- 1Department of Psychiatry, University of California, San Diego, San Diego, CA, United States
- 2Center for Behavior Genetics of Aging, University of California, San Diego, San Diego, CA, United States
Migraine is a neurological disorder with a prominent sex difference such that two thirds of sufferers are female. The mechanisms behind the preponderance of migraine in women have yet to be elucidated. With data on 51,872 participants from the Swedish Twin Registry, we report results from two distinct analyses intended to clarify the degree to which genetic and environmental factors contribute to sex differences in migraine. First, we fit a sex-limitation model to determine if quantitative genetic differences (i.e., is migraine equally heritable across men and women) and/or qualitative genetic differences (i.e., are different genes involved in migraine across men and women) were present. Next, we used a multilevel logistic regression model to compare the prevalence of migraine in individuals from opposite-sex and same-sex twin pairs to determine whether differences in the prenatal hormone environment contribute to migraine risk. In the final analytic sample, women were found to have a significantly higher rate of migraine without aura relative to men (17.6% vs. 5.5%). The results from an ADE sex-limitation model indicate that migraine is equally heritable in men and women, with a broad sense heritability of 0.45, (95% CI = 0.40–0.50), while results from a reduced AE sex-limitation model provide subtle evidence for differences in the genes underlying migraine across men and women. The logistic regression analysis revealed a significant increase in migraine risk for females with a male co-twin relative to females with a female co-twin (OR = 1.51, 95% CI = 1.26–1.81). These results suggest that the prominent sex difference in migraine prevalence is not entirely accounted for by genetic factors, while demonstrating that masculinization of the prenatal environment may increase migraine risk for females. This effect points to a potential prenatal neuroendocrine factor in the development of migraine.
Introduction
Migraine is a severe neurological disease characterized by repeated transient symptomatic episodes, affecting ~1 in 7 people. Although migraine presents with varying degrees of severity, it is the second largest cause of disability worldwide, and in 2020 the economic burden in the U.S. was estimated at $78 billion (1–3). A prominent sex difference in migraine exists such that roughly 18% of adult women report experiencing migraine compared to only 6% of adult men (4–7). While the preponderance of migraine in women is striking, to date little is known regarding the origins of this sex difference. Analytic methods available in the context of the classical twin design offer unique opportunities to explore this issue.
The classical twin design relies on the comparison of monozygotic (MZ) twins, who are genetically identical, with dizygotic (DZ) twins, who share on average 50% of their segregating DNA, to make inferences regarding the degree to which genetic and environmental factors contribute to disease risk within a population. As a variation of the classical twin model, the sex-limitation model employs data from male and female same-sex (SS) MZ and DZ twins, as well as opposite-sex (OS) DZ twins to test for qualitative and quantitative genetic differences between men and women (8). Qualitative genetic differences assess whether different genes are involved in disease risk across the sexes, while quantitative genetic differences determine if disease risk is equally heritable across the sexes.
Twin and family studies have shown that migraine has a substantial genetic component. First degree relatives of individuals with migraine have a 1.4–3.0 times higher risk of experiencing the disease compared to those with no immediate family history of migraine (9, 10). Concordance rates for migraine are also consistently higher among MZ twins relative to DZ twins, and twin-based heritability estimates range from.34 to.57, indicating that 34 to 57% of the risk liability (i.e., variation in migraine risk within a population) is attributable to genetic factors (11–15).
Several twin studies have explored the possibility of sex differences in the genetic and environmental determinants of migraine. In a study of over 8,000 Finish twin pairs, the heritability of migraine was essentially equal between men and women (0.42 and.4 07, respectively), suggesting little quantitative difference in the magnitude of genetic effects (16). These findings were largely replicated in a subsequent study of 5,000 Danish twins (17). In one of the largest twin studies conducted, Mulder et al. (18) examined the prevalence and heritability of migraine in eight twin cohorts across six countries (n = 330−12,121) and assessed both quantitative and qualitative differences. Heritability estimates ranged from 0.33 to 0.56 and could be equated between men and women both within and between countries, suggesting no quantitative sex differences. Similarly, looking at the individual-cohort level, there was no evidence for qualitative sex differences.
Although Mulder et al. provided strong evidence for a lack of quantitative genetic difference between men and women, the analysis did not leverage the larger combined sample in the test of qualitative genetic differences. One potential reason for this could be because the definition of migraine ranged from a single item response regarding “headache attacks” or receiving a physician diagnosis, to detailed surveys that aligned with the International Headache Society's (IHS) diagnostic criteria. Thus, a large-scale, high-powered test of qualitative genetic differences in a population with a detailed classification of migraine has yet to be conducted. The first goal of the present study was to replicate the sex-limitation model investigation done by Mulder et al. in a larger sample with greater statistical power, and for which migraine has been diagnosed using a consistent, detailed set of criteria.
In addition to the sex-limitation model, the comparison of prevalence rates of disease in twins from OS DZ pairs relative to SS pairs through an opposite-sex twin comparison paradigm provides insight into how variation within the prenatal environment can impact brain development and disease risk. While not a genetically informative analysis, this design does allow for inferences to be made regarding the role of the prenatal hormone milieu on lifetime outcomes.
There is substantial evidence to suggest that sex steroid hormones may contribute to the observed sex difference in the prevalence of migraine. The “estrogen withdrawal hypothesis” (19) specifically suggests that changes in serum estradiol levels are capable of precipitating migraine attacks. In support of this hypothesis, before puberty, when sex steroid hormones are largely dormant, the prevalence of migraine is equal in boys and girls (20, 21). In women, age at menarche is associated with age at migraine onset such that each one-year delay in onset of menarche decreases the odds of migraine by seven percent (22). During the reproductive period, menses, which corresponds to a cyclical decline in estradiol and progesterone, is associated with an elevated risk of migraine attack (23–25). During perimenopause, a transition period characterized by the fluctuation and progressive loss of estradiol, migraine attacks in women commonly increase in frequency (26, 27). Finally, a lower incidence of migraine is observed following the menopausal transition (6, 26, 27). Migraine throughout the male lifetime is understudied; however, men with migraine have been found to have lower testosterone and higher estradiol levels relative to age matched controls (28, 29).
In conjunction with the potential influence of circulating sex steroid hormones, evidence from both animal and human twin studies suggest an association between the prenatal hormonal milieu and disease risk later in life. Expressly, the “twin testosterone transfer hypothesis” posits that females gestated with a male co-twin will demonstrate physiological and behavioral masculinization (30). In support of this hypothesis, several rodent studies have shown that, compared to female rodents gestated with other females, female fetuses gestated with males demonstrated higher blood concentrations of testosterone and lower levels of estradiol, increased anogenital distance, and more aggressive and territorial behaviors (30–32). Critically, prenatal administration of an anti-androgen prevented the masculinization of female rodents, suggesting that the effects were driven by increased androgen exposure in utero. In humans, twin studies have demonstrated that females with a male co-twin exhibit lasting masculinization of perception, cognition, personality and risk of dementia (30, 33–36). Therefore, the second goal of this study was to conduct a novel comparison of SS and OS twins to examine the role of the prenatal environment, specifically hormonal variation within the prenatal environment, on migraine risk.
In the present study, we utilized data from the Swedish Twin Registry (STR), one of the largest population-based twin registries in the world (37), to conduct a comprehensive, genetically informed examination of sex differences in migraine. With data from over 18,000 complete twin pairs, this study represents the largest investigation of qualitative genetic differences in migraine ever conducted. In addition, we leveraged the presence of OS twin pairs to test the novel hypothesis that the prenatal hormonal milieu contributes to migraine risk in women. We hypothesized that the presence of a male co-twin would decrease migraine risk in females due to androgenization effects produced by the inter-twin transfer of testosterone.
Methods
Participants
The sample was pooled from 70,223 SS and OS MZ and DZ twins who participated in the STR. Structured interviews evaluating multiple health outcomes, including migraine, were administered as part of two independent studies: the Screening Across the Lifespan Twin Study (SALT) questionnaire one and two, and the Study of Twin Adults—Genes and Environment (STAGE). The analytic sample contains data from 51,872 twins with complete data born between 1959 and 1985 including 18,365 SS female twins, 15,335 SS male twins, 8,433 OS male twins and 9,739 OS female twins. See demographic information for the analytic sample in Table 1.
Assessment of Migraine Without Aura
Sufficient data from both SALT and STAGE allowed for the utilization of the IHS classification of migraine without aura (MO). Items selected best adhered to the IHS International Classification of Headache Disorders version three (ICHD-3) MO criteria A, B, C, and D including attack recurrence, duration, characteristics (unilateral location, pulsating quality, moderate or severe pain intensity, aggravation by or causing avoidance of routine physical activity), and symptoms (nausea and/or vomiting, photophobia and phonophobia; Table 2). Notably, STAGE did not have a proxy for duration of attack (between four and 72 h), so this criterion was omitted from migraine classification for the STAGE cohort.
Statistical Analyses
Sex-Limitation Model
Within the classical twin design, data from MZ and DZ twins are used to decompose the variance of a trait/phenotype into additive genetic (A) influences (i.e., genetic effects attributable to multiple genes that exert their independent influence in a linear fashion), non-additive genetic (D) influences [i.e., genetic effects attributable to dominance and/or epistasis; (38)], common or shared environmental (C) influences (i.e., environmental factors that make members of a twin pair similar to one another), and unique environmental (E) influences [i.e., environmental factors that make members of a twin pair different from one another, including measurement error; (8)].
Additive and non-additive genetic influences are assumed to correlate perfectly (rA and rD = 1.0) between MZ twins because they are genetically identical. In contrast, DZ twins share on average 50% of their segregating DNA and are therefore assumed to demonstrate a correlation of rA = 0.50 for additive genetic influences and rD = 0.25 for non-additive genetic influences. When standardized, the combined additive and non-additive genetic influences provide an estimate of broad-sense heritability. The shared environment, by definition, correlates perfectly between both members of a twin pair, regardless of their zygosity. Unique or non-shared environmental influences are uncorrelated among twin pairs.
Due to issues of model identification, A, D, C, and E variance components cannot be simultaneously fit with only twin data; therefore, researchers are restricted to fitting either an ACE or ADE model. For the present study, examination of cross-twin tetrachoric correlations revealed that for both male and female SS twins the MZ correlation was more than twice that of the DZ correlation (Male Twins: rMZ = 0.46, rDZ = 0.19; Female Twins: rMZ = 0.44, rDZ = 0.11), indicating a minimal impact of shared environmental influences (C) and that an ADE model would provide the most appropriate representation of the data. Therefore, we utilized the ADE model within the sex-limitation model framework.
The sex-limitation model involves the application of the ADE model to data from male and female twins simultaneously, thereby allowing tests of heterogeneity of the variance components between the sexes (i.e., are the magnitudes of genetic and environmental contributions equivalent; see Figure 1). Specifically, quantitative sex differences are tested by constraining the genetic and environmental variance components to be equal across the male and female groups (i.e., AM= AF, DM= DF, EM= EF). Should these constraints result in a significant change in model fit (p <0.05) relative to an unconstrained model, quantitative differences can be inferred. Furthermore, with the inclusion of OS twins, the sex-limitation model allows for estimation of the genetic correlation between male and female twins in OS pairs, which enables the additional test of qualitative genetic differences. Qualitative genetic differences are inferred when the genetic correlation between OS DZ twins deviates significantly from the value assumed for SS DZ twins (0.5 for additive genetic influences and 0.25 for non-additive genetic influences).
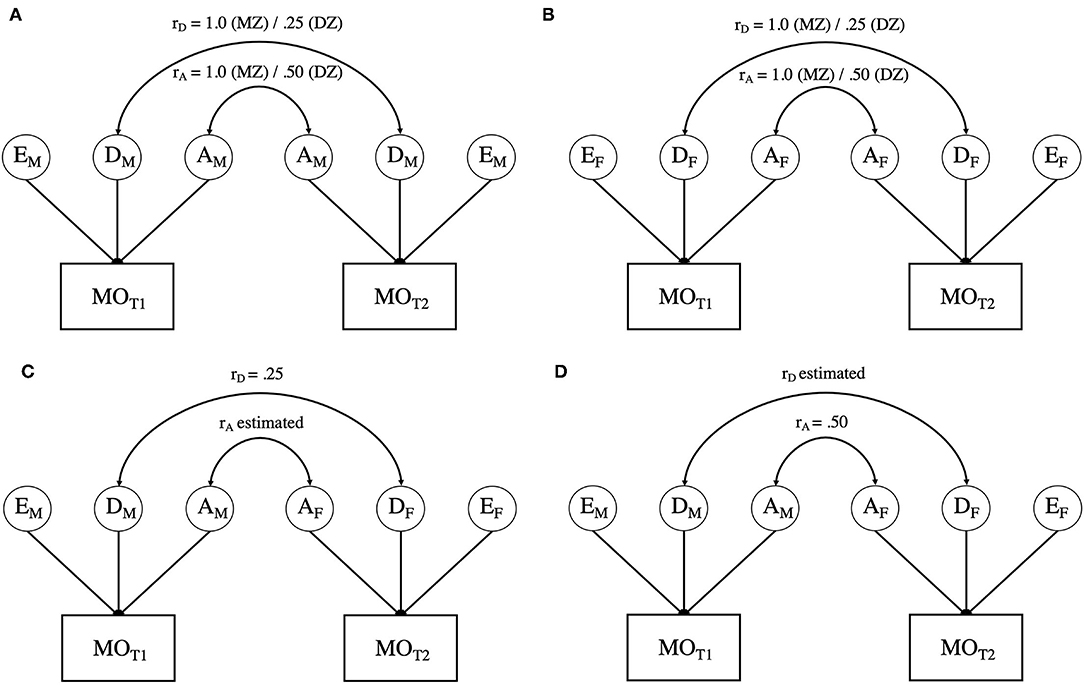
Figure 1. (A) presents the path diagram for male-male twin pairs. (B) presents the path diagram for female-female twin pairs. (C,D) offer two variations of estimating variation in opposite sex twins. As noted in the text, due to issues of model identification, rA, and rD cannot be simultaneously estimated. Variance components with the same subscript are constrained to be equal within and across all twin pairs. MO = Migraine without aura; T1 = twin 1; T2 = twin 2; A = Additive genetic influences; C = Common environmental influences; E = Unique or non-shared environmental influences. Aspects of the model with an “M” subscript indicate those elements that are specific to males, whereas those with an “F” are specific to females.
To evaluate all aspects of the sex-limitation model, a series of four models were fit and tested against the Saturated Model (see Table 3). The Saturated Model provides a mathematical representation of the data against which all theoretical (i.e., sex-limitation) models are compared. Model 1 addressed qualitative differences by allowing the correlation between the additive genetic components (rA) of the OS twins to be estimated while constraining the correlation of the non-additive genetic influences to the expected DZ twin value (i.e., rD = 0.25; see Figure 1C). Model 2 inverted these constraints (restricting the additive genetic correlation to rA = 0.5, in line with the expected DZ twin correlation and estimating the non-additive genetic correlation (rD); see Figure 1D). Model 3 constrained both the additive and non-additive genetic correlations to the expected DZ values (rA = 0.5 and rD = 0.25). While Models 1−3 allowed the ADE variance parameters to be independently estimated for men and women, Model 4 constrained the variance components to be equivalent across men and women (i.e., AM = AF, DM = DF, EM = EF) while maintaining the restraints on the additive and non-additive genetic correlations from Model 3, thereby allowing for the test of quantitative sex differences.
Models were fit to all available raw data by full information maximum-likelihood, using the structural equation modeling software OpenMx (v2.19.5) within R (39–41). Given the binary nature of the outcome (i.e., positive or negative history of MO), threshold liability models were utilized whereby a threshold for migraine was estimated for male and female twins, and the phenotypic variance was constrained to 1.0 (8). Evaluation of model fit employed the likelihood-ratio chi-square test (LRT), which is calculated as the difference in the negative 2 log-likelihood (-2LL) of a model relative to that of a comparison model. The Akaike's information criterion (AIC) was used as a secondary indicator of model fit, with lower AIC values indicating a preferred balance between model fit and parsimony (42). Significance of individual model parameters (e.g., rA, rD, A, D, E) was determined by calculation of likelihood-based 95% confidence intervals (CIs).
Opposite-Sex Twin Comparison
To compare the rates of migraine in SS and OS twin pairs, a multilevel logistic regression model was fit to all available data using the glmer function from the lme4 package [version 1.1.27.1; (43)] in R (version 4.0.4; 43). Fit was accomplished by maximum-likelihood estimation via the Laplace approximation with the nlminb optimizer wrapped through optimx (44, 45). Migraine diagnosis was included as the binary outcome (yes/no), predicted by study (included as a 3-level categorical variable: SALT questionnaire 1, SALT questionnaire 2 and STAGE), and twin-pair type (included as a 4-level categorical variable: SS female, SS male, OS male, and OS female, with SS female as the reference level). The SALT study utilized two versions of its medical history interview, and while the items were equivalent, the different structure of the assessments warranted distinct coding. Twin pair was included as a random effect to account for non-independence of the observations. Given that the primary aim of this analysis was to compare the prevalence of migraine among SS vs. OS individuals as a function of sex (i.e., SS females vs. OS females, and SS males vs. OS males), an analogous model was fit to the data using SS male as the reference level.
Results
Of the 70,223 STR participants, 1,525 individuals did not have information available regarding co-twin sex, and an additional 16,826 individuals did not have sufficient information to determine migraine status, resulting in a total analytic sample of 51,872 individuals, representing 18,828 complete twin pairs. Out of the analytic sample, 6,247 (12.0%) individuals met the diagnostic criteria for migraine. Of those meeting the diagnostic criteria, 79.0% were female, such that the prevalence of migraine was 17.6% for women and 5.5% for men.
Qualitative and Quantitative Genetic Difference
As shown in Table 3, both variants of the unconstrained ADE sex-limitation model (Models 1 and 2), provided good fits relative to the Saturated Model (i.e., both models resulted in a non-significant change in −2LL). Indeed, both models provided near identical fits and resulted in AIC values that were negligibly different from one another (−67,714.81 vs. −67,714.85). Liability thresholds for men and women were allowed to vary, as equating them resulted in a significant reduction in fit (p <0.001), consistent with the substantially higher prevalence of migraine in women than men in the sample. Fixing the OS DZ twin genetic correlations to their expected values (Model 3: rA = 0.50, rD = 0.25) also resulted in a good fit relative to the Saturated Model (LRT = 8.54, ΔDF = 9, p = 0.48). Further, comparison of Model 3 with the variants of the unconstrained ADE sex limitation models (Models 1 and 2) indicated that constraining the genetic correlations did not result in a significant change in fit (fit relative to Model 1: LRT = 0.24, ΔDF = 1, p = 0.62; fit relative to Model 2: LRT = 0.28, ΔDF = 1, p = 0.59). The additional constraints did not significantly reduce model fit, which suggests that there is no qualitative sex difference in the genetic determinants of migraine (i.e., we found no evidence that migraine risk is driven by different genes in men and women).
Constraining the genetic and environmental variance components (A, D, E) to be equal across men and women (Table 3, Model 4) resulted in a non-significant change in fit relative to the Saturated Model and resulted in the lowest AIC value of all models tested. Change in fit relative to Model 3 (which tested for qualitative sex differences) was also non-significant (LRT = 1.06, ΔDF = 3, p = 0.79). Thus, here there is no evidence supporting quantitative genetic difference in migraine across the sexes.
In this final model, the broad sense heritability (A + D) of migraine was found to be.45 (95% CI:0.40 −0.50), indicating that 45% of the variation in risk for migraine was due to genetic factors. Much of this effect was attributable to non-additive genetic factors (D = 0.38, 95% CI:0.18–0.50), with a relatively small contribution of additive genetic factors (A = 0.07, 95% CI:0.04–0.26). The remainder of risk for migraine was accounted for by unique environmental factors (E = 0.55, 95% CI:0.50–0.60).
Reduced Genetic Model
The presence of both additive and non-additive genetic influences complicated the test of qualitative sex differences as we were unable to simultaneously estimate rA and rD in the OS DZ twins. We therefore fit a series of three reduced genetic models to determine if simplifying the number of model parameters improved our ability to detect qualitative genetic differences. For all reduced models, non-additive genetic influences were constrained to zero (D = 0), resulting in a model where only additive genetic (A) and unique environmental (E) variance components were estimated.
The unconstrained AE sex-limitation model resulted in a non-significant change in fit relative to the Saturated Model (LRT = 17.24, ΔDF = 10, p = 0.07). It is worth noting, however, that the AIC value for the unconstrained AE sex-limitation model was larger than the AIC values for either of the ADE variants, highlighting the importance of non-additive genetic factors to migraine. Constraining the additive genetic correlation (rA) to 0.50 within the OS DZ twins resulted in a significant change in fit relative to the Saturated Model (LRT = 21.61, ΔDF = 11, p = 0.03) and the unconstrained AE sex-limitation model (LRT = 4.37, ΔDF = 1, p = 0.04). Thus, the estimated rA of 0.32 (95% CI:0.16–0.49) was found to significantly deviate from the assumed 0.50 value, indicating qualitative sex differences in the additive genetic components of migraine between men and women. The final best-fitting model under this reduced genetic influence scenario was obtained by constraining the A and E variance components to be equal across men and women (A = 0.42, 95% CI:0.37–0.47; E = 0.58, 95% CI:0.53–0.63), while also estimating the genetic correlation between OS twins at 0.32. This simplified model suggests that there are slight qualitative genetic differences in migraine across the sexes.
Opposite Sex Twin Comparison Results
Both study and twin-pair type were significant predictors of migraine prevalence, as evidenced by significant improvements in fit compared to a pair of models with each predictor dropped (study: LRT = 40.7 ΔDF = 2, p <0.001 and twin-pair type: LRT = 1,542.9, ΔDF = 3, p <0.001). Specifically, STAGE was associated with an increased odds of migraine (OR = 1.74, 95% CI = 1.46–2.07) compared to SALT questionnaire 1, while the two SALT cohorts did not differ (SALT questionnaire 1 OR = 1.07, 95% CI = 0.80–1.44). This difference is likely driven by the inability of the STAGE interview to address ICHD-3 criteria B (Table 2), resulting in a higher prevalence of migraine (14.8 vs. 10.5% and 10.0% in SALT questionnaire 1 and 2, respectively).
As anticipated, due to the significantly higher prevalence of migraine among women than men, in comparison to SS females, both SS males and OS males had significantly lower odds of migraine (OR = 0.25, 95% CI = 0.20–0.32 and OR = 0.03, 95% CI = 0.02–0.04). However, contrary to our hypothesis, in comparison to SS females, OS females had significantly higher odds of migraine (OR = 1.51, 95% CI = 1.26–1.81). When SS males were included as the reference level, as expected, both SS and OS females demonstrated increased odds of migraine (OR = 4.08, 95% CI = 3.20–5.20 and OR = 6.15, 95% CI = 4.79–7.89), but OS males surprisingly had significantly lower odds of migraine (OR = 0.11, 95% CI = 0.08–0.15). See Figure 2 for odds ratios plot of migraine risk by twin type.
Discussion
In the present study we utilized one of the largest single-cohort twin samples ever collected to examine the origins of sex differences in migraine. Our findings from the ADE sex-limitation model are largely in alignment with previous literature (16–18). Specifically, we observed a broad-sense heritability of 0.45, which was primarily driven by non-additive genetic effects. Results from the ADE model also revealed that when additive and non-additive genetic influences were modeled together there is no evidence for qualitative or quantitative genetic differences in migraine risk across males and females. Prior studies, including those that have used data from the STR, have also not reported quantitative or qualitative genetic differences (18, 46). However, under a reduced model, one in which only additive genetic and unique environmental influences were modeled (AE model), we did find evidence for subtle qualitative differences in migraine across the sexes, while heritability remained equivalent.
Our finding of a qualitative genetic difference between men and women when a reduced, or simplified, genetic model was tested suggests that additional exploration of genetic differences in migraine is warranted. By fitting a model with only additive genetic influence, we reduced the number of model parameters and improved our ability to estimate the genetic correlation between OS twins. Thus, it may be the case that this effect is an artifact of the increased power of the analysis due to the simplification of the model. It is worth noting that the SALT and STAGE studies provided some of the most in-depth assessments of migraine available for genetically informative research. This in conjunction with the large sample size strongly supports the validity of the finding that non-additive and additive genetic factors contribute to migraine risk. Given this more complex genetic architecture of migraine, larger sample sizes will be needed to fully elucidate genetic effects, especially qualitative sex differences, both in the context of the twin design and genome-wide association studies.
Contrary to our hypothesis, the analysis of OS twins relative to SS female twins found that females with a male co-twin demonstrated a higher prevalence of migraine compared to females with a female co-twin. This result adds to a growing body of twin studies of behavioral phenotypes, perception, personality, cognition, and disease risk that support the “twin testosterone transfer hypothesis” (30, 33, 35). However, this effect was in opposition to our predictions, as we theorized that the presence of a male in utero would decrease a female's risk of migraine. The direction of the reported effect was surprising given that males have lower rates of migraine, suggesting that testosterone promotes resistance to the disease or demonstrates anti-nociceptive effects (28, 29, 47–49).
Although these findings were in opposition to our hypothesis, there is physiological evidence to suggest that the result are valid. For example, it is possible that female fetuses could have the biological mechanisms necessary to mitigate any lasting effects of exogenous gonadal hormones. In the developing female fetus, maternal testosterone is converted to estrogens via aromatase (50). Thus, one theory is that the testosterone transferred to the female fetus from a male co-twin may be fully converted to estrogen before any lasting androgenization occurs. While this could explain why we did not observe a decrease in disease risk for females with a male co-twin, this would not account for the increased migraine risk observed for those females.
To address this point, we theorize that having a male co-twin could modify the female endocrine system such that females become more sensitive to estrogen, or changes in estrogen, throughout their lifetime. Evidence supports organizational effects of prenatal androgens on the female endocrine system including estrogen receptors (51–53). It is also possible that prenatal hormones alter the female nervous system at the epigenetic level. Specifically, evidence suggests that in utero androgens impart lasting DNA methylation modifications in females with a male co-twin (54). OS females are discernable from SS females with OS females demonstrating greater similarities to male DNA methylation patterns (54). Critically, DNA methylation differences between OS females and SS females were related to nervous system regulation, a physiological component that strongly relates to migraine (55). Further research is clearly required to investigate these organizational and epigenetic theories, and to identify a more detailed mechanism to account for the observed results.
Limitations
The present study is not without its limitations. First, the STR does not offer data on migraine days per-month, a descriptor that is used to diagnoses subtypes of migraine (i.e., episodic, chronic, refractory, etc.). This prevented us from being able to parse out migraine without aura subtypes. Furthermore, the STR is also lacking data for age at onset of migraine, which would be advantageous when addressing the influence of lifetime hormonal events on sex differences in migraine. Data also lacked information on lifetime course of migraine. It is unknown if most of the suspected cases of migraine reflect lifelong conditions, more short-term events or newly onset symptoms. While the diagnostic assessments utilized were thorough, we were nevertheless unable to sufficiently address migraine with aura according to the ICHD-3 criteria, which more than 30% of all migraine patients experience. There is an ongoing debate about if and to what degree migraine with and without aura are distinct entities (56–59).
Future Directions
Results from the present study indicate that the sex-skew in the prevalence of migraine is not immediately attributable to differences in underlying genetic determinants. However, the presence of non-additive genetic effects complicates any genetically informed analysis, as most are based on simplified additive-only models. Even larger sample sizes than what was used here are needed to achieve sufficient power to fully elucidate the impact of these non-additive effects. Although the mechanism of action remains unclear, differences in the hormonal milieu of the prenatal environment do appear to alter disease risk for men and women. Under the traditional biometrical model of disease risk, the next avenue of exploration is likely to be interactions between genetic and environmental factors, as well as possible interactions between genetic and endocrine factors.
Ample knowledge is still to be gained surrounding the genetic epidemiology of migraine from data involving age at onset of disease and timing of critical hormonal events. These data will enable a thorough investigation into the genetic and environmental contributors to sex differences in migraine. The traditional approach of covarying for sex in a sample population with migraine is ineffective and dismissible as it does not account for the possibility of different factors contributing to migraine in men and women. Another potentially interesting future direction could be the examination of migraine risk among daughters of women with polycystic ovarian syndrome, due to fetal exposure to elevated testosterone levels (60, 61). Understanding migraine in a sex-specific manner is an important goal as it holds promise for improving clinical care, diagnostic abilities, and therapeutic interventions for both men and women.
Conclusions
Here we present the most in-depth analysis of the factors that contribute to migraine risk known to date. Specifically, we leveraged the largest migraine sample populations (n = 51,872) and present the first evidence that genetic factors in migraine risk may be different across the sexes. Additionally, although we hypothesized that the presence of a male co-twin would impart a masculinizing effect and reduce the risk of migraine in females, our analysis revealed an increase in migraine risk for females with a male co-twin relative to females with a female co-twin. Although the present analysis was unable to identify specific genes that differed across the sexes, we propose a series of potential mechanisms through which early environmental factors could influence migraine risk.
Data Availability Statement
The datasets presented in this article are not readily available because data was sourced from the Swedish Twin Registry. Requests to access the datasets should be directed to https://ki.se/en/research/swedish-twin-registry-for-researchers.
Author Contributions
MF and MP are credited with the conception of the study. MF was the primary manuscript author. All authors contributed to data analysis and interpretation, revising, and editing.
Conflict of Interest
The authors declare that the research was conducted in the absence of any commercial or financial relationships that could be construed as a potential conflict of interest.
Publisher's Note
All claims expressed in this article are solely those of the authors and do not necessarily represent those of their affiliated organizations, or those of the publisher, the editors and the reviewers. Any product that may be evaluated in this article, or claim that may be made by its manufacturer, is not guaranteed or endorsed by the publisher.
Abbreviations
References
1. GBD. Diseases Injuries Collaborators. 2020. Global burden of 369 diseases and injuries in 204 countries and territories, 1990-2019: a systematic analysis for the Global Burden of Disease Study 2019. Lancet. (2019) 396:1204–22.
2. Vos T, Abajobir AA, Abate KH, Abbafati C, Abbas KM, Abd-Allah F, et al. Global, regional, and national incidence, prevalence, and years lived with disability for 328 diseases and injuries for 195 countries, 1990–2016: a systematic analysis for the Global Burden of Disease Study 2016. Lancet. (2017) 390:1211–59. doi: 10.1016/S0140-6736(17)32154-2
3. Polson M, Williams TD, Speicher LC, Mwamburi M, Staats PS, Tenaglia AT. Concomitant medical conditions and total cost of care in patients with migraine: a real-world claims analysis. Am J Manag Care. (2020) 26:S3–7. doi: 10.37765/ajmc.2020.42543
4. Burch R, Rizzoli P, Loder E. The prevalence and impact of migraine and severe headache in the United States: figures and trends from government health studies. Headache. (2018) 58:496–505. doi: 10.1111/head.13281
5. Victor TW, Hu X, Campbell JC, Buse DC, Lipton RB. Migraine prevalence by age and sex in the United States: a life-span study. Cephalalgia. (2010) 30:1065–72. doi: 10.1177/0333102409355601
6. Sacco S, Ricci S, Degan D, Carolei A. Migraine in women: the role of hormones and their impact on vascular diseases. J Headache Pain. (2012) 13:177–89. doi: 10.1007/s10194-012-0424-y
7. Lipton RB, Stewart WF, Diamond S, Diamond ML, Reed M. Prevalence and burden of migraine in the United States: data from the American Migraine Study II. Headache. (2001) 41:646–57. doi: 10.1046/j.1526-4610.2001.041007646.x
8. Neale MC, Cardon LR. Methodology for genetic studies of twins and families. Springer Science & Business Media. (2013).
9. Russell MB, Iselius L, Olesen J. Inheritance of migraine investigated by complex segregation analysis. Hum Genet. (1995) 96:726–30. doi: 10.1007/BF00210307
10. Low NCP, Cui L, Merikangas KR. Sex differences in the transmission of migraine. Cephalalgia. (2007) 27:935–42. doi: 10.1111/j.1468-2982.2007.01378.x
11. Ulrich V, Gervil M, Kyvik KO, Olesen J, Russell MB. Evidence of a genetic factor in migraine with aura: a population-based Danish twin study. Ann Neurol. (1999) 45:242–6. doi: 10.1002/1531-8249(199902)45:2<242::aid-ana15>3.0.co;2-1
12. Cox HC, Lea RA, Bellis C, Carless M, Dyer TD, Curran J, et al. A genome-wide analysis of'Bounty'descendants implicates several novel variants in migraine susceptibility. Neurogenetics. (2012) 13:261–6. doi: 10.1007/s10048-012-0325-x
13. Wang XP, Liu JM, Zhao YB. Migraine: Sex-influenced trait model? Medical hypotheses. (2008) 71:14–21. doi: 10.1016/j.mehy.2007.12.015
14. Nyholt DR, Gillespie NG, Heath AC, Merikangas KR, Duffy DL, Martin NG. Latent class and genetic analysis does not support migraine with aura and migraine without aura as separate entities. Genet Epidemiol. (2004) 26:231–44. doi: 10.1002/gepi.10311
15. Ziegler DK, Hur YM, Bouchard Jr TJ, Hassanein RS, Barter R. Migraine in twins raised together and apart. Headache. (1998) 38:417–22. doi: 10.1046/j.1526-4610.1998.3806417.x
16. Honkasalo ML, Kaprio J, Winter T, Heikkilä K, Sillanpää M, Koskenvuo M. Migraine and concomitant symptoms among 8167 adult twin pairs. Headache. (1995) 35:70–78. doi: 10.1111/j.1526-4610.1995.hed3502070.x
17. Gervil M, Ulrich V, Kaprio J, Olesen J, Russell MB. The relative role of genetic and environmental factors in migraine without aura. Neurology. (1999) 53:995–995. doi: 10.1212/WNL.53.5.995
18. Mulder EJ, Van Baal C, Gaist D, Kallela M, Kaprio J, Svensson DA, et al. Genetic and environmental influences on migraine: a twin study across six countries. Twin Research and Human Genetics. (2003) 6:422–31. doi: 10.1375/136905203770326420
19. Reddy N, Desai MN, Schoenbrunner A, Schneeberger S, Janis JE. The complex relationship between estrogen and migraines: a scoping review. Syst Rev. (2021) 10:1–13. doi: 10.1186/s13643-021-01618-4
20. Lipton RB, Bigal ME. The epidemiology of migraine. Am J Med. (2005) 118:3–10. doi: 10.1016/j.amjmed.2005.01.014
21. MacGregor EA. Menstruation, sex hormones, and migraine. Neurol Clin. (1997) 15:125–41. doi: 10.1016/S0733-8619(05)70299-1
22. Maleki N, Kurth T, Field AE. Age at menarche and risk of developing migraine or non-migraine headaches by young adulthood: A prospective cohort study. Cephalalgia. (2017) 37:1257–63. doi: 10.1177/0333102416677999
23. Johannes CB, Linet MS, Stewart WF, Celentano DD, Lipton RB, Szklo M. Relationship of headache to phase of the menstrual cycle among young women: a daily diary study. Neurology. (1995) 45:1076–82. doi: 10.1212/WNL.45.6.1076
24. Stewart WF, Lipton RB, Chee E, Sawyer J, Silberstein SD. Menstrual cycle and headache in a population sample of migraineurs. Neurology. (2000) 55:1517–23. doi: 10.1212/wnl.55.10.1517
25. Wöber C, Brannath W, Schmidt K, Kapitan M, Rudel E, Wessely P, et al. Prospective analysis of factors related to migraine attacks: the PAMINA study. Cephalalgia. (2007) 27:304–314. doi: 10.1111/j.1468-2982.2007.01279.x
26. Martin VT, Pavlovic J, Fanning KM, Buse DC, Reed ML, Lipton RB. Perimenopause and menopause are associated with high frequency headache in women with migraine: results of the american migraine prevalence and prevention study. Headache. (2016) 56:292–305. doi: 10.1111/head.12763
27. Wang SJ, Fuh JL, Lu SR, Juang KD, Wang PH. Migraine prevalence during menopausal transition. Headache. (2003) 43:470–8. doi: 10.1046/j.1526-4610.2003.03092.x
28. Shields LB, Seifert T, Shelton BJ, Plato B. Testosterone levels in men with chronic migraine. Neurol Int. (2019) 11:18–20. doi: 10.4081/ni.2019.8079
29. van Oosterhout WP, Schoonman GG, van Zwet EW, Dekkers OM, Terwindt GM, MaassenVanDenBrink A, et al. Female sex hormones in men with migraine. Neurology. (2018) 91:e374–81. doi: 10.1212/WNL.0000000000005855
30. Tapp AL, Maybery MT, Whitehouse AJ. Evaluating the twin testosterone transfer hypothesis: a review of the empirical evidence. Horm Behav. (2011) 60:713–22. doi: 10.1016/j.yhbeh.2011.08.011
31. Ryan BC, Vandenbergh JG. Intrauterine position effects. Neurosci Biobehav Rev. (2002) 26:665–78. doi: 10.1016/S0149-7634(02)00038-6
32. Breedlove SM. Sexual differentiation of the human nervous system. Annu Rev Psychol. (1994) 45:389–418. doi: 10.1146/annurev.ps.45.020194.002133
33. Loehlin JC, Martin NG. Dimensions of psychological masculinity-femininity in adult twins from opposite-sex and same-sex pairs. Behav Genet. (2000) 30:19−28.
34. Vuoksimaa E, Kaprio J, Kremen WS, Hokkanen L, Viken RJ, Tuulio-Henriksson A, et al. Having a male co-twin masculinizes mental rotation performance in females. Psychol Sci. (2010) 21:1069–71. doi: 10.1177/0956797610376075
35. Verweij KJ, Mosing MA, Ullén F, Madison G. Individual differences in personality masculinity-femininity: Examining the effects of genes, environment, and prenatal hormone transfer. Twin Research and Human Genetics. (2016) 19:87–96. doi: 10.1017/thg.2016.8
36. Luo J, Beam CR, Karlsson IK, Pike CJ, Reynolds CA, Gatz M. Dementia risk in women higher in same-sex than opposite-sex twins. Alzheimers Dement (Amst). (2020) 12:e12049. doi: 10.1002/dad2.12049
37. Lichtenstein P, Sullivan PF, Cnattingius S, Gatz M, Johansson S, Carlströ E, et al. The Swedish Twin Registry in the third millennium: an update. Twin Res Hum Genet. (2006) 9:875–82. doi: 10.1375/twin.9.6.875
38. Rettew DC, Rebollo-Mesa I, Hudziak JJ, Willemsen G, Boomsma DI. Non-additive and additive genetic effects on extraversion in 3314 Dutch adolescent twins and their parents. Behav Genet. (2008) 38:223–33. doi: 10.1007/s10519-008-9192-5
39. R Core Team. R: A language and environment for statistical computing. R Foundation for Statistical Computing. Vienna, Austria. (2021). Available online at: https://www.R-project.org/
40. Boker S, Neale M, Maes H, Wilde M, Spiegel M, Brick T, et al. OpenMx: an open source extended structural equation modeling framework. Psychometrika. (2011) 76:306–17. doi: 10.1007/s11336-010-9200-6
41. Neale MC, Hunter MD, Pritikin JN, Zahery M, Brick TR, Kirkpatrick RM, et al. OpenMx 2.0: Extended structural equation and statistical modeling. Psychometrika. (2016) 81:535–49. doi: 10.1007/s11336-014-9435-8
42. Akaike H. Factor analysis and AIC. In Selected papers of hirotugu akaike. New York, NY: Springer. (1987). p. 371–86. doi: 10.1007/978-1-4612-1694-0_29
43. Bates D, Maechler M, Bolker B, Walker S. Fitting linear mixed-effects models using lme4. J Stat Softw. (2015) 67:1–48. doi: 10.18637/jss.v067.i01
44. Nash JC, Varadhan R. Unifying optimization algorithms to aid software system users: optimx for R. J Statist Software. (2011). 43:1–14. doi: 10.18637/jss.v043.i09
45. Nash JC. On best practice optimization methods in R. J Stat Softw. 60:1–14. doi: 10.18637/jss.v060.i02
46. Svensson DA, Larsson B, Waldenlind E, Pedersen NL. Shared rearing environment in migraine: results from twins reared apart and twins reared together. Headache. (2003) 43:235–44. doi: 10.1046/j.1526-4610.2003.03047.x
47. Fischer L, Clemente JT, Tambeli CH. The protective role of testosterone in the development of temporomandibular joint pain. J Pain. (2007) 8:437–42. doi: 10.1016/j.jpain.2006.12.007
48. Vetvik KG, MacGregor EA. Sex differences in the epidemiology, clinical features, and pathophysiology of migraine. Lancet Neurol. (2017) 16:76–87. doi: 10.1016/S1474-4422(16)30293-9
49. Glaser R, Dimitrakakis C, Trimble N, Martin V. Testosterone pellet implants and migraine headaches: a pilot study. Maturitas. (2012) 71:385–8. doi: 10.1016/j.maturitas.2012.01.006
50. Kallak TK, Hellgren C, Skalkidou A, Sandelin-Francke L, Ubhayasekhera K, Bergquist J, et al. Maternal and female fetal testosterone levels are associated with maternal age and gestational weight gain. Eur J Endocrinol. (2017) 177:379–88. doi: 10.1530/EJE-17-0207
51. Landers RS, Padmanabhan V, Cardoso RC. Developmental programming: gestational testosterone excess disrupts LH secretion in the female sheep fetus. Reprod Biol Endocrinol. (2020) 18:1–11. doi: 10.1186/s12958-020-00667-z
52. Kühnemann S, Brown TJ, Hochberg RB, MacLusky NJ. Sex differences in the development of estrogen receptors in the rat brain. Horm Behav. (1994) 28:483–91. doi: 10.1006/hbeh.1994.1046
53. Vito CC, Fox TO. Androgen and estrogen receptors in embryonic and neonatal rat brain. Brain Res. (1981) 2:97–110. doi: 10.1016/0165-3806(81)90062-6
54. Kong S, Peng Y, Chen W, Ma X, Wei Y, Zhao Y, et al. Epigenetic consequences of hormonal interactions between opposite-sex twin fetuses. Clin Transl Med. (2020) 10:e234. doi: 10.1002/ctm2.234
55. Shechter A, Stewart WF, Silberstein SD, Lipton RB. Migraine and autonomic nervous system function: a population-based, case-control study. Neurology. (2002) 58:422–7. doi: 10.1212/WNL.58.3.422
56. Kincses ZT, Veréb D, Faragó P, Tóth E, Kocsis K, Kincses B, et al. Are migraine with and without aura really different entities? Front Neurol. (2019) 10:982. doi: 10.3389/fneur.2019.00982
57. Vgontzas A, Burch R. Episodic migraine with and without aura: key differences and implications for pathophysiology, management, and assessing risks. Curr Pain Headache Rep. (2018) 22:1–8. doi: 10.1007/s11916-018-0735-z
58. Granziera C, DaSilva AFM, Snyder J, Tuch DS, Hadjikhani N. Anatomical alterations of the visual motion processing network in migraine with and without aura. PLoS Med. (2006) 3:e402. doi: 10.1371/journal.pmed.0030402
59. Russell MB, Rasmussen BK, Fenger K, Olesen J. Migraine without aura and migraine with aura are distinct clinical entities: a study of four hundred and eighty-four male and female migraineurs from the general population. Cephalalgia. (1996) 16:239–45. doi: 10.1046/j.1468-2982.1996.1604239.x
60. Dumesic DA, Hoyos LR, Chazenbalk GD, Naik R, Padmanabhan V, Abbott DH. Mechanisms of intergenerational transmission of polycystic ovary syndrome. Reproduction. (2020) 159:R1–R13. doi: 10.1530/REP-19-0197
Keywords: migraine, twins, heritability, sex-differences, hormones
Citation: Fitzgerald MC, Saelzler UG and Panizzon MS (2021) Sex Differences in Migraine: A Twin Study. Front. Pain Res. 2:766718. doi: 10.3389/fpain.2021.766718
Received: 29 August 2021; Accepted: 19 November 2021;
Published: 16 December 2021.
Edited by:
Till Sprenger, Deutsche Klinik für Diagnostik, GermanyReviewed by:
James Russell Couch, University of Oklahoma Health Sciences Center, United StatesJ. Ivan Lopez, Marshall University, United States
Copyright © 2021 Fitzgerald, Saelzler and Panizzon. This is an open-access article distributed under the terms of the Creative Commons Attribution License (CC BY). The use, distribution or reproduction in other forums is permitted, provided the original author(s) and the copyright owner(s) are credited and that the original publication in this journal is cited, in accordance with accepted academic practice. No use, distribution or reproduction is permitted which does not comply with these terms.
*Correspondence: Matthew S. Panizzon, bXNwYW5penpvbiYjeDAwMDQwO2hlYWx0aC51Y3NkLmVkdQ==