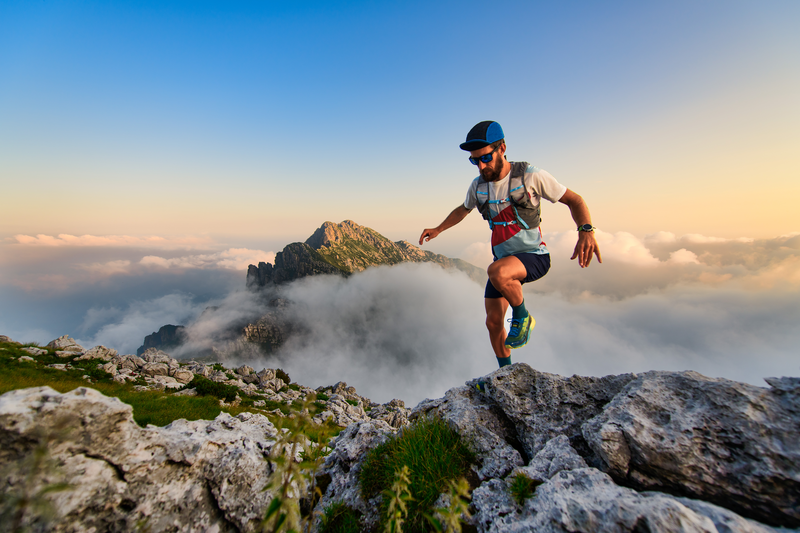
95% of researchers rate our articles as excellent or good
Learn more about the work of our research integrity team to safeguard the quality of each article we publish.
Find out more
ORIGINAL RESEARCH article
Front. Pain Res. , 27 April 2022
Sec. Pediatric Pain
Volume 3 - 2022 | https://doi.org/10.3389/fpain.2022.872587
Neonatal painful procedures causes acute pain and trigger long-term changes in nociceptive processing and anxiety behavior, highlighting the need for adequate analgesia during this critical time. Spinal serotonergic receptors 5-HT1a and 5-HT3 play an important role in modulating incoming nociceptive signals in neonates. The current study aims to attenuate acute and long-term hypersensitivity associated with neonatal procedural pain using ondansetron (a 5-HT3 antagonist) and buspirone (a 5-HT1a agonist) in a well-established rat model of repetitive needle pricking. Sprague-Dawley rat pups of both sexes received ondansetron (3 mg/kg), buspirone (3 mg/kg) or saline prior to repetitive needle pricks into the left hind-paw from postnatal day 0–7. Control animals received tactile stimulation or were left undisturbed. Acute, long-term, and post-operative mechanical sensitivity as well as adult anxiety were assessed. Neonatal 5-HT1a receptor agonism completely reverses acute hypersensitivity from P0-7. The increased duration of postoperative hypersensitivity after re-injury in adulthood is abolished by 5-HT3 receptor antagonism during neonatal repetitive needle pricking, without affecting baseline sensitivity. Moreover, 5-HT1a and 5-HT3 receptor modulation decreases adult state anxiety. Altogether, our data suggests that targeted pharmacological treatment based on the modulation of spinal serotonergic network via the 5-HT1a and 5-HT3 receptors in neonates may be of use in treatment of neonatal procedural pain and its long-term consequences. This may result in a new mechanism-based therapeutic venue in treatment of procedural pain in human neonates.
Newborns admitted to the neonatal intensive care unit (NICU) can be exposed to repetitive nociceptive input during a vulnerable time frame for the central nervous system, as part of their care and treatment. The neonatal period is unique in how it processes nociceptive stimuli, and the nociceptive network undergoes developmental changes in early life (1–3). Due to its activity-dependent maturation, the nociceptive network develops differently when exposed to excessive noxious input in early life (4–7). Both clinical and fundamental studies have provided evidence for neonatal painful stimulation induced alterations in the developing nociceptive network, resulting in subsequent long-term alterations in pain sensitivity (3, 8–11) and exacerbated sensitivity after re-injury in adulthood (12–17). Neonatal procedural pain induced effects are not limited to the nociceptive network but also affect anxiety behavior amongst others (18–23), suggesting a common neurocircuitry that regulates both pain and anxiety behavior is involved.
Serotonin (5-hydroxytryptamine, 5-HT) is a key modulator of anxiety behavior and perception of pain, and its ascending and descending fibers are distributed throughout the brain and spinal cord (24). Virtually all serotonergic innervation of the spinal cord originates from supraspinal sources in the brainstem, specifically the nucleus raphe magnus (NRM), which provides serotonergic input to the spinal dorsal horn (25). Based on the receptors involved, the net effect of serotonergic descending modulation might be either inhibitory or facilitatory (26). In the healthy adult rodent, serotonin is inhibiting spinal nociceptive neurotransmission (26).
Descending serotonergic modulation of the spinal nociceptive network, originating in the rostral ventromedial medulla (RVM), is immature in early life and undergoes developmental changes in anatomy and functionality. Importantly, the functionality switches from facilitation to inhibition of nociceptive signaling in early life (26, 27). Since treatment of neonatal pain is still a clinical challenge, analgesic therapy that considers the neurodevelopmental phase may result in more targeted treatment of neonatal procedural pain (27). In this study, we focus on two serotonin receptors: the inhibitory 5-HT1a and the excitatory 5-HT3. The serotonin receptors 5-HT1a is a Gi-coupled, transmembrane receptor expressed in the spinal dorsal horn, mainly on nociceptive afferents and inhibitory interneurons. When activated, the 5-HT1a receptor leads to inhibition of nociceptive signaling through a reduced glutamate release from primary afferent terminals (28). The 5-HT3 is a ligand-gated ion channel, expressed on nociceptive afferents, projection neurons, and both inhibitory and excitatory interneurons. Activation of this receptor leads to antinociception through activation of inhibitory interneurons in the spinal cord, who in turn release GABA and reduce transmission of nociceptive signals. However, 5-HT3 is also been implied in facilitation of nociceptive stimuli, especially in the neonatal period, through its expression on wide dynamic range (WDR) secondary nociceptive afferents (25).
During the neonatal period, facilitation of nociceptive signaling is mediated via the 5-HT3 receptors (R) in the dorsal horn (28–30) whereas the 5-HT1aR plays a role in inhibition of nociception (25, 28, 29, 31, 32). Therefore, activation of the 5-HT1aR or inactivation of the 5-HT3R in neonates may provide neurodevelopmental relevant analgesia, and could restore injury-evoked disruptions in the normal balance of excitation and inhibition in the spinal dorsal horn (17, 33–35). In addition, early postnatal signaling of the 5-HT1aR is required for normal development of anxiety-related brain areas and behavior (36, 37). Antagonizing the 5-HT3R in adulthood shows promising anxiolytic effects (38). Targeting the 5-HT3R and 5-HT1aR in newborns may therefore attenuate both nociception and anxiety related long-term consequences of neonatal painful stimuli, while providing adequate acute analgesia.
The aim of this study is to prevent the acute and long-term consequences of repetitive neonatal procedural pain by pharmacological targeting the serotonin 5-HT1aR or 5-HT3R in neonates. Here, we used buspirone, a full agonist of the 5-HT1aR to produce inhibition (39), or ondansetron, a selective 5-HT3R antagonist to prevent facilitation (40), in a well-established rat model of neonatal repetitive needle pricking. The effects of these interventions are studied in the context of acute, long-term and post-operative pain (or mechanical sensitivity) as well as adult anxiety.
All experiments and procedures were performed in line with the European Directive for Protection of Vertebrate Animal Used for Experimental and Other Scientific Purposes (2010/63/EU), and were ethically approved by the Committee for Experiments on Animals, Maastricht University, Maastricht, the Netherlands (DEC protocol 2017-017). Adult Sprague-Dawley rats were purchased from Charles River Laboratory (Sulzfeld, Germany), and were mated at Maastricht University. Animals were housed under reversed day-night conditions (12:12 h, lights on 7 p.m.– 7 a.m.), in temperature (21 ± 1°C) and humidity (55 ± 15%) controlled rooms with food and water available ad libitum. Since the circadian rhythm of animals seems capable of affecting pain sensitivity, especially mechanical sensitivity [reviewed in (41)], all behavioral experiments were performed in the active, and thus dark phase. Pups were collected and sexed at birth [postnatal (P) day 0], and litters were culled to a maximum of 10 pups to ensure equal caretaking by the dam. Conditions were distributed equally over litters and sexes (Table 1). Pups were weaned at P21 and randomly housed in same-sex groups of two or three in individually ventilated cages for the remainder of the study. The animal order of behavioral testing was randomized, and researchers were blinded to treatment groups throughout the entire experimental protocol. Behavioral testing and surgeries were performed by the same researcher.
On the day of birth (P0) newborn pups were randomized, using a computer-generated randomization list, to one of four conditions; 1. repetitive needle pricking (needle pricking, NP; n = 12), 2. NP with buspirone treatment (NP+BUS; 3 mg/kg; n = 12), 3. NP with ondansetron treatment (NP+OND; 3 mg/kg; n = 13) or 4. repetitive tactile stimulation (TC; n = 12). A separate litter was left completely undisturbed (UC; n = 10). Rat pups (conditions 1–3) were noxiously stimulated with repetitive needle pricks (NP) four times a day at 09:00 a.m., 10:00 a.m., 11:00 a.m. and 12:00 p.m. from P0 to P7 as previously described (13, 14). Each noxious stimulation consisted of a single 2 mm deep needle prick with a 25G needle in the mid-plantar surface of the left hind paw. Age-matched littermates used for the tactile stimulation (TC) (condition 4) received four tactile stimulations by stroking the left hind haw with a cotton tip swab at similar intervals. Drug doses (50 μl) were administered subcutaneously (sc.) 10 min before the first and third NP to cover the entire period of repetitive needle pricking. TC and NP animals received sc. saline to control for injection procedures and handling. Mechanical sensitivity was assessed before (baseline, BL) and 1, 3, and 5 h after the last needle prick or tactile stimulation from P0 to P7, using dorsal application of calibrated Von Frey filaments [bending force 0.407, 0.692, 1.202, 2.041, 3.63, (from P4 onwards) and 5.495 (from P6 onwards)].
For 5-HT3R antagonism, the selective 5-HT3R antagonist Ondansetron (OND; ondansetron hydrochloride dihydrate, Sigma-Aldrich, O3639) was dissolved in sterile saline in a concentration of 2 μg/μl. For 5-HT1aR agonism, the selective agonist Buspirone (BUS, buspirone hydrochloride; Sigma-Aldrich, B7148) was dissolved in sterile saline to a concentration of 10 μg/μl. Pilot studies testing 1, 3 and 5 mg/kg BUS and 0.3, 1 and 3 mg/kg OND showed that 3 mg/kg BUS and 3 mg/kg OND were most promising in preventing acute hypersensitivity associated with neonatal repetitive needle pricking (see Figure 1), without adverse side effects (including body weight changes or mortality). Based on pup's body weight per postnatal day, 50 μl solution containing OND (3 mg/kg) or BUS (3 mg/kg) was injected sc. twice daily (at 9 a.m. before the 1st needle prick and 11 a.m. before the 3 rd needle prick). TC and NP animals received an equivalent volume of saline.
Figure 1. Dose-response pilots of ondansetron (0.1, 1, and 3 mg/kg) and buspirone (1, 3, and 5 mg/kg). (A) PWTs increase over time, but not equally for all conditions [F (93, 636) = 4.841; p < 0.01]. Repetitive needle pricking (NP; n = 12) results in a decreased paw-withdrawal threshold (PWT) over time, that was significantly different from OND 3 mg/kg from P5 onwards. (B) The area under the curve (AUC) over the entire neonatal period is significantly different between neonatal conditions for the ipsilateral paw-withdrawal thresholds (PWT) [F (3, 16) = 4.432; p = 0.0189]. AUC was significantly increased in NP animals after administration of 0.3 mg/kg (p = 0.0329) and OND 3 mg/kg (p = 0.0143), as compared to NP animals receiving saline. (C) PWTs increase over time, but not equally for all groups [F (93,496) = 2.600; p < 0.01]. Repetitive needle pricking (NP) results in decreased PWTs over time, that was significant different from BUS 1 mg/kg from P5 onwards, from BUS 3 mg/kg from P4 onwards and BUS 5 mg/kg from P3 onwards. (D) The AUC over the entire neonatal period is significantly different between conditions [F (3, 16) = 3.745; p = 0.0327]. NP animals receiving BUS 5 mg/kg showed significantly increased AUC as compared to NP animals receiving saline (p = 0.0151). BS, baseline measurement; NP, needle pricking animals; BUS, buspirone; OND, ondansetron; P (0–7), postnatal day 0–7; +1, +3, +5, measurement 1, 3 and 5 h after needle prick or tactile stimulation. Data is presented as mean ± SEM, NP vs. NP+BUS. *P < 0.05.
To assess the effect of neonatal 5-HT1aR agonism and 5-HT3R antagonism on general postnatal development, physical developmental milestones such as pinna detachment (unfolding of the external ear), incisor eruption (of lower and upper teeth), fur development, complete opening of both ears and complete opening of both eyes were expressed as the number of postnatal days required for the appearance of these milestones (42). These milestones were assessed every 24 h at 1 p.m. (after VF testing to reduce separation), until all pups of a given litter developed all milestones. The surface righting reflex was tested from P1 to P5, to assess reflex development and motor function. Pups were placed in a supine position on a flat surface and then released. The righting reflex is defined as the number of seconds required for a newborn pup to turn over on all four limbs, and was considered completed if the pup did so within 30 s (43).
To assess acute post-operative hypersensitivity, a plantar hind-paw incision surgery (or Brennan model for postoperative pain) was performed on all animals (44). Briefly, under isoflurane anesthesia (4–5% induction, 2% maintenance), a 1 cm longitudinal incision through the skin and fascia of the ipsilateral paw was made, followed by the elevation and incision (1–2 mm at midline) of the plantaris muscle. The skin was closed using two mattress sutures (5.0 Ethicon Ethylon, Somerville, New Jersey).
Mechanical sensitivity was assessed by determining the paw withdrawal thresholds (PWT) of the hind paws to calibrated Von Frey filaments. Briefly, animals were placed in a transparent box resting on an elevated mesh floor. After a 15-min acclimation period, a series of von Frey filaments (bending forces 1.202, 2.041, 3.63, 5.495, 8.511, 15.136, and 28.84 g; Stoelting, USA) were applied to the plantar surface of the hind paw for 5 s using the up-down method (45). Mechanical sensitivity was assessed weekly from 3 to 8 weeks of age, and every other day after adult re-injury (at 1, 3, 5, and 7 days post-incision). The animal order of behavioral testing was randomized, and researchers were blinded to treatment groups during behavioral testing and surgery throughout the entire experimental protocol.
The open field test was used to evaluate locomotor activity and anxiety-related behavior in adulthood (at 8 weeks of age) (23). Briefly, individual animals were placed into the center of a plexiglass square arena (100 × 100 × 40cm), and allowed to explore the environment for 20 min. The arena was thoroughly cleaned with 70% ethanol in between sessions. A camera mounted directly above the maze recorded the behavior of the animal, analyzed by the image tracking system (Noldus Ethovision XT software, Noldus Information Technology, the Netherlands). Variables included time spend in the center (%) and center crossings (number of entries from and to the center) for the first 5 min, and the total distance traveled (locomotor path in whole arena in cm) for a 20-min duration.
The Elevated Zero Maze (EZM) was used to assess anxiety-related behavior (23). Briefly: a black annular maze (100 cm diameter, 10 cm path width) was placed 70 cm above floor level, with four equal quadrants (two opposite quadrants are “closed” by black Perspex walls, two remaining quadrants are “open”). Animals were placed in an open arm facing one of the closed arms and allowed to explore the maze for 5 min. The arena was thoroughly cleaned with 70% ethanol between sessions. An infrared camera mounted directly above the maze recorded the behavior of the animal, analyzed by the image tracking system (Noldus Ethovision XT software, Noldus Information Technology, the Netherlands). Variables included time spend in open and closed arms, latency to enter each arm, and open arm entries. The time spend in the open arms as a percentage of total (corrected) trial time (= total trial duration—latency to enter first closed arm) was classified as anxiety-like behavior.
All data are presented as mean ± SEM and plotted using Graphpad Prism 9 (GraphPad Software, San Diego, USA). A P-value <0.05 was considered statistically significant. Mechanical force resulting in a 50% withdrawal frequency was assigned as the PWT and was calculated using a sigmoid curve fitting in Graphpad Prism 9. For the neonatal period, an area under the curve (AUC) analysis was performed. The AUC was calculated based on the PWTs for each group over the entire neonatal period (P 0–7) and statistically compared using a one-way ANOVA with Bonferroni post-hoc correction. Differences in PWT in the first neonatal week, mechanical sensitivity throughout development, and post-operative mechanical sensitivity were analyzed using a repeated measures analysis of variance (ANOVA) with Bonferroni post-hoc correction. Both within- and between-group analysis were run. A non-parametric Kruskal-Wallis tests was used to compare differences in developmental milestones between conditions. A two-way ANOVA was performed to compare the effects of condition and sex on anxiety-like behavioral measurements. For all analysis, the assumptions for statistical analysis were checked and met. For all behavioral analysis but the anxiety-like behavioral testing, the sex effect was tested and not significant, therefore data of both sexes was pooled to increase statistical power.
Pinnae detachment, lower and upper incisor eruption, fur development, ear opening and eye opening are unaffected by neonatal condition (p > 0.05; Figures 2C–H). In addition, no significant differences between conditions in their surface righting reflex [F (3, 46) = 0.0232; p = 0.9951; Figure 2I] or weight [F (3, 46) = 0.6406; p = 0.5928; Figures 2A,B] during the first postnatal week are observed.
Figure 2. Developmental milestones and weightduring the first postnatal week and during development from 3–8 week of age. (A) Weight during the first postnatal week significantly increases over time [F (2.541, 116.9) = 2628.0; p < 0.01], but is not significantly different between conditions [F (3, 46) = 0.6406; p = 0.5928]. (B) Weight increases significantly with time during development [F (1.105, 59.65) = 579.6; p < 0.01] but does not significantly differ between conditions [F (4, 54) = 0.3339; P = 0.8540]. Developmental milestones pinnea detachment [(C); H (3) = 5.550; p = 0.1357]; lower [(D); same time point in all groups, p > 0.999] and upper [(E); H (3) = 4.184; p = 0.2423] incision eruption; fur development [(F); same time point in all groups, p > 0.999]; ear opening [(G); H (3) = 2.992; p = 0.3928]; eye opening [(H); H (3) = 5.163; p = 0.1602] were not different between neonatal conditions. (I) The surface righting reflex developed in a similar manner in all groups [F (3, 46) = 0.08918; p = 0.9656], while postnatal day had a significant effect [F (2.362,101.6) = 15.27; p < 0.001]. NP, needle prick animals; NP+BUS, needle prick animals receiving buspirone; NP+OND, needle prick animals receiving ondansetron; PWT, paw withdrawal threshold; TC, tactile control animals; UD, undisturbed control animals. Data is presented as mean ± SEM, *P < 0.05 **P < 0.01.
During the first postnatal week ipsilateral mechanical sensitivity increases over time, but not equally for all conditions [interaction effect: F (93, 1426) = 4.798; p < 0.001]. Post hoc analysis revealed that repetitive NP results in significantly lower PWTs as compared to repetitive tactile stimulation from postnatal day 2 onwards (Figure 3A). Neonatal 5-HT1aR agonism using buspirone reverses the drop in PWTs after repetitive NP in NP+BUS animals from P4 onwards, whereas the drop in PWTs is still present after neonatal 5-HT3R antagonism using ondansetron (NP+OND; Figure 3A). The AUC analysis over the entire neonatal period reveals a significant decreased ipsilateral AUC in NP animals and NP+OND, as compared to both TC and NP+BUS animals [effect of condition: F (3, 46) = 10.79; p < 0.01; Figure 3B]. Contralateral mechanical sensitivity increases over time [effect of time: F (10.02, 460.7) = 62.68; p < 0.01] but is not significantly different between conditions [effect of condition: F (3, 46) = 0.9085; p = 0.444; Figure 3C]. Similarly, contralateral AUC are not significantly different between conditions [effect of condition: F (3, 46) = 0.9818; p = 0.4096; Figure 3D].
Figure 3. (A) Graphical representation of timeline of experiment. Mechanical sensitivity to von Frey filaments during first neonatal week. (B) Repetitive needle pricking (NP; n = 12) results in a decreased paw-withdrawal threshold (PWT) in the ipsilateral paw as compared to repetitive tactile stimulation (TC; n = 12) from postnatal day (P) 2 onwards, indicating the development of acute mechanical hypersensitivity in NP pups. Neonatal 5-HT1aR agonism (NP + BUS; n = 12), but not 5-HT3R antagonism (NP + OND; n = 14), is able to reverse the acute mechanical hypersensitivity after repetitive needle pricking [interaction effect: F (93, 1426) = 4.798; p < 0.001]. (C) The area under the curve (AUC) over the entire neonatal period is significantly different between neonatal conditions for the ipsilateral paw-withdrawal thresholds (PWT) [F (3, 46) = 10.79; p < 0.01]. NP as well as NP+OND animals show a decreased AUC as compared to TC animals, whereas NP+BUS reverses the decreased AUC back to TC control values. (D) Mechanical sensitivity of the contralateral paw increases over time all groups, but was not affected by neonatal condition [F (3, 46) = 0.9085; p = 0.444]. (E) The area under the curve analysis (AUC) over the entire neonatal period does not show significant differences in contralateral PWTs between conditions [F (3, 46) = 0.9818; p = 0.4096]. BS, baseline measurement; NP, needle pricking animals; NP+BUS, needle prick animals receiving buspirone; NP+OND, needle prick animals receiving ondansetron; PWT, paw withdrawal threshold; P (0–7), postnatal day 0–7; +1, +3, +5, measurement 1, 3, and 5 hours after needle prick or tactile stimulation; TC, tactile controls. Data is presented as mean ± SEM, NP vs. NP+BUS. *P < 0.05, **P < 0.01, NP vs. TC, #P < 0.05, ##P < 0.01.
During development from 3 to 8 weeks of age, PWT based on mechanical sensitivity to von Frey-filaments significantly increases over time for both paws [ipsilateral, F (3.048, 164.6) = 105.2, p < 0.01; contralateral, F (23.319, 179.2) = 81.69; p < 0.01]. The PWT increase over time seems dependent upon neonatal condition for the ipsilateral [interaction effect: F (20, 270) = 2.865, p < 0.01; Figure 4A] as well as the contralateral paw [interaction effect: F (20, 270) = 2.104, p < 0.01; Figure 4B]. NP+BUS animals show significantly lower ipsilateral PWTs compared to other groups at various time points: 3 weeks (NP+BUS 1.824 vs. NP 2.992; vs. NP+OND 3.411; vs. TC 3.189; vs. UD 3.576), 4 weeks (NP+BUS 3.103 vs. NP 3.626; vs. NP+OND 5.565; vs. TC 3.908; vs. UD 4.770) and 5 weeks (NP+BUS 3.876 vs. NP+OND 5.925). Contralateral PWTs of NP+BUS animals are significantly lower at 3 weeks (NP+BUS 1.787 vs. NP 3.103; vs. NP+OND 3.658, vs. UD 3.642) and 4 weeks (NP+BUS 2.876 vs. NP+OND 5.538, vs. UD 4.889). This effect ceases as NP+BUS animals grow older (>4–5 weeks of age). In addition, PWT of both hind-paws are significantly increased in NP and TC animals as compared to UD animals at 8 weeks of age (UD 6.682 vs. NP 9.622; vs. TC 10.90), suggesting differences in PWTs before incision. NP+OND animals do not show any differences in mechanical sensitivity from 3 to 8 weeks of age (p > 0.05).
Figure 4. Mechanical sensitivity to von Frey filaments throughout development. (A) Ipsilateral PWTs increase over time for all groups [F (3.048, 164.6) = 105.2, p < 0.01], but show a significant time*condition interaction [F (20,270) = 2.865, p < 0.01]. Posthoc analysis revealed a lower PWTs for the NP+BUS animals as compared to NP (at 3 and 4 weeks), NP+OND (at 3, 4, and 5 weeks), TC (at 3 weeks) and UD animals (at 3 and 4 weeks). NP and TC animals show higher PWTs at 8 weeks as compared to UD animals. (B) Contralateral PWTs increase over time for all groups [F (23.319, 179.2) = 81.69; p < 0.01], but show a significant time*condition effect [F (20, 270) = 2.104, p < 0.01]. Lower PWTs for the NP+BUS animals as compared to NP (at 3 weeks), NP+OND (at 3 and 4 weeks) and UD (at 3 and 4 weeks) are noted. Significantly higher PWTs are noted in NP and TC animals as compared to UD animals at 8 weeks. NP, needle prick animals; NP+BUS, needle prick animals receiving buspirone; NP+OND, needle prick animals receiving ondansetron; PWT, paw withdrawal threshold; TC, tactile control animals; UD, undisturbed control animals. Data is presented as mean ± SEM, *P < 0.05.
The effect of re-injury to the same dermatome on the PWT is measured in adulthood from 1-day post-incision (PI+1) to 7 days post-incision (PI+7). All animals develop acute hypersensitivity 1 and 3 days after incision, however a significant interaction effect of time*condition is observed [F (16, 216) = 2.915; p < 0.01; Figure 5A]. Within group analysis shows that UD PWTs return to pre-incision values by 5 days post-incision (Figure 5F), whereas NP and TC animals return to baseline values by 7 days post-incision (Figures 5B,E). This increased duration of mechanical hypersensitivity after re-injury is abolished in NP+OND (Figure 5C), but not NP+BUS animals (Figure 5D), indicated by a recovery to pre-incision values at PI+5 rather than PI+7.
Figure 5. Mechanical sensitivity after re-injury in adulthood. (A) All groups show mechanical hypersensitivity 1 and 3 days after incision in adulthood, but differences emerge between groups at 5 and 7 days post-incision. Return to pre-operative base-line PWT levels is noted in UD animals by 5 days postoperative (F), while PWTs of NP (B) and TC animals (E) are recovered to pre-operative baseline by 7 days postoperative. NP+OND (D), but not NP+BUS (C), abolishes this longer recovery time after incision. BL, baseline; NP, needle prick animals; NP+OND, needle prick animals receiving ondansetron; NP+BUS, needle prick animals receiving buspirone; PI, paw incision, +1, +3, +5; measurement 1, 3, and 5 days after paw incision; TC, tactile stimulation animals; UD, undisturbed control animals. Data is presented as mean ± SEM, *P < 0.05, **P < 0.01 as compared to their respective baseline.
A significant interaction between time and condition is noted in contralateral PWTs [F (16, 216) = 1.891; p = 0.0225], with UD animals showing lower contralateral PWTs at PI+7 as compared to NP, NP+BUS and NP+OND animals. The injury-induced change from baseline PWTs is not significantly affected by condition and only shows a significant effect of time [F (2.877, 155.3) = 5.099; p = 0.0025, data not shown].
In the OFT, no significant effect of condition [F (4, 49) = 1.972; p = 0.1135) or sex (F (1, 49) = 0.001; p = 0.9772; Figure 6B] on the percentage of time spend in the center of the arena is observed. In addition, the number of center crossings does not significantly differ between sex (F (1, 19) = 0.3304; p = 0.5681) or condition [F (4, 49) = 0.8757; p = 0.4853; Figure 6D]. Locomotor activity significantly differs between males and females [F (1, 49) = 21.20, p < 0.01] but is unaffected by neonatal condition [F (4, 49) = 1.177; p = 0.3325].
Figure 6. Neonatal conditions and adult anxiety behavior. (A) The percentage of time spend in the anxio-genic (open arm) region of the elevated zero maze (EZM) showed a significant difference between males and females [F (1, 49) = 19.75; p < 0.01]. No differences between conditions are observed in females, whereas UD males spend significant less time in the open arms as compared to all other neonatal conditions [F (4, 25) = 3.545; p = 0.0201]. (B) The percentage of time spend in the anxio-genic (center) region of the open field test (OFT) is not influenced by sex or condition. (C) The frequency of open arm entries is significantly affected by condition [F (4, 49) = 2.788; p = 0.0365] and sex [F (2, 49) = 16.62; p < 0.01]. UD animals exhibit significantly less open arm entries as compared to NP+BUS animals in males only. (D) The number of center crossings in the OFT is not affected by sex [F (1, 19) = 0.3304; p = 0.5681] or condition [F (4, 49) = 0.8757; p = 0.4853]. (E) Locomotor activity significantly differs between males and females [F (1, 49) = 21.20, p < 0.01] but is unaffected by condition [F (4, 49) = 1.177; p = 0.3325]. NP, needle prick animals; NP + OND, needle prick animals receiving ondansetron; NP+BUS, needle prick animals receiving buspirone; TC, tactile stimulation animals; UD, undisturbed control animals. Data is presented as mean ± SEM, *P < 0.05, **P < 0.01, ***P < 0.001.
In the EZM, the percentage of time spend in the open arms of the EZM significantly differs between males and females [effect of sex: F (1, 49) = 19.75; p < 0.01]. Females do not show differences between conditions in the time spend in the open arms of the EZM (effect of condition: F (4, 24) = 0.2669; p = 0.8963). In males, UD spend significant less time in the open arms of the EZM as compared to NP+BUS and NP+OND animals [effect of condition: F (4, 25) = 3.545; p = 0.0201]. The number of open arm entries in the EZM reveals a significant effect of sex [F (2, 49) = 16.62; p < 0.01] and condition [F (4, 49) = 2.788; p = 0.0365; Figure 6C]. NP+BUS animals exhibit significantly more open arm entries as compared to UD animals, in males only (NP + BUS 13.50 vs. UC 6.00; P = 0.0266).
Neonatal repetitive needle pricking leads to acute hypersensitivity and increased duration of post-operative recovery after re-injury in adulthood (12–17, 23). Here, we show that selective neonatal agonism of the 5-HT1aR using buspirone reduces the acute hypersensitivity associated with neonatal procedural pain, whereas antagonizing the 5-HT3R using ondansetron attenuates the long-term consequences of neonatal procedural pain. Moreover, modulation of both neonatal 5-HT1aR and 5-HT3R is associated with decreased adult anxiety. Altogether, our data suggests that targeted pharmacological treatment directed at the serotonergic 5-HT1a and 5-HT3 receptors in neonates is possible and may be of use in treatment of acute neonatal procedural pain and its long-term consequences.
The 5-HT3R and 5-HT1aR are important in a variety of neurodevelopment processes (24, 46). This implies that early pharmacological interventions with buspirone or ondansetron may affect normal development of rat pups. For this reason, we controlled for major developmental milestones and possible side-effects in our study. Our results show that major developmental milestones, reflex development, weight and locomotor behavior were unaffected by early life repetitive procedures or 5HT3R and 5-HT1aR modulation, in line with earlier studies (47–51).
The observation that neonatal 5-HT1aR agonism using buspirone reverses acute hypersensitivity after repetitive needle pricking in rat pups can be explained by its inhibitory role in spinal neurotransmission, previously shown in neonatal dorsal horn cells (25). Here we show for the first time that this system can be used in vivo to attenuate sensitivity after noxious stimuli in neonatal animals, suggesting the inhibitory effect of the 5HT1aR is present and functional during the neonatal period. This is important as descending serotonergic RVM-spinal dorsal horn have been reported to act in a facilitatory way in neonates (26). In addition, the anti-nociceptive effect of 5-HT1aR becomes more apparent from postnatal day 4 onwards, and this coincides with progressive increase in acute hypersensitivity with cumulative exposure to repetitive needle pricking (14–16, 23). 5-HT1aR in neonates inhibit nociceptive signaling through a reduced glutamate release from primary afferent terminals or by increasing the incidence of long-term depression induced inhibition of postsynaptic dorsal horn responses (28, 29, 31, 32). 5-HT1aR mediated binding, via the use of selective agonists, thereby restores neonatal procedural pain-evoked disruptions in the normal balance of excitation and inhibition in the spinal dorsal horn (17, 33–35). 5-HT1aR is expressed on 70-80% of RVM neurons in neonatal rats (52), and may also mediate its anti-nociceptive effects by blocking descending serotonergic facilitation from the RVM observed in younger animals (25).
Our data indicates that acute hypersensitivity in rat pups exposed to neonatal procedural pain is likely not mediated via the 5-HT3R, as the neonatal 5-HT3R antagonist ondansetron showed no behavioral effect in our study. This is remarkable, as descending serotonergic release from the RVM are thought to play an important role in 5-HT3R mediated facilitation of nociceptive signaling up to P21 in rats (26, 53–55). 5-HT3R expression in the dorsal horn has been reported to be stable from P7 up to adulthood in rodents (26), but expression patterns are yet to be documented for the first postnatal week. Activation of neonatal 5-HT3R in vitro is involved in facilitation of most dorsal horn cells, although the level of facilitation remains modest (25, 28, 29). 5-HT3R function in the neonate is dose-dependent, facilitating nociceptive signaling at low levels while inhibiting at higher concentrations. This dual effect may be explained by an autoreceptor mediated function, where the 5-HT3R regulates basal release of 5-HT, GABA and glycine in the neonatal dorsal horn (30). Moreover, non-noxious mechanical stimuli, as used in this study, are not facilitated by descending serotonergic projections at P8 (26). In infant rats at P21, ondansetron reduces dorsal horn activity in response to nociceptive, non-nociceptive stimuli and mechanical stimuli (26). Thus it is likely, that although 5-HT3R antagonism may induce direct changes in the physiological response to nociceptive and non-nociceptive stimuli in neonates, this does not translate to a behavioral effect of 5-HT3R antagonism on mechanical hypersensitivity after neonatal procedural pain based on von Frey testing as in our model.
Neonatal procedural pain has been associated with changes in pain sensitivity that persist beyond infancy, in both clinical and preclinical settings (4–7). Here, we show that 5-HT3R antagonism during neonatal procedural pain prevents the increased duration of neonatal injury-induced postoperative hypersensitivity in adulthood (Figure 4). This suggests that the underlying injury-induced alteration in nociceptive pathways are regulated, at least in part, by neonatal 5-HT3R activation. The 5-HT3R plays a prominent role in network formation and function of the neonatal brain (46). Within the nociceptive network, 5-HT3R are located on primary afferent fibers, glutamate terminals and projection neurons in the dorsal horn (56–59). Neonatal injury increases the excitatory drive onto spinal sensory neurons, resulting in enhanced glutamate signaling (35, 60, 61), a process known to be of pivotal importance in sensitization of the nociceptive network (62). Descending serotonergic projections facilitate noxious spinal processing via the 5-HT3R in the dorsal horn in early life (26). Hence, the blocked facilitation of 5-HT3R during early neonatal procedural pain is likely to underlie the effect of ondansetron at later stages.
In contrast to the acute anti-nociceptive effect, neonatal 5-HT1aR agonism did not affect extended post-operative recovery in adulthood in our study. Chronic treatment with selective 5-HT1aR agonists for 7 days, like in our study, desensitizes 5-HT1a auto-receptors, leading to impaired functioning of this receptor (63–65). Likewise, substance P that is released upon nociceptive C-fiber activation, likely to occur after repetitive needle pricking, also desensitizes 5-HT1aR and may impact receptor functioning (66, 67). Future studies should include anatomical as well as functional analysis to detect changes in 5-HT1aR functioning after neonatal targeting. During development, we observed a temporary increase in mechanical sensitivity in NP animals when exposed to neonatal 5-HT1aR agonist buspirone that ceased with increasing age (>5 weeks). Repetitive activation of the 5-HT1aR from P0 to P7 may result in long-term depression (28), leading to altered synaptic plasticity in the spinal nociceptive circuit which consequently may alter basal nociceptive behavior.
Serotonin signaling also regulates the normal development of emotional behavior, including anxiety (24). Hence, sensitive windows in development through which serotonergic signaling is altered may differentially encode anxiety behaviors. Neonatal 5-HT1aR agonism decreased adult male anxiety in the EZM as compared to undisturbed males, but did not affect anxiety-related behavior in the OFT (Figure 6). 5-HT1aR have been reported to be central players in controlling anxiety (36), and thus interference with early postnatal functioning of the 5-HT1aR (buspirone) may affect normal anxiety behavior (36, 37). Neonatal 5-HT3R antagonism using ondansetron also shows some anxiogenic properties in males in the EZM, albeit small. Previous studies on the role of 5-HT3R antagonism related to anxiety remain contradictory (38, 68), Of note, our results demonstrate the absence of any difference in adult state and trait anxiety levels between TC and NP animals and neonatal 5-HT1aR or 5-HT3R modulation, suggesting receptor modulation does have an additive effect on anxiety. Interestingly as related to anxiety-related behavior as measured using the EZM, a significant difference between sex in anxiety is noted that was not observed or assessed in earlier studies using a similar neonatal model (20, 22, 23, 50). Lower levels of anxiety in females compared to males, predominantly observed in the undisturbed animals in our study, is not uncommon in rodent models that use both sexes (69).
The current study is not without limitations. First, the specificity of buspirone and ondansetron as pharmacological modulators of the 5-HT1aR and 5-HT3R, respectively, is of importance. Buspirone has high affinity for 5-HT1aR but also increases dopamine levels via D2 inactivation as well as noradrenaline levels via α2R in the frontal cortex (70, 71). Therefore, buspirone-mediated effects in our study may not be uniquely related to 5-HT1aR functioning, but can also be (partially) the result of D2R or α2R binding. Ondansetron is a potent, highly selective antagonist of the 5-HT3R (72). Although it shows some affinity for 5-HT1b, 5-HT4, opioid and α1-adrenergic receptors, it has a selectivity of 1,000:1 toward 5-HT3R (40). Hence, the effects of ondansetron as observed in this study are most likely exclusively mediated by 5-HT3R binding. Next, ondansetron and buspirone were administered systemically and this limits the interpretation of location-specific effects in the spinal cord nociceptive network. Future studies should include local intrathecal administration to further specify the involvement of spinal 5-HT1aR and 5-HT3R in the observed effects. Lastly, baseline differences between animals exposed to repetitive needle pricking or tactile stimulation and undisturbed animals at 8 weeks of age, prior to re-incision were observed Nevertheless and based on within-group analysis any possible effects related to the differences in baseline value differences on post-operative mechanical sensitivity can be excluded (14–16).
Our data shows that neonatal targeted pharmacological modulation of the 5-HT1aR and 5-HT3R attenuates the acute and long-term effects associated with neonatal procedural pain. These results may form the fundament of a new mechanism-based therapeutic venue in treatment of procedural pain in human neonates.
The raw data supporting the conclusions of this article will be made available by the authors, without undue reservation.
The animal study was reviewed and approved by Committee for Experiments on Animals, Maastricht University, Maastricht, Netherlands (DEC 2017-017).
AK, EJ, JP, DT, and NH conceived the research. AK, EJ, and NH designed and conceptualized the research and contributed to its structure and content. AK performed the research, analyzed the data, and drafted the manuscript and figures. EJ provided the resources for this research. All authors commented on previous versions of the manuscript, critically revised, and quality assessed the manuscript. All authors have read and approved the final version of the manuscript.
AK received financial support for this research provided by the Pain Knowledge Centre from Maastricht and an institutional grant from University Maastricht, School of Mental Health and Neuroscience.
The authors declare that the research was conducted in the absence of any commercial or financial relationships that could be construed as a potential conflict of interest.
All claims expressed in this article are solely those of the authors and do not necessarily represent those of their affiliated organizations, or those of the publisher, the editors and the reviewers. Any product that may be evaluated in this article, or claim that may be made by its manufacturer, is not guaranteed or endorsed by the publisher.
The authors would like to thank Wouter Gerrits (Department of Translational Neuroscience, Maastricht University) for providing technical support during experiments and surgeries.
1. Verriotis M, Chang P, Fitzgerald M, Fabrizi L. The development of the nociceptive brain. Neuroscience. (2016) 338:207–19. doi: 10.1016/j.neuroscience.2016.07.026
2. Fitzgerald M. The development of nociceptive circuits. Nat Rev Neurosci. (2005) 6:507–20. doi: 10.1038/nrn1701
3. Van den Hoogen NJ, Patijn J, Tibboel D, Joosten E. Neonatal plasticity of the nociceptive system: mechanisms, effects, and treatment of repetitive painful procedures during NICU admittance. Curr Pharm Des. (2017) 23:5902–10. doi: 10.2174/1381612823666170921130320
4. Beggs S, Torsney C, Drew LJ, Fitzgerald M. The postnatal reorganization of primary afferent input and dorsal horn cell receptive fields in the rat spinal cord is an activity-dependent process. Eur J Neurosci. (2002) 16:1249–58. doi: 10.1046/j.1460-9568.2002.02185.x
5. Granmo M, Petersson P, Schouenborg J. Action-based body maps in the spinal cord emerge from a transitory floating organization. J Neurosci. (2008) 28:5494–503. doi: 10.1523/JNEUROSCI.0651-08.2008
6. Waldenström A, Thelin J, Thimansson E, Levinsson A, Schouenborg J. Developmental learning in a pain-related system: evidence for a cross-modality mechanism. J Neurosci. (2003) 23:7719–25. doi: 10.1523/JNEUROSCI.23-20-07719.2003
7. Koch SC, Fitzgerald M. Activity-dependent development of tactile and nociceptive spinal cord circuits. Ann N Y Acad Sci. (2013) 1279:97–102. doi: 10.1111/nyas.12033
8. Walker SM, editor. Long-term effects of neonatal pain. Semin Fetal Neonatal Med. (2019) 24:101005. doi: 10.1016/j.siny.2019.04.005
9. Williams MD, Lascelles BDX. Early neonatal pain—a review of clinical and experimental implications on painful conditions later in life. Front Pediatr. (2020) 8:30. doi: 10.3389/fped.2020.00030
10. Walker SM. Translational studies identify long-term impact of prior neonatal pain experience. Pain. (2017) 158:S29–42. doi: 10.1097/j.pain.0000000000000784
11. Beggs S. Long-term consequences of neonatal injury. Can J Psychiatry. (2015) 60:176–80. doi: 10.1177/070674371506000404
12. Knaepen L, Patijn J, Tibboel D, Joosten E. Sex differences in inflammatory mechanical hypersensitivity in later life of rats exposed to repetitive needle pricking as neonates. Neurosci Lett. (2012) 516:285–9. doi: 10.1016/j.neulet.2012.04.012
13. Knaepen L, Patijn J, van Kleef M, Mulder M, Tibboel D, Joosten EA. Neonatal repetitive needle pricking: plasticity of the spinal nociceptive circuit and extended postoperative pain in later life. Dev Neurobiol. (2013) 73:85–97. doi: 10.1002/dneu.22047
14. van den Hoogen NJ, Patijn J, Tibboel D, Joosten E. Repetitive noxious stimuli during early development affect acute and long-term mechanical sensitivity in rats. Pediatr Res. (2020) 87:26–31. doi: 10.1038/s41390-019-0420-x
15. Van den Hoogen NJ, Tibboel D, Honig W, Hermes D, Patijn J, Joosten E. Neonatal paracetamol treatment reduces long-term nociceptive behaviour after neonatal procedural pain in rats. Eur J Pain. (2016) 20:1309–18. doi: 10.1002/ejp.855
16. van den Hoogen NJ, de Geus TJ, Patijn J, Tibboel D, Joosten EA. Methadone effectively attenuates acute and long-term consequences of neonatal repetitive procedural pain in a rat model. Pediatr Res. (2021) 89:1681–6. doi: 10.1038/s41390-020-01353-x
17. van den Hoogen NJ, Patijn J, Tibboel D, Joosten BA, Fitzgerald M, Kwok CH. Repeated touch and needle-prick stimulation in the neonatal period increases the baseline mechanical sensitivity and postinjury hypersensitivity of adult spinal sensory neurons. Pain. (2018) 159:1166. doi: 10.1097/j.pain.0000000000001201
18. Anand K, Coskun V, Thrivikraman K, Nemeroff C, Plotsky P. Long-term behavioral effects of repetitive pain in neonatal rat pups. Physiol Behav. (1999) 66:627–37. doi: 10.1016/S0031-9384(98)00338-2
19. Chen M, Xia D, Min C, Zhao X, Chen Y, Liu L, et al. Neonatal repetitive pain in rats leads to impaired spatial learning and dysregulated hypothalamic-pituitary-adrenal axis function in later life. Sci Rep. (2016) 6:39159. doi: 10.1038/srep39159
20. Page GG, Blakely WP, Kim M. The impact of early repeated pain experiences on stress responsiveness and emotionality at maturity in rats. Brain Behav Immun. (2005) 19:78–87. doi: 10.1016/j.bbi.2004.05.002
21. Schellinck HM, Stanford L, Darrah M. Repetitive acute pain in infancy increases anxiety but does not alter spatial learning ability in juvenile mice. Behav Brain Res. (2003) 142:157–65. doi: 10.1016/S0166-4328(02)00406-0
22. Zuke JT, Rice M, Rudlong J, Paquin T, Russo E, Burman MA. The effects of acute neonatal pain on expression of corticotropin-releasing hormone and juvenile anxiety in a rodent model. Eneuro. (2019) 6:ENEURO.0162-19.2019. doi: 10.1523/ENEURO.0162-19.2019
23. de Kort AR, Joosten EA, Patijn J, Tibboel D, van den Hoogen NJ. Neonatal procedural pain affects state, but not trait anxiety behavior in adult rats. Dev Psychobiol. (2021). doi: 10.1002/dev.22210
24. Suri D, Teixeira CM, Cagliostro MKC, Mahadevia D, Ansorge MS. Monoamine-sensitive developmental periods impacting adult emotional and cognitive behaviors. Neuropsychopharmacology. (2015) 40:88–112. doi: 10.1038/npp.2014.231
25. de Kort AR, Joosten EA, Patijn J, Tibboel D, van den Hoogen NJ. The development of descending serotonergic modulation of the spinal nociceptive network: a life span perspective. Pediatr Res. (2021) 1–9. doi: 10.1038/s41390-021-01638-9
26. Schwaller F, Kanellopoulos A, Fitzgerald M. The developmental emergence of differential brainstem serotonergic control of the sensory spinal cord. Sci Rep. (2017) 7:1–12. doi: 10.1038/s41598-017-02509-2
27. van den Hoogen NJ, de Kort AR, Allegaert KM, Joosten EA, Simons SH, Tibboel D, etal., editors. Developmental neurobiology as a guide for pharmacological management of pain in neonates. Semin Fetal Neonatal Med. (2019) 24:101012. doi: 10.1016/j.siny.2019.05.004
28. Garraway SM, Hochman S. Serotonin increases the incidence of primary afferent-evoked long-term depression in rat deep dorsal horn neurons. J Neurophysiol. (2001) 85:1864–72. doi: 10.1152/jn.2001.85.5.1864
29. Garraway SM, Hochman S. Pharmacological characterization of serotonin receptor subtypes modulating primary afferent input to deep dorsal horn neurons in the neonatal rat. Br J Pharmacol. (2001) 132:1789–98. doi: 10.1038/sj.bjp.0703983
30. Iwasaki T, Otsuguro K-i, Kobayashi T, Ohta T, Ito S. Endogenously released 5-HT inhibits A and C fiber-evoked synaptic transmission in the rat spinal cord by the facilitation of GABA/glycine and 5-HT release via 5-HT2A and 5-HT3 receptors. Eur J Pharmacol. (2013) 702:149–57. doi: 10.1016/j.ejphar.2013.01.058
31. Wallis DI, Wu J, Wang XC. Is 5-hydroxytryptamine mediating descending inhibition in the neonatal rat spinal cord through different receptor subtypes? Eur J Pharmacol. (1993) 250:371–7. doi: 10.1016/0014-2999(93)90023-B
32. Li P, Zhuo M. Silent glutamatergic synapses and nociception in mammalian spinal cord. Nature. (1998) 393:695–8. doi: 10.1038/31496
33. Li J, Baccei ML. Neonatal injury alters sensory input and synaptic plasticity in GABAergic interneurons of the adult mouse dorsal horn. J Neurosci. (2019) 39:7815–25. doi: 10.1523/JNEUROSCI.0509-19.2019
34. Li J, Blankenship ML, Baccei ML. Deficits in glycinergic inhibition within adult spinal nociceptive circuits after neonatal tissue damage. Pain. (2013) 154:1129–39. doi: 10.1016/j.pain.2013.03.030
35. Li J, Kritzer E, Craig PE, Baccei ML. Aberrant synaptic integration in adult lamina I projection neurons following neonatal tissue damage. J Neurosci. (2015) 35:2438–51. doi: 10.1523/JNEUROSCI.3585-14.2015
36. Gross C, Zhuang X, Stark K, Ramboz S, Oosting R, Kirby L, et al. Serotonin 1A receptor acts during development to establish normal anxiety-like behaviour in the adult. Nature. (2002) 416:396–400. doi: 10.1038/416396a
37. Vinkers CH, Oosting RS, van Bogaert MJ, Olivier B, Groenink L. Early-life blockade of 5-HT1A receptors alters adult anxiety behavior and benzodiazepine sensitivity. Biol Psychiatry. (2010) 67:309–16. doi: 10.1016/j.biopsych.2009.08.013
38. de Oliveira Citó MdC, da Silva FCC, Silva MIG, Moura BA, Macêdo DS, Woods DJ, et al. Reversal of cocaine withdrawal-induced anxiety by ondansetron, buspirone and propranolol. Behav Brain Res. (2012) 231:116–23. doi: 10.1016/j.bbr.2012.01.056
39. Loane C, Politis M. Buspirone: what is it all about? Brain Res. (2012) 1461:111–8. doi: 10.1016/j.brainres.2012.04.032
40. Butler A, Hill J, Ireland S, Jordan C, Tyers M. Pharmacological properties of GR38032F, a novel antagonist at 5-HT3 receptors. Br J Pharmacol. (1988) 94:397–412. doi: 10.1111/j.1476-5381.1988.tb11542.x
41. Warfield AE, Prather JF, Todd WD. Systems and Circuits Linking Chronic Pain and Circadian Rhythms. Front Neurosci. (2021) 15:705173. doi: 10.3389/fnins.2021.705173
42. Soujanya M, Reddy PR, Reddy PS. Perinatal exposure of biochanin-A induced abnormalities in offspring of rats. J Infertil Reprod Biol. (2014) 2:115–23. Available online at: https://dormaj.org/index.php/JIRB/article/view/362
43. de Castro VL, Destefani CR, Diniz C, Poli P. Evaluation of neurodevelopmental effects on rats exposed prenatally to sulfentrazone. Neurotoxicology. (2007) 28:1249–59. doi: 10.1016/j.neuro.2007.06.001
44. Brennan TJ, Vandermeulen EP, Gebhart G. Characterization of a rat model of incisional pain. Pain. (1996) 64:493–502. doi: 10.1016/0304-3959(95)01441-1
45. Chaplan SR, Bach F, Pogrel J, Chung J, Yaksh T. Quantitative assessment of tactile allodynia in the rat paw. J Neurosci Methods. (1994) 53:55–63. doi: 10.1016/0165-0270(94)90144-9
46. Engel M, Smidt MP, Van Hooft JA. The serotonin 5-HT3 receptor: a novel neurodevelopmental target. Front Cell Neurosci. (2013) 7:76. doi: 10.3389/fncel.2013.00076
47. Budylin T, Guariglia SR, Duran LI, Behring BM, Shaikh Z, Neuwirth LS, et al. Ultrasonic vocalization sex differences in 5-HT1A-R deficient mouse pups: predictive phenotypes associated with later-life anxiety-like behaviors. Behav Brain Res. (2019) 373:112062. doi: 10.1016/j.bbr.2019.112062
48. Djavadian R, Wielkopolska E, Turlejski K. Postnatal treatment with NAN-190 but not with 5-HT1A receptor agonists retards growth of the rat brain. Int J Dev Neurosci. (2005) 23:485–93. doi: 10.1016/j.ijdevneu.2005.02.001
49. Olivier B, Molewijk H, Van der Heyden J, Van Oorschot R, Ronken E, Mos J, et al. Ultrasonic vocalizations in rat pups: effects of serotonergic ligands. Neurosci Biobehav Rev. (1998) 23:215–27. doi: 10.1016/S0149-7634(98)00022-0
50. Ranger M, Tremblay S, Chau CM, Holsti L, Grunau RE, Goldowitz D. Adverse behavioral changes in adult mice following neonatal repeated exposure to pain and sucrose. Front Psychol. (2019) 9:2394. doi: 10.3389/fpsyg.2018.02394
51. Khatri N, Simpson KL, Lin RC, Paul IA. Lasting neurobehavioral abnormalities in rats after neonatal activation of serotonin 1A and 1B receptors: possible mechanisms for serotonin dysfunction in autistic spectrum disorders. Psychopharmacology. (2014) 231:1191–200. doi: 10.1007/s00213-013-3242-2
52. Liu Q, Wong-Riley MT. Postnatal changes in the expressions of serotonin 1A, 1B, and 2A receptors in ten brain stem nuclei of the rat: implication for a sensitive period. Neuroscience. (2010) 165:61–78. doi: 10.1016/j.neuroscience.2009.09.078
53. Hathway G, Koch S, Low L, Fitzgerald M. The changing balance of brainstem–spinal cord modulation of pain processing over the first weeks of rat postnatal life. J Physiol. (2009) 587:2927–35. doi: 10.1113/jphysiol.2008.168013
54. Schwaller F, Kwok C, Fitzgerald M. Postnatal maturation of the spinal-bulbo-spinal loop: brainstem control of spinal nociception is independent of sensory input in neonatal rats. Pain. (2016) 157:677. doi: 10.1097/j.pain.0000000000000420
55. Koch SC, Fitzgerald M. The selectivity of rostroventral medulla descending control of spinal sensory inputs shifts postnatally from A fibre to C fibre evoked activity. J Physiol. (2014) 592:1535–44. doi: 10.1113/jphysiol.2013.267518
56. Conte D, Legg E, McCourt A, Silajdzic E, Nagy G, Maxwell D. Transmitter content, origins and connections of axons in the spinal cord that possess the serotonin (5-hydroxytryptamine) 3 receptor. Neuroscience. (2005) 134:165–73. doi: 10.1016/j.neuroscience.2005.02.013
57. Kidd EJ, Laporte A, Langlois X, Fattaccini C-M, Doyen C, Lombard M, et al. 5-HT3 receptors in the rat central nervous system are mainly located on nerve fibres and terminals. Brain Res. (1993) 612:289–98. doi: 10.1016/0006-8993(93)91674-H
58. Laporte A, Koscielniak T, Ponchant M, Verge D, Hamon M, Gozlan H. Quantitative autoradiographic mapping of 5-HT3 receptors in the rat CNS using [125I] iodo-zacopride and [3H] zacopride as radioligands. Synapse. (1992) 10:271–81. doi: 10.1002/syn.890100402
59. Lee S, Hjerling-Leffler J, Zagha E, Fishell G, Rudy B. The largest group of superficial neocortical GABAergic interneurons expresses ionotropic serotonin receptors. J Neurosci. (2010) 30:16796–808. doi: 10.1523/JNEUROSCI.1869-10.2010
60. Li J, Baccei ML. Neonatal tissue damage facilitates nociceptive synaptic input to the developing superficial dorsal horn via NGF-dependent mechanisms. Pain. (2011) 152:1846–55. doi: 10.1016/j.pain.2011.04.001
61. Li J, Walker SM, Fitzgerald M, Baccei ML. Activity-dependent modulation of glutamatergic signaling in the developing rat dorsal horn by early tissue injury. J Neurophysiol. (2009) 102:2208–19. doi: 10.1152/jn.00520.2009
62. Ji R-R, Kohno T, Moore KA, Woolf CJ. Central sensitization and LTP: do pain and memory share similar mechanisms? Trends Neurosci. (2003) 26:696–705. doi: 10.1016/j.tins.2003.09.017
63. Assié MB, Lomenech H, Ravailhe V, Faucillon V, Newman-Tancredi A. Rapid desensitization of somatodendritic 5-HT1A receptors by chronic administration of the high-efficacy 5-HT1A agonist, F13714: a microdialysis study in the rat. Br J Pharmacol. (2006) 149:170–8. doi: 10.1038/sj.bjp.0706859
64. Kreiss DS, Lucki I. Chronic administration of the 5-HT1A receptor agonist 8-OH-DPAT differentially desensitizes 5-HT1A autoreceptors of the dorsal and median raphe nuclei. Synapse. (1997) 25:107–16. doi: 10.1002/(SICI)1098-2396(199702)25:2<107::AID-SYN1>3.0.CO;2-G
65. Hensler JG. Regulation of 5-HT1A receptor function in brain following agonist or antidepressant administration. Life Sci. (2003) 72:1665–82. doi: 10.1016/S0024-3205(02)02482-7
66. Santarelli L, Gobbi G, Debs PC, Sibille EL, Blier P, Hen R, et al. Genetic and pharmacological disruption of neurokinin 1 receptor function decreases anxiety-related behaviors and increases serotonergic function. Proc Nat Acad Sci. (2001) 98:1912–7. doi: 10.1073/pnas.98.4.1912
67. Guiard BP, Froger N, Hamon M, Gardier AM, Lanfumey L. Sustained pharmacological blockade of NK1 substance P receptors causes functional desensitization of dorsal raphe 5-HT1A autoreceptors in mice. J Neurochem. (2005) 95:1713–23. doi: 10.1111/j.1471-4159.2005.03488.x
68. Lopes LT, Canto-de-Souza L, Baptista-de-Souza D, de Souza RR, Nunes-de-Souza RL, Canto-de-Souza A. The interplay between 5-HT2C and 5-HT3A receptors in the dorsal periaqueductal gray mediates anxiety-like behavior in mice. Behav Brain Res. (2021) 417:113588. doi: 10.1016/j.bbr.2021.113588
69. Donner NC, Lowry CA. Sex differences in anxiety and emotional behavior. Pflügers Arch. (2013) 465:601–26. doi: 10.1007/s00424-013-1271-7
70. Silverstone PH, Lalies MD, Hudson A. Quetiapine and buspirone both elevate cortical levels of noradrenaline and dopamine in vivo, but do not have synergistic effects. Front Psychiatry. (2012) 3:82. doi: 10.3389/fpsyt.2012.00082
71. Gobert A, Rivet J-M, Cistarelli L, Melon C, Millan M. Buspirone modulates basal and fluoxetine-stimulated dialysate levels of dopamine, noradrenaline and serotonin in the frontal cortex of freely moving rats: activation of serotonin1A receptors and blockade of α2-adrenergic receptors underlie its actions. Neuroscience. (1999) 93:1251–62. doi: 10.1016/S0306-4522(99)00211-0
Keywords: neonatal pain, procedural pain, treatment, serotonin, ondansetron, buspirone
Citation: de Kort AR, Joosten EA, Patijn J, Tibboel D and van den Hoogen NJ (2022) Selective Targeting of Serotonin 5-HT1a and 5-HT3 Receptors Attenuates Acute and Long-Term Hypersensitivity Associated With Neonatal Procedural Pain. Front. Pain Res. 3:872587. doi: 10.3389/fpain.2022.872587
Received: 09 February 2022; Accepted: 30 March 2022;
Published: 27 April 2022.
Edited by:
Dusica Bajic, Boston Children's Hospital and Harvard Medical School, United StatesReviewed by:
Mariano Soiza-Reilly, CONICET Institute of Physiology, Molecular Biology and Neurosciences (IFIBYNE), ArgentinaCopyright © 2022 de Kort, Joosten, Patijn, Tibboel and van den Hoogen. This is an open-access article distributed under the terms of the Creative Commons Attribution License (CC BY). The use, distribution or reproduction in other forums is permitted, provided the original author(s) and the copyright owner(s) are credited and that the original publication in this journal is cited, in accordance with accepted academic practice. No use, distribution or reproduction is permitted which does not comply with these terms.
*Correspondence: Nynke J. van den Hoogen, bnlua2UudmFuZGVuaG9vZ2VuQHVjYWxnYXJ5LmNh
Disclaimer: All claims expressed in this article are solely those of the authors and do not necessarily represent those of their affiliated organizations, or those of the publisher, the editors and the reviewers. Any product that may be evaluated in this article or claim that may be made by its manufacturer is not guaranteed or endorsed by the publisher.
Research integrity at Frontiers
Learn more about the work of our research integrity team to safeguard the quality of each article we publish.