.2012.42.4.A1
9. Oliveira CB, Maher CG, Pinto RZ, Traeger AC, Lin CWC, Chenot JF, et al. Clinical practice guidelines for the management of non-specific low back pain in primary care: an updated overview. Eur Spine J. (2018) 27(11):2791–803. doi: 10.1007/s00586-018-5673-2
10. Kamper SJ, Maher CG, Hancock MJ, Koes BW, Croft PR, Hay E. Treatment-based subgroups of low back pain: a guide to appraisal of research studies and a summary of current evidence. Best Pract Res Clin Rheumatol. (2010) 24(2):181–91. doi: 10.1016/j.berh.2009.11.003
11. Mauck MC, Lotz J, Psioda MA, Carey TS, Clauw DJ, Majumdar S, et al. The back pain consortium (BACPAC) research program: structure, research priorities, and methods. Pain Medicine. (2023) 24:pnac202. doi: 10.1093/pm/pnac202
12. Hodges PW, Danneels L. Changes in structure and function of the back muscles in low back pain: different time points, observations, and mechanisms. J Orthop Sports Phys Ther. (2019) 49(6):464–76. doi: 10.2519/jospt.2019.8827
13. Van Dieën JH, Reeves NP, Kawchuk G, Van Dillen LR, Hodges PW. Motor control changes in low back pain: divergence in presentations and mechanisms. J Orthop Sports Phys Ther. (2019) 49(6):370–9. doi: 10.2519/jospt.2019.7917
14. Van Dieën JH, Reeves NP, Kawchuk G, Van Dillen LR, Hodges PW. Analysis of motor control in patients with low back pain: a key to personalized care? J Orthop Sports Phys Ther. (2019) 49(6):380–8. doi: 10.2519/jospt.2019.7916
15. Pincus T, Burton AK, Vogel S, Field AP. A systematic review of psychological factors as predictors of chronicity/disability in prospective cohorts of low back pain. Spine. (2002) 27(5):E109. doi: 10.1097/00007632-200203010-00017
16. Ramond A, Bouton C, Richard I, Roquelaure Y, Baufreton C, Legrand E, et al. Psychosocial risk factors for chronic low back pain in primary care—a systematic review. Fam Pract. (2011) 28(1):12–21. doi: 10.1093/fampra/cmq072
17. Rocha JRDO, Uhlig S, Karloh M, Santos ARSD, Sousa TRD. Characterization of biopsychosocial factors of patients with chronic nonspecific low back pain. BrJP. (2021) 4:332–8. doi: 10.5935/2595-0118.20210062
18. Fitzcharles MA, Cohen SP, Clauw DJ, Littlejohn G, Usui C, Häuser W. Nociplastic pain: towards an understanding of prevalent pain conditions. Lancet. (2021) 397(10289):2098–110. doi: 10.1016/S0140-6736(21)00392-5
19. Stecco A, Giordani F, Fede C, Pirri C, De Caro R, Stecco C. From muscle to the myofascial unit: current evidence and future perspectives. Int J Mol Sci. (2023) 24(5):4527. doi: 10.3390/ijms24054527
20. Tagliaferri SD, Ng SK, Fitzgibbon BM, Owen PJ, Miller CT, Bowe SJ, et al. Relative contributions of the nervous system, spinal tissue and psychosocial health to non-specific low back pain: multivariate meta-analysis. Eur J Pain. (2022) 26(3):578–99. doi: 10.1002/ejp.1883
21. Skootsky SA, Jaeger B, Oye RK. Prevalence of myofascial pain in general internal medicine practice. West J Med. (1989) 151(2):157–60. PMID: 2788962.2788962
22. Gerwin RD. Classification, epidemiology, and natural history of myofascial pain syndrome. Curr Pain Headache Rep. (2001) 5(5):412–20. doi: 10.1007/s11916-001-0052-8
23. Dor A, Kalichman L. A myofascial component of pain in knee osteoarthritis. J Bodyw Mov Ther. (2017) 21(3):642–7. doi: 10.1016/j.jbmt.2017.03.025
24. Lisi AJ, Breuer P, Gallagher RM, Rodriguez E, Rossi MI, Schmader K, et al. Deconstructing chronic low back pain in the older adult–step by step evidence and expert-based recommendations for evaluation and treatment: part II: myofascial pain. Pain Med. (2015) 16(7):1282–9. doi: 10.1111/pme.12821
25. Iglesias-González JJ, Muñoz-García MT, Rodrigues-de-Souza DP, Alburquerque-Sendín F, Fernández-de-las-Peñas C. Myofascial trigger points, pain, disability, and sleep quality in patients with chronic nonspecific low back pain. Pain Med. (2013) 14(12):1964–70. doi: 10.1111/pme.12224
26. Shah JP, Thaker N, Heimur J, Aredo JV, Sikdar S, Gerber LH. Myofascial trigger points then and now: a historical and scientific perspective. PM R. (2015) 7(7):746–61. doi: 10.1016/j.pmrj.2015.01.024
27. Cooper NA, Scavo KM, Strickland KJ, Tipayamongkol N, Nicholson JD, Bewyer DC, et al. Prevalence of gluteus medius weakness in people with chronic low back pain compared to healthy controls. Eur Spine J. (2016) 25(4):1258–65. doi: 10.1007/s00586-015-4027-6
28. Kameda M, Tanimae H. Effectiveness of active soft tissue release and trigger point block for the diagnosis and treatment of low back and leg pain of predominantly gluteus medius origin: a report of 115 cases. J Phys Ther Sci. (2019) 31(2):141–8. doi: 10.1589/jpts.31.141
29. Hua N K, Van der Does E. The occurrence and inter-rater reliability of myofascial trigger points in the quadratus lumborum and gluteus medius: a prospective study in non-specific low back pain patients and controls in general practice. Pain. (1994) 58(3):317–23. doi: 10.1016/0304-3959(94)90125-2
30. Langevin HM. Fascia mobility, proprioception, and myofascial pain. Life. (2021) 11(7):668. doi: 10.3390/life11070668
31. Srbely JZ, Dickey JP, Bent LR, Lee D, Lowerison M. Capsaicin-Induced central sensitization evokes segmental increases in trigger point sensitivity in humans. J Pain. (2010) 11(7):636–43. doi: 10.1016/j.jpain.2009.10.005
32. Duarte FCK, Hurtig M, Clark A, Brown S, Simpson J, Srbely J. Experimentally induced spine osteoarthritis in rats leads to neurogenic inflammation within neurosegmentally linked myotomes. Exp Gerontol. (2021) 149:111311. doi: 10.1016/j.exger.2021.111311
33. Srbely JZ, Dickey JP, Montaholi Y. Experimentally induced central sensitization evokes segmental autonomic responses in humans. Int Phys Med Rehabil J. (2017) 1(3):1–7. doi: 10.15406/ipmrj.2017.01.00013
34. Duarte FCK, Hurtig M, Clark A, Simpson J, Srbely JZ. Association between naturally occurring spine osteoarthritis in geriatric rats and neurogenic inflammation within neurosegmentally linked skeletal muscle. Exp Gerontol. (2019) 118:31–8. doi: 10.1016/j.exger.2019.01.002
35. Stecco A, Gesi M, Stecco C, Stern R. Fascial components of the myofascial pain syndrome. Curr Pain Headache Rep. (2013) 17(8):352. doi: 10.1007/s11916-013-0352-9
36. Benjamin M. The fascia of the limbs and back—a review. J Anat. (2009) 214(1):1–18. doi: 10.1111/j.1469-7580.2008.01011.x
37. Langevin HM, Fox JR, Koptiuch C, Badger GJ, Greenan- Naumann AC, Bouffard NA, et al. Reduced thoracolumbar fascia shear strain in human chronic low back pain. BMC Musculoskelet Disord. (2011) 12(1):203. doi: 10.1186/1471-2474-12-203
38. Gerwin RD. Myofascial and visceral pain syndromes: visceral-somatic pain representations. J Musculoskelet Pain. (2002) 10(1–2):165–75. doi: 10.1300/J094v10n01_13
39. Doggweiler-Wiygul R. Urologic myofascial pain syndromes. Current Sci Inc. (2004) 8(6):445–51. doi: 10.1007/s11916-004-0065-1
40. Stratton P, Khachikyan I, Sinaii N, Ortiz R, Shah J. Association of chronic pelvic pain and endometriosis with signs of sensitization and myofascial pain. Obstet Gynecol. (2015) 125(3):719–28. doi: 10.1097/AOG.0000000000000663
41. Srbely JZ. New trends in the treatment and management of myofascial pain syndrome. Curr Pain Headache Rep. (2010) 14(5):346–52. doi: 10.1007/s11916-010-0128-4
42. Woolf CJ. Central sensitization: implications for the diagnosis and treatment of pain. PAIN. (2011) 152(3, Supplement):S2–15. doi: 10.1016/j.pain.2010.09.030
43. Corrêa JB, Costa LOP, de Oliveira NTB, Sluka KA, Liebano RE. Central sensitization and changes in conditioned pain modulation in people with chronic nonspecific low back pain: a case–control study. Exp Brain Res. (2015) 233(8):2391–9. doi: 10.1007/s00221-015-4309-6
44. Li W, Gong Y, Liu J, Guo Y, Tang H, Qin S, et al. Peripheral and central pathological mechanisms of chronic low back pain: a narrative review. JPR. (2021) 14:1483–94. doi: 10.2147/JPR.S306280
45. Mosabbir A. Mechanisms behind the development of chronic low back pain and its neurodegenerative features. Life. (2023) 13(1):84. doi: 10.3390/life13010084
46. Urits I, Burshtein A, Sharma M, Testa L, Gold PA, Orhurhu V, et al. Low back pain, a comprehensive review: pathophysiology, diagnosis, and treatment. Curr Pain Headache Rep. (2019) 23(3):23. doi: 10.1007/s11916-019-0757-1
47. Groef AD, Kampen MV, Moortgat P, Anthonissen M, den Kerckhove EV, Christiaens MR, et al. An evaluation tool for myofascial adhesions in patients after breast cancer (MAP-BC evaluation tool): concurrent, face and content validity. PLOS ONE. (2018) 13(3):e0193915. doi: 10.1371/journal.pone.0193915
48. De Groef A, Van Kampen M, Dieltjens E, De Geyter S, Vos L, De Vrieze T, et al. Identification of myofascial trigger points in breast cancer survivors with upper limb pain: interrater reliability. Pain Med. (2018) 19(8):1650–6. doi: 10.1093/pm/pnx299
49. Coyle S. Pridinol for cancer-related myofascial pain. BMJ Supportive & Palliative Care 2022; Available at: https://spcare.bmj.com/content/early/2022/09/13/spcare-2022-003821 (Cited September 22, 2023).
50. Ji RR, Berta T, Nedergaard M. Glia and pain: is chronic pain a gliopathy? PAIN. (2013) 154:S10. doi: 10.1016/j.pain.2013.06.022
51. Liu GT, Ferguson AR, Crown ED, Bopp AC, Miranda RC, Grau JW. Instrumental learning within the rat spinal cord: localization of the essential neural circuit. Behav Neurosci. (2005) 119(2):538–47. doi: 10.1037/0735-7044.119.2.538
52. Tuckey B, Srbely J, Rigney G, Vythilingam M, Shah J. Impaired lymphatic drainage and interstitial inflammatory stasis in chronic musculoskeletal and idiopathic pain syndromes: exploring a novel mechanism. Front Pain Res. (2021) 2:45. doi: 10.3389/fpain.2021.691740
53. Suarez-Rodriguez V, Fede C, Pirri C, Petrelli L, Loro-Ferrer JF, Rodriguez-Ruiz D, et al. Fascial innervation: a systematic review of the literature. Int J Mol Sci. (2022) 23(10):5674. doi: 10.3390/ijms23105674
54. Mense S. Innervation of the thoracolumbar fascia. Eur J Transl Myol. (2019) 29(3):8297. doi: 10.4081/ejtm.2019.8297
55. Stecco C, Porzionato A, Macchi V, Stecco A, Vigato E, Parenti A, et al. The expansions of the pectoral girdle muscles onto the brachial fascia: morphological aspects and spatial disposition. CTO. (2008) 188(3):320–9. doi: 10.1159/000121433
56. Hoheisel U, Rosner J, Mense S. Innervation changes induced by inflammation of the rat thoracolumbar fascia. Neuroscience. (2015) 300:351–9. doi: 10.1016/j.neuroscience.2015.05.034
57. Krause F, Wilke J, Vogt L, Banzer W. Intermuscular force transmission along myofascial chains: a systematic review. J Anat. (2016) 228(6):910–8. doi: 10.1111/joa.12464
58. Stecco C, Stern R, Porzionato A, Macchi V, Masiero S, Stecco A, et al. Hyaluronan within fascia in the etiology of myofascial pain. Surg Radiol Anat. (2011) 33(10):891–6. doi: 10.1007/s00276-011-0876-9
59. Fede C, Angelini A, Stern R, Macchi V, Porzionato A, Ruggieri P, et al. Quantification of hyaluronan in human fasciae: variations with function and anatomical site. J Anat. (2018) 233(4):552–6. doi: 10.1111/joa.12866
60. Hughes EJ, McDermott K, Funk MF. Evaluation of hyaluronan content in areas of densification compared to adjacent areas of fascia. J Bodyw Mov Ther. (2019) 23(2):324–8. doi: 10.1016/j.jbmt.2019.01.017
61. Cowman MK, Schmidt TA, Raghavan P, Stecco A. Viscoelastic properties of hyaluronan in physiological conditions. F1000Res. (2015) 4:622. doi: 10.12688/f1000research.6885.1
62. Raghavan P, Lu Y, Mirchandani M, Stecco A. Human recombinant hyaluronidase injections for upper limb muscle stiffness in individuals with cerebral injury: a case series. EBioMedicine. (2016) 9:306–13. doi: 10.1016/j.ebiom.2016.05.014
63. Menon RG, Raghavan P, Regatte RR. Quantifying muscle glycosaminoglycan levels in patients with post-stroke muscle stiffness using T1ρ MRI. Sci Rep. (2019) 9(1):14513. doi: 10.1038/s41598-019-50715-x
64. Shah JP, Phillips TM, Danoff JV, Gerber LH. An in vivo microanalytical technique for measuring the local biochemical milieu of human skeletal muscle. J Appl Physiol. (2005) 99(5):1977–84. doi: 10.1152/japplphysiol.00419.2005
65. Shah JP, Danoff JV, Desai MJ, Parikh S, Nakamura LY, Phillips TM, et al. Biochemicals associated with pain and inflammation are elevated in sites near to and remote from active myofascial trigger points. Arch Phys Med Rehabil. (2008) 89(1):16–23. doi: 10.1016/j.apmr.2007.10.018
66. Stecco A, Stern R, Fantoni I, De Caro R, Stecco C. Fascial disorders: implications for treatment. PM&R. (2016) 8(2):161–8. doi: 10.1016/j.pmrj.2015.06.006
67. Ballyns JJ, Shah JP, Hammond J, Gebreab T, Gerber LH, Sikdar S. Objective sonographic measures for characterizing myofascial trigger points associated with cervical pain. J Ultrasound Med. (2011) 30(10):1331–40. doi: 10.7863/jum.2011.30.10.1331
68. Turo D, Otto P, Hossain M, Gebreab T, Armstrong K, Rosenberger WF, et al. Novel use of ultrasound elastography to quantify muscle tissue changes after dry needling of myofascial trigger points in patients with chronic myofascial pain. J Ultrasound Med. (2015) 34(12):2149–61. doi: 10.7863/ultra.14.08033
69. Turo D, Otto P, Shah JP, Heimur J, Gebreab T, Zaazhoa M, et al. Ultrasonic characterization of the upper trapezius muscle in patients with chronic neck pain. Ultrason Imaging. (2013) 35(2):173–87. doi: 10.1177/0161734612472408
70. Menon RG, Oswald SF, Raghavan P, Regatte RR, Stecco A. T1ρ-Mapping for musculoskeletal pain diagnosis: case series of variation of water bound glycosaminoglycans quantification before and after fascial manipulation® in subjects with elbow pain. Int J Environ Res Public Health. (2020) 17(3):708. doi: 10.3390/ijerph17030708
71. Sollmann N, Mathonia N, Weidlich D, Bonfert M, Schroeder SA, Badura KA, et al. Quantitative magnetic resonance imaging of the upper trapezius muscles—assessment of myofascial trigger points in patients with migraine. J Headache Pain. (2019) 20(1):8. doi: 10.1186/s10194-019-0960-9
72. Fortin M, Rye M, Roussac A, Naghdi N, Macedo LG, Dover G, et al. The effects of combined motor control and isolated extensor strengthening versus general exercise on paraspinal muscle morphology and function in patients with chronic low back pain: a randomised controlled trial protocol. BMC Musculoskelet Disord. (2021) 22(1):472. doi: 10.1186/s12891-021-04346-x
73. Wiley JF, Bei B, Bower JE, Stanton AL. Relationship of psychosocial resources with allostatic load: a systematic review. Psychosom Med. (2017) 79(3):283–92. doi: 10.1097/PSY.0000000000000395
74. Járomi M, Szilágyi B, Velényi A, Leidecker E, Raposa BL, Hock M, et al. Assessment of health-related quality of life and patient’s knowledge in chronic non-specific low back pain. BMC Public Health. (2021) 21(Suppl 1):1479. doi: 10.1186/s12889-020-09506-7
75. Lucas N, Macaskill P, Irwig L, Moran R, Bogduk N. Reliability of physical examination for diagnosis of myofascial trigger points: a systematic review of the literature. Clin J Pain. (2009 ) 25(1):80–9. doi: 10.1097/AJP.0b013e31817e13b6
76. Mayoral del Moral O, Lacomba M T, Russell IJ, Sánchez Méndez Ó, Sánchez Sánchez B. Validity and reliability of clinical examination in the diagnosis of myofascial pain syndrome and myofascial trigger points in upper quarter muscles. Pain Medicine. (2018) 19(10):2039–50. doi: 10.1093/pm/pnx315
77. Duarte FCK, West DWD, Linde LD, Hassan S, Kumbhare DA. Re-Examining myofascial pain syndrome: toward biomarker development and mechanism-based diagnostic criteria. Curr Rheumatol Rep. (2021) 23(8):69. doi: 10.1007/s11926-021-01024-8
78. Travell JG, Simons DG. Myofascial pain and dysfunction: The trigger point manual. Baltimore, MD: Lippincott Williams & Wilkins (1983). 660.
79. Phan V, Shah J, Tandon H, Srbely J, DeStefano S, Kumbhare D, et al. Myofascial pain syndrome: a narrative review identifying inconsistencies in Nomenclature. PM&R. (2020) 12(9):916–25. doi: 10.1002/pmrj.12290
80. den Bandt HL, Paulis WD, Beckwée D, Ickmans K, Nijs J, Voogt L. Pain mechanisms in low back pain: a systematic review with meta-analysis of mechanical quantitative sensory testing outcomes in people with nonspecific low back pain. J Orthop Sports Phys Ther. (2019) 49(10):698–715. doi: 10.2519/jospt.2019.8876
81. Moriguchi D, Ishigaki S, Lin X, Kuyama K, Koishi Y, Takaoka R, et al. Clinical identification of the stimulus intensity to measure temporal summation of second pain. Sci Rep. (2022) 12(1):12915. doi: 10.1038/s41598-022-17171-6
82. Goodin BR, Bulls HW, Herbert MS, Schmidt J, King CD, Glover TL, et al. Temporal summation of pain as a prospective predictor of clinical pain severity in adults aged 45 years and above with knee osteoarthritis: ethnic differences. Psychosom Med. (2014) 76(4):302–10. doi: 10.1097/PSY.0000000000000058
83. Staud R, Weyl EE, Iii JLR, Fillingim RB. Slow temporal summation of pain for assessment of central pain sensitivity and clinical pain of fibromyalgia patients. PLOS ONE. (2014) 9(2):e89086. doi: 10.1371/journal.pone.0089086
84. Rolke R, Magerl W, Campbell KA, Schalber C, Caspari S, Birklein F, et al. Quantitative sensory testing: a comprehensive protocol for clinical trials. Eur J Pain. (2006) 10(1):77–88. doi: 10.1016/j.ejpain.2005.02.003
85. Rolke R, Baron R, Maier C, Tölle TR, Treede RD, Beyer A, et al. Quantitative sensory testing in the German research network on neuropathic pain (DFNS): standardized protocol and reference values. PAIN®. (2006) 123(3):231–43. doi: 10.1016/j.pain.2006.01.041
86. McGill S. Low back disorders: Evidence-based prevention and rehabilitation. 2nd ed Champaign, IL: Human Kinetics (2007). 312. Available at: https://www.ncbi.nlm.nih.gov/pmc/articles/PMC3280122/
87. O’Sullivan PB, Phyty GDM, Twomey LT, Allison GT. Evaluation of specific stabilizing exercise in the treatment of chronic low back pain with radiologic diagnosis of spondylolysis or spondylolisthesis. Spine. (1997) 22(24):2959–67. doi: 10.1097/00007632-199712150-00020
88. Ossipov MH, Morimura K, Porreca F. Descending pain modulation and chronification of pain. Curr Opin Support Palliat Care. (2014) 8(2):143–51. doi: 10.1097/SPC.0000000000000055
89. Stanton TR, Henschke N, Maher CG, Refshauge KM, Latimer J, McAuley JH. After an episode of acute low back pain, recurrence is unpredictable and not as common as previously thought. Spine. (2008) 33(26):2923. doi: 10.1097/BRS.0b013e31818a3167
90. Von Korff M, Saunders K. The course of back pain in primary care. Spine. (1996) 21(24):2833. doi: 10.1097/00007632-199612150-00004
91. Cherkin DC, Deyo RA, Street JH, Barlow W. Predicting poor outcomes for back pain seen in primary care using Patients' own criteria. Spine. (1996) 21(24):2900. doi: 10.1097/00007632-199612150-00023
92. George SZ, Fritz JM, Childs JD. Investigation of elevated fear-avoidance beliefs for patients with low back pain: a secondary analysis involving patients enrolled in physical therapy clinical trials. J Orthop Sports Phys Ther. (2008) 38(2):50–8. doi: 10.2519/jospt.2008.2647
93. Kuijer W, Groothoff JW, Brouwer S, Geertzen JHB, Dijkstra PU. Prediction of sickness absence in patients with chronic low back pain: a systematic review. J Occup Rehabil. (2006) 16(3):430–58. doi: 10.1007/s10926-006-9021-8
94. van der Hulst M, Vollenbroek-Hutten MMR, IJzerman MJ. A systematic review of sociodemographic, physical, and psychological predictors of multidisciplinary rehabilitation—or, back school treatment outcome in patients with chronic low back pain. Spine. (2005) 30(7):813. doi: 10.1097/01.brs.0000157414.47713.78
95. McEwen BS. Plasticity of the hippocampus: adaptation to chronic stress and allostatic load. Ann N Y Acad Sci. (2001) 933(1):265–77. doi: 10.1111/j.1749-6632.2001.tb05830.x
96. McEwen BS, Kalia M. The role of corticosteroids and stress in chronic pain conditions. Metab Clin Exp. (2010) 59:S9–15. doi: 10.1016/j.metabol.2010.07.012
97. Forbrich A, Heinmiller A, Zemp RJ. Photoacoustic imaging of lymphatic pumping. JBO. (2017) 22(10):106003. doi: doi: 10.1117/1.JBO.22.10.106003
98. Li M, Tang Y, Yao J. Photoacoustic tomography of blood oxygenation: a mini review. Photoacoustics. (2018) 10:65–73. doi: 10.1016/j.pacs.2018.05.001
99. Tang K, Liu M, Zhu Y, Zhang M, Niu C. The clinical application of ultrasonography with superb microvascular imaging-a review. J Clin Ultrasound. (2022) 50(5):721–32. doi: 10.1002/jcu.23210
100. Bartels EM, Sørensen ER, Harrison AP. Multi-frequency bioimpedance in human muscle assessment. Physiol Rep. (2015) 3(4):e12354. doi: 10.14814/phy2.12354
101. Shiose K, Tanabe Y, Ohnishi T, Takahashi H. Effect of regional muscle damage and inflammation following eccentric exercise on electrical resistance and the body composition assessment using bioimpedance spectroscopy. J Physiol Sci. (2019) 69(6):895–901. doi: 10.1007/s12576-019-00702-8
Keywords: low back pain, myofascial pain syndrome, personalized diagnostics, mechanism-based clinical assessment, biopsychosocial model, myofascial unit
Citation: Sikdar S, Srbely J, Shah J, Assefa Y, Stecco A, DeStefano S, Imamura M and Gerber LH (2023) A model for personalized diagnostics for non-specific low back pain: the role of the myofascial unit. Front. Pain Res. 4:1237802. doi: 10.3389/fpain.2023.1237802
Received: 10 June 2023; Accepted: 2 October 2023;
Published: 13 October 2023.
Edited by:
Maja R. Radojcic, The University of Manchester, United KingdomReviewed by:
Lingxiao Chen, The University of Sydney, AustraliaQingyu Sun, Shandong University, China
© 2023 Sikdar, Srbely, Shah, Assefa, Stecco, DeStefano, Imamura and Gerber. This is an open-access article distributed under the terms of the Creative Commons Attribution License (CC BY). The use, distribution or reproduction in other forums is permitted, provided the original author(s) and the copyright owner(s) are credited and that the original publication in this journal is cited, in accordance with accepted academic practice. No use, distribution or reproduction is permitted which does not comply with these terms.
*Correspondence: Siddhartha Sikdar c3Npa2RhckBnbXUuZWR1