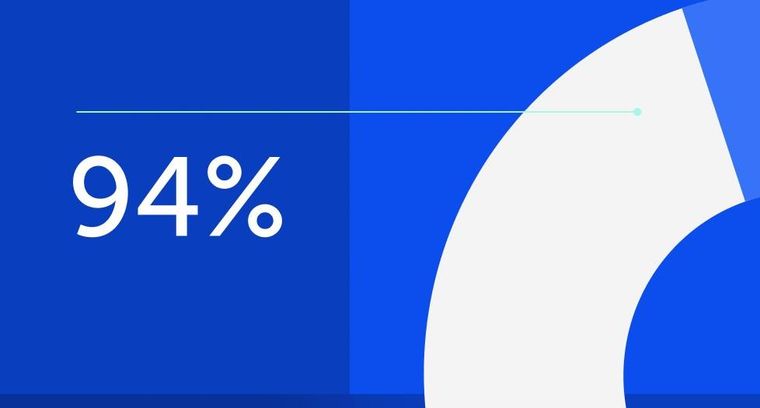
94% of researchers rate our articles as excellent or good
Learn more about the work of our research integrity team to safeguard the quality of each article we publish.
Find out more
MINI REVIEW article
Front. Ophthalmol., 20 July 2023
Sec. Pediatric Ophthalmology and Strabismus
Volume 3 - 2023 | https://doi.org/10.3389/fopht.2023.1233866
This article is part of the Research TopicInsights in Pediatric Ophthalmology and Strabismus: 2022View all 8 articles
Strabismus, or misalignment of the eyes, is the most common ocular disorder in the pediatric population, affecting approximately 2%–4% of children. Strabismus leads to the disruption of binocular vision, amblyopia, social and occupational discrimination, and decreased quality of life. Although it has been recognized since ancient times that strabismus runs in families, its inheritance patterns are complex, and its precise genetic mechanisms have not yet been defined. Family, population, and twin studies all support a role of genetics in the development of strabismus. There are multiple forms of strabismus, and it is not known if they have shared genetic mechanisms or are distinct genetic disorders, which complicates studies of strabismus. Studies assuming that strabismus is a Mendelian disorder have found areas of linkage and candidate genes in particular families, but no definitive causal genes. Genome-wide association studies searching for common variation that contributes to strabismus risk have identified two risk loci and three copy number variants in white populations. Causative genes have been identified in congenital cranial dysinnervation disorders, syndromes in which eye movement is limited or paralyzed. The causative genes lead to either improper differentiation of cranial motor neurons or abnormal axon guidance. This article reviews the evidence for a genetic contribution to strabismus and the recent advances that have been made in the genetics of comitant strabismus, the most common form of strabismus.
Strabismus is the most common ocular disorder in children and can have lifelong consequences, including amblyopia, loss of binocular vision, decreased quality of life (for both children and parents), and social and occupational discrimination (1–7). Strabismus is classified by the alignment of the eyes relative to each other. When one eye is fixating, the other eye can deviate inwards (esotropia), outwards (exotropia), or vertically (hyper/hypotropia). Deviations can be the same in all positions of gaze (comitant strabismus) or vary based on eye position (incomitant strabismus). There are a group of rare syndromes (congenital cranial dysinnervation disorders, CCDDs) with incomitant, paralytic strabismus, which show typical Mendelian inheritance patterns, in which causative genes have been identified (8). Those identified genes influence cranial motor neuron development and affected patients and model organisms have abnormal development of the cranial nerves (9–13). This review focuses on the evidence for genetic contributions to the more common forms of comitant strabismus, in which both eyes are able to move fully. It has long been recognized that strabismus runs in families, and population, family, and twin studies all support a genetic contribution, but inheritance patterns are complex, and no definitive causative genes have been identified (14–20).
Genetic variants vary in both frequency (the prevalence in the population) and penetrance (the proportion of individuals with the genetic variant who have the disease). Mendelian disorders are usually caused by rare variants of high penetrance, whereas common diseases often have contributions of many common variants, each with relatively low penetrance (21). Different study techniques are used to identify these different types of variants, and both types of study have been performed in strabismus, which are detailed below.
The prevalence of strabismus is approximately 2%–4% in the pediatric population (22–24), but the prevalence varies based on geographical regions and strabismus subtypes. A meta-analysis of 56 prevalence studies showed that the highest prevalence of esotropia and total strabismus was in the Americas and Europe, but that there was a higher prevalence of exotropia in Asia, especially China, and in Australia (25). A recent study in Hong Kong of ethnically Chinese children found a prevalence of strabismus of 3.1%, with an exotropia to esotropia ratio of 9.75 : 1 (26). Within the United States, the Multiethnic Pediatric Eye Disease study showed differences in the proportion of esotropia and exotropia between ethnic groups. Non-Hispanic white children had higher rates of esotropia than exotropia, whereas in African American, Asian, and Hispanic children exotropia was more common than esotropia (23, 27). The Baltimore Pediatric Eye Disease study, in contrast, reported that both white and black children had equal rates of esotropia and exotropia (24). Multiple other studies have reported that there is a higher prevalence of esotropia than exotropia in the white population (28–32), suggesting that population genetic differences contribute to the risk of esotropia in particular.
Strabismus has both genetic and environmental risk factors. In addition to family history, non-ocular strabismus risk factors include low birth weight, prematurity, maternal smoking during pregnancy, intrauterine drug exposure, neurologic diseases, and advanced maternal age (26, 33–38). Ocular risk factors include hyperopia (>2 D), and moderate anisometropia (26, 39), which likely have genetic risk factors. There is no significant difference in the incidence of strabismus between genders (40). Interestingly, several of these environmental risk factors have been associated with epigenetic changes, specifically changes in methylation, in the newborn population. Maternal smoking, birthweight, prematurity, and greater maternal age are all independently associated with altered methylation (41–44). Methylation patterns affect gene expression, suggesting that there is a possible mechanistic link between genetic and environmental risk factors for strabismus.
Hippocrates first noted that strabismus tends to run in families; he observed: “Children of parents having distorted eyes squint also for the most part” (45). Although genetic risk clearly plays a role in the development of strabismus (16), it is unknown whether esotropia, exotropia, and vertical deviations result from unique genetic risk factors, or if a common genetic risk underlies all forms of strabismus. In most families, all affected individuals have the same general type of strabismus (esotropia vs. exotropia), but there are examples of families that are discordant (46–49). It is clear that there is not one single gene that accounts for all strabismus cases.
Twin studies support there being a strong genetic contribution, particularly for esodeviations (20). Monozygotic twins have concordance rates for any strabismus of 54%–82%, while dizygotic twins have concordance rates of 14%–47% (19, 50). Several family studies have shown a high prevalence of strabismus among family members of a proband with strabismus (15, 51–53). Combining 12 previous family studies, Paul and Hardage (16) reported that 30.6% of strabismus patients had a close relative with strabismus. A more recent study reported that 18.7% of strabismus cases had at least one affected first-degree relative (54). The relative risk for first-degree relatives of an affected proband is estimated to be between 3 and 5 (14, 16–19). The heritability factor remains significant following correction for the environmental risk factors identified above (17).
In large strabismus pedigrees in which strabismus is inherited in apparently Mendelian patterns, several studies have used linkage analysis to identify potential loci associated with strabismus (Table 1). A locus on 7p22.1 (STBM1) was mapped in one large family, under a recessive model, although inheritance appeared to be dominant (18). This locus was replicated in another family under a dominant model (55). By combining Japanese families, linkage was shown at 4q28.22 in a dominant model and 7q31.2 in a recessive model (56). MGST2 and WNT2 have been proposed as candidate genes at these loci, but specific variants have not been reported (61). In one large family, with variable strabismus phenotypes between family members, linkage was shown at 14q12; the top candidate variant is a non-coding 4 bp deletion near FOXG1 (59). There have also been two studies in large consanguineous families in which some individuals had infantile esotropia and others had Duane retraction syndrome. The first study identified a recessive 6MB band on chromosome 16p13.12-p12.3 (57); the other study identified two potential loci (3p26.3–26.2 and 6q24.2–25.1) in a family with three siblings with esotropia and four with Duane retraction syndrome (58). In one large Chinese family, linkage was identified at 2q22.3–2q32.1, and exome sequencing identified a rare heterozygous variant in LRP2 within the area of linkage. An additional rare variant in the same gene was identified in an unrelated family (60). Exome analysis of another Chinese family, in which the proband, both parents, and a maternal grandfather had exotropia, identified several candidate variants, but no functional evidence was presented for any of the variants (62). Another study reported an exome analysis of 18 strabismus families. In five families, a potential risk variant—defined as a rare, predicted deleterious variant that segregated with disease—was identified. The genes with potential risk variants are FAT3, KCNH2, CELSR1, and TTYH1 (63). Since each of these studies has identified different potential risk variants in different families, it is clear that there is significant genetic heterogeneity in strabismus.
Diseases that are common in the population often have multiple genes that contribute, with each individual variant having a small effect size. In search for common variations contributing to the risk of strabismus, two genome-wide association studies (with different inclusion criteria) have each reported one risk allele (64, 65). A GWAS study of non-accommodative esotropia, including 826 patients of European ancestry from the USA in the discovery cohort and 689 patients from Australia and the United Kingdom in the replication cohort, identified one risk allele, a functional single nucleotide polymorphism (SNP) in an intron of the WRB gene on chromosome 21 at rs2244352, which affects the expression of WRB and neighboring genes (64). The area is imprinted, and the risk allele is preferentially inherited paternally (64). A second GWAS, including 1,345 individuals with self-reported strabismus in the UK Biobank, identified a locus on chromosome 17q25, with lead SNP within TSPAN10 (65). The 17q25 locus extends across the NPLOC4–TSPAN10–PDE6G gene cluster, which has been associated through GWAS with several other eye conditions, including macular thickness (66), astigmatism (67), retinal microvascular size (68), and myopia (69). Phenotyping in the UK Biobank was based on self-reporting by participants aged between 37 and 73. Specifically, participants reported whether they wore glasses and, if they did, were asked to select the reason(s). Participants who chose “squint or turn in an eye since childhood (called strabismus)” were classified as having strabismus. This has the potential to select for accommodative esotropia (and associated hyperopia) or to misclassify participants with strabismus as controls if they do not wear glasses for the condition. The strabismus group was more hyperopic than the control group, but the association between strabismus and the 17q25 locus was independent of refractive error. The association with this locus was replicated in a cohort of 7-year-olds examined by an orthoptist and determined to have strabismus. In that population of 5,200 children (of whom 145 had strabismus), the lead variant was associated with any strabismus and exotropia, but not with esotropia independently. The authors note, however, that some of the exotropic children might have been exotropic following surgery for esotropia and that the number of exotropic children was quite small (only 28) (65). The association of each of these alleles with strabismus was replicated in the FinnGenn cohort, in which patients were phenotyped based on electronic health record data. In the Finnish cohort, which comprised 3,515 cases of all subtypes of strabismus (including convergent, divergent, paralytic, and vertical strabismus, accommodative esotropia, intermittent heterotropia, and others) and 173,384 controls, the WRB polymorphism was associated with “any strabismus” and “divergent strabismus”, and the TSPAN10 polymorphism was associated with “any strabismus”, “convergent strabismus”, and “divergent strabismus” (70). Overall, the data indicate that these two polymorphisms contribute significantly to the population-attributable risk of strabismus, but more GWAS studies, with larger sample sizes and consistent phenotyping, are needed (71).
Additional sources of genetic variation in the population are copy number variants (CNVs) and structural variants. CNVs are duplications or deletions of large regions of DNA, whereas structural variants are rearrangements of the DNA, such as inversions, insertion of sequence from one chromosome to another, or translocation between chromosomes. CNVs represent an important source of genetic variation and are also a force that shapes genome evolution (72, 73). Many common CNVs represent benign polymorphisms, but rare CNVs at specific loci contribute to disease. CNVs contribute to the genetic risk for several neuropsychiatric disorders with complex inheritance (74), including intellectual disability (75), autism spectrum disorder (76, 77), major depressive disorder (78), attention-deficit hyperactivity disorder (79), and Tourette’s syndrome (80). In strabismus, one study has reported both a larger number of CNVs in esotropia samples and three specific duplications that significantly increase the risk of esotropia (81). They compared 1,614 white individuals with isolated esotropia to 3,992 ethnically matched control individuals, all genotyped on Illumina Omni SNP arrays, and used two hidden Markov model (hmm) CNV-calling algorithms, PennCNV (82) and QuantiSNP (83), to identify CNVs. Three rare, recurrent duplications were significantly (p <1 × 10–6) more common in esotropia patients. A 23 kb duplication at 4p15.2 includes exon 1 of the uncharacterized lncRNA LOC101929161 (also known as lnc-SEL1L3–2) and shows conservation with monkeys, but not other animals. The duplication on chromosome 2p11.2 spans the lncRNA CYTOR and microRNA miR4435, contains several putative regulatory regions, and has areas with conservation among mammals but not other vertebrates. The duplication on chromosome 10q11.22 spans two lncRNAs: LINC00842 and LOC105378577; three protein-coding genes: ANTXRL (anthrax toxin receptor-like), ANXA8L1 (annexin A8 like 1), and NPY4R (neuropeptide Y receptor 4); and three transcribed pseudogenes: ANTXRLP1, FAM25BP, and HNRNPA1P33. Each duplication substantially increased esotropia risk [OR 11.1 (95% CI 4.6–25.2), OR 14.16 (95% CI 5.4–38.1), and OR 8.96 (95% CI 5.4–14.9), respectively]. Further study to identify the mechanisms by which these duplications contribute to strabismus is ongoing.
There are many reports of copy number variants associated with neurodevelopmental disorders that include strabismus (see section 9). In neurotypical patients, two cases who presented with Kallmann syndrome, X-linked ichthyosis, and strabismus, who have partially overlapping deletions on the X chromosome, have been reported. The deletion of ANOS1 and STS explains the Kallmann syndrome and X-linked ichthyosis, respectively. The other four genes deleted in both patients are NLGN4X, VCX-A, PUDP, and PNPLA4. Neither patient had an intellectual disability or developmental delay (84).
To date, the only causal genes for strabismus have been found in CCDDs. Patients with ocular CCDDs have paralysis of one more extraocular muscles, due to abnormalities of neuronal differentiation or axon guidance of the corresponding cranial motor nerve (85). Congenital fibrosis of the extraocular muscles (CFEOM) affects cranial nerves 3 and 4 and is caused by autosomal dominant missense variants in KIF21A, TUBB3, TUBB2B or TUBA1A or autosomal recessive variants in PHOX2A (9, 10, 12, 86, 87). Duane retraction syndrome affects cranial nerve 6, leading to limited abduction of one or both eyes. Although most cases do not have an identifiable genetic cause, autosomal dominant variants in CHN1 or MAFB can cause isolated Duane syndrome (11, 88), and variants in SALL4 cause Duane-radial ray syndrome (Duane syndrome plus abnormalities of forearm development) (89). Duane syndrome is also a feature of Athabascan brain dysgenesis syndrome (ABDS)/Bosley–Salih–Alorainy syndrome (BSAS), caused by autosomal recessive variants in HOXA1 (90, 91). Horizontal gaze palsy with progressive scoliosis (HGPPS) involves an inability to abduct or adduct the eyes, and results from autosomal recessive variants in ROBO3 (92). HOXB1 variants result in a syndrome that includes facial paresis and esotropia with full eye movements (93). It is clear, however, that comitant strabismus results from different mechanisms than the CCDDs, since patients with comitant strabismus have full motility and normal cranial motor nerve function and appearance on MRI.
Although comitant strabismus is most often an isolated ocular disorder, it is also a common feature of many genetic syndromes and neurodevelopmental disorders (16, 94). The incidence of strabismus in individuals with Down syndrome is 33% (95); strabismus has also been reported with many other chromosomal disorders associated with intellectual disability, including partial trisomy 7q (96, 97), partial trisomy 10p (98), and duplication 13q (99). Individuals with intellectual disability of any cause are significantly more likely to have strabismus than the general population; the odds ratio is 5.46 (100). The incidence of strabismus in individuals with autism spectrum disorder is also increased (8%–22%) (101–104). Ye et al. (105) compiled a list of 233 genes potentially involved in strabismus that was based on strabismus being a feature of their associated genetic syndrome. Analysis of these potential genes reveals their preferential expression in the cerebellum, amygdala, and posteroventral parietal cortex, specifically at the fetal and early developmental stages.
Strabismus is also common in ocular disorders that lead to poor vision, including retinal dystrophies, congenital cataract, optic nerve hypoplasia, and albinism (106–109). Poor vision contributes to the development of strabismus in these disorders, but it is also possible that the genetic changes that lead to retinal, optic nerve, or lens maldevelopment or dysfunction affect the oculomotor system.
Although strabismus has a clear heritability, its genetic mechanisms are complex and heterogeneous. Several linked loci and candidate genes have been identified in individual families, but, thus far, exome sequencing has not revealed definitive causative variants present in significant proportions of strabismus patients. Across the population, two loci have been identified by GWAS as increasing the risk of strabismus, each with a fairly small effect size. Three CNVs increase the risk of strabismus; they have large ORs but are rare. Many neurodevelopmental disorders include strabismus; one hypothesis is that isolated strabismus may result from altered regulation of genes whose loss of function causes more severe neurologic dysfunction. Altered genetic regulation may be a common mechanism that links all the risk factors for strabismus. CNVs may alter gene regulation by disrupting the proximity of enhancers and silencers to promoters, the SNPs identified by GWAS affect the regulation of nearby genes, and the environmental risk factors affect epigenetic methylation, which in turn affects gene regulation. Further study and improved methods of identifying variants in regulatory regions are needed to fully understand the genetic causes of strabismus.
MMS and MCW wrote and edited the manuscript. All authors contributed to the article and submitted and approved the submitted section.
Supported by the National Eye Institute (5R01EY032539), Boston Children’s Hospital Translational Research Program, and the Children’s Hospital Ophthalmology Foundation.
The authors declare that the research was conducted in the absence of any commercial or financial relationships that could be construed as a potential conflict of interest.
The author MCW declared that they were an editorial board member of Frontiers, at the time of submission. This had no impact on the peer review process and the final decision.
All claims expressed in this article are solely those of the authors and do not necessarily represent those of their affiliated organizations, or those of the publisher, the editors and the reviewers. Any product that may be evaluated in this article, or claim that may be made by its manufacturer, is not guaranteed or endorsed by the publisher.
1. Paysse EA, Steele EA, Mccreery KM, Wilhelmus KR, Coats DK. Age of the emergence of negative attitudes toward strabismus. J AAPOS (2001) 5:361–6. doi: 10.1067/mpa.2001.119243
2. Uretmen O, Egrilmez S, Kose S, Pamukcu K, Akkin C, Palamar M. Negative social bias against children with strabismus. Acta Ophthalmol Scand (2003) 81:138–42. doi: 10.1034/j.1600-0420.2003.00024.x
3. Mojon-Azzi SM, Mojon DS. Strabismus and employment: the opinion of headhunters. Acta Ophthalmol (2009) 87:784–8. doi: 10.1111/j.1755-3768.2008.01352.x
4. Davidson S, Quinn GE. The impact of pediatric vision disorders in adulthood. Pediatrics (2011) 127:334–9. doi: 10.1542/peds.2010-1911
5. Wen G, Mckean-Cowdin R, Varma R, Tarczy-Hornoch K, Cotter SA, Borchert M, et al. General health-related quality of life in preschool children with strabismus or amblyopia. Ophthalmology (2011) 118:574–80. doi: 10.1016/j.ophtha.2010.06.039
6. Schuster AK, Elflein HM, Pokora R, Schlaud M, Baumgarten F, Urschitz MS. Health-related quality of life and mental health in children and adolescents with strabismus - results of the representative population-based survey KiGGS. Health Qual Life Outcomes (2019) 17:81. doi: 10.1186/s12955-019-1144-7
7. Hatt SR, Leske DA, Castañeda YS, Wernimont SM, Liebermann L, Cheng-Patel CS, et al. Association of strabismus with functional vision and eye-related quality of life in children. JAMA Ophthalmol (2020) 138(5):528–35. doi: 10.1001/jamaophthalmol.2020.0539
8. Whitman MC, Engle EC. Ocular congenital cranial dysinnervation disorders (CCDDs): insights into axon growth and guidance. Hum Mol Genet (2017) 26:R37–44. doi: 10.1093/hmg/ddx168
9. Nakano M, Yamada K, Fain J, Sener EC, Selleck CJ, Awad AH, et al. Homozygous mutations in ARIX(PHOX2A) result in congenital fibrosis of the extraocular muscles type 2. Nat Genet (2001) 29:315–20. doi: 10.1038/ng744
10. Yamada K, Andrews C, Chan WM, Mckeown CA, Magli A, De Berardinis T, et al. Heterozygous mutations of the kinesin KIF21A in congenital fibrosis of the extraocular muscles type 1 (CFEOM1). Nat Genet (2003) 35:318–21. doi: 10.1038/ng1261
11. Miyake N, Chilton J, Psatha M, Cheng L, Andrews C, Chan WM, et al. Human CHN1 mutations hyperactivate alpha2-chimaerin and cause Duane’s retraction syndrome. Science (2008) 321:839–43. doi: 10.1126/science.1156121
12. Tischfield MA, Baris HN, Wu C, Rudolph G, Van Maldergem L, He W, et al. Human TUBB3 mutations perturb microtubule dynamics, kinesin interactions, and axon guidance. Cell (2010) 140:74–87. doi: 10.1016/j.cell.2009.12.011
13. Cheng L, Desai J, Miranda CJ, Duncan JS, Qiu W, Nugent AA, et al. Human CFEOM1 mutations attenuate KIF21A autoinhibition and cause oculomotor axon stalling. Neuron (2014) 82:334–49. doi: 10.1016/j.neuron.2014.02.038
14. Crone RA, Velzeboer CM. Statistics on strabismus in the Amsterdam youth; researches into the origin of strabismus. AMA Arch Ophthalmol (1956) 55:455–70. doi: 10.1001/archopht.1956.00930030459002
15. Dufier JL, Briard ML, Bonaiti C, Frezal J, Saraux H. Inheritance in the etiology of convergent squint. Ophthalmologica (1979) 179:225–34. doi: 10.1159/000308899
16. Paul TO, Hardage LK. The heritability of strabismus. Ophthalmic Genet (1994) 15:1–18. doi: 10.3109/13816819409056905
17. Podgor MJ, Remaley NA, Chew E. Associations between siblings for esotropia and exotropia. Arch Ophthalmol (1996) 114:739–44. doi: 10.1001/archopht.1996.01100130731018
18. Parikh V, Shugart YY, Doheny KF, Zhang J, Li L, Williams J, et al. A strabismus susceptibility locus on chromosome 7p. Proc Natl Acad Sci U.S.A. (2003) 100:12283–8. doi: 10.1073/pnas.2035118100
19. Wilmer JB, Backus BT. Genetic and environmental contributions to strabismus and phoria: evidence from twins. Vision Res (2009) 49:2485–93. doi: 10.1016/j.visres.2009.08.006
20. Sanfilippo PG, Hammond CJ, Staffieri SE, Kearns LS, Melissa Liew SH, Barbour JM, et al. Heritability of strabismus: genetic influence is specific to eso-deviation and independent of refractive error. Twin Res Hum Genet (2012) 15:624–30. doi: 10.1017/thg.2012.22
21. Mccarthy MI, Abecasis GR, Cardon LR, Goldstein DB, Little J, Ioannidis JP, et al. Genome-wide association studies for complex traits: consensus, uncertainty and challenges. Nat Rev Genet (2008) 9:356–69. doi: 10.1038/nrg2344
22. Matsuo T, Matsuo C. The prevalence of strabismus and amblyopia in Japanese elementary school children. Ophthalmic Epidemiol (2005) 12:31–6. doi: 10.1080/09286580490907805
23. Multi-Ethnic Pediatric Eye Disease Study, G. Prevalence of amblyopia and strabismus in African American and Hispanic children ages 6 to 72 months the multi-ethnic pediatric eye disease study. Ophthalmology (2008) 115:1229–1236 e1221. doi: 10.1016/j.ophtha.2007.08.001
24. Friedman DS, Repka MX, Katz J, Giordano L, Ibironke J, Hawse P, et al. Prevalence of amblyopia and strabismus in white and African American children aged 6 through 71 months the Baltimore Pediatric Eye Disease Study. Ophthalmology (2009) 116:2128–2134 e2121-2122.
25. Hashemi H, Pakzad R, Heydarian S, Yekta A, Aghamirsalim M, Shokrollahzadeh F, et al. Global and regional prevalence of strabismus: a comprehensive systematic review and meta-analysis. Strabismus (2019) 27:54–65. doi: 10.1080/09273972.2019.1604773
26. Zhang XJ, Lau YH, Wang YM, Kam KW, Ip P, Yip WW, et al. Prevalence of strabismus and its risk factors among school aged children: The Hong Kong Children Eye Study. Sci Rep (2021) 11:13820. doi: 10.1038/s41598-021-93131-w
27. Mckean-Cowdin R, Cotter SA, Tarczy-Hornoch K, Wen G, Kim J, Borchert M, et al. Prevalence of amblyopia or strabismus in asian and non-Hispanic white preschool children: multi-ethnic pediatric eye disease study. Ophthalmology (2013) 120:2117–24. doi: 10.1016/j.ophtha.2013.03.001
28. Kvarnström G, Jakobsson P, Lennerstrand G. Visual screening of Swedish children: an ophthalmological evaluation. Acta Ophthalmol Scand (2001) 79:240–4. doi: 10.1034/j.1600-0420.2001.790306.x
29. Ohlsson J, Villarreal G, Sjöström A, Cavazos H, Abrahamsson M, Sjöstrand J. Visual acuity, amblyopia, and ocular pathology in 12- to 13-year-old children in Northern Mexico. J aapos (2003) 7:47–53. doi: 10.1016/S1091-8531(02)42011-3
30. Robaei D, Rose KA, Kifley A, Cosstick M, Ip JM, Mitchell P. Factors associated with childhood strabismus: findings from a population-based study. Ophthalmology (2006) 113:1146–53. doi: 10.1016/j.ophtha.2006.02.019
31. Mohney BG. Common forms of childhood strabismus in an incidence cohort. Am J Ophthalmol (2007) 144:465–7. doi: 10.1016/j.ajo.2007.06.011
32. Lança C, Serra H, Prista J. Strabismus, visual acuity, and uncorrected refractive error in portuguese children aged 6 to 11 years. Strabismus (2014) 22:115–9. doi: 10.3109/09273972.2014.932395
33. Hakim RB, Tielsch JM. Maternal cigarette smoking during pregnancy. A risk factor for childhood strabismus. Arch Ophthalmol (1992) 110:1459–62. doi: 10.1001/archopht.1992.01080220121033
34. Chew E, Remaley NA, Tamboli A, Zhao J, Podgor MJ, Klebanoff M. Risk factors for esotropia and exotropia. Arch Ophthalmol (1994) 112:1349–55. doi: 10.1001/archopht.1994.01090220099030
35. Bremer DL, Palmer EA, Fellows RR, Baker JD, Hardy RJ, Tung B, et al. Strabismus in premature infants in the first year of life. Cryotherapy for Retinopathy of Prematurity Cooperative Group. Arch Ophthalmol (1998) 116:329–33. doi: 10.1001/archopht.116.3.329
36. Altintas O, Etus V, Etus H, Ceylan S, Caglar Y. Risk of strabismus and ambylopia in children with hydrocephalus. Graefes Arch Clin Exp Ophthalmol (2005) 243:1213–7. doi: 10.1007/s00417-005-0001-z
37. Torp-Pedersen T, Boyd HA, Poulsen G, Haargaard B, Wohlfahrt J, Holmes JM, et al. In-utero exposure to smoking, alcohol, coffee, and tea and risk of strabismus. Am J Epidemiol (2010) 171:868–75. doi: 10.1093/aje/kwq010
38. Lingham G, Mackey DA, Sanfilippo PG, Mountain J, Hewitt AW, Newnham JP, et al. Influence of prenatal environment and birth parameters on amblyopia, strabismus, and anisometropia. J aapos (2020) 24:74.e71–7. doi: 10.1016/j.jaapos.2019.12.013
39. Schaal LF, Schellini SA, Pesci LT, Galindo A, Padovani CR, Corrente JE. The prevalence of strabismus and associated risk factors in a southeastern region of Brazil. Semin Ophthalmol (2018) 33:357–60. doi: 10.1080/08820538.2016.1247176
40. Roberts J. Eye examination findings among children. United States. Vital Health Stat (1972) 11:1–47.
41. Joubert BR, Felix JF, Yousefi P, Bakulski KM, Just AC, Breton C, et al. DNA methylation in newborns and maternal smoking in pregnancy: genome-wide consortium meta-analysis. Am J Hum Genet (2016) 98:680–96. doi: 10.1016/j.ajhg.2016.02.019
42. Markunas CA, Wilcox AJ, Xu Z, Joubert BR, Harlid S, Panduri V, et al. Maternal age at delivery is associated with an epigenetic signature in both newborns and adults. PloS One (2016) 11:e0156361. doi: 10.1371/journal.pone.0156361
43. Küpers LK, Monnereau C, Sharp GC, Yousefi P, Salas LA, Ghantous A, et al. Meta-analysis of epigenome-wide association studies in neonates reveals widespread differential DNA methylation associated with birthweight. Nat Commun (2019) 10:1893. doi: 10.1038/s41467-019-09671-3
44. Merid SK, Novoloaca A, Sharp GC, Kupers LK, Kho AT, Roy R, et al. Epigenome-wide meta-analysis of blood DNA methylation in newborns and children identifies numerous loci related to gestational age. Genome Med (2020) 12:25. doi: 10.1186/s13073-020-0716-9
46. Schlossman A, Priestley BS. Role of heredity in etiology and treatment of strabismus. AMA Arch Ophthalmol (1952) 47:1–20. doi: 10.1001/archopht.1952.01700030004001
47. Maumenee IH, Alston A, Mets MB, Flynn JT, Mitchell TN, Beaty TH. Inheritance of congenital esotropia. Trans Am Ophthalmol Soc (1986) 84:85–93.
48. Ferreira RDC, Oelrich F, Bateman B. Genetic aspects of strabismus. Arquivos Brasileiros Oftalmol (2002) 65:S0004-27492022005005215. doi: 10.1590/S0004-27492002000200004
49. Chaudhuri Z, John J, Aneja S, Thelma BK. Pedigree analysis of familial primary concomitant horizontal strabismus in northern india. Strabismus (2017) 25:200–13. doi: 10.1080/09273972.2017.1350865
50. Matsuo T, Hayashi M, Fujiwara H, Yamane T, Ohtsuki H. Concordance of strabismic phenotypes in monozygotic versus multizygotic twins and other multiple births. Jpn J Ophthalmol (2002) 46:59–64. doi: 10.1016/S0021-5155(01)00465-8
51. Worth C. Squint; its causes, pathology and treatment. Philadelphia, Blakiston: John Bale, Sons & Danielsson (1903). p. 1903.
52. Cohn H. Uber Vererbung und Behandlung des Einwarts-Schielen. Berl Klin Wochenschr (1904) 41:1047–51.
54. Ziakas NG, Woodruff G, Smith LK, Thompson JR. A study of heredity as a risk factor in strabismus. Eye (Lond) (2002) 16:519–21. doi: 10.1038/sj.eye.6700138
55. Rice A, Nsengimana J, Simmons IG, Toomes C, Hoole J, Willoughby CE, et al. Replication of the recessive STBMS1 locus but with dominant inheritance. Invest Ophthalmol Vis Sci (2009) 50:3210–7. doi: 10.1167/iovs.07-1631
56. Shaaban S, Matsuo T, Fujiwara H, Itoshima E, Furuse T, Hasebe S, et al. Chromosomes 4q28.3 and 7q31.2 as new susceptibility loci for comitant strabismus. Invest Ophthalmol Vis Sci (2009) 50:654–61. doi: 10.1167/iovs.08-2437
57. Khan AO, Shinwari J, Abu Dhaim N, Khalil D, Al Sharif L, Al Tassan N. Potential linkage of different phenotypic forms of childhood strabismus to a recessive susceptibility locus (16p13.12-p12.3). Mol Vis (2011) 17:971–6.
58. Khan AO, Shinwari J, Al Sharif L, Khalil D, Al-Gehedan S, Tassan NA. Infantile esotropia could be oligogenic and allelic with Duane retraction syndrome. Mol Vis (2011) 17:1997–2002.
59. Ye XC, Roslin NM, Paterson AD, Lyons CJ, Pegado V, Richmond P, et al. Linkage analysis identifies an isolated strabismus locus at 14q12 overlapping with FOXG1 syndrome region. J Med Genet (2020) 59(1):46–55. doi: 10.1101/2020.04.24.20077586
60. Wang Y, Chen X, Jiang T, Gu Y, Zhang X, Yuan W, et al. Expanding the phenotypic spectrum of mutations in LRP2: a novel candidate gene of non-syndromic familial comitant strabismus. J Transl Med (2021) 19:495. doi: 10.1186/s12967-021-03155-z
61. Zhang J, Matsuo T. MGST2 and WNT2 are candidate genes for comitant strabismus susceptibility in Japanese patients. PeerJ (2017) 5:e3935. doi: 10.7717/peerj.3935
62. Li J, Ma Y, Zhou W, Lyu W, Wang L, Mao S, et al. Novel variants identified in a three−generation family with concomitant exotropia. Exp Ther Med (2022) 24:688. doi: 10.3892/etm.2022.11624
63. An JY, Jung JH, Choi L, Wieben ED, Mohney BG. Identification of possible risk variants of familial strabismus using exome sequencing analysis. Genes (Basel) (2021) 12:75. doi: 10.3390/genes12010075
64. Shaaban S, Mackinnon S, Andrews C, Staffieri SE, Maconachie GDE, Chan WM, et al. Genome-wide association study identifies a susceptibility locus for comitant esotropia and suggests a parent-of-origin effect. Invest Ophthalmol Vis Sci (2018) 59:4054–64. doi: 10.1167/iovs.18-24082
65. Plotnikov D, Shah RL, Rodrigues JN, Cumberland PM, Rahi JS, Hysi PG, et al. A commonly occurring genetic variant within the NPLOC4-TSPAN10-PDE6G gene cluster is associated with the risk of strabismus. Hum Genet (2019) 138:723–37. doi: 10.1007/s00439-019-02022-8
66. Gao XR, Huang H, Kim H. Genome-wide association analyses identify 139 loci associated with macular thickness in the UK Biobank cohort. Hum Mol Genet (2019) 28:1162–72. doi: 10.1093/hmg/ddy422
67. Shah RL, Guggenheim JA, Eye UKB, Vision C. Genome-wide association studies for corneal and refractive astigmatism in UK Biobank demonstrate a shared role for myopia susceptibility loci. Hum Genet (2018) 137:881–96. doi: 10.1007/s00439-018-1942-8
68. Jensen RA, Sim X, Smith AV, Li X, Jakobsdottir J, Cheng CY, et al. Novel genetic loci associated with retinal microvascular diameter. Circ Cardiovasc Genet (2016) 9:45–54. doi: 10.1161/CIRCGENETICS.115.001142
69. Kiefer AK, Tung JY, Do CB, Hinds DA, Mountain JL, Francke U, et al. Genome-wide analysis points to roles for extracellular matrix remodeling, the visual cycle, and neuronal development in myopia. PloS Genet (2013) 9:e1003299. doi: 10.1371/journal.pgen.1003299
70. Plotnikov D, Pärssinen O, Williams C, Atan D, Guggenheim JA. Commonly occurring genetic polymorphisms with a major impact on the risk of nonsyndromic strabismus: replication in a sample from Finland. J aapos (2022) 26:12.e11–6. doi: 10.1016/j.jaapos.2021.07.015
71. Tam V, Patel N, Turcotte M, Bossé Y, Paré G, Meyre D. Benefits and limitations of genome-wide association studies. Nat Rev Genet (2019) 20:467–84. doi: 10.1038/s41576-019-0127-1
72. Perry GH, Yang F, Marques-Bonet T, Murphy C, Fitzgerald T, Lee AS, et al. Copy number variation and evolution in humans and chimpanzees. Genome Res (2008) 18:1698–710. doi: 10.1101/gr.082016.108
73. Collins RL, Brand H, Karczewski KJ, Zhao X, Alföldi J, Francioli LC, et al. A structural variation reference for medical and population genetics. Nature (2020) 581:444–51. doi: 10.1038/s41586-020-2287-8
74. Rees E, Kendall K, Pardinas AF, Legge SE, Pocklington A, Escott-Price V, et al. Analysis of intellectual disability copy number variants for association with schizophrenia. JAMA Psychiatry (2016) 73:963–9. doi: 10.1001/jamapsychiatry.2016.1831
75. Qiao Y, Mercier E, Dastan J, Hurlburt J, Mcgillivray B, Chudley AE, et al. Copy number variants (CNVs) analysis in a deeply phenotyped cohort of individuals with intellectual disability (ID). BMC Med Genet (2014) 15:82. doi: 10.1186/1471-2350-15-82
76. Bucan M, Abrahams BS, Wang K, Glessner JT, Herman EI, Sonnenblick LI, et al. Genome-wide analyses of exonic copy number variants in a family-based study point to novel autism susceptibility genes. PloS Genet (2009) 5:e1000536. doi: 10.1371/journal.pgen.1000536
77. Iakoucheva LM, Muotri AR, Sebat J. Getting to the cores of autism. Cell (2019) 178:1287–98. doi: 10.1016/j.cell.2019.07.037
78. Kendall KM, Rees E, Bracher-Smith M, Legge S, Riglin L, Zammit S, et al. Association of rare copy number variants with risk of depression. JAMA Psychiatry (2019) 76:818–25. doi: 10.1001/jamapsychiatry.2019.0566
79. Gudmundsson OO, Walters GB, Ingason A, Johansson S, Zayats T, Athanasiu L, et al. Attention-deficit hyperactivity disorder shares copy number variant risk with schizophrenia and autism spectrum disorder. Transl Psychiatry (2019) 9:258. doi: 10.1038/s41398-019-0599-y
80. Huang AY, Yu D, Davis LK, Sul JH, Tsetsos F, Ramensky V, et al. Rare copy number variants in NRXN1 and CNTN6 increase risk for tourette syndrome. Neuron (2017) 94:1101–1111 e1107. doi: 10.1016/j.neuron.2017.06.010
81. Whitman MC, Di Gioia SA, Chan WM, Gelber A, Pratt BM, Bell JL, et al. Recurrent rare copy number variants increase risk for esotropia. Invest Ophthalmol Vis Sci (2020) 61:22. doi: 10.1167/iovs.61.10.22
82. Wang K, Li M, Hadley D, Liu R, Glessner J, Grant SF, et al. PennCNV: an integrated hidden Markov model designed for high-resolution copy number variation detection in whole-genome SNP genotyping data. Genome Res (2007) 17:1665–74. doi: 10.1101/gr.6861907
83. Colella S, Yau C, Taylor JM, Mirza G, Butler H, Clouston P, et al. QuantiSNP: an Objective Bayes Hidden-Markov Model to detect and accurately map copy number variation using SNP genotyping data. Nucleic Acids Res (2007) 35:2013–25. doi: 10.1093/nar/gkm076
84. Ma W, Mao J, Wang X, Duan L, Song Y, Lian X, et al. Novel microdeletion in the X chromosome leads to kallmann syndrome, ichthyosis, obesity, and strabismus. Front Genet (2020) 11:596. doi: 10.3389/fgene.2020.00596
85. Whitman MC. Axonal growth abnormalities underlying ocular cranial nerve disorders. Annu Rev Vis Sci (2021) 7:827–50. doi: 10.1146/annurev-vision-093019-114307
86. Cederquist GY, Luchniak A, Tischfield MA, Peeva M, Song Y, Menezes MP, et al. An inherited TUBB2B mutation alters a kinesin-binding site and causes polymicrogyria, CFEOM and axon dysinnervation. Hum Mol Genet (2012) 21:5484–99. doi: 10.1093/hmg/dds393
87. Jurgens JA, Barry BJ, Lemire G, Chan WM, Whitman MC, Shaaban S, et al. Novel variants in TUBA1A cause congenital fibrosis of the extraocular muscles with or without malformations of cortical brain development. Eur J Hum Genet (2021) 29:816–26. doi: 10.1038/s41431-020-00804-7
88. Park JG, Tischfield MA, Nugent AA, Cheng L, Di Gioia SA, Chan WM, et al. Loss of MAFB function in humans and mice causes duane syndrome, aberrant extraocular muscle innervation, and inner-ear defects. Am J Hum Genet (2016) 98:1220–7. doi: 10.1016/j.ajhg.2016.03.023
89. Al-Baradie R, Yamada K, St Hilaire C, Chan WM, Andrews C, Mcintosh N, et al. Duane radial ray syndrome (Okihiro syndrome) maps to 20q13 and results from mutations in SALL4, a new member of the SAL family. Am J Hum Genet (2002) 71:1195–9. doi: 10.1086/343821
90. Tischfield MA, Bosley TM, Salih MA, Alorainy IA, Sener EC, Nester MJ, et al. Homozygous HOXA1 mutations disrupt human brainstem, inner ear, cardiovascular and cognitive development. Nat Genet (2005) 37:1035–7. doi: 10.1038/ng1636
91. Bosley TM, Alorainy IA, Salih MA, Aldhalaan HM, Abu-Amero KK, Oystreck DT, et al. The clinical spectrum of homozygous HOXA1 mutations. Am J Med Genet A (2008) 146A:1235–40. doi: 10.1002/ajmg.a.32262
92. Jen JC, Chan WM, Bosley TM, Wan J, Carr JR, Rüb U, et al. Mutations in a human ROBO gene disrupt hindbrain axon pathway crossing and morphogenesis. Science (2004) 304:1509–13. doi: 10.1126/science.1096437
93. Webb BD, Shaaban S, Gaspar H, Cunha LF, Schubert CR, Hao K, et al. HOXB1 founder mutation in humans recapitulates the phenotype of Hoxb1-/- mice. Am J Hum Genet (2012) 91:171–9. doi: 10.1016/j.ajhg.2012.05.018
94. Whitman MC, Engle EC. Genetics of Strabismus. In: Albert D, Miller J, Azar D, Young LH, editors. Albert and Jakobiec’s Principles and Practice of Ophthalmology. Springer (2020).
95. Muñoz-Ortiz J, Charry-Sánchez JD, Bechara-Arango I, Blanco-Becerra M, Talero-Gutiérrez C, Gomez-Suarez M, et al. Prevalence of ophthalmological manifestations in pediatric and adolescent populations with Down syndrome: a systematic review of the literature. Syst Rev (2022) 11:75. doi: 10.1186/s13643-022-01940-5
96. Romain DR, Cairney H, Stewart D, Columbano-Green LM, Garry M, Parslow MI, et al. Three cases of partial trisomy 7q owing to rare structural rearrangements of chromosome 7. J Med Genet (1990) 27:109–13. doi: 10.1136/jmg.27.2.109
97. Chen CP, Lin SP, Chern SR, Tsai FJ, Lee MS, Chen YJ, et al. Pure interstitial duplication of chromosome 7q (7q31.2–>q33) in a 4-year-old girl with growth restriction, short stature, speech delay and intellectual disability. Genet Couns (2011) 22:425–30.
98. Megarbane A, Gosset P, Souraty N, Lapierre JM, Korban R, Zahed L, et al. Chromosome 10p11.2-p12.2 duplication: report of a patient and review of the literature. Am J Med Genet (2001) 104:204–8. doi: 10.1002/ajmg.10021
99. Mathijssen IB, Hoovers JM, Mul AN, Man HY, Ket JL, Hennekam RC. Array comparative genomic hybridization analysis of a familial duplication of chromosome 13q: a recognizable syndrome. Am J Med Genet A (2005) 136:76–80. doi: 10.1002/ajmg.a.30758
100. Ye XC, van der Lee R, Wasserman WW, Causes S, Friedman JM, Lehman A. Strabismus in children with intellectual disability: part of a broader motor control phenotype? Pediatr Neurol (2019) 100:87–91. doi: 10.1016/j.pediatrneurol.2019.04.002
101. Ikeda J, Davitt BV, Ultmann M, Maxim R, Cruz OA. Brief report: incidence of ophthalmologic disorders in children with autism. J Autism Dev Disord (2013) 43:1447–51. doi: 10.1007/s10803-012-1475-2
102. Kabatas EU, Ozer PA, Ertugrul GT, Kurtul BE, Bodur S, Alan BE. Initial ophthalmic findings in turkish children with autism spectrum disorder. J Autism Dev Disord (2015) 45:2578–81. doi: 10.1007/s10803-015-2428-3
103. Chang MY, Doppee D, Yu F, Perez C, Coleman AL, Pineles SL. Prevalence of ophthalmologic diagnoses in children with autism spectrum disorder using the optum dataset: APopulation-based study. Am J Ophthalmol (2021) 221:147–53. doi: 10.1016/j.ajo.2020.08.048
104. Gutiérrez C, Santoni JLM, Merino P, De Liaño PG. Ophthalmologic manifestations in autism spectrum disorder. Turk J Ophthalmol (2022) 52:246–51. doi: 10.4274/tjo.galenos.2021.46588
105. Ye XC, van der Lee R, Wasserman WW. Curation and bioinformatic analysis of strabismus genes supports functional heterogeneity and proposes candidate genes with connections to RASopathies. Gene (2019) 697:213–26. doi: 10.1016/j.gene.2019.02.020
106. Kumar A, Gottlob I, Mclean RJ, Thomas S, Thomas MG, Proudlock FA. Clinical and oculomotor characteristics of albinism compared to FRMD7 associated infantile nystagmus. Invest Ophthalmol Vis Sci (2011) 52:2306–13. doi: 10.1167/iovs.10-5685
107. Hertle RW. Albinism: particular attention to the ocular motor system. Middle East Afr J Ophthalmol (2013) 20:248–55. doi: 10.4103/0974-9233.114804
108. Demirkilinc Biler E, Bozbiyik DI, Uretmen O, Kose S. Strabismus in infants following congenital cataract surgery. Graefes Arch Clin Exp Ophthalmol (2015) 253:1801–7. doi: 10.1007/s00417-015-2983-5
Keywords: strabismus, genetics, esotropia, exotropia, copy number variant, linkage, GWAS, gene
Citation: Martinez Sanchez M and Whitman MC (2023) Genetics of strabismus. Front. Ophthalmol. 3:1233866. doi: 10.3389/fopht.2023.1233866
Received: 02 June 2023; Accepted: 05 July 2023;
Published: 20 July 2023.
Edited by:
Rohit Saxena, All India Institute of Medical Sciences, IndiaReviewed by:
Gail Maconachie, The University of Sheffield, United KingdomCopyright © 2023 Martinez Sanchez and Whitman. This is an open-access article distributed under the terms of the Creative Commons Attribution License (CC BY). The use, distribution or reproduction in other forums is permitted, provided the original author(s) and the copyright owner(s) are credited and that the original publication in this journal is cited, in accordance with accepted academic practice. No use, distribution or reproduction is permitted which does not comply with these terms.
*Correspondence: Mary C. Whitman, bWFyeS53aGl0bWFuQGNoaWxkcmVucy5oYXJ2YXJkLmVkdQ==
Disclaimer: All claims expressed in this article are solely those of the authors and do not necessarily represent those of their affiliated organizations, or those of the publisher, the editors and the reviewers. Any product that may be evaluated in this article or claim that may be made by its manufacturer is not guaranteed or endorsed by the publisher.
Research integrity at Frontiers
Learn more about the work of our research integrity team to safeguard the quality of each article we publish.