- 1Division of Neonatology, Department of Pediatrics, University of Utah, Salt Lake City, UT, United States
- 2Division of Neurology, Department of Pediatrics, University of Utah, Salt Lake City, UT, United States
- 3Intermountain Healthcare, Provo, UT, United States
- 4Division of Pediatric Clinical Pharmacology, University of Utah, Salt Lake City, UT, United States
- 5Division of Pediatric Clinical Pharmacology and Division of Critical Care, University of Utah, Salt Lake City, UT, United States
Background: Neonatal hypoxia-ischemia encephalopathy (HIE) is the leading cause of neonatal death and poor neurodevelopmental outcomes worldwide. Therapeutic hypothermia (TH), while beneficial, still leaves many HIE treated infants with lifelong disabilities. Furthermore, infants undergoing TH often require treatment for pain and agitation which may lead to further brain injury. For instance, morphine use in animal models has been shown to induce neuronal apoptosis. Dexmedetomidine is a potent α2-adrenergic receptor agonist that may be a better alternative to morphine for newborns with HIE treated with TH. Dexmedetomidine provides sedation, analgesia, and prevents shivering but does not suppress ventilation. Importantly, there is increasing evidence that dexmedetomidine has neuroprotective properties. Even though there are limited data on pharmacokinetics (PK), safety and efficacy of dexmedetomidine in infants with HIE, it has been increasingly administered in many centers.
Objectives: To review the current approach to treatment of pain, sedation and shivering in infants with HIE undergoing TH, and to describe a new phase II safety and pharmacokinetics randomized controlled trial that proposes the use of dexmedetomidine vs. morphine in this population.
Methods: This article presents an overview of the current management of pain and sedation in critically ill infants diagnosed with HIE and undergoing TH for 72 h. The article describes the design and methodology of a randomized, controlled, unmasked multicenter trial of dexmedetomidine vs. morphine administration enrolling 50 (25 per arm) neonates ≥36 weeks of gestation with moderate or severe HIE undergoing TH and that require pain/sedation treatment.
Results and Conclusions: Dexmedetomidine may be a better alternative to morphine for the treatment of pain and sedation in newborns with HIE treated with TH. There is increasing evidence that dexmedetomidine has neuroprotective properties in several preclinical studies of injury models including ischemia-reperfusion, inflammation, and traumatic brain injury as well as adult clinical trials of brain trauma. The Dexmedetomidine Use in Infants undergoing Cooling due to Neonatal Encephalopathy (DICE) trial will evaluate whether administration of dexmedetomidine vs. morphine is safe, establish dexmedetomidine optimal dosing by collecting opportunistic PK data, and obtain preliminary neurodevelopmental data to inform a large Phase III efficacy trial with long term neurodevelopment impairment as the primary outcome.
Introduction
Neonatal hypoxia-ischemia encephalopathy (HIE) is the leading cause of neonatal death and poor neurodevelopmental outcomes worldwide (1–3). Up to 12,000 infants are affected each year in the US (2). Therapeutic Hypothermia (TH) initiated within 6 h of life has become standard of care in developed countries and has been shown to mitigate brain damage (4–6). However, current data from the more recent cooling trials demonstrate that up to 30% of treated infants either died or had moderate to severe neurologic disabilities including long-term motor and cognitive dysfunction (7). Disabilities include cerebral palsy, intellectual disability, epilepsy and visual impairment among others adverse outcomes. No additional therapies have yet proven to be efficacious in further reducing brain injury and impairment for these high risk infants.
Newborns with moderate-to-severe HIE may also present with multiorgan failure and may develop cardiovascular instability, renal and liver insufficiency, seizures and respiratory failure requiring mechanical ventilation. Due to their critical condition in the Neonatal Intensive Care Unit (NICU) they commonly receive opiate drugs such as morphine for pain and sedation management and to prevent shivering (8, 9).
The present review will evaluate the current approach to treatment of pain, sedation and shivering in infants with HIE undergoing TH, and will describe a new phase II safety and pharmacokinetics (PK) randomized controlled trial that proposes the use of dexmedetomidine vs. morphine in this population.
Adequate Pain and Sedation Management May Improve Outcomes
In neonates with HIE who are treated with TH, emerging data suggest that appropriate treatment of pain and agitation may improve outcomes (10–12). Infants with HIE are subjected to the stresses of an adverse perinatal event, resuscitation, hypothermia itself and painful procedures. These events may alter nociceptive pathways during a critical developmental period and adversely affect neuropsychological outcomes (13). Thoresen et al reported that TH was not protective after HIE in unsedated piglets, but TH reduced the severity of brain injury in piglets receiving appropriate sedation (14). However, in most trials of TH for neonatal HIE the administration of sedation drugs was provider-driven and there have not been any large randomized controlled trial addressing this important question (8, 9).
Morphine Is the Most Common Sedative Used in Neonates With HIE During Therapeutic Hypothermia
A recent study including 125 neonatal intensive care units (NICUs) demonstrated that up to 64% of neonates with HIE and treated with TH received opioids (15). Unpublished data from the ongoing High Dose Erythropoetin for Asphyxia and Encephalopathy (HEAL) trial showed that 328/501 (65%) infants with HIE received morphine in the first 4 days of life at 17 participating NICUs (NCT02811263).
However, the use of morphine for pain and sedation has raised concerns for safety including depressed ventilation, hypotension, gastrointestinal dysmotility and importantly, potential adverse effects on neurodevelopmental outcomes (10–12). For instance, morphine has been shown to increase apoptosis in both human microglial cells and neuronal like cells of neonatal rats (16–18). Furthermore, animal studies support long-term negative effects on behavior and brain function following the administration of morphine to neonatal rats. Pups that received morphine from day 1–7 of life showed retarded motor development and as adults had impaired motor coordination, altered gait, and altered patterns of activity in an open field (19).
In preterm neonates, the use of intermittent boluses of morphine for analgesia increased the rate of the composite primary outcome of neonatal death, severe intraventricular hemorrhage (IVH), and periventricular leukomalacia (NEOPAIN trial) (20). In a separate randomized control trial, continuous infusion of morphine in preterm infants decreased the incidence of IVH but did not affect neurodevelopmental outcomes (21). Even though the preterm infant is a different population, the concern for deleterious effects of morphine infusions on the developing brain are still applicable to the near term and term population.
Dexmedetomidine Is a Potent α2-Adrenergic Receptor Agonist That May Be a Better Alternative to Morphine for Newborns With HIE Treated With TH
Dexmedetomidine provides sedation, analgesia, and prevents shivering but does not suppress ventilation (22, 23). Importantly, dexmedetomidine demonstrates neuroprotective properties in several injury models including ischemia-reperfusion, anesthesia, inflammation and traumatic brain injury (24–29).
Dexmedetomidine Is Neuroprotective in Preclinical Studies
Dexmedetomidine is a highly protein-bound drug that can readily cross the blood–brain barrier as shown in pre-clinical animal studies (30, 31). Neonatal animal models of HIE have shown that dexmedetomidine acts as potent neuroprotector via stimulation of the α-2A adrenoreceptors (32).
Exposure to dexmedetomidine following perinatal HIE reduced cortical and white matter lesion sizes and was associated with improved neurologic functional deficit (33, 34). Other possible mechanisms of dexmedetomidine neuroprotection in HIE include reducing oxidative stress and inflammation in hippocampus (32). Similarly, following controlled cortical impact, adult mice that received 3 days of dexmedetomidine therapy had decreased brain edema, inflammation, secondary blood-brain barrier damage and apoptosis (30). Following subarachnoid hemorrhage in rats, dexmedetomidine reduced brain edema and apoptosis, and improved neurological outcome scores (29).
HIE pathophysiology in the newborn shares several of these apoptotic and inflammatory pathways, therefore dexmedetomidine may have an important role for neuroprotection following HIE in neonates.
Dexmedetomidine Is Neuroprotective in Adult Clinical Studies
In human studies, a recent meta-analysis assessed the neuroprotective effects of dexmedetomidine on ischemic brain injury in adults. Nineteen RCTs including 879 patients were analyzed and showed that compared with placebo, dexmedetomidine reduced the release of inflammatory mediators and neuroendocrine hormones and maintained intracranial homoeostasis, alleviating ischemic brain injury (35).
Dexmedetomidine Use in a Newborn Piglet Model of HIE Undergoing Hypothermia
The PK of dexmedetomidine administered to 9 newborn piglets following HIE was reported in a de-escalation dose study (loading dose: 1 μg/kg; maintenance infusion: from 10 down to 0.6 μg/kg/h). Clearance was reduced by 32.7% at a temperature of 33.5°C and by 55.8% following hypoxia-ischemia. Importantly, high plasma levels of dexmedetomidine (>1 μg/l) were associated with cardiovascular complications (36).
Dexmedetomidine PK in Neonates With HIE Receiving TH
Recently McAdams et al. evaluated PK and safety of dexmedetomidine in a phase I, single-center, open-label study in 7 neonates ≥ 36 weeks GA diagnosed with moderate-to-severe HIE. Infants received a continuous dexmedetomidine infusion during TH and the 6 h rewarming period. In cooled infants with HIE, clearance was either comparable or lower, distribution volume was larger, and elimination half-life was longer compared to corresponding estimates previously reported for normothermic newborns without HIE as shown in Table 1. Plasma concentrations in cooled newborns with HIE rose at a slower rate in the initial hours of infusion while similar steady-state levels were achieved. There were no adverse events (AEs) associated with dexmedetomidine treatment. The authors concluded that dexmedetomidine appeared safe for neonates with HIE at infusion doses up to 0.4 μg/kg/h. However, to overcome the initial lag in rise of plasma dexmedetomidine concentration a loading dose strategy is strongly suggested and therefore included in the present study (37).
The Safety and Potential Benefits of Dexmedetomidine in Infants With HIE and Multiorgan Failure Needs Further Evaluation
In neonates, side effects of dexmedetomidine appear to be dose dependent and are mainly restricted to hemodynamic alterations including hypertension, bradycardia, and hypotension secondary to pre- and postsynaptic α2-receptor activation, which causes vasoconstriction, vasodilatation, and reflex bradycardia (39–41). Of importance to the present proposal is that dexmedetomidine can affect thermoregulation by affecting vasoconstriction and non-shivering thermogenesis by lipolysis, both key mechanisms present in the newborn infant. Thus, neonates with HIE undergoing TH and receiving dexmedetomidine may be vulnerable to abnormal temperature regulation (42, 43).
The short-term safety of dexmedetomidine used for sedation in 19 term neonates undergoing TH for HIE showed that it did not impact heart rate, mean arterial blood pressures, or cerebral saturations. There was no increase in extubation failures or need for vasopressors (44).
The impact of dexmedetomidine vs. morphine on cerebral blood flow and clinical outcomes was also evaluated in 205 term neonates with HIE. Patients in the dexmedetomidine cohort (n = 46) were extubated sooner, required lower dose of inotropes, had decreased seizure burden and lower incidence of abnormal neuroimaging when compared to the control group (n = 159) (45). Other benefits included shorter time to full oral feedings following TH and decreased need for NG at time of discharge (44). In a retrospective, observational cohort study including neonates with HIE undergoing TH, Cosnahan et al showed that pain scores, hemodynamics, and time to enteral feeds were similar between dexmedetomidine (N = 35) and morphine patients (N = 35). Dexmedetomidine patients received more breakthrough morphine but less cumulative morphine, and morphine patients on invasive ventilation required increased ventilatory support.
The authors concluded that dexmedetomidine seemed effective and safe for sedation and analgesia during TH since It reduced total opioid usage without increased incidence of AEs (46). In summary, data from clinical studies using dexmedetomidine in newborn infants is emerging and indicates that dexmedetomidine seems to be a safe sedative agent with possible neuroprotective effects.
Dexmedetomidine Is Increasingly Used in Infants Undergoing TH for HIE but Rigorous Safety and PK Data Are Lacking
Even though there are limited data on PK, safety and efficacy of dexmedetomidine in infants with HIE, it has been increasingly used in many NICUs for pain and sedation during TH (38, 44, 46, 47). PK is likely different in this population compared to other infants due to both (1) altered physiology associated with critical illness and (2) TH (48). Furthermore, an immature or dysfunctional blood–brain barrier due to HIE may cause higher cerebrospinal fluid concentrations increasing sedative and analgesic effects (49).
DICE Study Design
The Dexmedetomidine Use in Infants undergoing Cooling due to Neonatal Encephalopathy (DICE trial) will be a Phase II multicenter, unmasked, randomized, safety and PK trial. Dr. Baserga (PI) holds the FDA IND number 152829. The study is registered in Clinical Trials.Gov NCT04772222.
Hypothesis
Our central hypothesis is that dexmedetomidine administered as sedative and analgesic therapy to infants ≥36 weeks gestational age with moderate to severe HIE undergoing TH and requiring analgesia and sedation will be safe and will be associated with improved short and long-term outcomes.
To test this hypothesis, we propose a Phase II multicenter, unmasked, randomized, safety and PK trial with the following Specific Aims:
Aim 1: Examine safety measures in infants receiving dex-medetomidine compared to infants receiving morphine. Need for sedation and pain management will be assessed using the Neonatal Pain, Agitation, and Sedation Scale (N-PASS) scores. Safety of dexmedetomidine will be evaluated during the first 4 days of life by documenting adverse events (hypotension, hypertension, bradycardia, cardiac arrhythmias, hypothermia, acute renal failure, liver failure, and seizures) outside of normal range for the study population.
Aim 2: Determine dexmedetomidine PK in this population of newborns with HIE during treatment with TH. Two opportunistic PK samples (at time of routine laboratories) and a PRN PK sample any time there is an adverse event will be obtained for measurement of Dexmedetomidine plasma concentrations.
Aim 3: Determine the correlation between dexme-detomidine vs. morphine use with short-term outcomes (clinical outcomes during initial hospitalization) and on neurodevelopmental outcomes. Battery of tests will include: (1) Generalized Movement Assessment (cerebral palsy assessment) performed 1 week after sedation is weaned off and at 3–4 months of life; (2) Hammersmith Infant Neurological Exam test performed at 3–4 and at 6–9 months of life; (3) Test of Infant Motor Performance performed at 3–4 months of life; and (4) Peabody Developmental Motor Skills test performed at 6–9 months of life. The Ages and Stages Questionnaires will also be completed by parents at the 6–9 months of life visit.
Population
The DICE trial will enroll 50 infants (n = 25/arm) ≥ 36 weeks gestational age with HIE and requiring pain treatment and/or sedation. Infants will be randomized to receive either dexmedetomidine or morphine. Figure 1 depicts the study timeline and interventions.
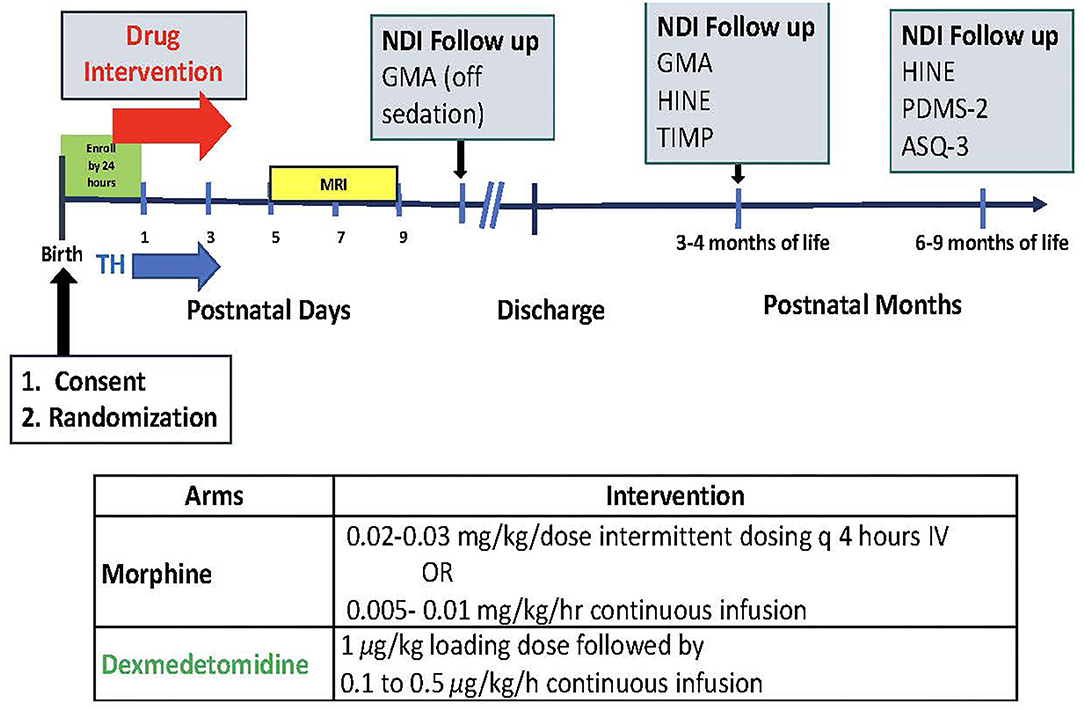
Figure 1. Study timeline and interventions. TH, brain MRI, neurodevelopmental impairment (NDI) follow up with GMA (Generalized Movement Assessment); HINE (Hammersmith Infant Neurological Exam); TIMP (Test of Infant Motor Performance); PDMS-2 (Peabody Developmental Motor Skills); ASQ-3 (The Ages and Stages Questionnaires).
Inclusion/exclusion Criteria: Eligible neonates need to be ≥36 weeks' GA diagnosed with moderate-to-severe HIE and treated with TH (target temperature 33.5°C) for a planned duration of 72 h. To be considered for the study, infant needs to require analgesia, sedation and/or treatment to prevent shivering during TH as assessed by the N-PASS scores and a modified Bedside Shivering Assessment Scale (50, 51).
Exclusion criteria include known chromosomal anomalies; cyanotic congenital heart defects and other major congenital anomalies, as well as redirection of care being considered (not expected to survive >24 h).
Parental consent, randomization and initial treatment need to occur within 24 h of birth and as close to the initiation of TH as possible. The rationale for early intervention to treat pain and provide sedation, as well as potentially provide adjunctive neuroprotection, is based on the notion that in the early phases following HIE, dexmedetomidine could complement hypothermia through diverse mechanisms of action including anti-inflammatory and anti-apoptotic properties.
The infrastructure for this proposal consists of 5 large Level III-IV NICUs in Utah with 4,000 admissions a year. These centers have a long tradition in clinical research and participate in the Neonatal Research Network (NRN): University of Utah Hospital; Intermountain Medical Center; Primary Children's Hospital; McKay-Dee Hospital; and Utah Valley Hospital. Cumulatively, a total of 50–60 infants receive TH for HIE at these 5 participating NICUs each year. Our historical consent rate for interventional RCTs is ~60%. Including all 5 centers would allow us to complete the study in 2 years. Institutional Board Review has been obtained in 2 of the 5 centers and is in process at Intermountain Health System (3 remainder hospitals).
Intervention
Infants will be randomized to receive either dexmedetomidine (1 μg/kg for loading dose followed by 0.1–0.5 μg/kg/h continuous infusion) or morphine (0.02–0.03 mg/kg/dose intermittent dosing q 4 h IV or as continuous infusion dose of 0.005–0.01 mg/kg/h) (37) using a REDCap centralized randomization model.
Initial study drug dose will be given as soon as possible after randomization and no later than 24 h of age. Initiation and adjustment of the dexmedetomidine and morphine doses (i.e., increasing, decreasing or holding the dose) during TH will be standardized using the N-PASS scores prior to and during study drug exposure as shown in Figure 2 to determine sedation and analgesia effectiveness of both drugs (50, 52).
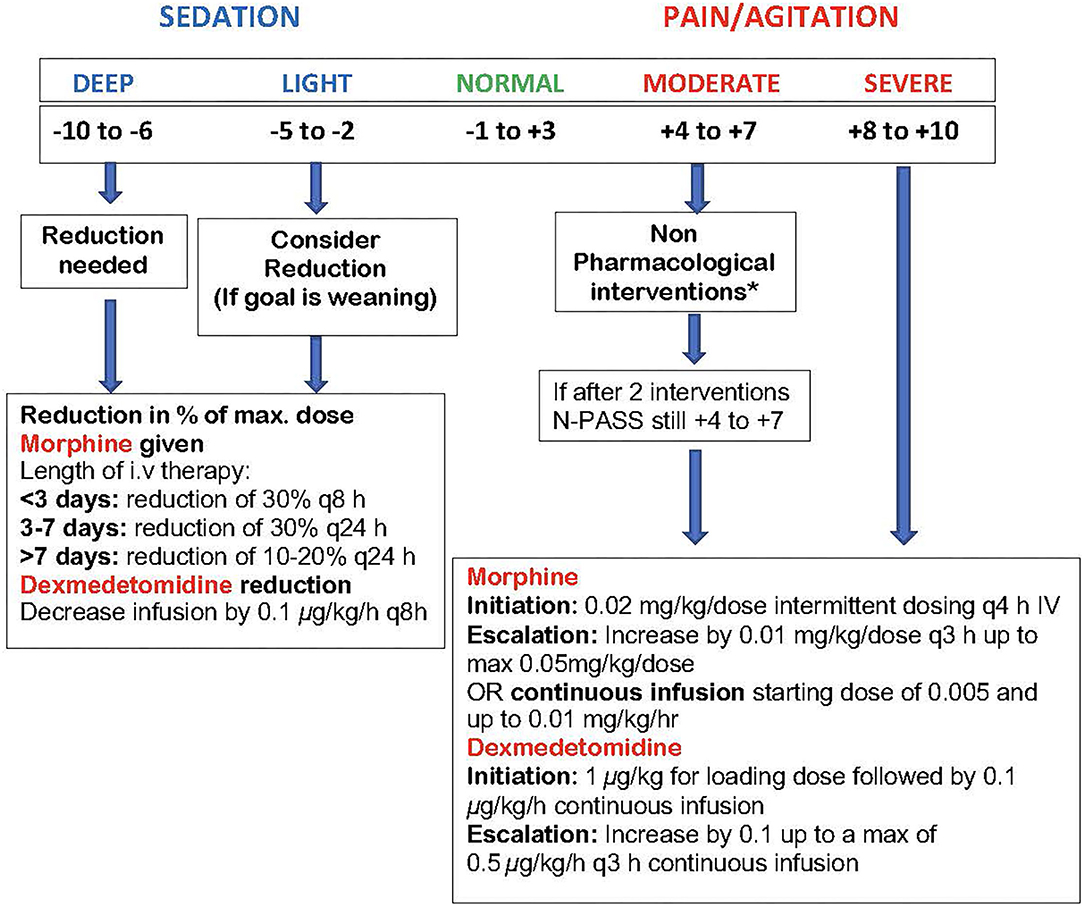
Figure 2. DICE trial N-PASS Assessment for Pain, Agitation and Sedation. Performed every 3 h and after 30 min post escalation or de-escalation of medication. The N-PASS tool uses 5 assessment criteria (crying/irritability, behavior/state, facial expression, extremities/tone, and vital signs); each criterion is graded 0, −1, or −2 for sedation and 0, 1, or 2 for pain/agitation. · N-PASS score >3 (significant pain or agitation) = supplemental sedation or analgesia therapy considered. · N-PASS score >8 = initiate/escalate therapy. · N-PASS score <-2 (light sedation) = consider therapy weaning. · N-PASS score <-5 = weaning warranted. *Non-Pharmacological Interventions (maybe limited during hypothermia): Nonnutritive sucking, swaddling, change of position, Kangaroo care.
Outcomes
The primary outcome of this study will be safety. Secondary outcomes will include PK and short and long-term clinical outcomes including clinical course during the initial hospitalization (incidence of shivering, respiratory support, time to full oral feedings, seizure burden, etc.) as well as neurodevelopmental follow up (up to 9 months of age).
Safety
We will determine safety in infants receiving dexmedetomidine compared with infants receiving morphine during the first 4 days of life by documenting AEs such as hypotension, hypertension, acute renal failure, liver failure, poor cardiac function, cardiac arrhythmias, respiratory failure, hypothermia and seizures outside of normal range for the study population (9, 53). This population includes critically ill infants that have morbidities associated with HIE that can overlap or potentiate some of the side effects of TH, morphine and dexmedetomidine. We will therefore compare morbidities between the 2 interventional groups (morphine vs. Dexmedetomidine) and with historical cohorts of infants that underwent cooling in large RCTs (4–6, 9, 53). We will consider AEs those that are outside of normal range expected for the study population.
Severe adverse events (SAEs) will be defined as any event that results in death, a life-threatening event, a persistent or significant disability that prolongs inpatient hospitalization. SAEs will be reported until 30 days of life or hospital discharge, whichever occurs first.
Criteria for withholding or stopping the study drug will be the occurrence of an AE requiring intervention: severe bradycardia (heart rate <60 sustained for >30 min); serious cardiac arrythmias (atrial fibrillation); severe hypotension (<30 mm Hg MBP) or hypertension (sustained SBP >95th centile) requiring treatment; and severe hypothermia (esophageal temperature <31°C). An independent Data Safety Monitoring Committee (DSMC) composed by members qualified by training and experience to monitor the progress of the investigation will monitor study progress, safety and efficacy. An interim safety analysis will be performed after 10 infants are enrolled. The trial will be temporarily suspended for the initial safety review and as deemed necessary by the DSMC for any subsequent safety concerns.
Pharmacokinetics: We will determine dexmedetomidine PK in this population of neonates with HIE during treatment with TH and during rewarming periods (Day 1–4). To avoid excessive phlebotomy losses, a sparse sampling strategy will be employed. Two opportunistic blood samples (0.1 mL) will be obtained for each participant at the time of routine laboratories. Additional PK samples will be collected any time there is an AE that is at least possibly related to dexmedetomidine.
The rich sampling strategy employed in adult PK studies is rarely feasible in infant PK studies due to barriers to informed consent and limited blood volume. A sparse sampling strategy when combined with modeling approaches such as population PK and physiologically-based PK have successfully characterized the PK in infants and children and this approach is recommended by regulatory agencies for pediatric studies (54–61).
Plasma will be frozen at −80°C until shipped to the Bioanalytical Core at Children's Hospital of Philadelphia for analysis. Dexmedetomidine concentrations in plasma will be quantified using an existing, validated HPLC-MS/MS assay. Accuracy and precision are within the FDA bioanalytical assay validation criteria (±15%). This method was employed in a dexmedetomidine PK study in neonates after cardiac surgery (62). Dexmedetomidine plasma PK data will be analyzed with a non-linear mixed effects modeling approach using NONMEM (version 7.4, Icon Solutions, Ellicott City, MD, USA).
Clinical and Neurodevelopmental Outcomes
To determine the extent to which dexmedetomidine is associated with improved short-term clinical outcomes during initial hospitalization the following data will be collected:
1. Cardiovascular: Severe Hypotension (Sustained Mean Blood Pressure <30 mm Hg MAP) or Hypertension (Sustained Systolic Blood Pressure >95th Centile), Bradycardia/Tachycardia, Need for Inotropes, Need for Inhaled Nitric Oxide, and Echocardiogram Results.
2. Respiratory Support: Need for Nasal Cannula, CPAP, Invasive Mechanical Ventilation, Time to Extubation, Extubation Failure. Ventilatory Settings and Oxygen Requirements Will be Recorded.
3. Shivering: Will be Assessed Every 3 h With Other Vital Signs by the Bedside Nurse Using an Adapted Version of the Bedside Shivering Assessment Scale (51).
4. Other: Time to Full PO Feedings; Need for Gastric Tube/NG Feedings at Discharge; Seizure Burden (Total Duration of Seizures in first 24 h of Life); Neurologic Exam (at 7 Days of Life or at Discharge); Magnetic Resonance Imaging, Head Ultrasound; EEG Results.
Neurodevelopmental Follow up
All HIE infants that receive TH automatically qualify for follow up by the University of Utah Neonatal Follow Up Program and Pediatric Neurology. The following well established tests will be used: (1) Generalized Movement Assessment (GMA) (cerebral palsy assessment) performed 1 week after sedation is weaned off and at 3–4 months of life; (2) Hammersmith Infant Neurological Exam (HINE) test performed at 3–4 and at 6–9 months of life; (3) Test of Infant Motor Performance (TIMP) performed at 3–4 months of life; and (4) Peabody Developmental Motor Skills (PDMS-2) test performed at 6–9 months of life. These validated tests are core components of the neurodevelopmental assessment for early diagnosis of cerebral palsy (63–65). In the present proposal, these tools will be used as markers of neurodevelopmental safety following the use of dexmedetomidine. The Ages and Stages Questionnaires (ASQ-3) will be completed by parents at the 6–9 month visit to screen infants in areas of communication, gross and fine motor, and personal-social (66). This data will be used to power a Phase III dexmedetomidine neuroprotective efficacy trial.
Sample Size and Statistical Analyses
For the sample size calculation, 50 subjects (25 in each arm) would yield 80% power to compare dexmedetomidine relative to morphine for key safety measures using tolerance limits of 0.5 SDs for quantitative measures of organ function. The safety markers and clinical meaningful changes that we have identified to calculate the current power statement include the incidence of hypotension, bradycardia, persistent pulmonary hypertension (PPHN), renal adverse events, and hepatic dysfunction in both groups. The incidence of hypotension that required used of inotropes (40%), significant bradycardia (5%), PPHN (15%), renal adverse events (41%) and liver dysfunction (32%) has been reported in previous cooling cohorts and will be used as baseline (53).
This sample size is also considered adequate to evaluate clinical (hospitalization) and neurodevelopmental impairment as secondary outcomes. Lastly, the sample size of 50 is adequate to evaluate feasibility for a potential phase III trial that will be powered for long term outcomes.
For the analysis of safety effect, we will use an intention-to treat strategy. To compare AEs and SAEs counts between treatment groups a Poisson regression model with robust SEs adjusting for the severity of encephalopathy (moderate vs. severe) will be used. To calculate the effect of dexmedetomidine vs. morphine on developmental outcome measures, we will use 95% confidence intervals, and corresponding P-values (significance, P < 0.05) using a generalized linear model with robust SEs, and appropriately adjusting for patient covariates using an all subsets regression model with corrected Akaike information criterion (AICc). For summary statistics, we will compare categorical variables (GA, birthweight, gender) between randomized treatment groups by using a χ2 test, or Fisher's exact test when the expected count of any category is ≤5. To compare baseline continuous variables between treatment groups, a two-sided t-test will be used.
Limitations
We expect that a proportion of the DICE subjects will be transferred from referring institutions, challenging the time-sensitive consent process. For successful recruitment (window to obtain informed parental consent is 24 h) each site will follow a dedicated screening process to alert the research team of new eligible admissions. Our sites have significant experience in approaching families of potential subjects in a timely fashion as most RCTs require approaching parents within hours of admission to the NICU (67, 68). For instance, in the “Darbepoetin administration to neonates undergoing cooling for encephalopathy: a safety and pharmacokinetic trial (DANCE)” our randomization time was a mean of 8 h of life in 30 infants demonstrating feasibility of such studies in this sick population (68).
Lost to follow up (about 10% in our 5 centers) is another potential difficulty. To address this issue, we have a dedicated follow up research coordinator that maintains periodic contact with the participating families. Another advantage is that HIE/cooled infants automatically qualify for follow up in the University Developmental Assessment Clinics as well as with Pediatric Neurology at the same age as the NDI follow up is planned for the DICE study (3–4 months and 6–9 months of life).
Although not ideal, the design of the DICE trial will be unblinded due to the fact that masking a loading dose followed by continuous Dexmedetomidine infusion vs. Morphine to be given as intermittent dosing q 4 h IV or (less likely) as a continuous infusion would be challenging. Many centers use morphine as a PRN bolus drug so allocating all babies in the morphine arm to receive a continuous infusion would not be generalizable. For infusion therapies blinding can translate into a cumbersome and costly setup (69). Lastly, this is a Phase 2 safety and PK trial where blinding perhaps is not as crucial as in a phase III efficacy study in which masking key personnel in charge of assessing outcomes (bedside nurse obtaining pain scores, neurodevelopmental assessors, etc.) would be key.
Conclusions/Discussion
HIE remains an important cause of death and adverse neurological outcome. Because of the vulnerability of the brain following HIE, avoiding the use of potentially harmful drugs for analgesia and sedation such as morphine and replacing it with a potentially safer drug such as dexmedetomidine may further reduce brain injury. However, inappropriate dosing places these infants at risk for therapeutic failure and toxicity (49). Further research to determine safe and effective therapies for the treatment of pain and stress during hypothermia is essential to guide the development of clinical treatment protocols. The DICE trial will hopefully advance the knowledge about the safety and PK of dexmedetomidine in critically ill neonates with HIE undergoing TH. The PK and outcome data obtained from this trial will be used to inform larger Phase III trials with long-term neurodevelopment assessments at 2 years of age and school age as the primary outcome.
Data Availability Statement
The original contributions presented in the study are included in the article/Supplementary Material, further inquiries can be directed to the corresponding author/s.
Author Contributions
KW, MS, BO, and TD contributed to the study design and manuscript preparation. AB and TB contributed to the statistical study design and manuscript preparation. MB contributed to the study conception and design, and manuscript preparation. All authors read and approved the final manuscript.
Conflict of Interest
The authors declare that the research was conducted in the absence of any commercial or financial relationships that could be construed as a potential conflict of interest.
Publisher's Note
All claims expressed in this article are solely those of the authors and do not necessarily represent those of their affiliated organizations, or those of the publisher, the editors and the reviewers. Any product that may be evaluated in this article, or claim that may be made by its manufacturer, is not guaranteed or endorsed by the publisher.
References
1. Black RE, Cousens S, Johnson HL, Lawn JE, Rudan I, Bassani DG, et al. Global, regional, and national causes of child mortality in 2008: a systematic analysis. Lancet. (2010) 375:1969–95. doi: 10.1016/S0140-6736(10)60549-1
2. Kurinczuk JJ, White-Koning M, Badawi N. Epidemiology of neonatal encephalopathy and hypoxic-ischaemic encephalopathy. Early Hum Dev. (2010) 86:329. doi: 10.1016/j.earlhumdev.2010.05.010
3. Ariff S, Lee AC, Lawn J, Bhutta ZA. Global burden, epidemiologic trends, and prevention of intrapartum-related deaths in low-resource settings. Clin Perinatol. (2016) 43:593. doi: 10.1016/j.clp.2016.05.001
4. Shankaran S, Laptook AR, Ehrenkranz RA, Tyson JE, McDonald SA, Donovan EF, et al. Whole-body hypothermia for neonates with hypoxic-ischemic encephalopathy. N Engl J Med. (2005) 353:1574–84. doi: 10.1056/NEJMcps050929
5. Azzopardi DV, Strohm B, Edwards AD, Dyet L, Halliday HL, Juszczak E, et al. Moderate hypothermia to treat perinatal asphyxial encephalopathy. N Engl J Med. (2009) 361:1349–58. doi: 10.1056/NEJMoa0900854
6. Edwards AD, Brocklehurst P, Gunn AJ, Halliday H, Juszczak E, Levene M, et al. Neurological outcomes at 18 months of age after moderate hypothermia for perinatal hypoxic ischaemic encephalopathy: synthesis and meta-analysis of trial data. BMJ. (2010) 340:c363. doi: 10.1136/bmj.c363
7. Shankaran S, Laptook AR, Pappas A, McDonald SA, Das A, Tyson JE, et al. Effect of depth and duration of cooling on death or disability at age 18 months among neonates with hypoxic-ischemic encephalopathy: a randomized clinical trial. JAMA. (2017) 318:57. doi: 10.1001/jama.2017.7218
8. Natarajan G, Shankaran S, Laptook AR, McDonald SA, Pappas A, Hintz SR, et al. Association between sedation-analgesia and neurodevelopment outcomes in neonatal hypoxic-ischemic encephalopathy. J Perinatol. (2018) 38:1060–060 doi: 10.1038/s41372-018-0126-7
9. Bonifacio SL, McDonald SA, Chock VY, Wusthoff CJ, Hintz SR, Laptook AR, et al. Differences in patient characteristics and care practices between two trials of therapeutic hypothermia. Pediatr Res. (2019) 85:1008–008 doi: 10.1038/s41390-019-0371-2
10. Attarian S, Tran LC, Moore A, Stanton G, Meyer E, Moore RP. The neurodevelopmental impact of neonatal morphine administration. Brain Sci. (2014) 4:321ciS, Tran LC,3390/brainsci4020321
11. Molina PE. Opioids and opiates: analgesia with cardiovascular, haemodynamic and immune implications in critical illness. J Intern Med. (2006) 259:138Medpioids and1111/j.1365-2796.2005.01569.x
12. Wassink G, Lear CA, Gunn KC, Dean JM, Bennet L, Gunn AJ. Analgesics, sedatives, anticonvulsant drugs, and the cooled brain. Semin Fetal Neonatal Med. (2015) 20:109tal Neonatal 1016/j.siny.2014.10.003
13. Williams MD, Lascelles BDX. Early neonatal pain-a review of clinical and experimental implications on painful conditions later in life. Front Pediatr. (2020) 8:30. doi: 10.3389/fped.2020.00030
14. Thoresen M, Satas S, Loberg EM, Whitelaw A, Acolet D, Lindgren C, et al. Twenty-four hours of mild hypothermia in unsedated newborn pigs starting after a severe global hypoxic-ischemic insult is not neuroprotective. Pediatr Res. (2001) 50:405Respothermia 1203/00006450-200109000-00017
15. Berube MW, Lemmon ME, Pizoli CE, Bidegain M, Tolia VN, Cotten CM, et al. Opioid and benzodiazepine use during therapeutic hypothermia in encephalopathic neonates. J Perinatol. (2020) 40:79–88. doi: 10.1038/s41372-019-0533-4
16. Hu S, Sheng WS, Lokensgard JR, Peterson PK. Morphine induces apoptosis of human microglia and neurons. Neuropharmacology. (2002) 42:829rmacologyoken1016/S0028-3908(02)00030-8
17. Bajic D, Commons KG, Soriano SG. Morphine-enhanced apoptosis in selective brain regions of neonatal rats. Int J Dev Neurosci. (2013) 31:258v NeurosciG, 1016/j.ijdevneu.2013.02.009
18. Juul SE, Beyer RP, Bammler TK, Farin FM, Gleason CA. Effects of neonatal stress and morphine on murine hippocampal gene expression. Pediatr Res. (2011) 69:285Resyer RP, Ba1203/PDR.0b013e31820bd165
19. Handelmann GE, Dow-Edwards D. Modulation of brain development by morphine: effects on central motor systems and behavior. Peptides. (1985) 6:29pl5esnn GE, D1016/0196-9781(85)90131-7
20. Anand KJ, Hall RW, Desai N, Shephard B, Bergqvist LL, Young TE, et al. Effects of morphine analgesia in ventilated preterm neonates: primary outcomes from the NEOPAIN randomised trial. Lancet. (2004) 363:1673–6733 doi: 10.1016/S0140-6736(04)16251-X
21. Simons SH, van Dijk M, van Lingen RA, Roofthooft D, Duivenvoorden HJ, Jongeneel N, et al. Routine morphine infusion in preterm newborns who received ventilatory support: a randomized controlled trial. JAMA. (2003) 290:2419–4190 doi: 10.1001/jama.290.18.2419
22. Lewis SR, Nicholson A, Smith AF, Alderson P. Alpha-2 adrenergic agonists for the prevention of shivering following general anaesthesia. Cochrane Database Syst Rev. (2015) 10:CD011107. doi: 10.1002/14651858.CD011107.pub2
23. Lam F, Bhutta AT, Tobias JD, Gossett JM, Morales L, Gupta P. Hemodynamic effects of dexmedetomidine in critically ill neonates and infants with heart disease. Pediatr Cardiol. (2012) 33:1069–069 doi: 10.1007/s00246-012-0227-6
24. Chen Y, Miao L, Yao Y, Wu W, Wu X, Gong C, et al. Dexmedetomidine ameliorate CLP-induced rat intestinal injury via inhibition of inflammation. Mediators Inflamm. (2015) 2015:918361. doi: 10.1155/2015/918361
25. Schoeler M, Loetscher PD, Rossaint R, Fahlenkamp AV, Eberhardt G, Rex S, et al. Dexmedetomidine is neuroprotective in an in vitro model for traumatic brain injury. BMC Neurol. (2012) 12:20. doi: 10.1186/1471-2377-12-20
26. Sanders RD, Sun P, Patel S, Li M, Maze M, Ma D. Dexmedetomidine provides cortical neuroprotection: impact on anaesthetic-induced neuroapoptosis in the rat developing brain. Acta Anaesthesiol Scand. (2010) 54:710esthesiol Sc1111/j.1399-6576.2009.02177.x
27. Tufek A, Tokgoz O, Aliosmanoglu I, Alabalik U, Evliyaoglu O, Ciftci T, et al. The protective effects of dexmedetomidine on the liver and remote organs against hepatic ischemia reperfusion injury in rats. Int J Surg. (2013) 11:96urgective effe1016/j.ijsu.2012.12.003
28. Perez-Zoghbi JF, Zhu W, Grafe MR, Brambrink AM. Dexmedetomidine-mediated neuroprotection against sevoflurane-induced neurotoxicity extends to several brain regions in neonatal rats. Br J Anaesth. (2017) 119:506sth JF, Zhu W1093/bja/aex222
29. Yin D, Zhou S, Xu X, Gao W, Li F, Ma Y, et al. Dexmedetomidine attenuated early brain injury in rats with subarachnoid haemorrhage by suppressing the inflammatory response: the TLR4/NF-kappaB pathway and the NLRP3 inflammasome may be involved in the mechanism. Brain Res. (2018) 1698:19818Ressome m1016/j.brainres.2018.05.040
30. Wang D, Xu X, Wu YG Lyu L, Zhou ZW, Zhang JN. Dexmedetomidine attenuates traumatic brain injury: action pathway and mechanisms. Neural Regen Res. (2018) 13:819egen Res YG L4103/1673-5374.232529
31. Wang C, Liu S, Han C, Yu M, Hu Y, Liu C. Effect and placental transfer of dexmedetomidine during caesarean section under epidural anaesthesia. J Int Med Res. (2017) 45:964d Resal anaes1177/0300060517698330
32. Chen X, Chen D, Li Q, Wu S, Pan J, Liao Y, et al. Dexmedetomidine alleviates hypoxia-induced synaptic loss and cognitive impairment via inhibition of microglial NOX2 activation in the hippocampus of neonatal rats. Oxid Med Cell Longev. (2021) 2021:6643171. doi: 10.1155/2021/6643171
33. Laudenbach V, Mantz J, Lagercrantz H, Desmonts JM, Evrard P, Gressens P. Effects of alpha(2)-adrenoceptor agonists on perinatal excitotoxic brain injury: comparison of clonidine and dexmedetomidine. Anesthesiology. (2002) 96:134iologyr agoni1097/00000542-200201000-00026
34. Ma D, Hossain M, Rajakumaraswamy N, Arshad M, Sanders RD, Franks NP, et al. Dexmedetomidine produces its neuroprotective effect via the alpha 2A-adrenoceptor subtype. Eur J Pharmacol. (2004) 502:87armacolbtype.1016/j.ejphar.2004.08.044
35. Jiang L, Hu M, Lu Y, Cao Y, Chang Y, Dai Z. The protective effects of dexmedetomidine on ischemic brain injury: A meta-analysis. J Clin Anesth. (2017) 40:25Anesths. u Y,1016/j.jclinane.2017.04.003
36. Ezzati M, Broad K, Kawano G, Faulkner S, Hassell J, Fleiss B, et al. Pharmacokinetics of dexmedetomidine combined with therapeutic hypothermia in a piglet asphyxia model. Acta Anaesthesiol Scand. (2014) 58:733esthesiol Sca1111/aas.12318
37. McAdams RM, Pak D, Lalovic B, Phillips B, Shen DD. Dexmedetomidine pharmacokinetics in neonates with hypoxic-ischemic encephalopathy receiving hypothermia. Anesthesiol Res Pract. (2020) 2020:2582965. doi: 10.1155/2020/2582965
38. Chrysostomou C, Schulman SR, Herrera Castellanos M, Cofer BE, Mitra S, da Rocha MG, et al. A phase II/III, multicenter, safety, efficacy, and pharmacokinetic study of dexmedetomidine in preterm and term neonates. J Pediatr. (2014) 164:276–82 e1-3. doi: 10.1016/j.jpeds.2013.10.002
39. MacMillan LB, Hein L, Smith MS, Piascik MT, Limbird LE. Central hypotensive effects of the alpha2a-adrenergic receptor subtype. Science. (1996) 273:801ic receptor 1126/science.273.5276.801
40. Ebert TJ, Hall JE, Barney JA, Uhrich TD, Colinco MD. The effects of increasing plasma concentrations of dexmedetomidine in humans. Anesthesiology. (2000) 93:382iology JE, Ba1097/00000542-200008000-00016
41. Estkowski LM, Morris JL, Sinclair EA. Characterization of dexmedetomidine dosing and safety in neonates and infants. J Pediatr Pharmacol Ther. (2015) 20:112–8. doi: 10.5863/1551-6776-20.2.112
42. Talke P, Tayefeh F, Sessler DI, Jeffrey R, Noursalehi M, Richardson C. Dexmedetomidine does not alter the sweating threshold, but comparably and linearly decreases the vasoconstriction and shivering thresholds. Anesthesiology. (1997) 87:835iologyeh F, S1097/00000542-199710000-00017
43. Finkel JC, Quezado ZM. Hypothermia-induced bradycardia in a neonate receiving dexmedetomidine. J Clin Anesth. (2007) 19:290nesthezado Z1016/j.jclinane.2006.08.011
44. O'Mara K, Weiss MD. Dexmedetomidine for sedation of neonates with HIE undergoing therapeutic hypothermia: a single-center experience. AJP Rep. (2018) 8:e168873. doi: 10.1055/s-0038-1669938
45. Surkov D. Is dexmedetomidine a potential neuroprotective agent for term neonates with hypoxic-ischemic encephalopathy? Pediatric Anesth Crit Care J. (2019) 7:22Jdc Anesthmed1038/s41372-021-00998-8
46. Cosnahan AS, Angert RM, Jano E, Wachtel EV. Dexmedetomidine vs. intermittent morphine for sedation of neonates with encephalopathy undergoing therapeutic hypothermia. J Perinatol. (2021) 41:2284–91. doi: 10.1038/s41372-021-00998-8
47. Tobias JD. Bradycardia during dexmedetomidine and therapeutic hypothermia. J Intensive Care Med. (2008) 23:403ive Care Med1177/0885066608324389
48. Anderson KB, Poloyac SM, Kochanek PM, Empey PE. Effect of hypothermia and targeted temperature management on drug disposition and response following cardiac arrest: a comprehensive review of preclinical and clinical investigations. Ther Hypothermia Temp Manag. (2016) 6:169pothermia Tem1089/ther.2016.0003
49. Kearns GL, Abdel-Rahman SM, Alander SW, Blowey DL, Leeder JS, Kauffman RE. Developmental pharmacology—drug disposition, action, and therapy in infants and children. N Engl J Med. (2003) 349:1157–17. doi: 10.1056/NEJMra035092
50. Hummel P, Puchalski M, Creech SD, Weiss MG. Clinical reliability and validity of the N-PASS: neonatal pain, agitation and sedation scale with prolonged pain. J Perinatol. (2008) 28:55atoluchalski 1038/sj.jp.7211861
51. Badjatia N, Strongilis E, Gordon E, Prescutti M, Fernandez L, Fernandez A, et al. Metabolic impact of shivering during therapeutic temperature modulation: the Bedside Shivering Assessment Scale. Stroke. (2008) 39:3242–242 doi: 10.1161/STROKEAHA.108.523654
52. Uner IL, Johansen T, Dahle J, Persson M, Stiris T, Andresen JH. Therapeutic hypothermia and N-PASS; results from implementation in a level 3 NICU. Early Hum Dev. (2019) 137:104828. doi: 10.1016/j.earlhumdev.2019.104828
53. Avant D, Wharton GT, Murphy D. Characteristics and changes of pediatric therapeutic trials under the best pharmaceuticals for children act. J Pediatr. (2018) 192:8trf pediatric1016/j.jpeds.2017.08.048
54. Autmizguine J, Benjamin DK, Smith PB, Sampson M, Ovetchkine P, Cohen-Wolkowiez M, et al. Pharmacokinetic studies in infants using minimal-risk study designs. Curr Clin Pharmacol. (2014) 9:350lin Pharmaco2174/1574884709666140520153308
55. Dallefeld SH, Atz AM, Yogev R, Sullivan JE, Al-Uzri A, Mendley SR, et al. A pharmacokinetic model for amiodarone in infants developed from an opportunistic sampling trial and published literature data. J Pharmacokinet Pharmacodyn. (2018) 45:419cokinet Pharm1007/s10928-018-9576-y
56. Gonzalez D, Melloni C, Yogev R, Poindexter BB, Mendley SR, Delmore P, et al. Use of opportunistic clinical data and a population pharmacokinetic model to support dosing of clindamycin for premature infants to adolescents. Clin Pharmacol Ther. (2014) 96:429rmacol Ther p1038/clpt.2014.134
57. Grimstein M, Yang Y, Zhang X, Grillo J, Huang SM, Zineh I, et al. Physiologically based pharmacokinetic modeling in regulatory science: an update from the U.S Food and Drug Administration in regulatory scienceneh Iupport. J Pharm Sci. (2019) 108:21 Scirug Admi1016/j.xphs.2018.10.033
58. Hornik CP, Wu H, Edginton AN, Watt K, Cohen-Wolkowiez M, Gonzalez D. Development of a pediatric physiologically-based pharmacokinetic model of clindamycin using opportunistic pharmacokinetic data. Clin Pharmacokinet. (2017) 56:1343–343 doi: 10.1007/s40262-017-0525-5
59. Thakkar N, Gonzalez D, Cohen-Wolkowiez M, Massaro MM, Bernhardt J, Zane NR, et al. An opportunistic study evaluating pharmacokinetics of sildenafil for the treatment of pulmonary hypertension in infants. J Perinatol. (2016) 36:744tol nistic s1038/jp.2016.79
60. Tremoulet A, Le J, Poindexter B, Sullivan JE, Laughon M, Delmore P, et al. Characterization of the population pharmacokinetics of ampicillin in neonates using an opportunistic study design. Antimicrob Agents Chemother. (2014) 58:3013–013 doi: 10.1128/AAC.02374-13
61. Moorthy GS, Vedar C, Moorthy AS, Prodell JL, Zuppa AF. An improved ultra-high-performance liquid chromatography-tandem mass spectrometric method for the quantitation of dexmedetomidine in small volume of pediatric plasma. Biomed Chromatogr. (2019) 33:e4487. doi: 10.1002/bmc.4487
62. Haataja L, Mercuri E, Guzzetta A, Rutherford M, Counsell S, Flavia Frisone M, et al. Neurologic examination in infants with hypoxic-ischemic encephalopathy at age 9 to 14 months: use of optimality scores and correlation with magnetic resonance imaging findings. J Pediatr. (2001) 138:332 imaging fin1067/mpd.2001.111325
63. Romeo DM, Bompard S, Serrao F, Leo G, Cicala G, Velli C, et al. Early neurological assessment in infants with hypoxic ischemic encephalopathy treated with therapeutic hypothermia. J Clin Med. (2019) 8:1247. doi: 10.3390/jcm8081247
64. Novak I, Morgan C, Adde L, Blackman J, Boyd RN, Brunstrom-Hernandez J, et al. Early, accurate diagnosis and early intervention in cerebral palsy: advances in diagnosis and treatment. JAMA Pediatr. (2017) 171:897atriagnosis an1001/jamapediatrics.2017.1689
65. Lipkin PH, Macias MM, Council Council on Children With Disabilities Section Section on Developmental and Behavioral Pediatrics. Promoting optimal development: identifying infants and young children with developmental disorders through developmental surveillance and screening. Pediatrics. (2020) 145:e20193449. doi: 10.1542/peds.2019-3449
66. Juul SE, Comstock BA, Wadhawan R, Mayock DE, Courtney SE, Robinson T, et al. A randomized trial of erythropoietin for neuroprotection in preterm infants. N Engl J Med. (2020) 382:233Medrial of er1056/NEJMoa1907423
67. Baserga MC, Beachy JC, Roberts JK, Ward RM, DiGeronimo RJ, Walsh WF, et al. Darbepoetin administration to neonates undergoing cooling for encephalopathy: a safety and pharmacokinetic trial. Pediatr Res. (2015) 78:315Resin adminis1038/pr.2015.101
68. Wan M, Orlu-Gul M, Legay H, Tuleu C. Blinding in pharmacological trials: the devil is in the details. Arch Dis Child. (2013) 98:656 Childl M, L1136/archdischild-2013-304037
Keywords: hypoxia-ischemia encephalopathy, therapeutic hypothermia, neuroprotection, pain, sedation, morphine, dexmedetomidine
Citation: Baserga M, DuPont TL, Ostrander B, Minton S, Sheffield M, Balch AH, Bahr TM and Watt KM (2021) Dexmedetomidine Use in Infants Undergoing Cooling Due to Neonatal Encephalopathy (DICE Trial): A Randomized Controlled Trial: Background, Aims and Study Protocol. Front. Pain Res. 2:770511. doi: 10.3389/fpain.2021.770511
Received: 03 September 2021; Accepted: 01 November 2021;
Published: 07 December 2021.
Edited by:
Christopher McPherson, Washington University School of Medicine in St. Louis, United StatesReviewed by:
Manon Ranger, University of British Columbia, CanadaKonstantin Boris Yenkoyan, Yerevan State Medical University, Armenia
Copyright © 2021 Baserga, DuPont, Ostrander, Minton, Sheffield, Balch, Bahr and Watt. This is an open-access article distributed under the terms of the Creative Commons Attribution License (CC BY). The use, distribution or reproduction in other forums is permitted, provided the original author(s) and the copyright owner(s) are credited and that the original publication in this journal is cited, in accordance with accepted academic practice. No use, distribution or reproduction is permitted which does not comply with these terms.
*Correspondence: Mariana Baserga, Mariana.Baserga@hsc.utah.edu