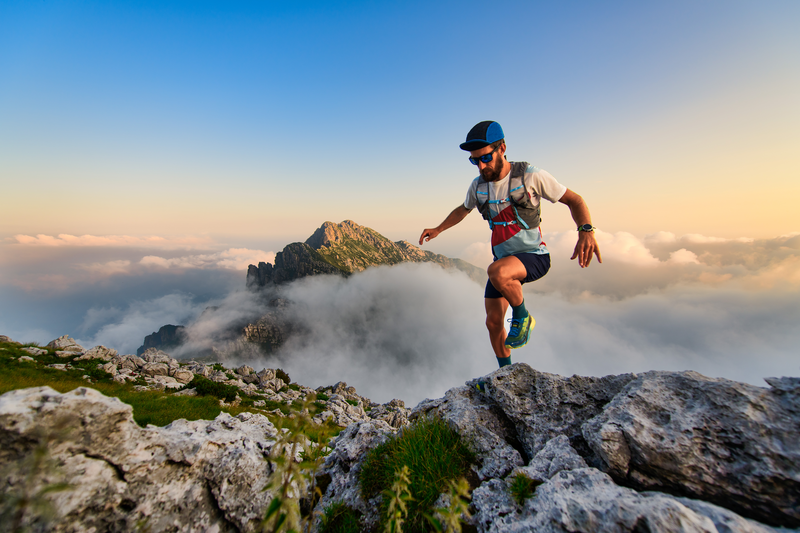
95% of researchers rate our articles as excellent or good
Learn more about the work of our research integrity team to safeguard the quality of each article we publish.
Find out more
REVIEW article
Front. Pain Res. , 06 July 2023
Sec. Pain Mechanisms
Volume 4 - 2023 | https://doi.org/10.3389/fpain.2023.1154597
An estimated 10%–50% of patients undergoing a surgical intervention will develop persistent postsurgical pain (PPP) lasting more than 3 months despite adequate acute pain management and the availability of minimally invasive procedures. The link between early and late pain outcomes for surgical procedures remains unclear—some patients improve while others develop persistent pain. The elective nature of a surgical procedure offers a unique opportunity for prophylactic or early intervention to prevent the development of PPP and improve our understanding of its associated risk factors, such as pre-operative anxiety and the duration of severe acute postoperative pain. Current perioperative pain management strategies often include opioids, but long-term consumption can lead to tolerance, addiction, opioid-induced hyperalgesia, and death. Pre-clinical models provide the opportunity to dissect mechanisms underpinning the transition from acute to chronic, or persistent, postsurgical pain. This review highlights putative mechanisms of PPP, including sensitisation of peripheral sensory neurons, neuroplasticity in the central nervous system and nociceptive signalling along the neuro-immune axis.
Acute pain after surgery typically resolves within 1–2 weeks with appropriate control therapies (1). However, for some patients, acute postsurgical pain continues beyond the normal time for tissue healing and transitions into a “chronic” or persistent pain state. Persistent postsurgical pain (PPP) is pain that is localized to the surgical field or to an innervation territory of a nerve situated in the surgical field, or to a dermatome after surgery in deep somatic or visceral tissue and persists for at least 3–6 months (2). A universally recognized definition of PPP is essential to guide future research strategies. The first definition was proposed by Macrae and Davies in 1999 (3):
1) Pain must develop after a surgical procedure
2) The pain is of at least 2 months duration
3) Other causes for pain have been excluded
4) The possibility that the pain is from a pre-existing condition has been excluded
One shortcoming of the original definition was the assumption that PPP is acute postsurgical pain that persists over a defined period. However, empirical studies show that a proportion of patients are pain-free or have mild pain in the acute post-operative period, with different sensations emerging or new pain onset weeks or months after the initial surgical insult (4, 5). The lack of correlation between early and late pain outcomes after surgery highlights the necessity to understand the biopsychosocial risk factors for developing PPP. Furthermore, excluding pre-existing pathologies or undiagnosed pain syndromes may be a confounding factor in reported studies. Schug et al. (6) described a reduction from 40% to 18% in the prevalence of PPP when only moderate or severe pain around the area of surgery was taken into consideration. But this dropped to 6% when patients who had pre-existing pain before surgery were excluded, highlighting the importance of considering pre-existing pain and its characteristics.
To enable adequate identification, diagnosis and therapy, PPP is now included in the International Classification of Diseases (ICD-11) (7). The updated definition postulates a more global measure of the impact of pain on quality of life for the individual (2, 8). It also considers pain severity (to identify chronic pain), and encompasses 3 dimensions: pain intensity, pain-related distress, and pain-related functional interference (9).
More than 300 million people worldwide undergo surgery each year. Although acute postsurgical pain is expected and can be well managed, even minor interventions are linked with the risk of developing PPP (10, 11). The development of persistent pain following surgical procedures remains a significant clinical problem that has a debilitating impact on postsurgical rehabilitation and quality of life. In the UK alone, severe PPP (defined as a score >5 on the Numeric Rating Scale (NRS) (7)) affects 2%–10% of adults undergoing surgery (12) and corresponds to at least 140,000 patients per year presenting with disabling pain. The global incidence of PPP varies depending on the type of surgery (5%–85%; see Table 1) and the median incidence of chronic pain at 6–12 months post-surgery is 20%–30%, with a small decrease over time (7, 15). The wide intra-variability in PPP incidence is predominantly due to methodological details caused by varying methods of data collection and lacking consensus in the definition of PPP (16). The incidence of PPP for different types of surgery are visible in Table 1.
Most clinical studies that aim to quantify pain outcomes following a surgical procedure focus on data composed either within a single institution or on a national level. Published studies based on single-site recruitment typically use patient data from the perioperative period, are often limited to one specific type of surgery and utilise small sample sizes (15, 17). On the other hand, nationwide studies constitute larger sample sizes, but often report retrospective data that can hinder the possibility to accurately assess temporal changes in the development of postsurgical pain and patient reported outcomes. More recently, large prospective international studies have begun to employ standardized methods for recording surgical and other perioperative characteristics (e.g., use of analgesics), as well as standardized questionnaires to gauge psychological factors (e.g., mood and catastrophizing) (18–20).
A major challenge in patient recruitment is distinguishing PPP from continuing pre-existing pain; a seminal study by Hofer et al. (21) emphasizes the need for patients with pre-existing pain to be evaluated more comprehensively, both pre- and post-surgery. Based on different patient outcomes, the findings of this study suggest that the use of cut-offs in categorizing patients into groups “PPP” or “no PPP” does not meet the needs of an individual subject, but rather that there is a transition from one group to the other. The results corroborate findings from other studies, where there are subgroups of patients that are “mixed” with milder pain symptoms, but little to no functional interference, or with essentially no pain and moderate interference with functional tasks (in addition to patients who experience pain with functional limitations). The comprehensive assessment of pain and functional interference led to lower PPP rates than previously reported. Pre-existing chronic pain, preoperative opioid medication and surgery type were also correlated with patient-reported pain outcomes 12 months post-operatively (21). In another population-based study, 18% of patients described moderate-to-severe pain at the surgical site 3–36 months after surgery (22). However, if patients who had similar pain ratings pre-surgery were excluded, only 10.5% would be classified to have PPP, while if patients with any pain before surgery were excluded, this drops to 6.2%. A change of pain characteristics or sensory disturbances, or location or spatial distribution pre-surgery can be additional diagnostic criteria, as 74.1% reported a change in the “kind of pain” compared to pre-operative pain (22). However, these postsurgical changes in pain characteristics are not addressed in the ICD-11, and questionnaires do not always consider deviations in temporal patterns and type of pain, leading to the potential misclassification of patients with PPP. Moreover, the common use of “unidimensional tools” in clinical studies, such as the sole use of the visual analogue scale (VAS), does not reflect the multi-faceted nature of the pain experience that can be better captured in combination with functional and psychometric measurements (23).
Identifying patients that may be at risk of PPP is a promising start for adequate postsurgical pain management. Current multimodal strategies to avert pain seem inadequate, mainly due to a lack of targeted approaches to personalized pain management (24–26). Improving acute pain after surgery is strongly dependent on identifying, preventing, and quantifying postsurgical pain in a timely manner; and recognizing early postsurgical pain may prevent pain from becoming chronic (27). Risk factors for PPP can be broadly identified by temporal characteristics in the pre-, peri- and post-operative periods and cover six general domains: genetic, demographic, psychosocial, pain, clinical and surgical factors. Whilst many studies have contributed to growing evidence of risk factors such as age, higher body mass index (BMI), psychological traits like depression and anxiety, and sex, besides the type of surgical intervention itself (24, 25), no single factor appears to dominate, and the question of causation remains unanswered. For example, less than 20% of the complete risk of chronic pain can be predicted by the severity of acute postsurgical pain (26). However, it is conceivable that the cumulative risk may become substantial in patients who present with multiple risk factors. Prediction models would be helpful if clinically applied for risk stratification and tailoring appropriate treatments for patients at most risk of developing PPP. This section will describe in detail some of these risk factors that have been addressed in clinical studies and are summarized in Table 2.
Pre-existing pain is an established and leading risk factor for PPP. Many lines of evidence demonstrate that the presence and intensity of preoperative chronic pain, the intensity of acute postsurgical pain, the time spent in severe pain after surgery, pain intensity in the weeks after surgery, and pain in other body parts reliably predict the extent of PPP across a variety of surgical procedures (25, 27). In a cohort of patients undergoing abdominal surgery, higher pain ratings immediately before surgery and the occurrence of chronic pain before surgery (lasting >6 months) independently predicted moderate to intense pain in the acute postsurgical period (28). Similarly, preoperative pain at or near the operative site is a useful tool to predict chronic pain after hernia and amputation surgery (29–31). Furthermore, the total number of painful sites (pre-operatively) corresponds to the probability of developing PPP (25, 27). Methodologically, it is important to measure pain status preoperatively, as retrospective recall of preoperative pain may be biased by presence and intensity of current pain.
More recently, Luedi et al. used pressure point thresholds (PPT) pre-, peri- and post-operatively on 128 subjects undergoing anorectal surgery, in addition to analysing the postsurgical analgesic medication consumption as a secondary outcome (32). This study demonstrated that patients with lower thresholds preoperatively had higher postsurgical pain scores on day 3 and at 4 weeks, but not day 1 after surgery. Thus, PPT testing may serve as an aid for identifying patients at risk of developing severe postsurgical pain. Furthermore, various studies have demonstrated the impact of chemo/radiotherapy on the chronification of pain, where either pre- or post-surgical exposure to chemotherapeutic medications have been shown to cause painful neuropathy, and in some patients, worsen PPP (33, 34). This may be due to the possible involvement of mitochondrial RNA dysregulation caused by chemotherapy-induced cellular toxicity (35–37).
Regarding age, older patients tend to have a lesser risk of developing PPP compared to younger patients (27, 38–40). This is opposed to findings in other chronic pain states where increased age can predispose one to higher rates of chronic pain (41). Female sex, which is a generalized risk factor for developing severe acute and chronic pain is also a risk factor for PPP (27, 38). Another recent report identified factors related with acute postsurgical pain trajectories and whether this can predict chronic postsurgical pain in a cohort of patients undergoing gastrointestinal surgery (42). In the acute pain trajectory, female sex and preoperative anxiety were predictive factors of chronic pain 6 months postoperatively, whereas age was a predictive factor for chronic pain 3 months after surgery. Overall, the mechanistic basis of sex differences in the prevalence of PPP are unknown but putative links have been made to genetic, psychological, socio-cultural, and pharmacokinetic differences between cis men and cis women (43).
In relation to members of the transgender community, many experience distress, depression and anxiety which may contribute to the development of persistent pain states, such as fibromyalgia (44). Whether or not transgender individuals are more prone to PPP is still unknown due to the underrepresentation of minority groups in clinical research, although one recent study demonstrated 27.4% of trans-men undergoing bilateral mastectomy reported either unilateral or bilateral persistent pain after surgery (45). Studies with larger cohorts and documented medications such as hormone therapies are required to identify susceptibility of transgender individuals to PPP.
Chronic pain is currently regarded a complex trait and has a heritability in the range of 30%–70%, therefore it is unsurprizing that PPP would have genetic risk factors. However, no single genetic cause has been identified (46, 47). Both single nucleotide polymorphisms (SNPs) and epigenetic changes can affect various functions in the inflammatory and stress responses. Pain susceptibility is known to be influenced by several genes, and the genetic basis of the individual physiological response to injury and pain management in post-operative patients is exemplified by the salient variation in a study where equivalent numbers of patients undergoing thoracotomies for lung cancer (up to 25% in a cohort of >500) report either low or high pain scores already in the first 2 post-operative days (48). Most human genetic studies focused on postsurgical pain have largely investigated polymorphisms in candidate genes. One study showed that the severity of PPP is correlated with the mRNA expression of signal transduction genes (49). The presence of PPP has also been associated with mutations in genes encoding catechol-O-methyltransferase (an enzyme in the monoaminergic pathway influencing pain inhibition) and Oprm1 encoding the μ-opioid receptor (50).
The first genome-wide association study identified single-nucleotide polymorphisms associated with the development of PPP 3 months after surgery based on two independent cohorts (51). A discovery cohort of patients undergoing hysterectomy was utilized and potential candidates were further explored in a replication cohort of orthopaedic and abdominal surgeries to verify findings. One locus (NAV3) was associated with PPP in the replication cohort, and two loci (CRTC3 and IQGAP1) were significantly associated with PPP in the meta-analysis of both cohorts (51). Moreover, a meta-analysis by Chidambaran et al. (2020) identified significant gene variants for 26 genes involved in neurotransmission, pain signalling and immune responses linked with PPP in 21 human genetic studies (47). This study establishes the role of genetic factors with different functions in PPP, and that single genetic factors have small effect sizes in explaining PPP pathophysiology. The heterogeneity in surgical cohorts, population, outcome definitions and the number of available studies evaluating these variants restricts further analysis. Thus, there is a need for large-scale, homogeneous, replication studies to validate candidate genes and recognise the fundamental biological networks for underpinning PPP. One study that is expected to finish in 2025 combines several outcome measures in a large cohort (10,000 patients) and will enable stratification for patients based on pain outcomes using genetic, demographic and clinical factor prediction models (52).
Chronic pain is now commonly considered as a “sociopsychobiomedical” issue, which supports the paradigm shift in the approach to investigating and reporting PPP (53). Psychosocial risk factors for the development of PPP entail both cognitive behavioral and emotional concepts, including preoperative anxiety and trait anxiety, as well as fears associated with impending surgery, and the concept of catastrophizing (54). Furthermore, negative cognitive-affective states including perioperative depression (55, 56), anxiety (56–59), pain catastrophizing (18, 59) and post-traumatic stress symptoms (19, 60) predict the onset of PPP. The protective role of positive psychological factors has also been addressed, such as resilience and optimism, largely interpreted as the capacity to respond effectively to risk or adversity (20, 61). Furthermore, a study by Armstrong et al. (62) indicated that anxiety, current smoking, other psychological conditions and preoperative opioid use were significantly associated with higher reported pain scores before surgery, as well as 24–48 h post-operatively.
Although pre-operative opioid consumption is warranted by the potential to effectively control acute postsurgical pain and patient function, it has also been surprisingly shown to be a risk factor for PPP (in ca. ∼25% of surgical interventions) (60, 61, 63, 64). For example, intraoperative remifentanil dosage is highly correlated with increased incidence of PPP up to 1 year after cardiac surgery (65, 66). This may be in part due to opioid-induced hyperalgesia (61, 67) as some patients who are already on long-term opioids may have developed this phenomenon, subsequently leading to poorly controlled postsurgical pain and increased opioid requirements (68, 69). Several studies address the influence of preoperative opioid consumption on PPP (66, 70), notably Keller et al. (71) demonstrated that 48% patients consuming opioids before thoracotomy had chronic pain post-surgery, in contrast to 5% of patients not consuming opioids. Furthermore, opioid administration during surgery may activate N-methyl-d-aspartate (NMDA) receptors and/or glial cells leading to sensitisation of peripheral and central nervous system centres (46, 61, 72, 73).
During the intra/post-operative periods, imminent surgical factors include the type of surgery, the surgical technique, the anatomical location, the extent of nerve injury and tissue ischemia. As an example, the degree of intercostal nerve injury is thought to be the chief element of persistent-post-thoracotomy pain (74, 75).
Several clinical reports highlight nerve damage during surgery as one of the main factors contributing to PPP. During surgery, nerves are at risk of injury from partial or complete transection, stretching, crushing, electrical damage, entrapment, or compression (35, 74, 76–78). Nerve injury is associated with many molecular and cellular changes comprizing neuroimmune interactions (79, 80). It is entirely conceivable that duration of surgery, associated with sustained peripheral injury, could trigger changes in the central nervous system (CNS). Yet there is still relatively little data to support the notion that the more severe the surgical insult, the greater the risk of persistent pain (28, 81). Surgically-induced neuropathic pain is reported to occur in 60% of patients after limb amputation (82), in 20%–40% after mastectomy (4, 83, 84), in 20% after hernia repair (85) and in 20%–40% after thoracotomy (86, 87).
The link between acute pain after surgery and PPP has been demonstrated in some surgical cohorts, including patients undergoing hernia, breast, thoracic, and laparoscopic cholecystectomy surgery (3, 12, 88). Likewise, severe pain immediately before or after lower limb amputation predicts persistent pain in the phantom/residual limb (89). Furthermore, pain trajectories in the acute postsurgical period have revealed that trajectories with constant higher pain intensity carry the most risk (90, 91). However, the specific nature of the mechanisms and whether the relationship is causal has yet to be determined (38).
In a heterogeneous sample of patients having minor, intermediate and major surgery, Peters et al. (92) reported that surgeries lasting longer than 3 h led to increased pain and poor functional outcomes at 6 months. Severe acute postsurgical pain was also indicative of increased pain at 6 months. Other factors such as the presence and intensity of acute pain have been found to predict pain weeks and months after surgery (93). Inpatients undergoing herniotomy or total knee joint replacements, greater experimental pain ratings pre-operatively is also a recognized as a risk factor for PPP (43). Other factors are increased temporal summation, as a measure of increased excitability, and decreased conditioned pain modulation (CPM), as a manifestation of decreased inhibitory function pre-operatively.
Improving postsurgical pain management will reduce the socioeconomic impact of persistent pain and associated strain on the healthcare system. Preclinical studies provide a means of dissecting mechanisms of PPP and the aetiology of incisional pain. In this section we discuss animal models of postsurgical pain, and their limitations (94).
The Brennan model of post-incisional pain was the first rodent model of surgical pain and continues to be widely used (1, 95–97). This model has been adapted in terms of the depth of incision (with/without muscle injury) and the location of incision [e.g., hindpaw (98, 99), thigh (100), back (101) as well as others (102–106)]. Additional surgical pain models have since been established to mimic specific surgical procedures such as the post-thoracotomy (107) and laparotomy model (106), which can enable a better understanding of procedure-specific pain after surgery (108). Moreover, the use of prolonged tissue retraction (a method used during surgery to expose the surgery site) is captured in some models including the skin and muscle incision retraction (SMIR) model, the inguinal hernia repair model and post-thoracotomy model. These models are characterized by chronic pain behavior and may recapitulate the transition from acute to chronic pain. The variations are shown in Figure 1, and are categorized as acute, transitional, or chronic in Table 3.
Figure 1. Summary of preclinical models of postsurgical pain. A series of incisional and postsurgical pain models were developed, stemming from the classical plantar incision model. Illustration modified from Pogatzski-Zahn et al. (97) created using Biorender.com. Details of the models are collated from several studies (98–103, 106, 107, 109). SMIR, skin and muscle incision retraction.
To understand the neurophysiological mechanisms involved in incision-induced postsurgical pain, the plantar incision model was developed by Brennan et al. (98). Under general anesthesia in rats, a 1 cm longitudinal incision is made through the skin, fascia, and plantar muscle, to extend towards the middle of the rat hindpaw. The skin is sutured, and the animals recover from anesthesia, after which pain behavior is measured from 1 h afterward. The Brennan model is an adequate recapitulation of tissue trauma experienced by patients undergoing surgery, due to the combination of trans-section of the skin, fascia and muscle retraction (1). Comparable to patients after surgery, temporary non-evoked guarding pain behaviors and longer-lasting pain behavior to punctate mechanical stimuli in rodent models are seen as proxy for non-evoked resting pain and evoked pain, respectively; and are hence pertinent to postsurgical pain in patients (130, 131). The plantar incision model was initially developed in the rat, but in 2003 this was extended to the mouse with a few modifications (i.e., 5 mm incision) and displayed similar results in behavioral pain studies (132). Mechanical hyperalgesia can be measured on the ipsilateral paw surrounding the incision (primary hyperalgesia) and in an area surrounding the injury (secondary hyperalgesia), for up to 6 days after incision (133). Additionally, heat hypersensitivity (but not cold) is robust at the site of incision and lasts for approximately 6–7 days (133). Since postsurgical pain is associated with anxiety and depression in patients, more recently studies have been underway to investigate anxiety- and depression-like behaviors after plantar incision. Rats have demonstrated an increase in both anxiety- and depression-like behaviors, and some reports show that anxiety-like behaviors lasted longer than hyperalgesia (134–136). An incision model in humans is also viable and may bridge the gap between animal studies and patients (137–139).
The SMIR model was developed to mimic prolonged pain after a surgical incision that is comparable to patients (109). In this model, an incision (1.5–2 cm long) is made in the skin of the medial inner thigh and the superficial (gracilis) muscle layer is incised (7–10 mm) and is retracted with a microdissecting retractor for an hour, before suturing. Mechanical hyperalgesia lasts much longer than after plantar incision, for up to 3 weeks; however, heat hyperalgesia is not observed in this model. The SMIR model has been reproduced in a porcine model, which presents with numerous advantages (140). Notably, pigs are phylogenetically closer in proximity to humans in the sense so share similar metabolic pathways and comparable skin anatomy, including similar homology in wound healing compared to rodents (141). These features could be implemented for validating novel topical treatment options for postsurgical pain in patients. Many other pain models linked to surgical incision have been developed and are summarized in Figure 1. More recently, modified versions of the hyperalgesic priming paradigm (142) that use the Brennan hindpaw incision as a “priming” stimulus, followed by a second noxious insult to induce chronic pain [e.g., i.pl. PGE2 (143)], have been used to study the transition from acute to chronic postoperative pain. The use of perioperative opioids in rodents also precipitates persistent hypersensitivity after hindpaw incision (122), providing an ideal model for perioperative opioid-induced hypersensitivity observed in the clinic.
Specificity and clinically relevant behavioral outcome measures is a pertinent factor in defining the translational value of animal models of pain. Reflex-based behavior is one of the primary assessment methods to measure pain in rodents; nociceptive responses to pinprick and punctate stimuli are assessed in rodents frequently and similar stimuli are used to measure hyperalgesia near the surgical wound in patients (144). This provides some basis for parallels in mechanisms that are relevant across species (8, 145). Non-evoked guarding behaviors in rodents after incision may mirror non-evoked resting pain after surgery in humans (1). However, 44% of all studies published between 2014 and 2017 still assessed only one pain modality after incision, mainly including reflex responses (97). The use of multifaceted pain assays, such as gait analysis (146), locomotor function (147), and anxiety/depression-like behaviors (134) would be of great relevance to study the mechanisms for the transition from acute to chronic pain after surgery.
Another approach to aid translation of preclinical findings is to promote the use of large animal models, such as veterinary patients receiving surgery. Postsurgical pain models in the pig may be a useful alternative approach to induce experimental pain (140) as the skin of pigs is similar to human skin—a relatively thick epidermis, comparable wound healing processes, dermal blood vessel size, skin permeability, innervation of unmyelinated fibres and similar collagen make-up (141, 148, 149). The drawbacks to large animal models include difficulty in standardizing pain-related behavioral measures and cost. Beyond the nociceptive response, other physiological parameters such as inflammation, are monitored postoperatively in patients and could be back translated in preclinical models. Changes in inflammatory mediators in the wound vicinity have only recently been reported in the Brennan model, i.e., increased COX-2 activity and leukotriene B4 levels after incision (150). Furthermore, protein C, which is a natural anticoagulant, displayed antinociceptive activity (attenuation of guarding score) when injected into the wound and is a possible mediator of pain at rest after surgery in patients (150). Unbiased proteomic approaches to studying postsurgical pain are also helping to bridge the translational gap by identifying protein-protein interactions at the incision site that differ between human and mouse (151).
To date, findings from preclinical studies suggest that sensitisation of peripheral sensory neurons, neuroplasticity in the CNS, and neuro-immune interactions, are all potential mechanisms involved in the development and maintenance of PPP. Our understanding of these mechanisms predominantly stems from preclinical studies that focus on acute, subacute, and chronic incisional pain models in rodents (summarized in Table 3).
Evidence for peripheral sensitisation in postsurgical pain derives from the use of the Brennan model of incisional pain in rodents, where typically, evoked pain behavior resolves by postoperative day (POD) 7 (98, 152). In vivo and in vitro electrophysiological data indicates that on POD 1, unmyelinated or poorly myelinated Aδ and C fibres display increased spontaneous activity and lower activation thresholds to heat and mechanical stimuli (153, 154). These findings correlate with both the non-evoked pain behaviors and mechanical and thermal hyperalgesia observed in behaving rodents. A number of pain mediators are thought to contribute to afferent sensitisation in the microenvironment of the incision site, which we describe in detail below.
Under ischemic conditions, tissue pH decreases, and lactate levels increase. The same is observed for several days after incision, with low tissue pH strongly correlated to pain behavior in rats that have received either an incision to the glabrous skin of the hindpaw, or the skin overlying the gastrocnemius muscle (155, 156). Surgically induced tissue ischemia was confirmed in further studies looking at tissue oxygen tension, which was significantly lower at the site of incision, and pimonidazole hydrochloride that was enhanced and is a marker for hypoxia (105). It is suggested that acid-sensing ion channel 3 (ASIC3) expressed by sensory neurons may be a sensor for hypoxia as it can detect lactic acid. Activation of ASIC channels on nociceptors generates ASIC currents, increasing the probability of action potential initiation, and neuron activation. Reducing ASIC3 activity with the antagonist APETx2 reduces thermal and spontaneous pain behaviors after incision in rats (112). Upregulation of ASIC3 in human chronic pain conditions has been described in biopsied samples from patients with Chron's disease, and is believed to contribute to visceral hypersensitivity in these patients (157). Whether or not it is upregulated at human incision sites remains to be determined.
Neurotrophic factors such as nerve growth factor (NGF) and artemin are upregulated in skin and muscle tissue in as little as 1 h after plantar incision in rats, returning to baseline at POD 7, but are not upregulated in the DRG (117, 158). It is believed that fibroblasts and Schwann cells adjacent to the injury are responsible for NGF release (117, 159). Sequestering NGF with the use of systemically applied monoclonal antibody either before or after incision led to a reduction in heat hyperalgesia and non-evoked pain behavior, with no effect on mechanical sensitivity, suggesting different mechanisms of activity (121, 160). Indeed, when NGF interacts with its cognate receptor TrkA on nociceptors, the upregulation of the thermoreceptor TRPV1 is facilitated at the peripheral terminals of sensory neurons, causing heat hyperalgesia (161). NGF is therefore considered an important contributing factor to acute pain after incision, but evidence for its role in human postsurgical pain is limited.
Several lines of evidence suggest that the cytokine IL-1 has an important role in the initiation and maintenance of incision-induced mechanical pain. Pro-inflammatory cytokine IL-1 is upregulated in the hindpaw tissue of rats after incision until pain behaviors resolve (117), IL-1 receptor knockout (KO) mice fail to develop mechanical hypersensitivity after injury, and the use of an IL-1 receptor antagonist either before or after incision ameliorated mechanical hypersensitivity (116). Indeed, the type 1 IL-1 receptor is highly expressed in a subpopulation of TRPV1+ nociceptors, conditional deletion of which also prevents the development of mechanical allodynia in a mouse model of RA (162). IL-6 is also upregulated in the skin and muscle of incised rats (117). It has previously been demonstrated that IL-6 causes protein synthesis in primary sensory neurons, which facilitates increased nociceptive activity (163) likely by upregulating expression of pain-related ion channels in nociceptors, such as TRPV1 (164). Furthermore, intraplantar (i.pl.) injection of IL-6, or plantar incision induces persistent nociceptive sensitisation to a subsequent i.pl. injection of PGE2, after the resolution of the initial hypersensitivity in a hyperalgesic priming paradigm (165). Mice were treated with local injections of the naturally occurring polyphenol, resveratrol, at the time of, and shortly after either injection of IL-6, or incision. Resveratrol increases AMP-activated protein kinase (AMPK), which inhibits ATP consuming processes such as protein translation largely due to inhibition of mammalian target of rapamycin (mTOR) signalling, and inhibition of mitogen activated protein kinase (MAPK) signalling (165). The study found that resveratrol dose-dependently attenuated acute pain caused by i.pl. IL-6 injection and incision and also prevented the transition to a chronic pain state when the animals were injected with PGE2, potentially through prevention of IL-6-dependent protein synthesis in nociceptors (165). Targeting the IL-6-signalling pathway in rheumatoid arthritis (RA) patients with the use of the monoclonal antibody, tocilizumab, is analgesic (166). However, caution should be employed if targeting this cytokine for postsurgical pain, as IL-6 has an important role in wound healing, and RA patients undergoing tocilizumab treatment experience slower wound healing after surgery (167).
Recent work has described the upregulation of the chemokines CCL17 and CCL22 by skin-resident dendritic cells and Langerhans cells in response to tissue injury caused by incision (114). This same study argued that their interaction with the cognate receptor, CCR4, on sensory neurons causes both mechanical and thermal hypersensitivity through CCL22, and only mechanical hypersensitivity with CCL17. CCR4 KO mice have significantly reduced acute postsurgical pain, as do control animals treated with either a CCR4 antagonist, or siRNA, or those depleted of dendritic cells. Furthermore, the group demonstrated that small diameter neurons in whole DRG cultures were able to produce action potentials in response to CCL22 application, with firing even greater in primary DRG cultures derived from animals that had been exposed to an incision. Whether this is due to a direct interaction between CCL22 and sensory neurons, or via a cell intermediate, remains to be determined.
The chemokine CXCL1/KC and its receptors CXCR1/CXCR2 also play a role in postsurgical pain, by recruiting inflammatory neutrophils to the incised paw. Neutrophil depletion reduced mechanical hyperalgesia after incision (120). Indeed, the cxcl1 and cxcl2 gene were reported as the most rapidly expressed genes (1 h) at the site of incision in a recent unbiased cell sequencing study (168). Other studies indicate a role for neutrophils in chronic pain conditions, including fibromyalgia (169), although how they activate or sensitise nociceptors has not been resolved.
Complement C5a also contributes to incisional pain (113). Hindpaw incision causes increased expression of C5 and C5aR mRNA, and C5a protein in the skin (but not the DRG or spinal cord) for at least 72 h after incision. Local administration of the C5aR antagonist PMX53 via i.pl. injection before and after incision significantly attenuated thermal and mechanical hypersensitivity. Thus, high local concentrations of C5a produced in wounds likely contributes to postsurgical pain. Further work by the same group demonstrated that global deletion of C5aR in mice reduces thermal and mechanical hypersensitivity, and inflammatory responses (including IL-1β and NGF expression, and neutrophil infiltration at the wound site) after hindpaw incision (170).
The presence of the above mediators in the wound environment causes an afferent barrage from sensitized nociceptors to the spinal cord, initiating the process of central sensitisation.
Central sensitisation refers to the amplification of central pain pathways and is a form of long-term adaptive neuroplasticity. It is responsible not only for enhanced behavioral sensitivity (hyperalgesia), but also spreading of the sensitized area (171, 172). Evidence for postsurgical central sensitisation is observed in rodent neurophysiological studies in vivo, which have demonstrated increased activity in the dorsal horn (DH) neurons of animals on POD 1. This is observed as spontaneous activity, expansion of the receptive field, and enhanced activity in response to mechanical stimulation (173, 174). Sensitisation in the DH can be reversed by blockade of afferent input indicating that peripheral drive is essential at this stage for central sensitisation. We refer primarily to spinal sensitisation within this review, but several studies have also focussed on brain neuroplasticity in postsurgical pain (175).
In contrast to inflammatory and neuropathic pain, the initial stages of incision-induced spinal sensitisation are believed to be NMDA-independent. Early after incision, the NMDA antagonist dizocilpine (MK-801) fails to block DH sensitisation, unlike the non-NMDA ionotropic EAA receptor antagonist, NBQX [2,3-dioxo-6-nitro-7-sulfamoyl-benzo(f)quinoxaline] (176). The latter finding indicates a role for AMPA receptors in spinal sensitisation after incision, which has been confirmed by further pharmacological studies (110). Further mechanistic insight shows hindpaw incision leads to phosphorylation of the GluR1 subunit of the AMPA receptor at serine-831 by protein kinase C gamma (PKCγ) (123) This leads to increased insertion of Ca2+ permeable AMPA receptors to the neuronal plasma membrane, enhancing neuronal activity and DH excitability. Intrathecal (i.t.) pre-treatment of rats with small interfering siRNA targeting PKC reduced pain hypersensitivity 3 h after incision. Further evidence for the role of the GluR1 subunit in acute incisional pain is observed when the transmembrane protein stargazin, responsible for regulating synaptic targeting of AMPA receptors, is pharmacologically down-regulated in a rat incision model, preventing an incision-induced increase in membrane GluR1 in the ipsilateral DH, as well as decreasing postsurgical pain at 3 h (115). Therefore, spinal sensitisation after plantar incision is initially maintained by the barrage of peripheral input from nociceptors, as well as non-NMDA/AMPA receptors.
Epigenetic events include modification of histone proteins, microRNA expression, and changes in global DNA methylation, which have all been demonstrated to occur after hindpaw incision in rodents. Blockade of DNA methyltransferase (DNMT) in mice leads to a reduction in incision-induced thermal and mechanical hyperalgesia and in oedema (177). The identified methylation target was the μ-opioid receptor gene Oprm1, which has increased expression in the skin following hindpaw incision (but not the spinal cord or DRG) at POD 1 and 3, and was increased further at POD 1 when DNMT was inhibited with 5-AZA-CdR (5-Aza-2′-deoxycytidine).
Additionally, incisional pain in rodents is associated with enhanced Bdnf [brain-derived neurotrophic factor, the agonist for tyrosine kinase receptor B(trkB)] and Pdyn (prodynorphin, the precursor to the k-opioid agonist, dynorphin) expression in the spinal cord, at POD 1 and 3, respectively (178). Chromatin immunoprecipitation assays demonstrated that Bdnf and Pdyn promotors were more strongly associated with acetylated H3K9 after morphine administration and incision (rather than either alone). I.t. injection of the TrkB antagonist ANA-12, and the κ-opioid receptor (KOR) selective antagonist norbinaltorphimine (nor-BNI) both reduced hyperalgesia on POD 1 or 3; however, when used individually only nor-BNI reduced hyperalgesia on POD 3. Co-administration of histone acetyltransferase inhibitor anacardic acid daily attenuated incision enhanced hyperalgesia in morphine treated mice. Therefore, both the BDNF-TrkB and dynorphin-KOR systems may be accessible therapeutic targets for postsurgical pain (178).
“Latent sensitisation” refers to a phenomenon of long-lasting pain vulnerability after opioid exposure, stress, or tissue damage (179). Opioid-dependent analgesic systems are thought to be an adaptive response to surgery which allows for natural recovery to acute surgical pain, masking a heightened state of sensitisation (180). Pain can be “unmasked” by opioid inverse agonists/antagonists to precipitate hyperalgesia after the resolution of tissue injury-induced hypersensitivity. In one study where mice received a plantar incision and pain behavior was monitored up until POD 20 when mechanical sensitivity had returned to baseline, animals received naloxone or the KOR selective antagonist nor-BNI on POD 21 and developed a hypersensitivity comparable to POD 2—also shown to be a NMDA receptor-dependent process (126). Interestingly, peripherally restricted naloxone methiodide did not produce hyperalgesia to the same extent as naloxone, indicative of a central role for KOR. Other groups have confirmed this finding, and that tonic endogenous KOR-mediated inhibition of latent sensitisation is more pronounced in females. μ-opioid receptors (MOR) also contribute equally in both sexes to this phenomenon (125). Latent sensitisation (induced by mild heat injury) has also been described in humans, although there was heterogeneity between volunteers suggesting individual differences in the adaptive opioid response (181). It is hypothesized that the inability of a patient to engage this endogenous analgesia may be a predictor of the likelihood of a patient to go onto developing chronic postsurgical pain, indeed, those that have less efficient diffuse noxious inhibitory control (DNIC), are more likely to develop chronic post-thoracotomy pain (182). While the contribution of supraspinal structures to latent sensitization is out of the scope of this review, we direct the reader to recent studies addressing these (124, 183).
The hyperalgesic priming paradigm can be used in rodents to reproduce PPP (143, 184). For example, following the Brennan hindpaw incision and resolution of mechanical allodynia by POD 13, mice receive an i.pl. injection of PGE2 into the same paw to precipitate persistent hypersensitivity. By using hyperalgesic priming, Asiedu and colleagues describe a mechanism of central sensitisation responsible for the maintenance of incisional pain (127). Protein kinase M ζ (PKMζ), is essential for supraspinal late long-term potentiation (LTP) and PKMζ inhibition leads to the loss of previously established memories (185). Thus, the group hypothesized that PKMζ is responsible for promoting a sensitized state in the DH after the induction of PPP in rodents, by the formation of a so called “pain memory” at the level of the spinal cord. Indeed, they found that inhibiting this pathway with an i.t. injection of the PKMζ inhibitor ZIP prior to PGE2 injection ameliorated the persistent allodynia induced by PGE2 (127). Another group went on to explore this further by using a modified form of the above model, injecting carrageenan instead of PGE2, prior to incision (186). Treatment with ZIP prior to incision attenuated hypersensitivity, again indicating a spinal PKMζ-dependent mechanism.
Sustained opioid use can lead to postsurgical OIH in humans (187) and in rodent models of postsurgical pain (188). Although opioids remain the analgesic of choice for acute pain management, caution must be taken with over prescription as they can facilitate postsurgical pain in patients. One of the mechanisms is thought to involve NMDA receptors; as studies using perioperative ketamine, an NMDA receptor antagonist, have demonstrated that ketamine prevents enhanced postsurgical pain in fentanyl treated rats and can improve pain management with exogenous opioids (122). Thus, ketamine can prevent central sensitisation that is triggered by nociceptive input (surgery) and exaggerated by fentanyl.
The use of pre-clinical models of incisional pain enables a better understanding of the mechanisms that underpin the development and maintenance of PPP. This, alongside unbiased transcriptomic (168) and proteomic studies (151) is essential to identifying novel targets for its treatment, as the efficacy of current clinical interventions for PPP is limited and will be discussed in the following sections.
Effective translation of basic and clinical scientific findings into clinical practice remains the major hurdle to prevention and/or adequate treatment of PPP. Advancements in surgical technique such as laparoscopic or thoracoscopic access do not necessarily prevent the development of PPP in all patients (189, 190), and analgesic treatments are not targeted to specific aspects of postsurgical pain, such as movement-evoked pain or adequate wound healing. With risk factors such as the invasiveness of surgery and consequent tissue damage, as well as the severity of acute postsurgical pain, PPP offers a unique interventional window during the pre- intra- and immediately post-operative periods.
One putative risk factor for PPP is the extent of tissue damage during surgery, including damage to nerves. The latter can be minimized by identifying and preserving these during surgery and has been shown to reduce the development of chronic pain after inguinal hernia repair (191). There is also some evidence to suggest that minimally invasive surgery, such as laparoscopic surgery can lead to less persistent pain and numbness with this particular type of operation (192). However, a more recent meta-analysis argued against this instead suggesting that there was no difference between chronic pain in patients that underwent either laparoscopic or open surgery for an inguinal hernia repair, despite positively impacting acute postsurgical pain (193). As both reviews highlighted, one of the downsides of laparoscopic surgery is the increased operating time, as well as an increased incidence of serious complications such as visceral and vascular injury. Importantly, the duration of surgery is a risk factor for PPP (although this may be due to increased complexity of pathology, and/or intraoperative tissue damage) (92).
In the case of other procedures such as hysterectomy, studies have reported that a laparoscopic approach, rather than vaginal, reduces acute pain without impacting on the prevalence of chronic pain (194). Despite this, the manner of wound closure after hysterectomy can impact upon the development of PPP, with closure of the parietal peritoneum increasing its incidence (195).
Performing less extensive surgery may reduce the incidence of PPP for some interventions. Post-mastectomy PPP manifests as pain localized to the axilla, the medial upper arm, and/or the anterior chest wall on the affected side. In the case of axillary node dissection, this may be caused by damage to the intercostobrachial nerve. Other nerves including the medial and lateral pectoral, long thoracic, or thoracodorsal nerves may also be damaged during breast cancer surgery and can contribute to neuropathic pain. It is possible to prevent damage to the intercostobrachial nerve by performing a sentinel lymph node biopsy during a lumpectomy or mastectomy, which, depending on the outcome, may prevent unnecessary axillary dissection (196).
In the case of thoracotomy (a form of surgery with the highest incidence of PPP), there are many surgical variables linked to the development of chronic pain (76). These include the types of incision for an open procedure (rib resection vs. retraction), as well as the extent of intercostal nerve preservation. It has been demonstrated that resection rather than retraction of the ribs causes less damage to the intercostal nerve, thus reducing the incidence of PPP (197). In a recent meta-analysis, the use of video-assisted thoracoscopic surgery vs. open thoracotomy was shown to also reduce the incidence of PPP at 6 months post-surgery (198). Efforts to reduce the invasiveness of surgery also extend to orthopaedic surgery, in an attempt to reduce tissue and nerve damage, as this may lead to PPP (199).
Nevertheless, there is still insufficient evidence to recommend a definitive surgical technique to prevent the development of PPP. On the other hand, risk may be minimized by avoiding damage to local nerves, minimizing the duration of surgery, choosing a minimally invasive procedure, and avoiding extensive surgery where possible.
A major predictor of PPP is the presence of pain before surgery, and the extent and duration of acute postsurgical pain. Pain management strategies include the use of regional anesthesia before, during and/or soon after surgery. Cochrane Systematic review and meta-analysis pooling results from surgeries with the highest rate of PPP (breast surgery, thoracotomy and limb amputation) found that regional anesthesia with local anesthesia led to a reduction in the development of PPP, likely through preventing and/or minimizing peripheral sensitisation and the subsequent development of central sensitisation (200). This was demonstrated in patients receiving an epidural prior to undergoing thoracotomy, or those that received an infusion of intravenous (i.v.) local anesthetics for breast cancer surgery. Although results could not be synthesized from limb amputation due to differences in the mode of treatment or in the reporting of results, an example of successful regional anesthesia during limb amputation found that the use of optimized epidural analgesia or intravenous PCA (patient-controlled analgesia), starting 48 h preoperatively and continuing for 48 h postoperatively, significantly decreased phantom limb pain after 6 months in limb amputees, compared to those receiving conventional analgesia (201).
The development of PPP may also be prevented with the use of preventative pharmacotherapies. Preclinical studies suggest that perioperative ketamine may be effective in reducing opioid consumption and acute postsurgical pain (122), but a meta-analysis has reported that the use of perioperative ketamine for analgesia in humans has so far proven inconclusive (202), although may be useful in reducing opioid consumption and pain intensity in the postoperative period in patients taking opioids prior to surgery (203). Despite this, an earlier systematic review found that by separating studies depending on the route of ketamine delivery, those that received i.v. ketamine reported a statistically significant reduction in the prevalence PPP at 3- and 6-months post-surgery (204). These contradictory findings and clinical equipoise led to a large multicentre Phase 3 randomized clinical trial with the primary aim of “reduction of chronic postsurgical pain with ketamine” (ROCKet trial, registration: ACTRN12617001619336). Ketamine will be administered as an i.v. infusion intraoperatively and continued postoperatively for a maximum of 72 h. The primary endpoint is the incidence of PPP reported by the patient at telephone follow-up, 12 months after surgery (205). Ketamine is generally well-tolerated in patients, however, there are a number of common adverse effects associated with its use including headaches, dizziness, and drowsiness limited to the duration of administration and shortly after (206).
GabapentinoidsMany incidences of PPP include an element of neuropathic pain that can be measured using standardized questionnaires (e.g., DN4) and bedside sensory testing for allodynia (87). Anticonvulsants such as pregabalin and gabapentin, which are indicated as first or second line analgesics for pain relief from neuropathy, have also been trialled for the prevention of PPP. These drugs bind to the alpha-2-delta subunit of pre-synaptic voltage-gated calcium channels and inhibit calcium influx. This in turn attenuates glutamate release therefore reducing pain transmission and central sensitisation (207). The introduction of gabapentinoid use perioperatively was initially met with enthusiasm, with an early study describing a single preoperative dose of gabapentin having an analgesic effect 4 h post-surgery. Studies such as these, on the backdrop of the opioid crisis, have led to the wide use of gabapentinoids in an attempt to decrease opioid consumption for pain relief in the postoperative period (208). However, recommendations for the use of gabapentinoids in the management of postsurgical pain are inconsistent, and increasingly emphasise the potential for adverse events, such as risk of abuse, dizziness, and respiratory depression (209). Consequently, the former American Pain Society and European Society of Regional Anesthesia pain therapy guidelines offer conflicting recommendations for the use of gabapentinoids in the perioperative period. A meta-analysis including 281 trials has reported no meaningful analgesic effect of gabapentinoids in comparison to placebo on acute or chronic postsurgical pain. Furthermore, while preventing vomiting and nausea, adverse events such a dizziness and visual disturbance were increased postoperatively (209). Further well-controlled trials are recommended for prolonged perioperative use following specific surgical incisions [sternotomy for cardiac surgery (202)].
Systemic administration of the sodium channel blocker lidocaine has been tested for its capacity to prevent of PPP (202). Clinical trials so far have been cautiously optimistic for the use of i.v. lidocaine during surgical interventions as a preventative intervention and further evidence is needed to validate the efficacy of this approach, including adverse effects (202).
Non-steroidal anti-inflammatory drugs (NSAIDs) block cyclo-oxygenase (COX) enzymes and inhibit the production of prostaglandins, resulting in an anti-inflammatory effect and analgesia (210). Positive effects in the prevention of PPP have been reported in a recent meta-analysis (202). A statistically significant treatment effect was observed in patients 12 months after surgery, when drugs were administered for 24 h or less, but not in those that had received treatment for more than 24 h, at either 3, 6, or 12 months post-surgery, indicating the duration of treatment as an important factor. NSAIDs are considered safe with minimal adverse effects, although caution should be used when prescribing to patients with underlying chronic kidney disease, a cardiac history, or gastrointestinal complications (211).
Opioids remain the gold standard for pain relief for moderate to severe perioperative pain. Achieving adequate pain control with opioids is an established approach for preventing persistent post-thoracotomy pain (212) and phantom limb pain (201). However, their use should be approached with caution not only due to serious side effects including sedation, respiratory depression, dependence and tolerance, but also due to the risk of developing OIH, as may be the case with remifentanil use at high doses intraoperatively (212, 213).
The use of multimodal anesthesia during the perioperative period has also been demonstrated to be effective in the prevention of PPP, i.e., a combination of agents that have different mechanisms of analgesia that may have a synergistic effect by modulating pain signals at various points of the pain pathway, rather than using a single drug. This can include combinations of gabapentinoids, NSAIDs, acetaminophen as well as regional anesthesia (214). There are few studies evaluating the efficacy of multimodal analgesia on PPP, however an example of its effectiveness can be seen in a breast cancer surgery trial, whereby pain and analgesic consumption was significantly reduced at 3 months in patients that received gabapentin, a mixture of local anesthetic cream, and ropivacaine wound infiltration (215). Pre-clinical models demonstrate analgesic synergy between clinically approved analgesics, as well as novel pharmacotherapies including a combination of selective Nav1.7 inhibitors, with baclofen or opioids (124, 216). A detailed summary of randomized controlled trials using pharmacological interventions to prevent the development of PPP can be found in the following review (202).
Many of the interventions used to treat pain in the acute postoperative period continue to be used for the management of PPP. In the first instance, it is important to identify the aetiology and type of pain if PPP is suspected, and to exclude recurrent malignancy in the case of cancer surgery, or to exclude other postoperative complications such as infection. Strong opioid use is to be avoided at this stage to avoid risk of dependency, which highlights the need for novel analgesics.
Current pharmacotherapies for the management of PPP include those used in neuropathic pain such as the anticonvulsants (gabapentinoids), tricyclic antidepressants (amitriptyline and nortriptyline), serotonin-norepinephrine reuptake inhibitors (duloxetine and venlafaxine), and topical lidocaine or capsaicin as a first line of defence, the studies of which have recently been summarized (217). However, a 2017 meta-analysis of the available randomized trials for PPP pharmacological treatment revealed that most pharmacological interventions tested were in isolation and did not include multimodal or multidisciplinary treatment programmes (218). Furthermore, there was no sufficient data to conclude on effectiveness or safety.
For patients whose pain levels are not responsive to pharmacotherapy, interventions such as nerve blocks, nerve ablation and neuromodulation can also be used. In the case of sternotomy-induced neuralgia, successful treatment was achieved using repeated bupivacaine blocks, phenol blocks or alcohol blocks (219). Furthermore, epidural injections have proven to provide pain relief for lumbar or cervical post-surgery syndrome (220).
Nerve entrapment or damage of nerves in the abdominal wall is often the cause of chronic abdominal wall pain post-surgery. One case report describes neural blockade of the thoracolumbar nerves supplying the anterior abdominal wall by temporary local infusion of anesthetic agents producing long-term pain relief, thereby suggesting a peripheral source for the abdominal pain (221). Furthermore, axillary brachial plexus block in a patient suffering from complex regional pain syndrome type I (CRPS I) of the upper limb after surgical release of carpal tunnel syndrome on the right hand, successfully alleviated symptoms, when combined with patient-controlled analgesia (PCA) (222). Other neural blocks include the use of botulinum toxin injections, which when injected into painful areas of chronic post-thoracotomy pain can provide significant pain relief (223), as well as the abdominal wall after laparoscopic ventral hernia repair (224). Additionally, injection or infusion of lidocaine into the DRG transiently relieved stump or phantom limb pain (PLP) (225).
More recently, novel ultrasound guided blocks have proven effective in the management of PPP after breast and abdominal surgery. In pilot studies following breast surgery, either intercostobrachial or pectoral nerve block with bupivacaine, may provide pain relief (226, 227). For patients suffering with surgery-induced chronic abdominal wall pain, ultrasound-guided transverse abdominis plan (TAP) block with local anesthetics provided significant pain relief (228, 229)
Where phantom limb sensation is useful for prosthesis, stump pain or PLP can be a debilitating disorder and afflicts almost 60% of amputee patients 1 year post-surgery (82). Amputation directly affects the peripheral nervous system (PNS), which in turn causes alterations to the CNS due to changes in sensory and movement signalling. Whereas pharmacotherapy may potentially be useful in treating PLP, such as gabapentinoids to target the neuropathic component of the disease (230), nerve ablation techniques such as phenol injections or radiofrequency ablation of stump neuromas or DRG, have been found effective. Radiofrequency ablation of the DRG or dorsal root entry zone (DREZ) shows potential for the treatment of chronic ilioinguinal pain following inguinal hernia repair as well for chronic post-thoracotomy pain (231).
Other methods of PPP management include surgical resection in the case of neuroma formation, or physical therapies such as massage, physiotherapy and acupuncture. Psychological interventions, for example cognitive behavioral therapy, have also been found useful in managing chronic pain. Due to the complexity of PPP, an individualized patient-centred approach is essential in its prevention and treatment. High quality randomized trials including multimodal interventions are required to validate and build upon positive outcomes described in the trials above.
Chronic pain after surgery is a significant clinical problem that affects approximately 10%–50% of patients worldwide. Over the last decade improvement in study design has enabled better stratification of patients according to their risk of developing PPP, but the efficacy of current treatments for acute and chronic postsurgical pain remains contentious and clinical trials often focus on individual, as opposed to multimodal, treatment strategies. Contradictory reports on therapeutic efficacy of these approaches emphasise the need for large-scale homogeneous replication studies over different study sites in order to minimise variability and validate findings. Where current treatments may prove unsuccessful, studies in pre-clinical models of acute and persistent pain due to a surgical intervention continue to provide novel therapeutic targets for clinical benefit.
All Authors researched and wrote the manuscript. All reviewed and approved the submitted version.
This work was supported by the Physiological Society, Barts Charity (MGU0515), FOREUM (1016807), and Versus Arthritis UK (21734).
The authors would like to thank Dr Sibtain Anwar for his comments on this review.
The authors declare that the research was conducted in the absence of any commercial or financial relationships that could be construed as a potential conflict of interest.
All claims expressed in this article are solely those of the authors and do not necessarily represent those of their affiliated organizations, or those of the publisher, the editors and the reviewers. Any product that may be evaluated in this article, or claim that may be made by its manufacturer, is not guaranteed or endorsed by the publisher.
1. Brennan TJ. Pathophysiology of postoperative pain. Pain. (2011) 152(3 Suppl):S33–S40. doi: 10.1016/j.pain.2010.11.005
2. Werner MU, Kongsgaard UEI. Defining persistent post-surgical pain: is an update required? Br J Anaesth. (2014) 113(1):1–4. doi: 10.1093/bja/aeu012
4. Smith WC, Bourne D, Squair J, Phillips DO, Chambers WA. A retrospective cohort study of post mastectomy pain syndrome. Pain. (1999) 83(1):91–5. doi: 10.1016/S0304-3959(99)00076-7
5. Poobalan AS, Bruce J, Smith WCS, King PM, Krukowski ZH, Chambers WA. A review of chronic pain after inguinal herniorrhaphy. Clin J Pain. (2003) 19(1):48–54. doi: 10.1097/00002508-200301000-00006
6. Schug SA. Persistent post-surgical pain: a view from the other side of the fence. Pain. (2012) 153(7):1344–5. doi: 10.1016/j.pain.2012.02.041
7. Schug SA, Lavand’homme P, Barke A, Korwisi B, Rief W, Treede R-D, et al. The IASP classification of chronic pain for ICD-11: chronic postsurgical or posttraumatic pain. Pain. (2019) 160(1):45. doi: 10.1097/j.pain.0000000000001413
8. Lavand’homme P. Transition from acute to chronic pain after surgery. Pain. (2017) 158:S50. doi: 10.1097/j.pain.0000000000000809
9. Korwisi B, Hay G, Attal N, Aziz Q, Bennett MI, Benoliel R, et al. Classification algorithm for the international classification of diseases-11 chronic pain classification: development and results from a preliminary pilot evaluation. Pain. (2021) 162(7):2087–96. doi: 10.1097/j.pain.0000000000002208
10. Weiser TG, Haynes AB, Molina G, Lipsitz SR, Esquivel MM, Uribe-Leitz T, et al. Estimate of the global volume of surgery in 2012: an assessment supporting improved health outcomes. Lancet. (2015) 385(Suppl 2):S11. doi: 10.1016/S0140-6736(15)60806-6
11. Devine M, Taylor S, Renton T. Chronic post-surgical pain following the placement of dental implants in the maxilla: a case series. Eur J Oral Implantol. (2016) 9(Suppl 1(2)):179–86. http://ejoi.quintessenz.de/index.php?doc=abstract&abstractID=36392/27314126
12. Kehlet H, Jensen TS, Woolf CJ. Persistent postsurgical pain: risk factors and prevention. Lancet. (2006) 367(9522):1618–25. doi: 10.1016/S0140-6736(06)68700-X
13. Glare P, Aubrey KR, Myles PS. Transition from acute to chronic pain after surgery. Lancet. (2019) 393(10180):1537–46. doi: 10.1016/S0140-6736(19)30352-6
14. Steyaert A, Lavand’homme P. Prevention and treatment of chronic postsurgical pain: a narrative review. Drugs. (2018) 78(3):339–54. doi: 10.1007/s40265-018-0866-x
15. Fletcher D, Stamer UM, Pogatzki-Zahn E, Zaslansky R, Tanase NV, Perruchoud C, et al. Chronic postsurgical pain in Europe: an observational study. Eur J Anaesthesiol. (2015) 32(10):725–34. doi: 10.1097/EJA.0000000000000319
16. Schug SA, Bruce J. Risk stratification for the development of chronic postsurgical pain. Pain Rep. (2017) 2(6):e627. doi: 10.1097/PR9.0000000000000627
17. Guimarães-Pereira L, Farinha F, Azevedo L, Abelha F, Castro-Lopes J. Persistent postoperative pain after cardiac surgery: incidence, characterization, associated factors and its impact in quality of life. Eur J Pain. (2016) 20(9):1433–42. doi: 10.1002/ejp.866
18. Burns LC, Ritvo SE, Ferguson MK, Clarke H, Seltzer Z, Katz J. Pain catastrophizing as a risk factor for chronic pain after total knee arthroplasty: a systematic review. J Pain Res. (2015) 8:21–32. doi: 10.2147/JPR.S64730
19. Kleiman V, Clarke H, Katz J. Sensitivity to pain traumatization: a higher-order factor underlying pain-related anxiety, pain catastrophizing and anxiety sensitivity among patients scheduled for major surgery. Pain Res Manag. (2011) 16(3):169–77. doi: 10.1155/2011/932590
20. Bruce J, Thornton AJ, Powell R, Johnston M, Wells M, Heys SD, et al. Psychological, surgical, and sociodemographic predictors of pain outcomes after breast cancer surgery: a population-based cohort study. Pain. (2014) 155(2):232–43. doi: 10.1016/j.pain.2013.09.028
21. Hofer DM, Lehmann T, Zaslansky R, Harnik M, Meissner W, Stüber F, et al. Rethinking the definition of chronic postsurgical pain: composites of patient-reported pain-related outcomes vs pain intensities alone. Pain. (2022) 163(12):2457. doi: 10.1097/j.pain.0000000000002653
22. Johansen A, Romundstad L, Nielsen CS, Schirmer H, Stubhaug A. Persistent postsurgical pain in a general population: prevalence and predictors in the Tromsø study. Pain. (2012) 153(7):1390–6. doi: 10.1016/j.pain.2012.02.018
23. Baamer RM, Iqbal A, Lobo DN, Knaggs RD, Levy NA, Toh LS. Utility of unidimensional and functional pain assessment tools in adult postoperative patients: a systematic review. Br J Anaesth. (2022) 128(5):874–88. doi: 10.1016/j.bja.2021.11.032
24. Belfer I, Greco CM, Lokshin A, Vulakovich K, Landsittel D, Dai F, et al. The design and methods of genetic studies on acute and chronic postoperative pain in patients after total knee replacement. Pain Med. (2014) 15(9):1590–602. doi: 10.1111/pme.12487
25. Wylde V, Hewlett S, Learmonth ID, Dieppe P. Persistent pain after joint replacement: prevalence, sensory qualities, and postoperative determinants. Pain. (2011) 152(3):566–72. doi: 10.1016/j.pain.2010.11.023
26. Eisenach JC. Preventing chronic pain after surgery: who, how, and when? Reg Anesth Pain Med. (2006) 31(1):1–3. doi: 10.1016/j.rapm.2005.11.008
27. Gerbershagen HJ, Ozgür E, Dagtekin O, Straub K, Hahn M, Heidenreich A, et al. Preoperative pain as a risk factor for chronic post-surgical pain—six month follow-up after radical prostatectomy. Eur J Pain. (2009) 13(10):1054–61. doi: 10.1016/j.ejpain.2008.11.020
28. Caumo W, Schmidt AP, Schneider CN, Bergmann J, Iwamoto CW, Adamatti LC, et al. Preoperative predictors of moderate to intense acute postoperative pain in patients undergoing abdominal surgery. Acta Anaesthesiol Scand. (2002) 46(10):1265–71. doi: 10.1034/j.1399-6576.2002.461015.x
29. Liem MS, van Duyn EB, van der Graaf Y, van Vroonhoven TJ. Recurrences after conventional anterior and laparoscopic inguinal hernia repair: a randomized comparison. Ann Surg. (2003) 237(1):136–41. doi: 10.1097/00000658-200301000-00019
30. Wright D, Paterson C, Scott N, Hair A, O’Dwyer PJ. Five-year follow-up of patients undergoing laparoscopic or open groin hernia repair: a randomized controlled trial. Ann Surg. (2002) 235(3):333–7. doi: 10.1097/00000658-200203000-00004
31. Nikolajsen L, Ilkjær S, Krøner K, Christensen JH, Jensen TS. The influence of preamputation pain on postamputation stump and phantom pain. Pain. (1997) 72(3):393–405. doi: 10.1016/S0304-3959(97)00061-4
32. Luedi MM, Schober P, Hammoud B, Andereggen L, Hoenemann C, Doll D. Preoperative pressure pain threshold is associated with postoperative pain in short-stay anorectal surgery: a prospective observational study. Anesth Analg. (2021) 132(3):656–62. doi: 10.1213/ANE.0000000000005072
33. Seretny M, Currie GL, Sena ES, Ramnarine S, Grant R, MacLeod MR, et al. Incidence, prevalence, and predictors of chemotherapy-induced peripheral neuropathy: a systematic review and meta-analysis. Pain. (2014) 155(12):2461–70. doi: 10.1016/j.pain.2014.09.020
34. Ishiyama H, Niino K, Hosoya T, Hayakawa K. Results of a questionnaire survey for symptom of late complications caused by radiotherapy in breast conserving therapy. Breast Cancer. (2006) 13(2):197–201. doi: 10.2325/jbcs.13.197
35. Andersen KG, Kehlet H. Persistent pain after breast cancer treatment: a critical review of risk factors and strategies for prevention. J Pain. (2011) 12(7):725–46. doi: 10.1016/j.jpain.2010.12.005
36. Podratz JL, Knight AM, Ta LE, Staff NP, Gass JM, Genelin K, et al. Cisplatin induced mitochondrial DNA damage in dorsal root ganglion neurons. Neurobiol Dis. (2011) 41(3):661–8. doi: 10.1016/j.nbd.2010.11.017
37. Dropcho EJ. Neurotoxicity of radiation therapy. Neurol Clin. (2010) 28(1):217–34. doi: 10.1016/j.ncl.2009.09.008
38. Katz J, Seltzer Z. Transition from acute to chronic postsurgical pain: risk factors and protective factors. Expert Rev Neurother. (2009) 9(5):723–44. doi: 10.1586/ern.09.20
39. Katz J, Weinrib AZ, Clarke H. Chronic postsurgical pain: from risk factor identification to multidisciplinary management at the Toronto general hospital transitional pain service. Can J Pain. (2019) 3(2):49–58. doi: 10.1080/24740527.2019.1574537
40. Ip HYV, Abrishami A, Peng PWH, Wong J, Chung F. Predictors of postoperative pain and analgesic consumption: a qualitative systematic review. Anesthesiology. (2009) 111(3):657–77. doi: 10.1097/ALN.0b013e3181aae87a
41. Domenichiello AF, Ramsden CE. The silent epidemic of chronic pain in older adults. Prog Neuropsychopharmacol Biol Psychiatry. (2019) 93:284–90. doi: 10.1016/j.pnpbp.2019.04.006
42. Liu Q-R, Dai Y-C, Ji M-H, Qiu L-L, Liu P-M, Sun X-B, et al. Predictors and predictive effects of acute pain trajectories after gastrointestinal surgery. Sci Rep. (2022) 12(1):6530. doi: 10.1038/s41598-022-10504-5
43. Gerbershagen HJ, Aduckathil S, van Wijck AJ, Peelen LM, Kalkman CJ, Meissner W. Pain intensity on the first day after surgery: a prospective cohort study comparing 179 surgical procedures. Anesthesiology. (2013) 118(4):934–44. doi: 10.1097/ALN.0b013e31828866b3
44. Levit D, Yaish I, Shtrozberg S, Aloush V, Greenman Y, Ablin JN. Pain and transition: evaluating fibromyalgia in transgender individuals. Clin Exp Rheumatol. (2021) 39(Suppl 130(3)):27–32. doi: 10.55563/clinexprheumatol/pq0qp6
45. Lang CL, Day DL, Klit A, Mejdahl MK, Holmgaard R. Low risk of persistent pain, sensory disturbances, and complications following mastectomy after gender-affirming surgery. Transgend Health. (2021) 6(4):188–93. doi: 10.1089/trgh.2020.0070
46. Ji R-R, Berta T, Nedergaard M. Glia and pain: is chronic pain a gliopathy? Pain. (2013) 154(Suppl 1(0 1)):S10–S28. doi: 10.1016/j.pain.2013.06.022
47. Chidambaran V, Gang Y, Pilipenko V, Ashton M, Ding L. Systematic review and meta-analysis of genetic risk of developing chronic postsurgical pain. J Pain. (2020) 21(1):2–24. doi: 10.1016/j.jpain.2019.05.008
48. Lim E, Harris RA, McKeon HE, Batchelor TJ, Dunning J, Shackcloth M, et al. Impact of video-assisted thoracoscopic lobectomy versus open lobectomy for lung cancer on recovery assessed using self-reported physical function: VIOLET RCT. Health Technol Assess. (2022) 26(48):1–162. doi: 10.3310/THBQ1793
49. Saxena AK, Chilkoti GT, Chopra AK, Banerjee BD, Sharma T. Chronic persistent post-surgical pain following staging laparotomy for carcinoma of ovary and its relationship to signal transduction genes. Korean J Pain. (2016) 29(4):239–48. doi: 10.3344/kjp.2016.29.4.239
50. Hoofwijk DMN, van Reij RRI, Rutten BP, Kenis G, Buhre WF, Joosten EA. Genetic polymorphisms and their association with the prevalence and severity of chronic postsurgical pain: a systematic review. Br J Anaesth. (2016) 117(6):708–19. doi: 10.1093/bja/aew378
51. van Reij RRI, Hoofwijk DMN, Rutten BPF, Weinhold L, Leber M, Joosten E, et al. The association between genome-wide polymorphisms and chronic postoperative pain: a prospective observational study. Anaesthesia. (2020) 75(S1):e111–20. doi: 10.1111/anae.14832
52. Li S, van Boekel RLM, van den Heuvel SAS, Coenen MJH, Vissers KCP. Pain predict genetics: protocol for a prospective observational study of clinical and genetic factors to predict the development of postoperative pain. BMJ Open. (2022) 12(11):e066134. doi: 10.1136/bmjopen-2022-066134
53. Carr DB, Bradshaw YS. Time to flip the pain curriculum? Anesthesiology. (2014) 120(1):12–4. doi: 10.1097/ALN.0000000000000054
54. Theunissen M, Peters ML, Bruce J, Gramke H-F, Marcus MA. Preoperative anxiety and catastrophizing: a systematic review and meta-analysis of the association with chronic postsurgical pain. Clin J Pain. (2012) 28(9):819–41. doi: 10.1097/AJP.0b013e31824549d6
55. Attal N, Masselin-Dubois A, Martinez V, Jayr C, Albi A, Fermanian J, et al. Does cognitive functioning predict chronic pain? Results from a prospective surgical cohort. Brain. (2014) 137(3):904–17. doi: 10.1093/brain/awt354
56. Brandsborg B, Nikolajsen L. Chronic pain after hysterectomy. Curr Opin Anesthesiol. (2018) 31(3):268. doi: 10.1097/ACO.0000000000000586
57. VanDenKerkhof EG, Hopman WM, Goldstein DH, Wilson RA, Towheed TE, Lam M, et al. Impact of perioperative pain intensity, pain qualities, and opioid use on chronic pain after surgery: a prospective cohort study. Reg Anesth Pain Med. (2012) 37(1):19–27. doi: 10.1097/AAP.0b013e318237516e
58. Pagé MG, Katz J, Curtis K, Lutzky-Cohen N, Escobar EMR, Clarke HA. Acute pain trajectories and the persistence of post-surgical pain: a longitudinal study after total hip arthroplasty. J Anesth. (2016) 30(4):568–77. doi: 10.1007/s00540-016-2183-4
59. Pinto PR, McIntyre T, Araújo-Soares V, Almeida A, Costa P. Psychological factors predict an unfavorable pain trajectory after hysterectomy: a prospective cohort study on chronic postsurgical pain. Pain. (2018) 159(5):956–67. doi: 10.1097/j.pain.0000000000001170
60. Rozet I, Nishio I, Robbertze R, Rotter D, Chansky H, Hernandez AV. Prolonged opioid use after knee arthroscopy in military veterans. Anesth Analg. (2014) 119(2):454–9. doi: 10.1213/ANE.0000000000000292
61. Fletcher D, Martinez V. Opioid-induced hyperalgesia in patients after surgery: a systematic review and a meta-analysis. Br J Anaesth. (2014) 112(6):991–1004. doi: 10.1093/bja/aeu137
62. Armstrong AD, Hassenbein SE, Black S, Hollenbeak CS. Risk factors for increased postoperative pain and recommended Orderset for postoperative analgesic usage. Clin J Pain. (2020) 36(11):845–51. doi: 10.1097/AJP.0000000000000876
63. Hilliard PE, Waljee J, Moser S, Metz L, Mathis M, Goesling J, et al. Prevalence of preoperative opioid use and characteristics associated with opioid use among patients presenting for surgery. JAMA Surg. (2018) 153(10):929–37. doi: 10.1001/jamasurg.2018.2102
64. de Hoogd S, Ahlers SJGM, van Dongen EPA, van de Garde EMW, Hamilton-Ter Brake TAT, Dahan A, et al. Is intraoperative remifentanil associated with acute or chronic postoperative pain after prolonged surgery? An update of the literature. Clin J Pain. (2016) 32(8):726–35. doi: 10.1097/AJP.0000000000000317
65. van Gulik L, Ahlers SJGM, van de Garde EMW, Bruins P, van Boven WJ, Tibboel D, et al. Remifentanil during cardiac surgery is associated with chronic thoracic pain 1 year after sternotomy. Br J Anaesth. (2012) 109(4):616–22. doi: 10.1093/bja/aes247
66. Waljee JF, Cron DC, Steiger RM, Zhong L, Englesbe MJ, Brummett CM. Effect of preoperative opioid exposure on healthcare utilization and expenditures following elective abdominal surgery. Ann Surg. (2017) 265(4):715–21. doi: 10.1097/SLA.0000000000002117
67. Rivat C, Ballantyne J. The dark side of opioids in pain management: basic science explains clinical observation. Pain Rep. (2016) 1(2):e570. doi: 10.1097/PR9.0000000000000570
68. Chu LF, Angst MS, Clark D. Opioid-induced hyperalgesia in humans: molecular mechanisms and clinical considerations. Clin J Pain. (2008) 24(6):479. doi: 10.1097/AJP.0b013e31816b2f43
69. Mélik Parsadaniantz S, Rivat C, Rostène W, Réaux-Le Goazigo A. Opioid and chemokine receptor crosstalk: a promising target for pain therapy? Nat Rev Neurosci. (2015) 16(2):69–78. doi: 10.1038/nrn3858
70. Ben-Ari A, Chansky H, Rozet I. Preoperative opioid use is associated with early revision after total knee arthroplasty: a study of male patients treated in the veterans affairs system. J Bone Joint Surg Am. (2017) 99(1):1–9. doi: 10.2106/JBJS.16.00167
71. Keller SM, Carp NZ, Levy MN, Rosen SM. Chronic post thoracotomy pain. J Cardiovasc Surg (Torino). (1994) 35((6 Suppl 1)):161–4. PMID: 7775532
72. Salengros J-C, Huybrechts I, Ducart A, Faraoni D, Marsala C, Barvais L, et al. Different anesthetic techniques associated with different incidences of chronic post-thoracotomy pain: low-dose remifentanil plus presurgical epidural analgesia is preferable to high-dose remifentanil with postsurgical epidural analgesia. J Cardiothorac Vasc Anesth. (2010) 24(4):608–16. doi: 10.1053/j.jvca.2009.10.006
73. Chia YY, Liu K, Wang JJ, Kuo MC, Ho ST. Intraoperative high dose fentanyl induces postoperative fentanyl tolerance. Can J Anaesth. (1999) 46(9):872–7. doi: 10.1007/BF03012978
74. Wildgaard K, Ravn J, Kehlet H. Chronic post-thoracotomy pain: a critical review of pathogenic mechanisms and strategies for prevention. Eur J Cardiothorac Surg. (2009) 36(1):170–80. doi: 10.1016/j.ejcts.2009.02.005
75. Wildgaard K, Ravn J, Nikolajsen L, Jakobsen E, Jensen TS, Kehlet H. Consequences of persistent pain after lung cancer surgery: a nationwide questionnaire study. Acta Anaesthesiol Scand. (2011) 55(1):60–8. doi: 10.1111/j.1399-6576.2010.02357.x
76. Staff NP, Engelstad J, Klein CJ, Amrami KK, Spinner RJ, Dyck PJ, et al. Post-surgical inflammatory neuropathy. Brain. (2010) 133(10):2866–80. doi: 10.1093/brain/awq252
77. Wantz GE. Testicular atrophy and chronic residual neuralgia as risks of inguinal hernioplasty. Surg Clin North Am. (1993) 73(3):571–81. doi: 10.1016/S0039-6109(16)46038-X
78. Ravichandran D, Kalambe BG, Pain JA. Pilot randomized controlled study of preservation or division of ilioinguinal nerve in open mesh repair of inguinal hernia. Br J Surg. (2000) 87(9):1166–7. doi: 10.1046/j.1365-2168.2000.01529.x
79. Austin PJ, Moalem-Taylor G. The neuro-immune balance in neuropathic pain: involvement of inflammatory immune cells, immune-like glial cells and cytokines. J Neuroimmunol. (2010) 229(1–2):26–50. doi: 10.1016/j.jneuroim.2010.08.013
80. Su P-YP, Zhang L, He L, Zhao N, Guan Z. The role of neuro-immune interactions in chronic pain: implications for clinical practice. J Pain Res. (2022) 15:2223–48. doi: 10.2147/JPR.S246883
81. Richebé P, Capdevila X, Rivat C. Persistent postsurgical pain: pathophysiology and preventative pharmacologic considerations. Anesthesiology. (2018) 129(3):590–607. doi: 10.1097/ALN.0000000000002238
82. Manchikanti L, Singh V. Managing phantom pain. Pain Physician. (2004) 7(3):365–75. doi: 10.36076/ppj.2004/7/365
83. Stevens PE, Dibble SL, Miaskowski C. Prevalence, characteristics, and impact of postmastectomy pain syndrome: an investigation of women’s experiences. Pain. (1995) 61(1):61–8. doi: 10.1016/0304-3959(94)00162-8
84. Vilholm OJ, Cold S, Rasmussen L, Sindrup SH. The postmastectomy pain syndrome: an epidemiological study on the prevalence of chronic pain after surgery for breast cancer. Br J Cancer. (2008) 99(4):604–10. doi: 10.1038/sj.bjc.6604534
85. Massaron S, Bona S, Fumagalli U, Battafarano F, Elmore U, Rosati R. Analysis of post-surgical pain after inguinal hernia repair: a prospective study of 1,440 operations. Hernia. (2007) 11(6):517–25. doi: 10.1007/s10029-007-0267-7
86. Steegers MAH, Snik DM, Verhagen AF, van der Drift MA, Wilder-Smith OHG. Only half of the chronic pain after thoracic surgery shows a neuropathic component. J Pain. (2008) 9(10):955–61. doi: 10.1016/j.jpain.2008.05.009
87. Guastella V, Mick G, Soriano C, Vallet L, Escande G, Dubray C, et al. A prospective study of neuropathic pain induced by thoracotomy: incidence, clinical description, and diagnosis. Pain. (2011) 152(1):74–81. doi: 10.1016/j.pain.2010.09.004
88. Macrae WA. Chronic post-surgical pain: 10 years on. Br J Anaesth. (2008) 101(1):77–86. doi: 10.1093/bja/aen099
89. Hanley MA, Jensen MP, Smith DG, Ehde DM, Edwards WT, Robinson LR. Preamputation pain and acute pain predict chronic pain after lower extremity amputation. J Pain. (2007) 8(2):102–9. doi: 10.1016/j.jpain.2006.06.004
90. Pagé MG, Katz J, Romero Escobar EM, Lutzky-Cohen N, Curtis K, Fuss S, et al. Distinguishing problematic from nonproblematic postsurgical pain: a pain trajectory analysis after total knee arthroplasty. Pain. (2015) 156(3):460–8. doi: 10.1097/01.j.pain.0000460327.10515.2d
91. Althaus A, Hinrichs-Rocker A, Chapman R, Arránz Becker O, Lefering R, Simanski C, et al. Development of a risk index for the prediction of chronic post-surgical pain. Eur J Pain. (2012) 16(6):901–10. doi: 10.1002/j.1532-2149.2011.00090.x
92. Peters ML, Sommer M, de Rijke JM, Kessels F, Heineman E, Patijn J, et al. Somatic and psychologic predictors of long-term unfavorable outcome after surgical intervention. Ann Surg. (2007) 245(3):487–94. doi: 10.1097/01.sla.0000245495.79781.65
93. Gan TJ. Poorly controlled postoperative pain: prevalence, consequences, and prevention. J Pain Res. (2017) 10:2287–98. doi: 10.2147/JPR.S144066
94. Burma NE, Leduc-Pessah H, Fan CY, Trang T. Animal models of chronic pain: advances and challenges for clinical translation. J Neurosci Res. (2017) 95(6):1242–56. doi: 10.1002/jnr.23768
95. Brennan TJ, Zahn PK, Pogatzki-Zahn EM. Mechanisms of incisional pain. Anesthesiol Clin North Am. (2005) 23(1):1–20. doi: 10.1016/j.atc.2004.11.009
96. Pogatzki-Zahn EM, Zahn PK, Brennan TJ. Postoperative pain–clinical implications of basic research. Best Pract Res Clin Anaesthesiol. (2007) 21(1):3–13. doi: 10.1016/j.bpa.2006.11.003
97. Pogatzki-Zahn E, Segelcke D, Zahn P. Mechanisms of acute and chronic pain after surgery: update from findings in experimental animal models. Curr Opin Anaesthesiol. (2018) 31(5):575–85. doi: 10.1097/ACO.0000000000000646
98. Brennan TJ, Vandermeulen EP, Gebhart GF. Characterization of a rat model of incisional pain. Pain. (1996) 64(3):493–502. doi: 10.1016/0304-3959(95)01441-1
99. Wang C-F, Pancaro C, Gerner P, Strichartz G. Prolonged suppression of postincisional pain by a slow-release formulation of lidocaine. Anesthesiology. (2011) 114(1):135–49. doi: 10.1097/ALN.0b013e3182001996
100. Pogatzki EM, Niemeier JS, Brennan TJ. Persistent secondary hyperalgesia after gastrocnemius incision in the rat. Eur J Pain. (2002) 6(4):295–305. doi: 10.1053/eujp.2002.0339
101. Duarte AM, Pospisilova E, Reilly E, Mujenda F, Hamaya Y, Strichartz GR. Reduction of postincisional allodynia by subcutaneous bupivacaine: findings with a new model in the hairy skin of the rat. Anesthesiology. (2005) 103(1):113–25. doi: 10.1097/00000542-200507000-00018
102. Urata K, Shinoda M, Honda K, Lee J, Maruno M, Ito R, et al. Involvement of TRPV1 and TRPA1 in incisional intraoral and extraoral pain. J Dent Res. (2015) 94(3):446–54. doi: 10.1177/0022034514565645
103. Gao YH, Li CW, Wang JY, Tan LH, Duanmu CL, Jing XH, et al. Effect of electroacupuncture on the cervicospinal P2X7 receptor/fractalkine/CX3CR1 signaling pathway in a rat neck-incision pain model. Purinergic Signal. (2017) 13(2):215–25. doi: 10.1007/s11302-016-9552-1
104. Bree D, Moriarty O, O’Mahony CM, Morris B, Bannerton K, Broom DC, et al. Development and characterization of a novel, anatomically relevant rat model of acute postoperative pain. J Pain. (2015) 16(5):421–35.e1-6. doi: 10.1016/j.jpain.2015.01.010
105. Kang S, Lee D, Theusch BE, Arpey CJ, Brennan TJ. Wound hypoxia in deep tissue after incision in rats. Wound Repair Regen. (2013) 21(5):730–9. doi: 10.1111/wrr.12081
106. Kendall LV, Wegenast DJ, Smith BJ, Dorsey KM, Kang S, Lee NY, et al. Efficacy of sustained-release buprenorphine in an experimental laparotomy model in female mice. J Am Assoc Lab Anim Sci. (2016) 55(1):66–73. PMID: 26817982; PMCID: PMC4747013
107. Buvanendran A, Kroin JS, Kerns JM, Nagalla SNK, Tuman KJ. Characterization of a new animal model for evaluation of persistent postthoracotomy pain. Anesth Analg. (2004) 99(5):1453–60. doi: 10.1213/01.ANE.0000134806.61887.0D
108. White PF, Kehlet H. Improving postoperative pain management: what are the unresolved issues? Anesthesiology. (2010) 112(1):220–5. doi: 10.1097/ALN.0b013e3181c6316e
109. Flatters SJL. Characterization of a model of persistent postoperative pain evoked by skin/muscle incision and retraction (SMIR). Pain. (2008) 135(1-2):119–30. doi: 10.1016/j.pain.2007.05.013
110. Lee H-J, Pogatzki-Zahn EM, Brennan TJ. The effect of the AMPA/kainate receptor antagonist LY293558 in a rat model of postoperative pain. J Pain. (2006) 7(10):768–77. doi: 10.1016/j.jpain.2006.03.010
111. Pogatzki EM, Niemeier JS, Sorkin LS, Brennan TJ. Spinal glutamate receptor antagonists differentiate primary and secondary mechanical hyperalgesia caused by incision. Pain. (2003) 105(1):97–107. doi: 10.1016/S0304-3959(03)00169-6
112. Deval E, Noël J, Gasull X, Delaunay A, Alloui A, Friend V, et al. Acid-sensing ion channels in postoperative pain. J Neurosci. (2011) 31(16):6059–66. doi: 10.1523/JNEUROSCI.5266-10.2011
113. Jang JH, Liang D, Kido K, Sun Y, Clark DJ, Brennan TJ. Increased local concentration of complement C5a contributes to incisional pain in mice. J Neuroinflammation. (2011) 8(1):80. doi: 10.1186/1742-2094-8-80
114. Silva JR, Iftinca M, Fernandes Gomes FI, Segal JP, Smith OMA, Bannerman CA, et al. Skin-resident dendritic cells mediate postoperative pain via CCR4 on sensory neurons. Proc Natl Acad Sci U S A. (2022) 119(4):e2118238119. doi: 10.1073/pnas.2118238119
115. Guo R, Zhao Y, Zhang M, Wang Y, Shi R, Liu Y, et al. Down-regulation of stargazin inhibits the enhanced surface delivery of α-amino-3-hydroxy-5-methyl-4-isoxazole propionate receptor GluR1 subunit in rat dorsal horn and ameliorates postoperative pain. Anesthesiology. (2014) 121(3):609–19. doi: 10.1097/ALN.0000000000000291
116. Wolf G, Livshits D, Beilin B, Yirmiya R, Shavit Y. Interleukin-1 signaling is required for induction and maintenance of postoperative incisional pain: genetic and pharmacological studies in mice. Brain Behav Immun. (2008) 22(7):1072–7. doi: 10.1016/j.bbi.2008.03.005
117. Spofford Christina M, Brennan Timothy J. Gene expression in skin, muscle, and dorsal root ganglion after plantar incision in the rat. Anesthesiology. (2012) 117(1):161–72. doi: 10.1097/ALN.0b013e31825a2a2b
118. Sahbaie P, Li X, Shi X, Clark JD. Roles of Gr-1+leukocytes in postincisional nociceptive sensitization and inflammation. Anesthesiology. (2012) 117(3):602–12. doi: 10.1097/ALN.0b013e3182655f9f
119. Mueller A, Starobova H, Morgan M, Dekan Z, Cheneval O, Schroeder CI, et al. Antiallodynic effects of the selective NaV1.7 inhibitor Pn3a in a mouse model of acute postsurgical pain: evidence for analgesic synergy with opioids and baclofen. Pain. (2019) 160(8):1766–80. doi: 10.1097/j.pain.0000000000001567
120. Carreira EU, Carregaro V, Teixeira MM, Moriconi A, Aramini A, Verri Jr WA, et al. Neutrophils recruited by CXCR1/2 signalling mediate post-incisional pain. Eur J Pain. (2013) 17(5):654–63. doi: 10.1002/j.1532-2149.2012.00240.x
121. Banik RK, Subieta AR, Wu C, Brennan TJ. Increased nerve growth factor after rat plantar incision contributes to guarding behavior and heat hyperalgesia. Pain. (2005) 117(1):68–76. doi: 10.1016/j.pain.2005.05.017
122. Richebe P, Rivat C, Laulin JP, Maurette P, Simonnet G. Ketamine improves the management of exaggerated postoperative pain observed in perioperative fentanyl-treated rats. Anesthesiology. (2005) 102(2):421–8. doi: 10.1097/00000542-200502000-00028
123. Wang Y, Wu J, Guo R, Zhao Y, Wang Y, Zhang M, et al. Surgical incision induces phosphorylation of AMPA receptor GluR1 subunits at Serine-831 sites and GluR1 trafficking in spinal cord dorsal horn via a protein kinase Cγ-dependent mechanism. Neuroscience. (2013) 240:361–70. doi: 10.1016/j.neuroscience.2013.02.051
124. Cooper AH, Hedden NS, Prasoon P, Qi Y, Taylor BK. Postsurgical latent pain sensitization is driven by descending serotonergic facilitation and masked by µ-opioid receptor constitutive activity in the rostral ventromedial medulla. J Neurosci. (2022) 42(30):5870–81. doi: 10.1523/JNEUROSCI.2038-21.2022
125. Custodio-Patsey L, Donahue RR, Fu W, Lambert J, Smith BN, Taylor BK. Sex differences in kappa opioid receptor inhibition of latent postoperative pain sensitization in dorsal horn. Neuropharmacology. (2020) 163:107726. doi: 10.1016/j.neuropharm.2019.107726
126. Campillo A, Cabanero D, Romero A, Garcia-Nogales P, Puig MM. Delayed postoperative latent pain sensitization revealed by the systemic administration of opioid antagonists in mice. Eur J Pharmacol. (2011) 657(1–3):89–96. doi: 10.1016/j.ejphar.2011.01.059
127. Asiedu MN, Tillu DV, Melemedjian OK, Shy A, Sanoja R, Bodell B, et al. Spinal protein kinase M zeta underlies the maintenance mechanism of persistent nociceptive sensitization. J Neurosci. (2011) 31(18):6646–53. doi: 10.1523/JNEUROSCI.6286-10.2011
128. Chen H, Jiang Y-s, Sun Y, Xiong Y-c. P38 and interleukin-1 beta pathway via toll-like receptor 4 contributed to the skin and muscle incision and retraction-induced allodynia. J Surg Res. (2015) 197(2):339–47. doi: 10.1016/j.jss.2015.04.061
129. Ying Y-L, Wei X-H, Xu X-B, She S-Z, Zhou L-J, Lv J, et al. Over-expression of P2X7 receptors in spinal glial cells contributes to the development of chronic postsurgical pain induced by skin/muscle incision and retraction (SMIR) in rats. Exp Neurol. (2014) 261:836–43. doi: 10.1016/j.expneurol.2014.09.007
130. Srikandarajah S, Gilron I. Systematic review of movement-evoked pain versus pain at rest in postsurgical clinical trials and meta-analyses: a fundamental distinction requiring standardized measurement. Pain. (2011) 152(8):1734–9. doi: 10.1016/j.pain.2011.02.008
131. Cooper SA, Desjardins PJ, Turk DC, Dworkin RH, Katz NP, Kehlet H, et al. Research design considerations for single-dose analgesic clinical trials in acute pain: IMMPACT recommendations. Pain. (2016) 157(2):288–301. doi: 10.1097/j.pain.0000000000000375
132. Pogatzki EM, Raja SN. A mouse model of incisional pain. Anesthesiology. (2003) 99(4):1023–7. doi: 10.1097/00000542-200310000-00041
133. Zahn PK, Brennan TJ. Primary and secondary hyperalgesia in a rat model for human postoperative pain. Anesthesiology. (1999) 90(3):863–72. doi: 10.1097/00000542-199903000-00030
134. Kouya F, Iqbal Z, Charen D, Shah M, Banik RK. Evaluation of anxiety-like behaviour in a rat model of acute postoperative pain. Eur J Anaesthesiol. (2015) 32(4):242–7. doi: 10.1097/EJA.0000000000000052
135. Dai R-P, Li C-Q, Zhang J-W, Li F, Shi X-D, Zhang J-Y, et al. Biphasic activation of extracellular signal-regulated kinase in anterior cingulate cortex distinctly regulates the development of pain-related anxiety and mechanical hypersensitivity in rats after incision. Anesthesiology. (2011) 115(3):604–13. doi: 10.1097/ALN.0b013e3182242045
136. Su C, D’Amour J, Lee M, Lin H-Y, Manders T, Xu D, et al. Persistent pain alters AMPA receptor subunit levels in the nucleus accumbens. Mol Brain. (2015) 8:46. doi: 10.1186/s13041-015-0140-z
137. Fimer I, Klein T, Magerl W, Treede R-D, Zahn PK, Pogatzki-Zahn EM. Modality-specific somatosensory changes in a human surrogate model of postoperative pain. Anesthesiology. (2011) 115(2):387–97. doi: 10.1097/ALN.0b013e318219509e
138. Kawamata M, Takahashi T, Kozuka Y, Nawa Y, Nishikawa K, Narimatsu E, et al. Experimental incision-induced pain in human skin: effects of systemic lidocaine on flare formation and hyperalgesia. Pain. (2002) 100(1–2):77–89. doi: 10.1016/S0304-3959(02)00233-6
139. Pogatzki-Zahn EM, Wagner C, Meinhardt-Renner A, Burgmer M, Beste C, Zahn PK, et al. Coding of incisional pain in the brain: a functional magnetic resonance imaging study in human volunteers. Anesthesiology. (2010) 112(2):406–17. doi: 10.1097/ALN.0b013e3181ca4c82
140. Castel D, Willentz E, Doron O, Brenner O, Meilin S. Characterization of a porcine model of post-operative pain. Eur J Pain. (2014) 18(4):496–505. doi: 10.1002/j.1532-2149.2013.00399.x
141. Sullivan TP, Eaglstein WH, Davis SC, Mertz P. The pig as a model for human wound healing. Wound Repair Regen. (2001) 9(2):66–76. doi: 10.1046/j.1524-475x.2001.00066.x
142. Aley KO, Levine JD. Role of protein kinase a in the maintenance of inflammatory pain. J Neurosci. (1999) 19(6):2181–6. doi: 10.1523/JNEUROSCI.19-06-02181.1999
143. Moy JK, Szabo-Pardi T, Tilliu DV, Megat S, Pradahn G, Kume M, et al. Temporal and sex differences in the role of BDNF/TrkB signaling in hyperalgesic priming in mice and rats. Neurobiol Pain. (2019) 5:100024. doi: 10.1016/j.ynpai.2018.10.001
144. Paredes AC, Pinto JM, Almeida A, Pinto PR. Predictive value of quantitative sensory testing for acute and chronic postsurgical pain after total joint arthroplasty: a systematic review. Pain. (2022) 163(3):e385. doi: 10.1097/j.pain.0000000000002385
145. Lavand’homme P, De Kock M, Waterloos H. Intraoperative epidural analgesia combined with ketamine provides effective preventive analgesia in patients undergoing major digestive surgery. Anesthesiology. (2005) 103(4):813–20. doi: 10.1097/00000542-200510000-00020
146. Sahbaie P, Sun Y, Liang D-Y, Shi X-Y, Clark JD. Curcumin treatment attenuates pain and enhances functional recovery after incision. Anesth Analg. (2014) 118(6):1336–44. doi: 10.1213/ANE.0000000000000189
147. Zhang L, Guo S, Zhao Q, Li Y, Song C, Wang C, et al. Spinal protein kinase Mζ regulates α-amino-3-hydroxy-5-methyl-4-isoxazolepropionic acid receptor trafficking and dendritic spine plasticity via Kalirin-7 in the pathogenesis of remifentanil-induced postincisional hyperalgesia in rats. Anesthesiology. (2018) 129(1):173–86. doi: 10.1097/ALN.0000000000002190
148. Bartek MJ, LaBudde JA, Maibach HI. Skin permeability in vivo: comparison in rat, rabbit, pig and man. J Invest Dermatol. (1972) 58(3):114–23. doi: 10.1111/1523-1747.ep12538909
149. Obreja O, Schmelz M. Single-fiber recordings of unmyelinated afferents in pig. Neurosci Lett. (2010) 470(3):175–9. doi: 10.1016/j.neulet.2009.10.006
150. Kumar R, Gupta S, Gautam M, Jhajhria SK, Ray SB. Diverse characters of Brennan’s paw incision model regarding certain parameters in the rat. Korean J Pain. (2019) 32(3):168–77. doi: 10.3344/kjp.2019.32.3.168
151. Segelcke D, van der Burgt M, Kappert C, Schmidt Garcia D, Sondermann JR, Bigalke S, et al. Phenotype- and species-specific skin proteomic signatures for incision-induced pain in humans and mice. Br J Anaesth. (2023) 130(3):331–42. doi: 10.1016/j.bja.2022.10.040
152. Cowie AM, Stucky CL. A mouse model of postoperative pain. Bio Protoc. (2019) 9(2). doi: 10.21769/BioProtoc.3140
153. Pogatzki EM, Gebhart GF, Brennan TJ. Characterization of Adelta- and C-fibers innervating the plantar rat hindpaw one day after an incision. J Neurophysiol. (2002) 87(2):721–31. doi: 10.1152/jn.00208.2001
154. Banik RK, Brennan TJ. Spontaneous discharge and increased heat sensitivity of rat C-fiber nociceptors are present in vitro after plantar incision. Pain. (2004) 112(1):204–13. doi: 10.1016/j.pain.2004.08.026
155. Kim TJ, Freml L, Park SS, Brennan TJ. Lactate concentrations in incisions indicate ischemic-like conditions may contribute to postoperative pain. J Pain. (2007) 8(1):59–66. doi: 10.1016/j.jpain.2006.06.003
156. Woo Young C, Park Soo S, Subieta Alberto R, Brennan Timothy J. Changes in tissue pH and temperature after incision indicate acidosis may contribute to postoperative pain. Anesthesiology. (2004) 101(2):468–75. doi: 10.1097/00000542-200408000-00029
157. Yiangou Y, Facer P, Smith JA, Sangameswaran L, Eglen R, Birch R, et al. Increased acid-sensing ion channel ASIC-3 in inflamed human intestine. Eur J Gastroenterol Hepatol. (2001) 13(8):891–6. doi: 10.1097/00042737-200108000-00003
158. Wu C, Boustany L, Liang H, Brennan TJ. Nerve growth factor expression after plantar incision in the rat. Anesthesiology. (2007) 107(1):128–35. doi: 10.1097/01.anes.0000267512.08619.bd
159. Wu C, Erickson Mark A, Xu J, Wild Kenneth D, Brennan Timothy J. Expression profile of nerve growth factor after muscle incision in the rat. Anesthesiology. (2009) 110(1):140–9. doi: 10.1097/ALN.0b013e318190bc84
160. Zahn PK, Subieta A, Park SS, Brennan TJ. Effect of blockade of nerve growth factor and tumor necrosis factor on pain behaviors after plantar incision. J Pain. (2004) 5(3):157–63. doi: 10.1016/j.jpain.2004.02.538
161. Ji R-R, Samad TA, Jin S-X, Schmoll R, Woolf CJ. P38 MAPK activation by NGF in primary sensory neurons after inflammation increases TRPV1 levels and maintains heat hyperalgesia. Neuron. (2002) 36(1):57–68. doi: 10.1016/S0896-6273(02)00908-X
162. Mailhot B, Christin M, Tessandier N, Sotoudeh C, Bretheau F, Turmel R, et al. Neuronal interleukin-1 receptors mediate pain in chronic inflammatory diseases. J Exp Med. (2020) 217(9):e20191430. doi: 10.1084/jem.20191430
163. Melemedjian OK, Asiedu MN, Tillu DV, Peebles KA, Yan J, Ertz N, et al. IL-6- and NGF-induced rapid control of protein synthesis and nociceptive plasticity via convergent signaling to the eIF4F complex. J Neurosci. (2010) 30(45):15113–23. doi: 10.1523/JNEUROSCI.3947-10.2010
164. Fang D, Kong L-Y, Cai J, Li S, Liu X-D, Han J-S, et al. Interleukin-6-mediated functional upregulation of TRPV1 receptors in dorsal root ganglion neurons through the activation of JAK/PI3K signaling pathway: roles in the development of bone cancer pain in a rat model. Pain. (2015) 156(6):1124–44. doi: 10.1097/j.pain.0000000000000158
165. Tillu DV, Melemedjian OK, Asiedu MN, Qu N, De Felice M, Dussor G, et al. Resveratrol engages AMPK to attenuate ERK and mTOR signaling in sensory neurons and inhibits incision-induced acute and chronic pain. Mol Pain. (2012) 8:5. doi: 10.1186/1744-8069-8-5
166. Yazici Y, Curtis JR, Ince A, Baraf H, Malamet RL, Teng LL, et al. Efficacy of tocilizumab in patients with moderate to severe active rheumatoid arthritis and a previous inadequate response to disease-modifying antirheumatic drugs: the ROSE study. Ann Rheum Dis. (2012) 71(2):198–205. doi: 10.1136/ard.2010.148700
167. Momohara S, Hashimoto J, Tsuboi H, Miyahara H, Nakagawa N, Kaneko A, et al. Analysis of perioperative clinical features and complications after orthopaedic surgery in rheumatoid arthritis patients treated with tocilizumab in a real-world setting: results from the multicentre TOcilizumab in perioperative period (TOPP) study. Mod Rheumatol. (2013) 23(3):440–9. doi: 10.3109/s10165-012-0683-0
168. Goto T, Sapio MR, Maric D, Robinson JM, Domenichiello AF, Saligan LN, et al. Longitudinal peripheral tissue RNA-Seq transcriptomic profiling, hyperalgesia, and wound healing in the rat plantar surgical incision model. Faseb J. (2021) 35(10):e21852. doi: 10.1096/fj.202100347R
169. Caxaria S, Bharde S, Fuller AM, Evans R, Thomas B, Celik P, et al. Neutrophils infiltrate sensory ganglia and mediate chronic widespread pain in fibromyalgia. Proc Natl Acad Sci U S A. (2023) 120(17):e2211631120. doi: 10.1073/pnas.2211631120
170. Liang D-Y, Li X, Shi X, Sun Y, Sahbaie P, Li W-W, et al. The complement component C5a receptor mediates pain and inflammation in a postsurgical pain model. Pain. (2012) 153(2):366–72. doi: 10.1016/j.pain.2011.10.032
171. Woolf CJ. Evidence for a central component of post-injury pain hypersensitivity. Nature. (1983) 306(5944):686–8. doi: 10.1038/306686a0
172. Ji R-R, Kohno T, Moore KA, Woolf CJ. Central sensitization and LTP: do pain and memory share similar mechanisms? Trends Neurosci. (2003) 26(12):696–705. doi: 10.1016/j.tins.2003.09.017
173. Xu J, Richebe P, Brennan TJ. Separate groups of dorsal horn neurons transmit spontaneous activity and mechanosensitivity one day after plantar incision. Eur J Pain. (2009) 13(8):820–8. doi: 10.1016/j.ejpain.2008.10.005
174. Kang S, Brennan TJ. Mechanisms of postoperative pain. APM. (2016) 11(3):236–48. doi: 10.17085/apm.2016.11.3.236
175. Pogatzki-Zahn EM, Segelcke D, Schug SA. Postoperative pain-from mechanisms to treatment. Pain Rep. (2017) 2(2):e588. doi: 10.1097/PR9.0000000000000588
176. Zahn PK, Pogatzki-Zahn EM, Brennan TJ. Spinal administration of MK-801 and NBQX demonstrates NMDA-independent dorsal horn sensitization in incisional pain. Pain. (2005) 114(3):499–510. doi: 10.1016/j.pain.2005.01.018
177. Sun Y, Sahbaie P, Liang D, Li W, Shi X, Kingery P, et al. DNA Methylation modulates nociceptive sensitization after incision. PLoS ONE. (2015) 10(11):e0142046. doi: 10.1371/journal.pone.0142046
178. Sahbaie P, Liang D-Y, Shi X-Y, Sun Y, Clark JD. Epigenetic regulation of spinal cord gene expression contributes to enhanced postoperative pain and analgesic tolerance subsequent to continuous opioid exposure. Mol Pain. (2016) 12:1744806916641950. doi: 10.1177/1744806916641950
179. Rivat C, Laboureyras E, Laulin J-P, Le Roy C, Richebé P, Simonnet G. Non-nociceptive environmental stress induces hyperalgesia, not analgesia, in pain and opioid-experienced rats. Neuropsychopharmacology. (2007) 32(10):2217–28. doi: 10.1038/sj.npp.1301340
180. Taylor BK, Corder G. Endogenous analgesia, dependence, and latent pain sensitization. In: Taylor BK, Finn DP, editors. Behavioral neurobiology of chronic pain. Berlin, Heidelberg: Springer Berlin Heidelberg (2014). p. 283–325.
181. Pereira MP, Donahue RR, Dahl JB, Werner M, Taylor BK, Werner MU. Endogenous opioid-masked latent pain sensitization: studies from mouse to human. PLoS One. (2015) 10(8):e0134441. doi: 10.1371/journal.pone.0134441
182. Yarnitsky D, Crispel Y, Eisenberg E, Granovsky Y, Ben-Nun A, Sprecher E, et al. Prediction of chronic post-operative pain: pre-operative DNIC testing identifies patients at risk. Pain. (2008) 138(1):22–8. doi: 10.1016/j.pain.2007.10.033
183. Cooper AH, Hedden NS, Corder G, Lamerand SR, Donahue RR, Morales-Medina JC, et al. Endogenous µ-opioid receptor activity in the lateral and capsular subdivisions of the right central nucleus of the amygdala prevents chronic postoperative pain. J Neurosci Res. (2022) 100(1):48–65. doi: 10.1002/jnr.24846
184. Aley KO, Messing RO, Mochly-Rosen D, Levine JD. Chronic hypersensitivity for inflammatory nociceptor sensitization mediated by the ε isozyme of protein kinase C. J Neurosci. (2000) 20(12):4680–5. doi: 10.1523/JNEUROSCI.20-12-04680.2000
185. Shema R, Sacktor TC, Dudai Y. Rapid erasure of long-term memory associations in the cortex by an inhibitor of PKM zeta. Science. (2007) 317(5840):951–3. doi: 10.1126/science.1144334
186. An K, Zhen C, Liu ZH, Zhao Q, Liu HP, Zhong XL, et al. Spinal protein kinase Mzeta contributes to the maintenance of peripheral inflammation-primed persistent nociceptive sensitization after plantar incision. Eur J Pain. (2015) 19(1):39–47. doi: 10.1002/ejp.517
187. Angst Martin S, Clark JD. Opioid-induced hyperalgesia: a qualitative systematic review. Anesthesiology. (2006) 104(3):570–87. doi: 10.1097/00000542-200603000-00025
188. Célérier E, González Juan R, Maldonado R, Cabañero D, Puig Margarita M. Opioid-induced hyperalgesia in a murine model of postoperative pain: role of nitric oxide generated from the inducible nitric oxide synthase. Anesthesiology. (2006) 104(3):546–55. doi: 10.1097/00000542-200603000-00023
189. Bayman EO, Parekh KR, Keech J, Selte A, Brennan TJ. A prospective study of chronic pain after thoracic surgery. Anesthesiology. (2017) 126(5):938–51. doi: 10.1097/ALN.0000000000001576
190. Bendixen M, Jorgensen OD, Kronborg C, Andersen C, Licht PB. Postoperative pain and quality of life after lobectomy via video-assisted thoracoscopic surgery or anterolateral thoracotomy for early stage lung cancer: a randomised controlled trial. Lancet Oncol. (2016) 17(6):836–44. doi: 10.1016/S1470-2045(16)00173-X
191. Alfieri S, Rotondi F, Di Giorgio A, Fumagalli U, Salzano A, Di Miceli D, et al. Influence of preservation versus division of ilioinguinal, iliohypogastric, and genital nerves during open mesh herniorrhaphy: prospective multicentric study of chronic pain. Ann Surg. (2006) 243(4):553–8. doi: 10.1097/01.sla.0000208435.40970.00
192. McCormack K, Scott NW, Go PM, Ross S, Grant AM. Laparoscopic techniques versus open techniques for inguinal hernia repair. Cochrane Database Syst Rev. (2003) 2003(1):Cd001785. doi: 10.1002/14651858.CD001785
193. Karthikesalingam A, Markar SR, Holt PJE, Praseedom RK. Meta-analysis of randomized controlled trials comparing laparoscopic with open mesh repair of recurrent inguinal hernia. Br J Surg. (2009) 97(1):4–11. doi: 10.1002/bjs.6902
194. Brandsborg B. Pain following hysterectomy: epidemiological and clinical aspects. Dan Med J. (2012) 59(1):B4374. PMID: 22239844
195. Recker DC, Perry PM. Postsurgical pain syndromes: chronic pain after hysterectomy and cesarean section. Tech Reg Anesth Pain Manag. (2011) 15(3):133–9. doi: 10.1053/j.trap.2011.08.002
196. Jung BF, Ahrendt GM, Oaklander AL, Dworkin RH. Neuropathic pain following breast cancer surgery: proposed classification and research update. Pain. (2003) 104(1):1–13. doi: 10.1016/S0304-3959(03)00241-0
197. Gottschalk A, Cohen SP, Yang S, Ochroch EA. Preventing and treating pain after thoracic surgery. Anesthesiology. (2006) 104(3):594–600. doi: 10.1097/00000542-200603000-00027
198. Arends S, Böhmer AB, Poels M, Schieren M, Koryllos A, Wappler F, et al. Post-thoracotomy pain syndrome: seldom severe, often neuropathic, treated unspecific, and insufficient. Pain Rep. (2020) 5(2):e810. doi: 10.1097/PR9.0000000000000810
199. Vilardo L, Shah M. Chronic pain after hip and knee replacement. Tech Reg Anesth Pain Manag. (2011) 15(3):110–5. doi: 10.1053/j.trap.2011.09.002
200. Weinstein EJ, Levene JL, Cohen MS, Andreae DA, Chao JY, Johnson M, et al. Local anaesthetics and regional anaesthesia versus conventional analgesia for preventing persistent postoperative pain in adults and children. Cochrane Database Syst Rev. (2018) 4(4):Cd007105. doi: 10.1002/14651858.CD007105.pub3
201. Karanikolas M, Aretha D, Tsolakis I, Monantera G, Kiekkas P, Papadoulas S, et al. Optimized perioperative analgesia reduces chronic phantom limb pain intensity, prevalence, and frequency: a prospective, randomized, clinical trial. Anesthesiology. (2011) 114(5):1144–54. doi: 10.1097/ALN.0b013e31820fc7d2
202. Carley ME, Chaparro LE, Choinière M, Kehlet H, Moore RA, Van Den Kerkhof E, et al. Pharmacotherapy for the prevention of chronic pain after surgery in adults: an updated systematic review and meta-analysis. Anesthesiology. (2021) 135(2):304–25. doi: 10.1097/ALN.0000000000003837
203. Loftus RW, Yeager MP, Clark JA, Brown JR, Abdu WA, Sengupta DK, et al. Intraoperative ketamine reduces perioperative opiate consumption in opiate-dependent patients with chronic back pain undergoing back surgery. Anesthesiology. (2010) 113(3):639–46. doi: 10.1097/ALN.0b013e3181e90914
204. Mcnicol ED, Schumann R, Haroutounian S. A systematic review and meta-analysis of ketamine for the prevention of persistent post-surgical pain. Acta Anaesthesiol Scand. (2014) 58(10):1199–213. doi: 10.1111/aas.12377
205. Schug SA, Peyton P. Does perioperative ketamine have a role in the prevention of chronic postsurgical pain: the ROCKet trial. Br J Pain. (2017) 11(4):166–8. doi: 10.1177/2049463717736076
206. Katalinic N, Lai R, Somogyi A, Mitchell PB, Glue P, Loo CK. Ketamine as a new treatment for depression: a review of its efficacy and adverse effects. Aust N Z J Psychiatry. (2013) 47(8):710–27. doi: 10.1177/0004867413486842
207. Gajraj NM. Pregabalin: its pharmacology and use in pain management. Anesth Analg. (2007) 105(6):1805–15. doi: 10.1213/01.ane.0000287643.13410.5e
208. Kharasch ED, Clark JD, Kheterpal S. Perioperative gabapentinoids: deflating the bubble. Anesthesiology. (2020) 133(2):251–4. doi: 10.1097/ALN.0000000000003394
209. Verret M, Lauzier F, Zarychanski R, Perron C, Savard X, Pinard A-M, et al. Perioperative use of gabapentinoids for the management of postoperative acute pain: a systematic review and meta-analysis. Anesthesiology. (2020) 133(2):265–79. doi: 10.1097/ALN.0000000000003428
210. Varrassi G, Pergolizzi JV, Dowling P, Paladini A. Ibuprofen safety at the golden anniversary: are all NSAIDs the same? A narrative review. Adv Ther. (2020) 37(1):61–82. doi: 10.1007/s12325-019-01144-9
211. Chang RW, Tompkins DM, Cohn SM. Are NSAIDs safe? Assessing the risk-benefit profile of nonsteroidal anti-inflammatory drug use in postoperative pain management. Am Surg. (2021) 87(6):872–9. doi: 10.1177/0003134820952834
212. Humble SR, Dalton AJ, Li L. A systematic review of therapeutic interventions to reduce acute and chronic post-surgical pain after amputation, thoracotomy or mastectomy. Eur J Pain. (2015) 19(4):451–65. doi: 10.1002/ejp.567
213. Harrogate SR, Cooper JA, Zawadka M, Anwar S. Seven-year follow-up of persistent postsurgical pain in cardiac surgery patients: a prospective observational study of prevalence and risk factors. Eur J Pain. (2021) 25(8):1829–38. doi: 10.1002/ejp.1794
214. Clarke H, Poon M, Weinrib A, Katznelson R, Wentlandt K, Katz J. Preventive analgesia and novel strategies for the prevention of chronic post-surgical pain. Drugs. (2015) 75(4):339–51. doi: 10.1007/s40265-015-0365-2
215. Fassoulaki A, Triga A, Melemeni A, Sarantopoulos C. Multimodal analgesia with gabapentin and local anesthetics prevents acute and chronic pain after breast surgery for cancer. Anesth Analg. (2005) 101(5):1427–32. doi: 10.1213/01.ANE.0000180200.11626.8E
216. Cabañero D, Maldonado R. Synergism between oral paracetamol and nefopam in a murine model of postoperative pain. Eur J Pain. (2021) 25(8):1770–87. doi: 10.1002/ejp.1787
217. Thapa P, Euasobhon P. Chronic postsurgical pain: current evidence for prevention and management. Korean J Pain. (2018) 31(3):155–73. doi: 10.3344/kjp.2018.31.3.155
218. Wylde V, Dennis J, Beswick AD, Bruce J, Eccleston C, Howells N, et al. Systematic review of management of chronic pain after surgery. Br J Surg. (2017) 104(10):1293–306. doi: 10.1002/bjs.10601
220. Abdi S, Datta S, Trescot AM, Schultz DM, Adlaka R, Atluri SL, et al. Epidural steroids in the management of chronic spinal pain: a systematic review. Pain Physician. (2007) 10(1):185–212. doi: 10.36076/ppj.2007/10/185
221. Guirguis MN, Abd-Elsayed AA, Girgis G, Soliman LM. Ultrasound-guided transversus abdominis plane catheter for chronic abdominal pain. Pain Pract. (2013) 13(3):235–8. doi: 10.1111/j.1533-2500.2012.00570.x
222. Wang LK, Chen HP, Chang PJ, Kang FC, Tsai YC. Axillary brachial plexus block with patient controlled analgesia for complex regional pain syndrome type I: a case report. Reg Anesth Pain Med. (2001) 26(1):68–71. doi: 10.1053/rapm.2001.9879
223. Fabregat G, Asensio-Samper JM, Palmisani S, Villanueva-Pérez VL, De Andrés J. Subcutaneous botulinum toxin for chronic post-thoracotomy pain. Pain Pract. (2013) 13(3):231–4. doi: 10.1111/j.1533-2500.2012.00569.x
224. Smoot D, Zielinski M, Jenkins D, Schiller H. Botox A injection for pain after laparoscopic ventral hernia: a case report. Pain Med. (2011) 12(7):1121–3. doi: 10.1111/j.1526-4637.2011.01147.x
225. Vaso A, Adahan HM, Gjika A, Zahaj S, Zhurda T, Vyshka G, et al. Peripheral nervous system origin of phantom limb pain. Pain. (2014) 155(7):1384–91. doi: 10.1016/j.pain.2014.04.018
226. Wijayasinghe N, Andersen KG, Kehlet H. Analgesic and sensory effects of the pecs local anesthetic block in patients with persistent pain after breast cancer surgery: a pilot study. Pain Pract. (2017) 17(2):185–91. doi: 10.1111/papr.12423
227. Wijayasinghe N, Duriaud HM, Kehlet H, Andersen KG. Ultrasound guided intercostobrachial nerve blockade in patients with persistent pain after breast cancer surgery: a pilot study. Pain Physician. (2016) 19(2):E309–18. doi: 10.36076/ppj/2016.19.E309
228. Abd-Elsayed A, Malyuk D. Efficacy of transversus abdominis plane steroid injection for treating chronic abdominal pain. Pain Pract. (2018) 18(1):48–52. doi: 10.1111/papr.12580
229. Baciarello M, Migliavacca G, Marchesini M, Valente A, Allegri M, Fanelli G. Transversus abdominis plane block for the diagnosis and treatment of chronic abdominal wall pain following surgery: a case series. Pain Pract. (2018) 18(1):109–17. doi: 10.1111/papr.12570
230. Collins KL, Russell HG, Schumacher PJ, Robinson-Freeman KE, O’Conor EC, Gibney KD, et al. A review of current theories and treatments for phantom limb pain. J Clin Invest. (2018) 128(6):2168–76. doi: 10.1172/JCI94003
Keywords: PPP, risks, pre-clinical models, mechanisms, prevention
Citation: Fuller AM, Bharde S and Sikandar S (2023) The mechanisms and management of persistent postsurgical pain. Front. Pain Res. 4:1154597. doi: 10.3389/fpain.2023.1154597
Received: 30 January 2023; Accepted: 16 June 2023;
Published: 6 July 2023.
Edited by:
Craig T. Hartrick, Oakland University, United StatesReviewed by:
Donald Simone, University of Minnesota Twin Cities, United States© 2023 Fuller, Bharde and Sikandar. This is an open-access article distributed under the terms of the Creative Commons Attribution License (CC BY). The use, distribution or reproduction in other forums is permitted, provided the original author(s) and the copyright owner(s) are credited and that the original publication in this journal is cited, in accordance with accepted academic practice. No use, distribution or reproduction is permitted which does not comply with these terms.
*Correspondence: Alice M. Fuller YS5mdWxsZXJAcW11bC5hYy51aw==
†These authors have contributed equally to this work
Disclaimer: All claims expressed in this article are solely those of the authors and do not necessarily represent those of their affiliated organizations, or those of the publisher, the editors and the reviewers. Any product that may be evaluated in this article or claim that may be made by its manufacturer is not guaranteed or endorsed by the publisher.
Research integrity at Frontiers
Learn more about the work of our research integrity team to safeguard the quality of each article we publish.