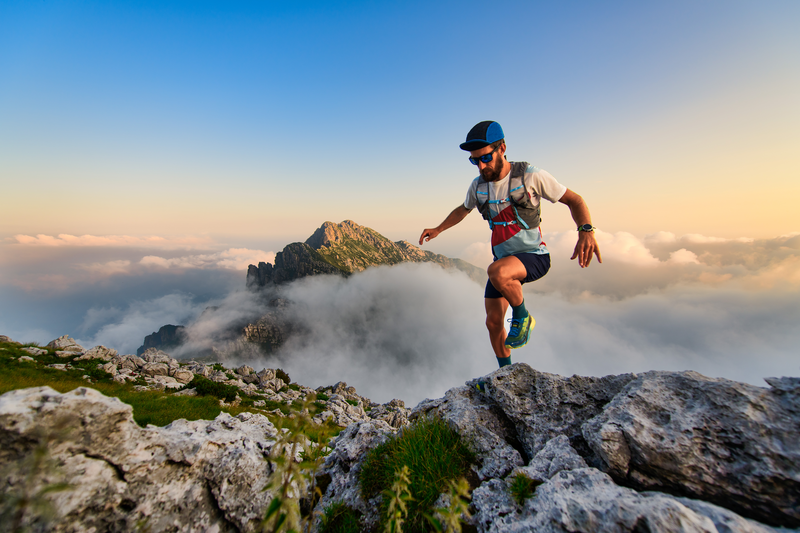
94% of researchers rate our articles as excellent or good
Learn more about the work of our research integrity team to safeguard the quality of each article we publish.
Find out more
OPINION article
Front. Ophthalmol. , 27 September 2024
Sec. Oculoplastics, Orbit and Trauma
Volume 4 - 2024 | https://doi.org/10.3389/fopht.2024.1478805
This article is part of the Research Topic Thyroid eye diseases View all 3 articles
Iodinated contrast media (ICM) is known to trigger thyroid dysfunction and thyrotoxicosis in both euthyroid and susceptible individuals (1–3). Despite this, there are few guidelines for screening and management of ICM administration in patients at risk for thyroid dysfunction (4, 5). In addition, the induction and exacerbation of thyroid orbitopathy are frequently overlooked as a sequelae of thyroid dysfunction. As examples of this, in the American Academy of Ophthalmology Basic and Clinical Science Course used by ophthalmology trainees, the computed tomography (CT) scan used to demonstrate the radiographic findings of thyroid eye disease (TED) is one with contrast enhancement (6). In another resource that is widely utilized by ophthalmology trainees and attendings to study for oral boards, Case 84 dictates the use of a CT scan with contrast to evaluate a patient with suspected TED (7). Understanding the pathophysiology of ICM in susceptible individuals as well as its effects on TED will ideally lead to improved patient outcomes.
All iodinated contrast agents contain a tri-iodinated benzene ring (8). The ability of the contrast agent to attenuate x-rays is based upon the number of iodine molecules present and the type of x-ray utilized (8). The most common routes of ICM administration include intravascular, enteric, and direct injection for imaging techniques including, but not limited to computed tomography, fluoroscopy, angiography, and venography. In general, the majority of patients receive 50-100 mL of contrast per CT scan, with higher doses required for more invasive procedures such as cardiac catheterizations and percutaneous coronary interventions (which can range from a fixed volume of 125 mL, a weight-based dose of 3 mL/kg, to a max volume of 300 mL) (2, 9). A typical CT scan provides 2,500 - 5,000 μg of bioavailable free iodine and 15 - 60 gm of total iodine (1, 2, 10). Non-bioavailable iodine can then be liberated to free iodide. Thus iodine stores may remain elevated for up to 4-8 weeks following ICM administration (2, 3). This is in significant excess when compared to the daily recommended iodine intake of 150 μg for an average adult (11).
In the thyroid gland, iodide is actively transported intracellularly into thyroid follicles through the sodium-iodide symporter (NIS), and used for the synthesis of thyroid hormone, thyroxine (T4) and triiodothyronine (T3). With excess iodine exposure, a transient decrease of thyroid hormone production occurs, also known as the Wolff-Chaikoff effect (11). This is due to formation of iodopeptides which inhibit thyroid peroxidase (TPO) activity, an enzyme involved in thyroid hormone production (2). Adaptation or escape from the Wolff-Chaikoff phenomenon occurs as a result of decreased expression of NIS, through downregulation of mRNA and protein production. As a result, less iodide is able to enter into the thyroid follicle (12). The majority of patients return to a euthyroid state within 48 hours to 4 weeks (1, 2).
However, patients with impaired autoregulation are at risk of iodine-induced hyperthyroidism or thyrotoxicosis, also known as the Jod-Basedow phenomenon (2, 10). Known risk factors for iodine-induced thyroid dysfunction include Graves’ disease (even with euthyroid or latent disease), Hashimoto’s thyroiditis, an autonomous thyroid nodule, nontoxic diffuse or nodular goiter, neonates or fetuses, patients with impaired renal function, individuals taking potential goitrogens (e.g. lithium), or a history of one of the following: long-standing iodine deficiency, prior thyroid surgery, subacute or postpartum thyroiditis, Type 2 amiodarone-induced thyrotoxicosis, and/or interferon-alpha therapy (2, 10). Both NIS dysfunction and presence of TPO autoantibodies have been implicated in iodine-induced hyperthyroidism (2). Case control studies have demonstrated an increased risk of thyroid dysfunction up to 9 months post ICM exposure (2, 3).
Thyroid eye disease (TED) is associated with autoimmune thyroid dysfunction, and is a risk factor for iodine-induced thyroid dysfunction (13). There is a growing body of evidence suggesting that higher thyroid antibody titers, which can result from increased thyroid stimulation (e.g. from the Jod-Basedow phenomenon), are associated with more severe and progressive thyroid orbitopathy (14, 15). On the other hand, the presence of thyroid peroxidase or thyroglobulin autoantibodies has been suggested to reduce thyroid dysfunction following ICM exposure (3). While the precise relationship between iodine-induced thyroid dysfunction and thyroid autoantibodies requires further investigation, it is interesting to note that NIS and TPO, enzymes whose dysregulation has been implicated in the Jod-Basedow phenomenon, are expressed in orbital fibrocytes (16). Despite the undetermined effect of excess iodine on orbital fibroblasts, the local effects of ICM on the NIS and autoantibodies targeting orbital tissues may present a mechanistic explanation for susceptible patients who experience an exacerbation of TED following ICM.
In the authors’ large tertiary referral practices, we have noticed that TED patients exposed to ICM are more likely to present with significant worsening of their orbitopathy within 8-12 weeks of exposure. A case series describing this temporal relationship is provided in Table 1, with Figure 1 (Case 2) demonstrating the dramatic exacerbation of TED symptoms following ICM. All patients had known medical diagnosis of thyroid dysfunction and/or thyroid eye disease, or were undergoing evaluation for suspected TED. None of the patients with known thyroid dysfunction were counseled on the risk of iodine-induced hyperthyroidism or received prophylactic steroids at the time of ICM exposure. Baseline mean thyroid stimulating immunoglobulin (TSI), available in two patients, was 2.17 IU/L (Normal < 0.10 IU/L). TSI at time of presentation following ICM exposure was elevated in the all 4 patients with available bloodwork results. Mean TSI was 10.39 IU/L, but was skewed by one patient with TSI of 39.2 IU/L. Our case series is limited by a small subset of patients; in our experience, many such patients experience an increase in anti-thyroid antibody titers following ICM exposure. For patients who have undergone thyroidectomy (e.g. Case 1), it is unclear whether the orbitopathy flare-up is related to stimulation of a miniscule amount of thyroid tissue versus a direct effect on orbital fibroblasts.
Figure 1. A 43-year-old woman (Case 2) presents with Graves’ disease (A). Following a facial CT scan with iodine contrast, she developed exacerbation of her left-sided periorbital edema, erythema, strabismus and proptosis (7 mm), with new-onset relative afferent pupillary defect (B).
Our experience reported herein suggests that the presence of, or risk for developing thyroid eye disease is a contraindication for administration of ICM. The worsening orbitopathy observed in our series did not appear to correlate with serum auto-antibody level, although our series is small and auto-antibody testing was not performed universally and consistently. Hence, we speculate that the effects on orbital tissues may be the result of iodine interacting directly with orbital fibroblasts. Given our observations, we recommend updating the teaching materials in the field of Ophthalmology to remove recommendations for ICM administration in evaluation of patients suspected of manifesting thyroid eye disease (6, 7).
For ophthalmologists who take care of patients with thyroid eye disease, knowledge of both the Wolff-Chaikoff and Jod-Basedow phenomenon is important. Patients should be cautioned against the elective use of iodinated contrast media and/or the risk of orbitopathy exacerbation following ICM administration. In evaluating patients with suspected TED, a CT scan without contrast can be used to evaluate extraocular muscle size, the orbital apex and optic nerve relationship, as well as for perioperative planning. If contrast is deemed important, we recommend that magnetic resonance imaging (MRI) with gadolinium contrast would be a safer option.
Practitioners should be aware of other common sources of excess iodine, including iodine-containing medications (e.g. amiodarone, potassium iodide), and dietary supplements (e.g. kelp) (11). Ophthalmologists should also be aware of the risk of systemic absorption of iodine from topical povidone-iodine, which has been reported to result in thyroid dysfunction after application to mucosal surfaces and large surgical wounds (11).
In patients at high risk for developing iodine-induced hyperthyroidism, management remains controversial. Antithyroid medications (methimazole or perchlorate) can be considered prophylactically prior to ICM administration in high-risk patients (5). Extrapolating from studies focused on preventing thyroid orbitopathy activation following radioactive iodine (RAI) and adverse reactions to iodine, patients can also be offered corticosteroid premedication and/or a 4-6 week steroid taper following ICM exposure (17–19). While routine thyroid function testing (i.e. serum TSH) is not generally recommended, TSH monitoring can be obtained 3-4 weeks after ICM exposure in patients at higher risk of thyroid dysfunction, such as elderly patients and patients with unstable cardiovascular disease (5).
If iodine-induced changes are suspected, confirmatory testing may include elevated iodine concentration in a serum or 24-hour urine specimen (2, 5). Depending on the clinical scenario, additional workup can include serum thyroid antibody panel, thyroid ultrasonography, and thyroid scintigraphy (5). For thyrotoxicosis, systemic therapy may include initiation of beta-adrenergic blockers and anti-thyroid medications. The use of oral sodium or potassium perchlorate may further be utilized to inhibit the sodium-iodide symporter, as well as lithium for its thyroid inhibitory effects. Patients may require multispecialty care in conjunction with endocrinology for close monitoring of thyroid function studies, as well as careful observation of TED. In our case series, our patients required additional intervention due to vision-threatening symptoms, including systemic steroids, IGF-1R antagonist therapy, and urgent orbital decompression surgery.
Our case series is limited by a small patient number, its retrospective nature, and lack of consistency in obtaining before/after serum bloodwork. A larger cohort study with analysis of pre- and post- ICM clinical exam findings, thyroid hormone levels, and thyroid autoantibody blood work would be required to further investigate this relationship. Given the variability in thyroid eye disease presentation, a study would need to be adequately powered to account for the diversity in clinical findings. Our goal for this publication is to stimulate provider awareness and encourage future study.
In summary, the totality of published data and our clinical experiences in high-volume TED practices suggest that ICM administration is a risk factor for flare-up of thyroid orbitopathy, whether or not the autoimmune thyroiditis is active. It is unclear whether the mechanism of this effect is indirect through the thyroid tissue or direct through orbital fibroblasts. Future studies are required to better understand the basic and clinical science of this phenomenon.
JS: Writing – original draft, Writing – review & editing. BS: Writing – original draft, Writing – review & editing. AK: Writing – original draft, Writing – review & editing.
The author(s) declare that no financial support was received for the research, authorship, and/or publication of this article.
AK is a consultant to BioTissue Inc. AK conducts research on thyroid eye disease with support from industry, not relevant to this study.
The remaining authors declare that the research was conducted in the absence of any commercial or financial relationships that could be construed as a potential conflict of interest.
The author(s) declared that they were an editorial board member of Frontiers, at the time of submission. This had no impact on the peer review process and the final decision.
All claims expressed in this article are solely those of the authors and do not necessarily represent those of their affiliated organizations, or those of the publisher, the editors and the reviewers. Any product that may be evaluated in this article, or claim that may be made by its manufacturer, is not guaranteed or endorsed by the publisher.
1. van der Molen AJ, Thomsen HS, Morcos SK, Contrast Media Safety Committee, European Society of Urogenital Radiology (ESUR). Effect of iodinated contrast media on thyroid function in adults. Eur Radiol. (2004) 14:902–7. doi: 10.1007/s00330-004-2238-z
2. Lee SY, Rhee CM, Leung AM, Braverman LE, Brent GA, Pearce EN. A review: Radiographic iodinated contrast media-induced thyroid dysfunction. J Clin Endocrinol Metab. (2015) 100:376–83. doi: 10.1210/jc.2014-3292
3. Rhee CM, Bhan I, Alexander EK, Brunelli SM. Association between iodinated contrast media exposure and incident hyperthyroidism and hypothyroidism. Arch Intern Med. (2012) 172:153–9. doi: 10.1001/archinternmed.2011.677
4. Rusandu A, Sjøvold BH, Hofstad E, Reidunsdatter RJ. Iodinated contrast media and their effect on thyroid function - Routines and practices among diagnostic imaging departments in Norway. J Med Radiat Sci. (2020) 67:111–8. doi: 10.1002/jmrs.v67.2
5. Bednarczuk T, Brix TH, Schima W, Zettinig G, Kahaly GJ. European thyroid association guidelines for the management of iodine-Based contrast media-Induced thyroid dysfunction. Eur Thyroid J. (2021) 10:269–84. doi: 10.1159/000517175
6. Korn BS, Burkat CN, Couch SM, Ediriwickrema LS, Lee BW, Lee NG. Oculofacial plastic and orbital surgery. Am Acad Ophthalmol. (2023). 7, 60
7. Pemberton JD, Daniels AB, Fay A. Ophthalmology Clinical Vignettes: Oral Exam Study Guide II. Little Rock, ARK: Crimson House Publishing LLC (2015).
8. Pasternak JJ, Williamson EE. Clinical pharmacology, uses, and adverse reactions of iodinated contrast agents: a primer for the non-radiologist. Mayo Clin Proc. (2012) 87:390–402. doi: 10.1016/j.mayocp.2012.01.012
9. Aoun J, Nicolas D, Brown JR, Jaber BL. Maximum allowable contrast dose and prevention of acute kidney injury following cardiovascular procedures. Curr Opin Nephrol Hypertens. (2018) 27:121–9. doi: 10.1097/MNH.0000000000000389
10. Leung AM, Braverman LE. Iodine-induced thyroid dysfunction. Curr Opin Endocrinol Diabetes Obes. (2012) 19:414–9. doi: 10.1097/MED.0b013e3283565bb2
11. Pramyothin P, Leung AM, Pearce EN, Malabanan AO, Braverman LE. Clinical problem-solving. A hidden solution. N Engl J Med. (2011) 365:2123–7. doi: 10.1056/NEJMcps1008908
12. Nikiforov YE, Biddinger PW, Thompson LDR. Diagnostic Pathology and Molecular Genetics of the Thyroid: A Comprehensive Guide for Practicing Thyroid Pathology. Philadelphia, PA: Lippincott Williams & Wilkins (2012).
14. Diana T, Kahaly GJ. Thyroid stimulating hormone receptor antibodies in thyroid eye disease—Methodology and clinical applications. Ophthalmic Plast Reconstructive Surgery. (2018) 34:S13. doi: 10.1097/IOP.0000000000001053
15. Ponto KA, Kanitz M, Olivo PD, Pitz S, Pfeiffer N, Kahaly GJ. Clinical relevance of thyroid-stimulating immunoglobulins in graves’ ophthalmopathy. Ophthalmology. (2011) 118:2279–85. doi: 10.1016/j.ophtha.2011.03.030
16. Fernando R, Lu Y, Atkins SJ, Mester T, Branham K, Smith TJ. Expression of thyrotropin receptor, thyroglobulin, sodium-iodide symporter, and thyroperoxidase by fibrocytes depends on AIRE. J Clin Endocrinol Metab. (2014) 99:E1236–44. doi: 10.1210/jc.2013-4271
17. Shiber S, Stiebel-Kalish H, Shimon I, Grossman A, Robenshtok E. Glucocorticoid regimens for prevention of Graves’ ophthalmopathy progression following radioiodine treatment: systematic review and meta-analysis. Thyroid. (2014) 24:1515–23. doi: 10.1089/thy.2014.0218
18. Lai A, Sassi L, Compri E, Marino F, Sivelli P, Piantanida E, et al. Lower dose prednisone prevents radioiodine-associated exacerbation of initially mild or absent graves’ orbitopathy: a retrospective cohort study. J Clin Endocrinol Metab. (2010) 95(3):1333–7. doi: 10.1210/jc.2009-2130
Keywords: thyroid eye disease, graves’ disease, iodine contrast, orbital inflammation, orbitopathy, graves orbitopathy, computed tomography, iodinated contrast media
Citation: Spadaro JZ, Simmons BA and Kahana A (2024) Iodine contrast should be avoided in patients with thyroid eye disease. Front. Ophthalmol. 4:1478805. doi: 10.3389/fopht.2024.1478805
Received: 10 August 2024; Accepted: 09 September 2024;
Published: 27 September 2024.
Edited by:
Mohammad Javed Ali, L. V. Prasad Eye Institute, IndiaReviewed by:
Ofira Zloto, Sheba Medical Center, IsraelCopyright © 2024 Spadaro, Simmons and Kahana. This is an open-access article distributed under the terms of the Creative Commons Attribution License (CC BY). The use, distribution or reproduction in other forums is permitted, provided the original author(s) and the copyright owner(s) are credited and that the original publication in this journal is cited, in accordance with accepted academic practice. No use, distribution or reproduction is permitted which does not comply with these terms.
*Correspondence: Alon Kahana, YWthaGFuYUBtaW9jdWxvcGxhc3RpY3MuY29t
Disclaimer: All claims expressed in this article are solely those of the authors and do not necessarily represent those of their affiliated organizations, or those of the publisher, the editors and the reviewers. Any product that may be evaluated in this article or claim that may be made by its manufacturer is not guaranteed or endorsed by the publisher.
Research integrity at Frontiers
Learn more about the work of our research integrity team to safeguard the quality of each article we publish.