- 1Division of Pain Medicine, Department of Anesthesiology and Critical Care Medicine, Johns Hopkins University School of Medicine, Baltimore, MD, United States
- 2Department of Physical Medicine and Rehabilitation, Harvard Medical School, Boston, MA, United States
- 3Veterans Affairs Boston Healthcare System—West Roxbury Division, Spinal Cord Injury Service, Boston, MA, United States
- 4Department of Physical Medicine and Rehabilitation, Baylor College of Medicine, Houston, TX, United States
- 5Department of Orthopaedics and Physical Medicine, Medical University of South Carolina, Charleston, SC, United States
- 6Department of Physical Medicine and Rehabilitation, McGovern Medical School, University of Texas HSC at Houston, Houston, TX, United States
A high prevalence of patients with spinal cord injury (SCI) suffer from chronic neuropathic pain. Unfortunately, the precise pathophysiological mechanisms underlying this phenomenon have yet to be clearly elucidated and targeted treatments are largely lacking. As an unfortunate consequence, neuropathic pain in the population with SCI is refractory to standard of care treatments and represents a significant contributor to morbidity and suffering. In recent years, advances from SCI-specific animal studies and translational models have furthered our understanding of the neuronal excitability, glial dysregulation, and chronic inflammation processes that facilitate neuropathic pain. These developments have served advantageously to facilitate exploration into the use of neuromodulation as a treatment modality. The use of intrathecal drug delivery (IDD), with novel pharmacotherapies, to treat chronic neuropathic pain has gained particular attention in both pre-clinical and clinical contexts. In this evidence-based narrative review, we provide a comprehensive exploration into the emerging evidence for the pathogenesis of neuropathic pain following SCI, the evidence basis for IDD as a therapeutic strategy, and novel pharmacologics across impactful animal and clinical studies.
Background
Spinal cord injury (SCI) is associated with a plethora of neurological complications including spasticity, sensory deficits, weakness, and neuropathic pain (1–3). While there exist various treatment strategies to treat neuropathic pain in the context of peripheral neuropathy, the treatment of neuropathic pain following SCI is particularly challenging and is a significant contributor to morbidity and suffering (2, 4, 5). In part, this clinical challenge is secondary to an incomplete understanding of the pathophysiological mechanisms underlying this phenomenon and the associated lack of targeted treatment strategies.
While traditionally it was suggested that neuropathic pain is a sequela of injury to somatosensory pathways following SCI, advances from SCI-specific animal studies and translational models in recent years have further enhanced our mechanistic understanding (1, 4, 6, 7). Notably, concepts of neuronal excitability, glial dysregulation, and chronic inflammatory processes have been increasingly recognized (8–11). These developments have also served advantageously to facilitate exploration into the use of neuromodulation as a treatment modality. The use of intrathecal drug delivery (IDD) is a particularly promising neuromodulation intervention as it allows for the introduction of analgesic agents directly into the cerebrospinal fluid (CSF) (12–14). This direct delivery allows for analgesic agents to be administered in a targeted fashion proximal to the dorsal cord, which contains a majority of key pharmacologic targets.
While there has been an extensive emergence of both pre-clinical and clinical research exploring post-SCI neuropathic pain mechanisms and treatments, the comprehensive appraisal and contextualization of this research are largely lacking. In this evidence-based narrative review, we provide a comprehensive exploration into the emerging evidence for the pathogenesis of neuropathic pain following SCI, the rationale for IDD as a particularly beneficial therapeutic strategy, and novel pharmacologic across impactful animal and clinical studies.
Methods
This review was an evidence-based narrative aimed at characterizing the available evidence exploring intrathecal agents for the treatment of neuropathic pain following spinal cord injury. Both animal model and human studies were included so that we could provide a robust discussion on the mechanisms of neuropathic pain pathogenesis as they translate to the clinical setting. Data sources surveyed include PubMed, Medline, prior systematic reviews, and reference lists surveyed from 1950 through April 2022. All the animal and clinical studies with clearly designated SCI mechanisms, intrathecal interventions, and measured outcomes for analgesia were considered for inclusion. Those studies that included discussion on mechanisms of neuropathic pain after SCI and/or IDD as an intervention were included.
Results
Utilizing the aforementioned search strategy and study selection process, we identified a total of 25 studies to be included in our evidence-based narrative. These studies were divided into animal model studies (N = 15) (Table 1) (15–29) or clinical studies (N = 10) (Table 2) (30–39). All the animal studies utilized mice or rat models, of which a majority underwent lower thoracic cord constriction or compression-based SCI. Clinical studies were largely of lower-level data, including one case report along with several case-series or small cohort studies. However, we did identify two randomized controlled studies, although these studies also included rather small cohorts of 11 and 15 patients (Table 2).
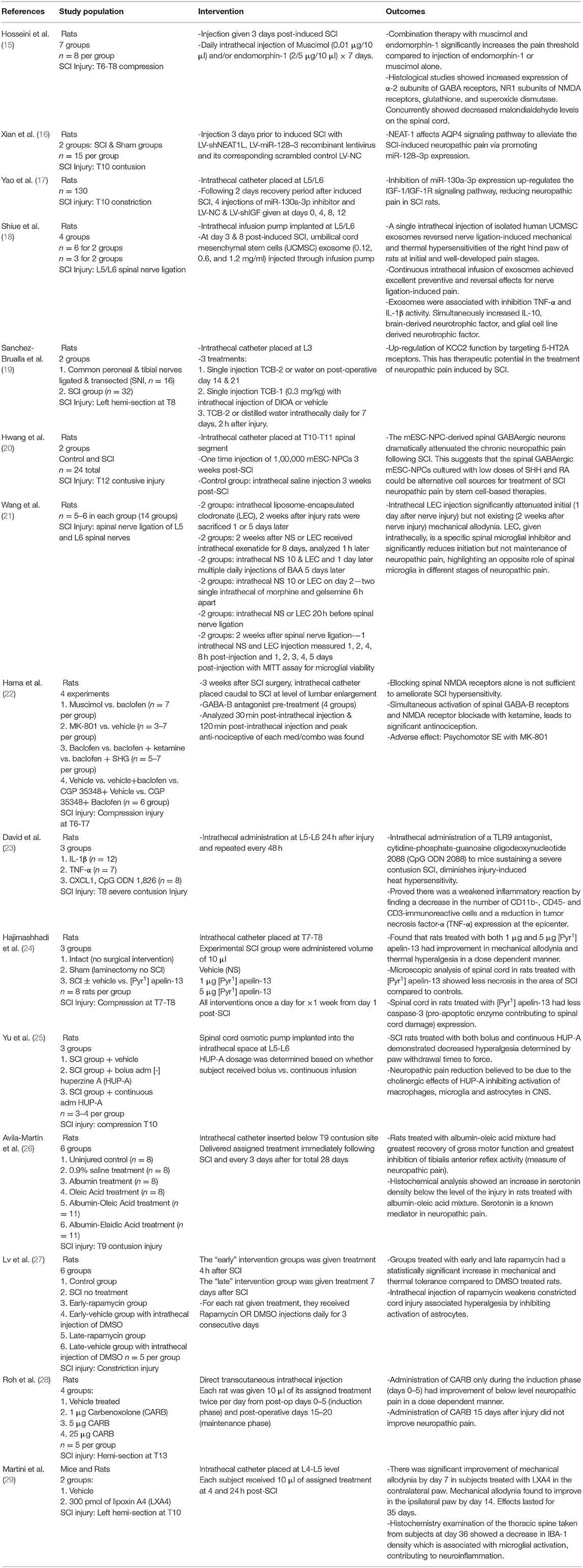
Table 1. Impactful animal studies exploring novel intrathecal therapies for the treatment of neuropathic pain following spinal cord injury.
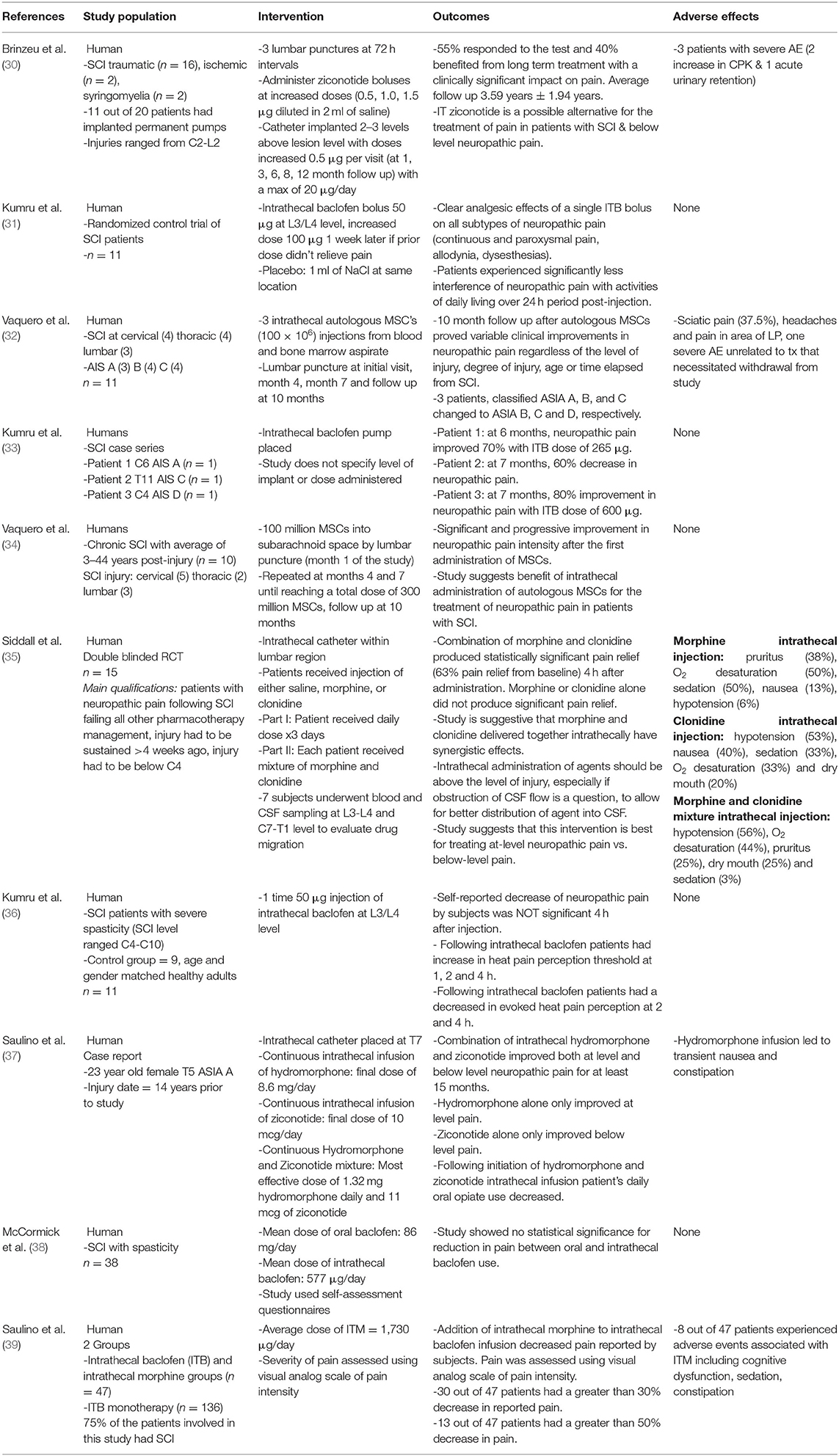
Table 2. Impactful clinical studies exploring novel intrathecal therapies for the treatment of neuropathic pain following spinal cord injury.
Discussion
Pathogenesis of Neuropathic Pain Following SCI: Established Evidence and Novel Understanding
Approximately 50% of individuals with SCI develop neuropathic pain, which is largely subdivided into above-level, at-level, or below-level pain presentations (4, 5, 7, 40). At-level neuropathic pain is the most prevalent subset and is designated by pain within one dermatome rostral and three dermatomes caudal to the neurological level of injury. A small subset of patients has below-level pain, which often affects the lower extremities in a more gradual pattern with less dysesthesia and allodynia than reported for at-level pain.
When attempting to understand the physiologic and mechanistic pathways leading to the emergence of neuropathic pain after a spinal cord injury, many established theories are rooted from studies of animal models of the peripheral nerve injury that originated in the 1950s (1, 4, 7, 40–43). This research, however, is limited given that the spinal cord contains a complex network of several tissue types, the vast majority of which are non-neural (44–46). Histological sections of the human spinal cord have found that the dorsal segments contain ~10-fold the density of glial cells relative to neurons (44). More nuanced animal studies utilizing animal models of SCI have been utilized in recent years and concordantly, there has been an emergence in the recognition of neuroimmune and glial dysregulation in facilitating neuropathic pain.
Studies from the early 1990s first demonstrated the presence of increased astrocyte density in the spinal dorsal horns as visualized by GFAP immunostaining (47, 48). More interestingly, this also correlated with hyperalgesia symptoms after a sciatic nerve compression. Subsequently, it was also demonstrated that glial activation led to hyperalgesia and inflammation in correlation with TNF-α. In the early 2000s, rat studies showed that rats with a thoracic 13 (T13) unilateral hemisection of the spinal cord produced microglia activation with TNF-α expression noted below the level of the lesion, which evidently corresponded with allodynia of the rat's hindpaw (9, 49, 50). Furthermore, when the rat with the T13 hemisection of the spinal cord was treated with a TNF-α blocker, such as etanercept, there was decreased mechanical allodynia displayed and decreased microglial activation. When specifically targeted with a microglial inhibitor, such as minocycline, the rat's pain behaviors were also improved, which showed that TNF-α in association with microglia played a critical role in the emergence of neuropathic pain after a spinal cord injury. Even more recently, inducible dysregulation macrophages have been shown to amplify chronic inflammation to facilitate pain and prevent neuronal regeneration (51–53). This diminished neuronal regeneration has been thought to counteract the productive benefits of spinal plasticity, which may advantageously dampen intraspinal pain circuitry (53–55). Just as importantly, it has been shown that this inducible inflammatory dysregulation and pain modulation may selectively occur at the spinal level, and not within supraspinal centers (55).
By taking a closer look into the interactions of these immune modulators, we can gain further understanding on the development of neuropathic pain after a spinal cord injury. Following SCI, nociceptors sensitize glial modulators, which include ATP, colony-stimulating factor-1 (CSF-1), chemokines, and cascade-6 (CASP6) (9, 48, 49). These modulators then activate the spinal microglia in the dorsal horns, thereby leading to increased expression of CX3CL1 receptors for the Fractalkine ligand for recruitment of immune cells and development of neuroinflammation. Microglia also enhance secretion of TNF-α and IL-1β, causing increased excitatory synaptic transmissions with decreased inhibitory transmissions of somatosensory information (including nociceptive) of the lamina II region of the spinal cord. Recent studies have also shown that activation of astrocytes in the dorsal horns causes an increased release of nerve growth factor (NGF), which leads to neuropathic pain (8, 9, 45–48). Thus, SCI causes activation of astrocytes and microglia that may extend longitudinally across the dorsal cord and facilitate neuropathic pain transmission in above-level, at-level, and below-level phenotypes.
When an SCI occurs, astrocytes usually respond first during the immune response and become reactive, leading to an increased release of cytokines (such as IL-1β, IL-6, TNF-α, and interferon-γ) and chemokines, which in turn leads to recruitment of neutrophils and pro-inflammatory macrophages (9, 48, 49). The adaptive immune response (including T- and B-lymphocytes) also contribute to the inflammatory response after a SCI which usually peaks in number around 1 week after injury and remains elevated during the chronic period. These processes of the immune response contribute to the notion of spinal neuroinflammation as a mechanism for the development of neuropathic pain after SCI.
Targeted Pharmacological Management: the Rationale for Intrathecal Drug Delivery
In addition to the neuropathic pain that develops as a consequence of glial activation and spinal neuroinflammation, there is also a neuronal dysregulation component that contributes to the development of neuropathic pain (8–11). Post-traumatic neuronal hyperexcitability and increased spontaneous activity in the dorsal root ganglion and spinal nerves correlate to noxious dysesthetic responses to chemical, mechanical, and thermal stimulation (1, 4, 5, 8–11). Nociceptive sensory neurons including myelinated A-delta fibers and unmyelinated C-fibers transmit ascending sensory input to supraspinal areas, including the brainstem and thalamus (8–11, 40). The transmitted nociceptive input is processed, and the midline relay is located at the periaqueductal gray and rostral ventromedial medulla before traveling down the descending pathways associated with the spinal cord. The usual modulators released as a communication process of the primary nociceptors include glutamate, ATP, neuropeptides (such as calcitonin gene-related peptide and substance P), chemokines (such as CCL2, CCL21, and CX3CL1), cytokines (such as IL-1β, IL-6, and TNF-α), and growth factors (such as BDNF, neuregulin 1, and basic fibroblast growth factor).
Irrespective of the precise mechanisms of microglial activation or spinal neuroinflammation, a majority of post-SCI noxious pathogenesis is thought to derive as a consequence of injury to somatosensory pathways. The delivery of spinal analgesics via IDD attenuates the transmission of pain pathways directly at the level of the dorsal columns, where noxious sensitization occurs (12–14). Consequently, IDD is a highly favorable pharmacologic delivery system given that most enteric medications fail to reach sufficient cerebrospinal fluid concentration and confer significant systemic side effects at higher dosages. Currently, the only FDA-approved medications for intrathecal analgesia include morphine and ziconotide. While not approved, other medications including bupivacaine, clonidine, and other opioids are supported for clinical use by the polyanalgesic consensus conference guidelines (12–14, 56). While all of these medications act largely to attenuate ascending somatosensory transmission and/or activate descending inhibitory pathways, medications to treat microglial activation and spinal neuroinflammation are lacking. As the importance and roles of these more novel mechanisms are increasingly recognized, we expect an emergence of targeted pharmacotherapies, including those that may be delivered intrathecally.
The pharmacokinetics of intrathecal agents are not fully understood but are largely thought to involve various parameters specific to the medication and catheter (12, 14). The major medication parameters are the drug baricity, the density relative to CSF, and octanol-water partition coefficient, the measure of lipophilicity (12–14, 56). Lipophilic agents, such as bupivacaine and fentanyl, have limited spread within the CSF and thereby may prove particularly advantageous for treating at-level neuropathic pain conditions. On the contrary, more diffuse pain syndromes may be better served with the use of hydrophilic agents. Similarly, hyperbaric agents demonstrate greater CSF spread relative to isobaric and hypobaric agents. With regard to catheter parameters, the tip location heavily influences medication response. Given that epidemiologically most patients with SCI have cervical level injuries, IDD catheters at the cervical level may prove most beneficial when attempting to use hyperbaric and lipophilic agents to treat at-level neuropathic pain (1–3, 12–14). However, the adverse effect associated with cervical catheter placement include medication diffusion to respiratory centers, leading to respiratory depression with opioid medications. Consequently, the catheter tip location must represent a judicious decision in accordance with the mono- or polyanalgesic pharmacotherapies utilized.
Future Promise of Intrathecal Drug Delivery: Novel and Emerging Pharmacotherapies
In patients with SCI, a reduction in inflammatory processes has been associated with a reduction of neuropathic pain. Common inflammatory markers, such as IL-6, IL-1β, and TNF-α, have all been known to increase following SCI, thereby, contributing to central nervous system inflammation (4, 18). These markers have differing roles in the inflammatory cascade and work by enhancing mechanical and heat hypersensitivity reactions that are measures of neuropathic pain in animal models. Animal studies have shown that reducing these inflammatory markers can be a promising mechanism to decrease neuropathic pain; however, this has not been tested in human subjects (18, 23, 57). For example, exosomes isolated from human umbilical mesenchymal stem cells have the ability of inhibiting TNF-α, IL-1β, while simultaneously increasing IL-10, an innate anti-inflammatory interleukin, when continuously infused into the intrathecal space (18). Pro-inflammatory pathways have been selectively targeted, such as toll-like-receptors (a component of the innate immune response), caspase-3 enzymes (proteolytic enzymes playing a role in apoptosis), and the ALX/FPR2 pathway (which modulates microglial activation) resulting in a decrease in neuropathic pain in rat subjects. As aforementioned, there are different pathways to evoke an anti-inflammatory response which has been proven to play a role in decreasing neuropathic pain in spinal cord injury. It is through these pathways that spinal cord inflammation can be reduced, leading to a decrease in neuropathic pain in rat subjects (23, 24, 29). In the study that targeted the ALX/FPR2 pathway with an endogenous lipid mediator, lipoxin A4, rodents demonstrated decreased mechanical allodynia for 35 days (29).
In addition, stem cell biologics display promise in improving neuropathic pain. Traditional stem cell therapy works to enhance local inflammation in tissues with limited vascularity to aid in healing. However, when used in spinal cord injury it can allow researchers to introduce known anti-neuropathic mediators directly to the site of injury. For example, mouse embryonic stem cell-derived neural pre-cursor cells (mESC-NPC) composed of GABAergic neurons were administered intrathecally 3 weeks after induced SCI; this resulted in a statistically significant reduction of neuropathic pain in rodents measured by mechanical allodynia and vocalization at the end of the 7-week threshold (20). Other studies have shown that persistent neuropathic pain following SCI is due to a loss of GABA inhibitory influences on the spinal dorsal horn neurons. Vaquero et al. introduced this idea to patients with SCI and injected 100 million autologous mesenchymal stromal cells (MSC) at three different times post-injury. At the 10-month follow-up, patients reported significant improvement in neuropathic pain (34). In another study with 11 patients with SCI with different incomplete AIS classifications, Vaquero et al. (32) was again able to further exhibit that patients given intrathecal MSC had a variable response in a reduction in the neuropathic pain. Since the study of MSC is in its infancy, further study is required, particularly with a focus on adverse effects and optimizing dose-benefit profiles.
A smaller subset of experimental treatments has been explored looking at the gene-based therapies aimed at producing nociceptive effects on the neuropathic pain. The wide breadth of the possibilities with these therapies has given researchers the ability to manipulate upstream and downstream genes that work to directly contribute to neuropathic pain. Animal rat studies inhibiting the expression of a specific microRNA allowed for the insulin-like growth factor signaling pathway (IGF-1/IGF-1R) to be upregulated, mitigating neuronal apoptosis and microglial activation (17). On the other hand, promoting the expression of long non-coding RNA variants, such as miR-128–3p, attenuates inflammation and clinically correlates with a reduction of neuropathic pain (16). The versatile utility of gene-based therapies has not been trialed with human subjects but has great future promise. Most importantly, within the studies performed utilizing gene-based therapies, no adverse effects have been documented within the rodent population.
As aforementioned, the only FDA-approved spinal analgesics include ziconotide and morphine. Intrathecal baclofen (ITB) is FDA approved for use in spasticity management, however, and interestingly may prove beneficial as an analgesic compound separate from its spasmolytic properties (31, 33, 36). When delivered intrathecally, baclofen works as a GABA-B analog and can target neurons in the dorsal horns with high-density GABA-B receptors. Neuropathic pain has been believed to be a result of neuronal hyperexcitability in this region due to loss of GABA inhibition. Oral baclofen has no statistically significant evidence for the reduction in neuropathic pain compared to ITB (38). However, these differences in analgesic capacity may be secondary to pharmacokinetic disparities given that conventional enteral baclofen doses reach minimal concentrations in the CSF.
The utility of ITB as an analgesic compound has been evidenced by the high -level randomized control trials. In these trials patients had reductions in neuropathic pain and improvements in their activities of daily living within 24 h post-injection of ITB (31). There does exist a great difficulty in characterizing ITB's analgesic-specific benefits given that the treatment of spasticity is a significant confounder. A recent review by Karri et al. (13) explored this phenomenon and concluded that ITB may be an effective analgesic agent independent of its spasmolytic effects. Although the evidence basis reviewed was largely vague, given the absence of clearly defined pain and spasticity outcome measures. Nonetheless, ITB was deemed to be a relatively safe and particularly beneficial therapy that warrants consideration in those patients with SCI with severe spasticity as well as neuropathic pain. Although, careful clinical surveillance and follow-up are prudent in this population given that ITB withdrawal specifically can prove fatal, unlike with withdrawal phenomena to other intrathecal agents including opioids and ziconotide.
Conclusion
Advances from animal studies and translational models continue to demonstrate that neuropathic pain following SCI involves complex pathogenesis that includes neuronal excitability, glial dysregulation, and chronic inflammation. While currently utilized intrathecal analgesic agents provide analgesic benefits, targeted treatments to modulate underlying pathogenesis are largely lacking. However, there exists increasingly recognized research supporting the promise of intrathecal immunomodulators, stem-cell-based treatments, and even genetic therapies for use in the chronic pain treatment. In addition, the use of ITB for analgesic indications is not approved but appears to have some rationale in patients with SCI. Given the benefits of targeted, site-specific pain treatment, IDD is a particularly beneficial treatment strategy in appropriate individuals and its benefits will only be commensurate with forthcoming novel pharmacologics.
Author Contributions
JK, AN, and SL conceived the idea and objectives of the project, made final revisions, and prepared the manuscript for submission. JK, JD, CV, and MC participated in literature review and principle authorship. All authors meet the ICMJE criteria for authorship, take responsibility for the integrity of this work, and have given their approval for publication.
Conflict of Interest
The authors declare that the research was conducted in the absence of any commercial or financial relationships that could be construed as a potential conflict of interest.
Publisher's Note
All claims expressed in this article are solely those of the authors and do not necessarily represent those of their affiliated organizations, or those of the publisher, the editors and the reviewers. Any product that may be evaluated in this article, or claim that may be made by its manufacturer, is not guaranteed or endorsed by the publisher.
References
1. Finnerup NB. Neuropathic pain and spasticity: intricate consequences of spinal cord injury. Spinal Cord. (2017) 55:1046–50. doi: 10.1038/sc.2017.70
2. Jensen MP, Kuehn CM, Amtmann D, Cardenas DD. Symptom burden in persons with spinal cord injury. Arch Phys Med Rehabil. (2007) 88:638–45. doi: 10.1016/j.apmr.2007.02.002
3. Thomas CK, Zaidner EY, Calancie B, Broton JG, Bigland-Ritchie BR. Muscle weakness, paralysis, and atrophy after human cervical spinal cord injury. Exp Neurol. (1997) 148:414–23. doi: 10.1006/exnr.1997.6690
4. Hulsebosch CE, Hains BC, Crown ED, Carlton SM. Mechanisms of chronic central neuropathic pain after spinal cord injury. Brain Res Rev. (2009) 60:202–13. doi: 10.1016/j.brainresrev.2008.12.010
5. Siddall PJ. Management of neuropathic pain following spinal cord injury: now and in the future. Spinal Cord. (2009) 47:352–9. doi: 10.1038/sc.2008.136
6. Yezierski RP. Spinal cord injury: a model of central neuropathic pain. Neurosignals. (2005) 14:182–93. doi: 10.1159/000087657
7. Finnerup NB, Jensen TS. Spinal cord injury pain–mechanisms and treatment. Eur J Neurol. (2004) 11:73–82. doi: 10.1046/j.1351-5101.2003.00725.x
8. Gwak YS, Hulsebosch CE, Leem JW. Neuronal-glial interactions maintain chronic neuropathic pain after spinal cord injury. Neural Plast. (2017) 2017:1–11. doi: 10.1155/2017/2480689
9. Gwak YS, Kang J, Unabia GC, Hulsebosch CE. Spatial and temporal activation of spinal glial cells: role of gliopathy in central neuropathic pain following spinal cord injury in rats. Exp Neurol. (2012) 234:362–72. doi: 10.1016/j.expneurol.2011.10.010
10. Allison DJ, Thomas A, Beaudry K, Ditor DS. Targeting inflammation as a treatment modality for neuropathic pain in spinal cord injury: a randomized clinical trial. J Neuroinflammation. (2016) 13:152. doi: 10.1186/s12974-016-0625-4
11. Hulsebosch CE. Gliopathy ensures persistent inflammation and chronic pain after spinal cord injury. Exp Neurol. (2008) 214:6–9. doi: 10.1016/j.expneurol.2008.07.016
12. Prager J, Deer T, Levy R, Bruel B, Buchser E, Caraway D, et al. Best practices for intrathecal drug delivery for pain. Neuromodulation. (2014) 17:354–72. doi: 10.1111/ner.12146
13. Karri J, Singh M, Modi DJ, Orhurhu V, Seale C, Saulino M, et al. Combination intrathecal drug therapy strategies for pain management. Pain Phys. (2021) 24:549–69.
14. Abd-Elsayed A, Karri J, Michael A, Bryce D, Sun J, Lee M, et al. Intrathecal drug delivery for chronic pain Syndromes: a review of considerations in practice management. Pain Physician. (2020) 23:E591–617. doi: 10.36076/ppj.2020.23.E591
15. Hosseini M, Karami Z, Yousefifard M, Janzadeh A, Zamani E, Nasirinezhad F. Simultaneous intrathecal injection of muscimol and endomorphin-1 alleviates neuropathic pain in rat model of spinal cord injury. Brain Behav. (2020) 10:e01576. doi: 10.1002/brb3.1576
16. Xian S, Ding R, Li M, Chen F. LncRNA NEAT1/miR-128-3p/AQP4 axis regulating spinal cord injury-induced neuropathic pain progression. J Neuroimmunol. (2021) 351:577457. doi: 10.1016/j.jneuroim.2020.577457
17. Yao L, Guo Y, Wang L, Li G, Qian X, Zhang J, et al. Knockdown of miR-130a-3p alleviates spinal cord injury induced neuropathic pain by activating IGF-1/IGF-1R pathway. J Neuroimmunol. (2021) 351:577458. doi: 10.1016/j.jneuroim.2020.577458
18. Shiue SJ, Rau RH, Shiue HS, Hung YW, Li ZX, Yang KD, et al. Mesenchymal stem cell exosomes as a cell-free therapy for nerve injury-induced pain in rats. Pain. (2019) 160:210–23. doi: 10.1097/j.pain.0000000000001395
19. Sánchez-Brualla I, Boulenguez P, Brocard C, Liabeuf S, Viallat-Lieutaud A, Navarro X, et al. Activation of 5-HT2A receptors restores KCC2 function and reduces neuropathic pain after spinal cord injury. Neuroscience. (2018) 387:48–57. doi: 10.1016/j.neuroscience.2017.08.033
20. Hwang I, Hahm SC, Choi KA, Park SH, Jeong H, Yea JH, et al. Intrathecal transplantation of embryonic stem cell-derived spinal GABAergic neural pre-cursor cells attenuates neuropathic pain in a spinal cord injury rat model. Cell Transplant. (2016) 25:593–607. doi: 10.3727/096368915X689460
21. Wang YR, Mao XF, Wu HY, Wang YX. Liposome-encapsulated clodronate specifically depletes spinal microglia and reduces initial neuropathic pain. Biochem Biophys Res Commun. (2018) 499:499–505. doi: 10.1016/j.bbrc.2018.03.177
22. Hama A, Sagen J. Combinations of intrathecal gamma-amino-butyrate receptor agonists and N-methyl-d-aspartate receptor antagonists in rats with neuropathic spinal cord injury pain. Eur J Pharmacol. (2012) 683:101–8. doi: 10.1016/j.ejphar.2012.03.015
23. David BT, Ratnayake A, Amarante MA, Reddy NP, Dong W, Sampath S, et al. A toll-like receptor 9 antagonist reduces pain hypersensitivity and the inflammatory response in spinal cord injury. Neurobiol Dis. (2013) 54:194–205. doi: 10.1016/j.nbd.2012.12.012
24. Hajimashhadi Z, Aboutaleb N, Nasirinezhad F. Chronic administration of [Pyr1] apelin-13 attenuates neuropathic pain after compression spinal cord injury in rats. Neuropeptides. (2017) 61:15–22. doi: 10.1016/j.npep.2016.08.010
25. Yu D, Thakor DK, Han I, Ropper AE, Haragopal H, Sidman RL, et al. Alleviation of chronic pain following rat spinal cord compression injury with multimodal actions of huperzine A. Proc Natl Acad Sci USA. (2013) 110:E746–55. doi: 10.1073/pnas.1300083110
26. Avila-Martin G, Galan-Arriero I, Gómez-Soriano J, Taylor J. Treatment of rat spinal cord injury with the neurotrophic factor albumin-oleic acid: translational application for paralysis, spasticity and pain. PLoS ONE. (2011) 6:e26107. doi: 10.1371/journal.pone.0026107
27. Lv J, Li Z, She S, Xu L, Ying Y. Effects of intrathecal injection of rapamycin on pain threshold and spinal cord glial activation in rats with neuropathic pain. Neurol Res. (2015) 37:739–43. doi: 10.1179/1743132815Y.0000000052
28. Roh DH, Yoon SY, Seo HS, Kang SY, Han HJ, Beitz AJ, et al. Intrathecal injection of carbenoxolone, a gap junction decoupler, attenuates the induction of below-level neuropathic pain after spinal cord injury in rats. Exp Neurol. (2010) 224:123–32. doi: 10.1016/j.expneurol.2010.03.002
29. Martini AC, Berta T, Forner S, Chen G, Bento AF, Ji RR, et al. Lipoxin A4 inhibits microglial activation and reduces neuroinflammation and neuropathic pain after spinal cord hemisection. J Neuroinflammation. (2016) 13:75. doi: 10.1186/s12974-016-0540-8
30. Brinzeu A, Berthiller J, Caillet JB, Staquet H, Mertens P. Ziconotide for spinal cord injury-related pain. Eur J Pain. (2019) 23:1688–700. doi: 10.1002/ejp.1445
31. Kumru H, Benito-Penalva J, Kofler M, Vidal J. Analgesic effect of intrathecal baclofen bolus on neuropathic pain in spinal cord injury patients. Brain Res Bull. (2018) 140:205–11. doi: 10.1016/j.brainresbull.2018.05.013
32. Vaquero J, Zurita M, Rico MA, Aguayo C, Bonilla C, Marin E, et al. Intrathecal administration of autologous mesenchymal stromal cells for spinal cord injury: safety and efficacy of the 100/3 guideline. Cytotherapy. (2018) 20:806–19. doi: 10.1016/j.jcyt.2018.03.032
33. Kumru H, Albu S, Kofler M, Vidal J. The long-term analgesic effect of intrathecal baclofen on neuropathic pain in patients with spinal cord injury. Neurologia. (2020) 35:679–81. doi: 10.1016/j.nrleng.2019.09.006
34. Vaquero J, Zurita M, Rico MA, Aguayo C, Fernández C, Gutiérrez R, et al. Intrathecal administration of autologous bone marrow stromal cells improves neuropathic pain in patients with spinal cord injury. Neurosci Lett. (2018) 670:14–8. doi: 10.1016/j.neulet.2018.01.035
35. Siddall PJ, Molloy AR, Walker S, Mather LE, Rutkowski SB, Cousins MJ. The efficacy of intrathecal morphine and clonidine in the treatment of pain after spinal cord injury. Anesth Analg. (2000) 91:1493–8. doi: 10.1097/00000539-200012000-00037
36. Kumru H, Kofler M, Flores MC, Portell E, Robles V, Leon N, et al. Effect of intrathecal baclofen on evoked pain perception: an evoked potentials and quantitative thermal testing study. Eur J Pain. (2013) 17:1039–47. doi: 10.1002/j.1532-2149.2012.00266.x
37. Saulino M. Successful reduction of neuropathic pain associated with spinal cord injury via of a combination of intrathecal hydromorphone and ziconotide: a case report. Spinal Cord. (2007) 45:749–52. doi: 10.1038/sj.sc.3102027
38. McCormick ZL, Chu SK, Binler D, Neudorf D, Mathur SN, Lee J, et al. Intrathecal versus oral baclofen: a matched cohort study of spasticity, pain, sleep, fatigue, and quality of life. PM R. (2016) 8:553–62. doi: 10.1016/j.pmrj.2015.10.005
39. Saulino M. Simultaneous treatment of intractable pain and spasticity: observations of combined intrathecal baclofen-morphine therapy over a 10-year clinical experience. Eur J Phys Rehabil Med. (2012) 48:39–45.
40. Huang Q, Duan W, Sivanesan E, Liu S, Yang F, Chen Z, et al. Spinal cord stimulation for pain treatment after spinal cord injury. Neurosci Bull. (2019) 35:527–39. doi: 10.1007/s12264-018-0320-9
41. Decosterd I, Woolf CJ. Spared nerve injury: an animal model of persistent peripheral neuropathic pain. Pain. (2000) 87:149–58. doi: 10.1016/S0304-3959(00)00276-1
42. Bennett GJ. An animal model of neuropathic pain: a review. Muscle Nerve. (1993) 16:1040–8. doi: 10.1002/mus.880161007
43. Lee BH, Won R, Baik EJ, Lee SH, Moon CH. An animal model of neuropathic pain employing injury to the sciatic nerve branches. Neuroreport. (2000) 11:657–61. doi: 10.1097/00001756-200003200-00002
44. Ruiz-Sauri A, Orduña-Valls JM, Blasco-Serra A, Tornero-Tornero C, Cedeño DL, Bejarano-Quisoboni D, et al. Glia to neuron ratio in the posterior aspect of the human spinal cord at thoracic segments relevant to spinal cord stimulation. J Anat. (2019) 235:997–1006. doi: 10.1111/joa.13061
45. Vallejo R, Tilley DM, Vogel L, Benyamin R. The role of glia and the immune system in the development and maintenance of neuropathic pain. Pain Pract. (2010) 10:167–84. doi: 10.1111/j.1533-2500.2010.00367.x
46. Vallejo R, Kelley CA, Gupta A, Smith WJ, Vallejo A, Cedeño DL. Modulation of neuroglial interactions using differential target multiplexed spinal cord stimulation in an animal model of neuropathic pain. Mol Pain. (2020) 16:1744806920918057. doi: 10.1177/1744806920918057
47. Popovich PG, Wei P, Stokes BT. Cellular inflammatory response after spinal cord injury in Sprague-Dawley and Lewis rats. J Comp Neurol. (1997) 377:443–64. doi: 10.1002/(sici)1096-9861(19970120)377:3<443::aid-cne10>3.0.co;2-s
48. Murray M, Wang SD, Goldberger ME, Levitt P. Modification of astrocytes in the spinal cord following dorsal root or peripheral nerve lesions. Exp Neurol. (1990) 110:248–57. doi: 10.1016/0014-4886(90)90036-R
49. Chambel S, Tavares I, Cruz C. Chronic pain after spinal cord injury: is there a role for neuron-immune dysregulation? Front Physiol. (2020) 11:748. doi: 10.3389/fphys.2020.00748
50. Walters E. Neuroinflammatory contributions to pain after SCI: roles for central glial mechanisms, an nociceptor-mediated host defense. Exp Neurol. (2014) 258:48–61. doi: 10.1016/j.expneurol.2014.02.001
51. Zhou X, He X, Ren Y. Function of microglia and macrophages in secondary damage after spinal cord injury. Neural Regen Res. (2014) 9:1787. doi: 10.4103/1673-5374.143423
52. Okubo M, Yamanaka H, Kobayashi K, Dai Y, Kanda H, Yagi H, et al. Macrophage-colony stimulating factor derived from injured primary afferent induces proliferation of spinal microglia and neuropathic pain in rats. PLoS ONE. (2016) 11:e0153375. doi: 10.1371/journal.pone.0153375
53. Taves S, Berta T, Chen G, Ji RR. Microglia and spinal cord synaptic plasticity in persistent pain. Neural Plast. (2013) 2013:1–9. doi: 10.1155/2013/753656
54. Chen G, Zhang YQ, Qadri YJ, Serhan CN, Ji RR. Microglia in pain: detrimental and protective roles in pathogenesis and resolution of pain. Neuron. (2018) 100:1292–311. doi: 10.1016/j.neuron.2018.11.009
55. Zhang F, Vadakkan KI, Kim SS, Wu LJ, Shang Y, Zhuo M. Selective activation of microglia in spinal cord but not higher cortical regions following nerve injury in adult mouse. Mol Pain. (2008) 4:1744–8069. doi: 10.1186/1744-8069-4-15
56. Deer TR, Pope JE, Hayek SM, Bux A, Buchser E, Eldabe S, et al. The polyanalgesic consensus conference (PACC): recommendations on intrathecal drug infusion systems best practices and guidelines. Neuromodulation. (2017) 20:96–132. doi: 10.1111/ner.12538
Keywords: spinal cord injury, neuropathic pain, intrathecal drug delivery, glial dysfunction, chronic inflammation
Citation: Karri J, Doan J, Vangeison C, Catalanotto M, Nagpal AS and Li S (2022) Emerging Evidence for Intrathecal Management of Neuropathic Pain Following Spinal Cord Injury. Front. Pain Res. 3:933422. doi: 10.3389/fpain.2022.933422
Received: 30 April 2022; Accepted: 14 June 2022;
Published: 28 July 2022.
Edited by:
Monique Van Velzen, Leiden University Medical Center, NetherlandsReviewed by:
Sufang Liu, Texas A&M University, United StatesCopyright © 2022 Karri, Doan, Vangeison, Catalanotto, Nagpal and Li. This is an open-access article distributed under the terms of the Creative Commons Attribution License (CC BY). The use, distribution or reproduction in other forums is permitted, provided the original author(s) and the copyright owner(s) are credited and that the original publication in this journal is cited, in accordance with accepted academic practice. No use, distribution or reproduction is permitted which does not comply with these terms.
*Correspondence: Jay Karri, amF5a2FycmlAZ21haWwuY29t