- 1Department of Neurobiology and Anatomy, McGovern Medical School at The University of Texas Health Science Center at Houston (UTHealth Houston), Houston, TX, United States
- 2The University of Texas MD Anderson Cancer Center, UT Health Houston Graduate School of Biomedical Sciences, Houston, TX, United States
Biallelic loss-of-function mutations in the syntaxin 3 gene have been linked to a severe retinal dystrophy in humans that presents in early childhood. In mouse models, biallelic inactivation of the syntaxin 3 gene in photoreceptors rapidly leads to their death. What is not known is whether a monoallelic syntaxin 3 loss-of-function mutation might cause photoreceptor loss with advancing age. To address this question, we compared the outer nuclear layer of older adult mice (≈ 20 months of age) that were heterozygous for syntaxin 3 with those of similarly-aged control mice. We found that the photoreceptor layer maintains its thickness in mice that are heterozygous for syntaxin 3 relative to controls and that photoreceptor somatic counts are comparable. In addition, dendritic sprouting of the rod bipolar cell dendrites into the outer nuclear layer, which occurs following the loss of functional rod targets, was similar between genotypes. Thus, syntaxin 3 appears to be haplosufficient for photoreceptor survival, even with advancing age.
Introduction
Syntaxin 3 is a N-ethylmaleimide-sensitive factor attachment protein receptor (SNARE) protein that catalyzes fusion between vesicles and their target membranes (1). In the retina, syntaxin 3 is expressed exclusively by photoreceptors and bipolar cells (2–5), where it is required for neurotransmitter release (4, 6, 7). Recently, biallelic loss-of-function mutations in the human retinal-specific syntaxin 3 spliceform, syntaxin 3B (STX3B), have been linked to an early-onset severe retinal dystrophy in young children (8). Furthermore, biallelic postnatal inactivation of the syntaxin 3 gene (Stx3) in mouse photoreceptors has shown to result in the rapid degeneration of photoreceptors and a dramatic thinning of the outer nuclear layer (ONL) of the retina (8, 9). Thus, in addition to catalyzing the exocytic release of neurotransmitter release that underlies chemical synaptic transmission at photoreceptor and bipolar cell synaptic terminals (4, 6, 7), Stx3 also has an essential role that is necessary for photoreceptor survival.
A cellular hallmark of many inherited disorders of vision is the progressive loss of photoreceptors with age. Given the rapidly devastating consequences of biallelic STX3/stx3 loss-of-function in both humans and mice, we wondered whether monoallelic Stx3 loss-of-function might lead to retinal degeneration later in life. As a first step towards addressing this possibility, we examined the outer nuclear layer of older adult mice that were heterozygous for Stx3 with those of age-matched controls. Analysis of outer nuclear layer thickness, number of photoreceptor somata and sprouting of bipolar cell dendrites into the outer nuclear layer (ONL) indicated that inactivation of a single allele of Stx3 does not drive age-related photoreceptor loss in the mouse retina.
Materials and methods
Animals
Animal procedures conformed to National Institutes of Health guidelines and were approved by the Animal Welfare Committee of the University of Texas Health Science Center at Houston. Male and female mice globally heterozygous for Stx3 (e.g. Stx3+/- and Stx3f/-) and control mice (e.g. Stx3wt, Stx3f/f, Stx3f/+) with a C57Bl6/J background were obtained by the breeding of mouse lines that we developed and characterized previously (8, 10). In contrast to the embryonic lethality of global Stx3 inactivation in mice, mice that are heterozygous for Stx3 are viable and fertile (10). Genotyping was performed by PCR using DNA isolated from tail snips (10, 11) and independently confirmed at least once. Founder lines were negative for the retinal degeneration mutations Rd1 and Rd8 (8). Mice were kept under standard housing conditions with unlimited access to food and water and with a 12 h light/dark cycle and euthanized by cervical dislocation followed by decapitation at 14-26 months of age. The mean age and age range was comparable between groups (control: 22 ± 1 months (range 14-26 months), n=15; Stx3 het: 20 ± 2 months (range 17-26 months), n=7; p = 0.6922).
Tissue preparation and immunolabeling
Following euthanasia, eyes were enucleated and lenses removed. The eyes were fixed in 4% para-formaldehyde in 0.1M sodium phosphate buffer (RT, 1h). After fixation, eyes were rinsed and cryoprotected in 30% sucrose (PBS, overnight, 4°C), embedded in OCT embedding medium (Tissue-Tek, Torrance, CA), fast-frozen, and sectioned into 14-16 µm cryostat sections. Sections from the central retina were collected on Superfrost Plus Gold microscope slides (Fisherbrand, Pittsburgh, PA) and stored at − 20°C until use. For immunolabeling, sections were thawed and incubated in blocking solution (5% normal donkey serum and 0.3% Triton X-100 in PBS) for 1 h, and primary antibodies were applied overnight at room temperature. After washing, secondary antibodies were applied for 2 h at room temperature. Sections were rinsed and cover-slipped in ProLong Gold antifade mounting medium with DAPI (Invitrogen, Eugene, OR). The retinal distribution of Stx3B was visualized with monoclonal antibody 12E5 raised against stx3 (MilliporeSigma, Burlington, MA, United States) (12), which we characterized further in Campbell et al., 2020, Supplementary Figure 1 (13). Rabbit monoclonal PKC alpha (ab32376, Abcam, Cambridge, UK) was used to label rod bipolar cells and their dendritic processes (14, 15). Secondary Cy3 conjugated donkey anti-mouse IgG (MilliporeSigma, Burlington, MA, United States), and Alexa Fluor 488 donkey anti-rabbit IgG (Jackson ImmunoResearch, West Grove, PA) were used for visualization. All antibodies were used at a 1:200 dilution.
Imaging and image analysis
Image acquisition and data analysis were conducted in similar manner to that described previously (13). Rod spherules and cone pedicles in retinal sections were identified by their characteristic appearance and respective locations within the outer plexiform layer (OPL) and by immunolabeling for Stx3 (2, 3, 13, 16). Images (Z-stacks) were acquired on a Zeiss 800 confocal microscope (Carl Zeiss Microscopy GmbH, Oberkochen, Germany). Analysis of images was performed blinded to genotype. Measurement of outer nuclear layer thickness and photoreceptor somata number was performed in ImageJ (17). The thickness (μm) of the outer nuclear layer (ONL) was measured in maximum intensity projections using the Image J straight tool. Photoreceptor nuclei were quantified in an 800 µm2 region of the ONL using the “grid” function of Image J and counted using the “multi-point” tool. Measurement of rod bipolar cell dendrite length was performed in Fiji/ImageJ2 (17, 18). Dendritic lengths were calculated in maximum intensity projections using the free hand line tool. Dendrites were traced from the border between the outer plexiform layer and outer nuclear layer to their terminal ends in the outer nuclear layer. For each measure, 1-4 histological sections were analyzed per mouse and results averaged together to produce a single value per animal for each measure. Data were compiled in Excel (Microsoft, Redmond, WA, United States), and statistical analyses were performed in Prism 7 (GraphPad Software, Inc., San Diego, CA, United States) using the Mann Whitney Test. Figure images are displayed as maximum intensity projections. Results are represented as mean ± SEM, where “n” represents the number of mice.
Results
In this study, we asked whether having only a single functional Stx3 allele might be a risk factor for age-related photoreceptor death. To address this question, we examined and compared the retinae of older adult mice that were heterozygous for Stx3 with those of similarly-aged controls (control: 22 ± 1 months, n=15; Stx3 het: 20 ± 2 months, n=7; p = 0.6922). In the representative confocal images shown in Figure 1A and in the compiled data from multiple animals (Figure 1B), ONL thickness was not diminished in mice that were heterozygous for Stx3 when compared to controls (control: 63 ± 2 µm, n=15; Stx3 het: 73 ± 3 µm, n=7; p = 0.0164). Furthermore, there was no difference in the number of photoreceptor somata per unit area between groups (Figures 1A, C; control: 70.5 ± 2.9, n=15; Stx3 het: 73.2 ± 2.8, n=7; p = 0.6173). Together, these results indicate that the outer nuclear layer (ONL) in mice heterozygous for Stx3 is comparable to that of control mice (Figure 1).
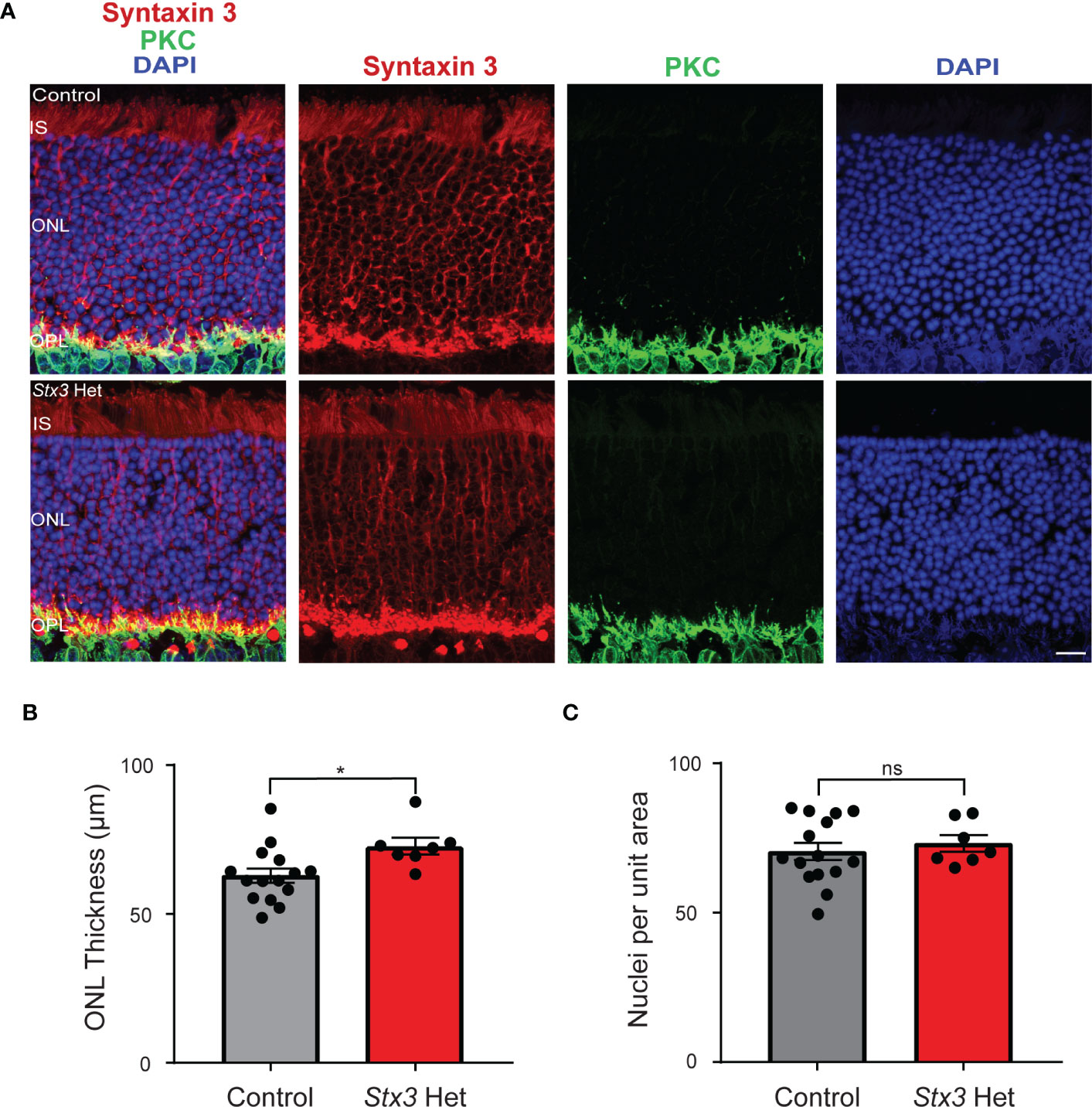
Figure 1 Outer nuclear layer (ONL) thickness and photoreceptor number are not reduced by Stx3 heterozygosity in the aged mouse retina. (A) A representative pair of confocal images from an older adult mouse heterozygous for Stx3 and an older adult control mouse show that the ONL was preserved in the Stx3 het mouse and comparable to that of the control. Stx3 (red), PKC (green), and DAPI (nuclear marker, blue). Scale bar 10µm. IS Inner segments, ONL outer nuclear layer, OPL outer plexiform layer. (B) The average thickness of the ONL was similar between groups, although it was slightly larger in the Stx3 het mice (p: 0.0164). (C) The number of nuclei in an 800 µm2 area of the ONL was not different between groups (p: 0.6173). For (B, C), Stx3 hets, n= 7 mice and for controls, n=15 mice. * denotes p value <0.05 and ns denotes p value is not significant.
When rod photoreceptors die and/or their ribbon-style synapses become non-functional, rod bipolar cells extend their dendrites beyond the outer plexiform layer (OPL) and into the ONL (19–23). We therefore measured and compared the length of PKC-labeled rod bipolar cell dendrites as a proxy of rod photoreceptor loss in older adult mice heterozygous for Stx3 and in similarly-aged control mice. Results show that dendritic lengths were virtually identical amongst the two groups, with each group having a similar percentage of dendritic length distributions that included the occasional longer ONL sprout (Figure 2). Taken together, these results demonstrate that one functional Stx3 allele is sufficient to maintain long-term photoreceptor viability.
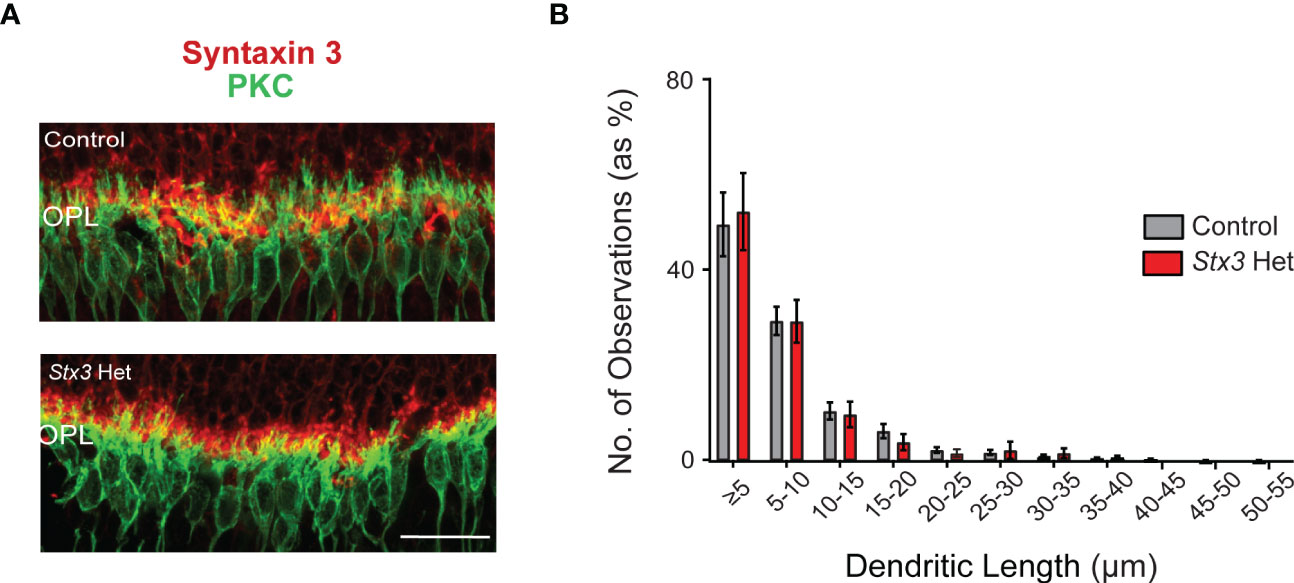
Figure 2 Rod bipolar cell dendritic length is not altered by Stx3 heterozygosity in the older adult mouse retina. (A) A representative pair of confocal images through the outer plexiform layer (OPL) of an older adult heterozygous Stx3 mouse and an older adult control mouse were labeled with antibodies against Stx3 (red) and PKC (green), show similar dendritic lengths. Scale bar 20 µm. OPL outer plexiform layer. (B) The amplitude distributions (by percentage) of dendritic lengths of rod bipolar cells in older adult mice heterozygous for Stx3 and aged control mice were virtually identical and not statistically different. Stx3 hets, n= 7 mice and for controls, n=15 mice.
Discussion
Syntaxin 3 is the only plasma membrane syntaxin known to be expressed by photoreceptors (2, 3, 24). In humans, biallelic loss-of-function mutations in syntaxin 3A, a syntaxin 3 splice form expressed widely throughout the body outside of the retina, gives rise to a devastating gastrointestinal disorder that presents in infancy (8, 25, 26). If the mutations are located in exons that are conserved between syntaxin 3A and the retinal-specific syntaxin 3 spliceform, syntaxin 3B (5, 8), the children additionally exhibited an early onset severe retinal dystrophy (8). In mice, the global inactivation of Stx3 is embryonic lethal (10), while inactivation of Stx3 selectively in photoreceptors produced a rapid loss of photoreceptors and a dramatic reduction in ONL thickness (8, 9). Thus, in addition to its role in synaptic transmission at retinal ribbon-style synapses (4, 6, 7), syntaxin 3 is also required for photoreceptor survival.
In this study, we examined the effects of deletion of a single Stx3 allele on the outer retina. We found no difference in the thickness of the outer nuclear layer between older adult controls and older adult mice that were heterozygous for Stx3. In addition, we did not observe a decrease in the number of photoreceptor somata per unit measure or an increase in the sprouting of rod bipolar cell dendrites. The latter might be expected if rods had died or retracted their spherules at a higher rate in older adult Stx3 heterozygous mice relative to age-matched controls or if the Stx3 heterozygous rod to rod bipolar cell synapses were non-functional (19, 21–23). We did note that most of the rod bipolar cell dendrites in older adult Stx3 heterozygous mice appropriately contacted rod terminals, suggesting that the primary reason for a lack of exuberant dendritic sprouting is that the dendritic targets, the rod terminals, demarcated by Stx3 immunolabeling, were still present and located close to the OPL/ONL border.
One of the motivations for conducting this study was to predict whether loss-of-function mutations in one STX3 allele might increase the risk of photoreceptor loss in human subjects later in life. Our results suggest that Stx3 is haplosufficient for photoreceptor survival, even at older ages. However, the situation could be very different if, rather than a loss-of-function mutation, there were a monoallelic dominant negative mutation. Indeed, SNAREopathies have been reported in which dominant mutations in one gene negatively affect the functionality of the wild-type transcript (27). Interestingly, of the identified human STX3 mutations associated with visual impairment to-date, all have been biallelic loss-of-function mutations (8). Thus, for these patients, the introduction of a wild-type gene could be sufficient to rescue the remaining photoreceptors and prevent further photoreceptor loss.
Data availability statement
The raw data supporting the conclusions of this article will be made available by the authors, without undue reservation.
Ethics statement
The animal study was reviewed and approved by Animal Welfare Committee of the University of Texas Health Science Center at Houston.
Author contributions
RH conceived and directed the project. MP-H and AS performed the experiments. MP-H, CD, and RH analyzed data. All authors contributed to data interpretation. RH and MH-P prepared the first draft. All authors contributed to the article and approved the submitted version.
Funding
This work was supported by R01EY012128 (RH) and P30EY028102. Additional funding was provided by the Vale-Asche Foundation via the Frederic B. Asche endowment (RH).
Acknowledgments
We thank Steve Massey and Vision Core Grant P30EY028102 for providing us with access to a state-of-the-art confocal microscopy facility.
Conflict of interest
The authors declare that the research was conducted in the absence of any commercial or financial relationships that could be construed as a potential conflict of interest.
Publisher’s note
All claims expressed in this article are solely those of the authors and do not necessarily represent those of their affiliated organizations, or those of the publisher, the editors and the reviewers. Any product that may be evaluated in this article, or claim that may be made by its manufacturer, is not guaranteed or endorsed by the publisher.
References
1. Rizo J, Sudhof TC. The membrane fusion enigma: SNAREs, Sec1/Munc18 proteins, and their accomplices–guilty as charged? Annu Rev Cell Dev Biol (2012) 28:279–308. doi: 10.1146/annurev-cellbio-101011-155818
2. Morgans CW, Brandstatter JH, Kellerman J, Betz H, Wassle H. A SNARE complex containing syntaxin 3 is present in ribbon synapses of the retina. J Neurosci (1996) 16(21):6713–21. doi: 10.1523/JNEUROSCI.16-21-06713.1996
3. Sherry DM, Mitchell R, Standifer KM, du Plessis B. Distribution of plasma membrane-associated syntaxins 1 through 4 indicates distinct trafficking functions in the synaptic layers of the mouse retina. BMC Neurosci (2006) 7:54. doi: 10.1186/1471-2202-7-54
4. Curtis L, Datta P, Liu X, Bogdanova N, Heidelberger R, Janz R. Syntaxin 3B is essential for the exocytosis of synaptic vesicles in ribbon synapses of the retina. Neuroscience (2010) 166(3):832–41. doi: 10.1016/j.neuroscience.2009.12.075
5. Curtis LB, Doneske B, Liu X, Thaller C, McNew JA, Janz R. Syntaxin 3b is a t-SNARE specific for ribbon synapses of the retina. J Comp Neurol (2008) 510(5):550–9. doi: 10.1002/cne.21806
6. Datta P, Gilliam J, Thoreson WB, Janz R, Heidelberger R. Two pools of vesicles associated with synaptic ribbons are molecularly prepared for release. Biophys J (2017) 113(10):2281–98. doi: 10.1016/j.bpj.2017.08.012
7. Hays CL, Grassmeyer JJ, Wen X, Janz R, Heidelberger R, Thoreson WB. Simultaneous release of multiple vesicles from rods involves synaptic ribbons and syntaxin 3B. Biophys J (2020) 118(4):967–79. doi: 10.1016/j.bpj.2019.10.006
8. Janecke AR, Liu X, Adam R, Punuru S, Viestenz A, Strauss V, et al. Pathogenic STX3 variants affecting the retinal and intestinal transcripts cause an early-onset severe retinal dystrophy in microvillus inclusion disease subjects. Hum Genet (2021) 140(8):1143–56. doi: 10.1007/s00439-021-02284-1
9. Kakakhel M, Tebbe L, Makia MS, Conley SM, Sherry DM, Al-Ubaidi MR, et al. Syntaxin 3 is essential for photoreceptor outer segment protein trafficking and survival. Proc Natl Acad Sci U S A (2020) 117(34):20615–24. doi: 10.1073/pnas.2010751117
10. Sanchez E, Gonzalez EA, Moreno DS, Cardenas RA, Ramos MA, Davalos AJ, et al. Syntaxin 3, but not syntaxin 4, is required for mast cell-regulated exocytosis, where it plays a primary role mediating compound exocytosis. J Biol Chem (2019) 294(9):3012–23. doi: 10.1074/jbc.RA118.005532
11. Li S, Chen D, Sauve Y, McCandless J, Chen YJ, Chen CK. Rhodopsin-iCre transgenic mouse line for cre-mediated rod-specific gene targeting. Genesis (2005) 41(2):73–80. doi: 10.1002/gene.20097
12. Zulliger R, Conley SM, Mwoyosvi ML, Stuck MW, Azadi S, Naash MI. SNAREs interact with retinal degeneration slow and rod outer segment membrane protein-1 during conventional and unconventional outer segment targeting. PloS One (2015) 10(9):e0138508. doi: 10.1371/journal.pone.0138508
13. Campbell JR, Li H, Wang Y, Kozhemyakin M, Hunt AJ Jr., Liu X, et al. Phosphorylation of the retinal ribbon synapse specific t-SNARE protein Syntaxin3B is regulated by light via a Ca(2 +)-dependent pathway. Front Cell Neurosci (2020) 14:587072. doi: 10.3389/fncel.2020.587072
14. Zhou ZY, Wan QF, Thakur P, Heidelberger R. Capacitance measurements in the mouse rod bipolar cell identify a pool of releasable synaptic vesicles. J Neurophysiol (2006) 96(5):2539–48. doi: 10.1152/jn.00688.2006
15. Haverkamp S, Wassle H. Immunocytochemical analysis of the mouse retina. J Comp Neurol (2000) 424(1):1–23. doi: 10.1002/1096-9861(20000814)424:1<1::AID-CNE1>3.0.CO;2-V
16. Liu X, Heidelberger R, Janz R. Phosphorylation of syntaxin 3B by CaMKII regulates the formation of t-SNARE complexes. Mol Cell Neurosci (2014) 60:53–62. doi: 10.1016/j.mcn.2014.03.002
17. Schneider CA, Rasband WS, Eliceiri KW. NIH Image to ImageJ: 25 years of image analysis. Nat Methods (2012) 9(7):671–5. doi: 10.1038/nmeth.2089
18. Schindelin J, Arganda-Carreras I, Frise E, Kaynig V, Longair M, Pietzsch T, et al. Fiji: An open-source platform for biological-image analysis. Nat Methods (2012) 9(7):676–82. doi: 10.1038/nmeth.2019
19. Dick O, tom Dieck S, Altrock WD, Ammermuller J, Weiler R, Garner CC, et al. The presynaptic active zone protein bassoon is essential for photoreceptor ribbon synapse formation in the retina. Neuron (2003) 37(5):775–86. doi: 10.1016/S0896-6273(03)00086-2
20. Liets LC, Eliasieh K, van der List DA, Chalupa LM. Dendrites of rod bipolar cells sprout in normal aging retina. Proc Natl Acad Sci U S A (2006) 103(32):12156–60. doi: 10.1073/pnas.0605211103
21. Fernandez-Sanchez L, de Sevilla Muller LP, Brecha NC, Cuenca N. Loss of outer retinal neurons and circuitry alterations in the DBA/2J mouse. Invest Ophthalmol Vis Sci (2014) 55(9):6059–72. doi: 10.1167/iovs.14-14421
22. Terzibasi E, Calamusa M, Novelli E, Domenici L, Strettoi E, Cellerino A. Age-dependent remodelling of retinal circuitry. Neurobiol Aging (2009) 30(5):819–28. doi: 10.1016/j.neurobiolaging.2007.08.017
23. Jones BW, Kondo M, Terasaki H, Lin Y, McCall M, Marc RE. Retinal remodeling. Jpn J Ophthalmol (2012) 56(4):289–306. doi: 10.1007/s10384-012-0147-2
24. Shekhar K, Lapan SW, Whitney IE, Tran NM, Macosko EZ, Kowalczyk M, et al. Comprehensive classification of retinal bipolar neurons by single-cell transcriptomics. Cell (2016) 166(5):1308–23 e30. doi: 10.1016/j.cell.2016.07.054
25. Wiegerinck CL, Janecke AR, Schneeberger K, Vogel GF, van Haaften-Visser DY, Escher JC, et al. Loss of syntaxin 3 causes variant microvillus inclusion disease. Gastroenterology (2014) 147(1):65–8 e10. doi: 10.1053/j.gastro.2014.04.002
26. Julia J, Shui V, Mittal N, Heim-Hall J, Blanco CL. Microvillus inclusion disease, a diagnosis to consider when abnormal stools and neurological impairments run together due to a rare syntaxin 3 gene mutation. J Neonatal Perinatal Med (2019) 12(3):313–9. doi: 10.3233/NPM-1852
Keywords: STX3, syntaxin 3B, SNAREopathy, retinal degeneration, retinal dystrophy, EOSRD, age-related, ribbon synapse
Citation: Perez-Hurtado M, Dao C, Saenz AE and Heidelberger R (2023) Syntaxin 3 is haplosufficient for long-term photoreceptor survival in the mouse retina. Front. Ophthalmol. 3:1208805. doi: 10.3389/fopht.2023.1208805
Received: 19 April 2023; Accepted: 24 May 2023;
Published: 14 June 2023.
Edited by:
Judith Mosinger Ogilvie, Saint Louis University, United StatesReviewed by:
David M. Sherry, University of Oklahoma Health Sciences Center, United StatesUlrike Grünert, The University of Sydney, Australia
Copyright © 2023 Perez-Hurtado, Dao, Saenz and Heidelberger. This is an open-access article distributed under the terms of the Creative Commons Attribution License (CC BY). The use, distribution or reproduction in other forums is permitted, provided the original author(s) and the copyright owner(s) are credited and that the original publication in this journal is cited, in accordance with accepted academic practice. No use, distribution or reproduction is permitted which does not comply with these terms.
*Correspondence: Ruth Heidelberger, cnV0aC5oZWlkZWxiZXJnZXJAdXRoLnRtYy5lZHU=
†These authors have contributed equally to this work and share first authorship