- 1Department of Psychology, Santa Clara University, Santa Clara, CA, United States
- 2Computer Science Department, City College of San Francisco, San Francisco, CA, United States
Glutamate is an important amino acid, metabolite and excitatory neurotransmitter, which is found in its free form in the extracellular spaces of the central nervous system (CNS). More than half of all synapses in CNS release glutamate. It is the main neurotransmitter driving the light responses in the retina. All types of photoreceptors, bipolar, ganglion and one type of glycinergic amacrine cells express specific subtypes of vesicular glutamate transporters and are the main source of endogenous glutamate in retina, besides Müller glia that are responsible for glutamate homeostasis, release and reuptake. Reduced or excessive extracellular glutamate was detected in the synaptic clefts of several naturally occurring or transgenic eye disease models, in which network rewiring and altered functions were observed. These led to the hypothesis that glutamate is one of the extrinsic signals for visual pathway development. This minireview examines experimental evidences supporting, or refuting, the influence of glutamate on prenatal and postnatal retinal development.
Glutamate: A key metabolite and a neurotransmitter
L-glutamate is one of the 20 ubiquitous amino acids and the metabolic precursor of inhibitory neurotransmitter γ-amino butyric acid (GABA). Glutamate oxidation stimulates insulin secretion by pancreatic β-cells; it is an essential Kreb’s cycle metabolite, required to produce NADH inside mitochondria (1). Most importantly, glutamate is a neurotransmitter in the CNS, and serves as an extrinsic signal in neural development. Glutamate does not cross blood-brain barrier. Endogenous glutamate is produced from glutamine inside glutamatergic cells and released by vesicular glutamate transporters (VGLUT1-3) mediated exocytosis (2). Glial cells actively uptake extracellular glutamate using excitatory amino acid transporters (Glia specific EAAT1/GLAST, neuron specific EAAT2-5) (3). The glutamate concentration is 10,000–12,000 μM/L inside neurons, but 0.5–2 μM/L in the extracellular fluids. EAATs help to maintain this gradient. Glutamate activates its postsynaptic and extrasynaptic receptors upon release into the synaptic cleft (4). AMPA (GluA1-4), KA (GluK1-5), NMDA (GluN1, GluN2A-D) and Delta (GluD1-2) ionotropic glutamate receptors (iGluRs) vary in their sensitivity to agonists, antagonists, biophysical properties and distribution pattern. AMPA/KA iGluRs mediate fast excitatory neurotransmission, with characteristic rise time below 1 ms and deactivation kinetics varying widely depending on subunit composition. NMDA iGluRs are slower, require glycine as co-agonist and removal of Mg2+ block for activation. Metabotropic glutamate receptors (mGluR Groups I-III) modulate the excitability of neurons via excitatory Gs/Gq (group I) or inhibitory Gi/Go (Group II-III) signaling pathways.
Glutamate in retinal circuitry
Vision is culturally and socially the most used, and hence most important of all animal senses (5). Retina is the first neural tissue in the visual pathway to sequester light, translate it into a change in membrane potential and transmit it to the synapses (6). There are three parallel image processing circuits in the retina that are driven by cones, with rods playing modulatory roles. They are object contours, color vision and motion detection circuits. Rods are monochromatic, 100 times more sensitive than polychromatic cones and drive night vision (Figure 1A) (9). These photoreceptors (PRs) form the outermost neural layer (ONL). Melanopsin containing intrinsically photosensitive retinal ganglion cells (mipRGCs) drive the blinking and pupillary light reflexes, photoentrainment of the circadian clock and encode the senses of ambient lighting condition (10). Glutamate drives PRs -> bipolars (BPs) -> RGCs neurotransmission. ON BPs express group III mGluR6 and horizontal cells (HCs) express iGluRs at the PRs ribbon synapses in the outer plexiform layer (OPL). OFF BPs form conventional synapses with PRs and express iGluRs. RGCs and ACs use iGluRs to receive glutamatergic inputs at ribbon dyads formed with BPs in the inner plexiform layer (IPL, 11). Light responses are fine-tuned by pre and postsynaptic mGluRs, mipRGCs and VGLUT3/glycine positive amacrine cells (ACs, 12–14). GABAergic HCs and GABAergic/glycinergic ACs reside in the inner nuclear layer (INL) and ganglion cell layer (GCL) and shape the temporal aspects of glutamatergic excitation. Gap junctions between retinal cells help in synchronizing the signals and lowering noise (15). RGCs relay the retinal output to lateral geniculate nucleus (LGN), suprachiasmatic nucleus (SCN), olivary pretectum (OP) and superior colliculus (SC). LGN relays visual information to striate cortex, SCN drives circadian rhythm, OP controls pupillary light reflex and SC controls eye movements required for focusing. Retina expresses VGLUTs in a complementary fashion. RGCs, Müller cell endfeets and some ACs and HCs express VGLUT2. PRs and BPs exclusively use VGLUT1, with the exception of a minority of cones that express VGLUT2 alongside VGLUT1 (16). VGLUT3 is expressed by a subtype of glycinergic ACs. Glutamatergic neurons in LGN, SCN, OP and SC express VGLUT2. Visual cortical areas use predominantly VGLUT1, except for VGLUT2 expressing layer 4 neurons, VGLUT3 expressing astrocytes and a sparse population of VGLUT3 neurons.
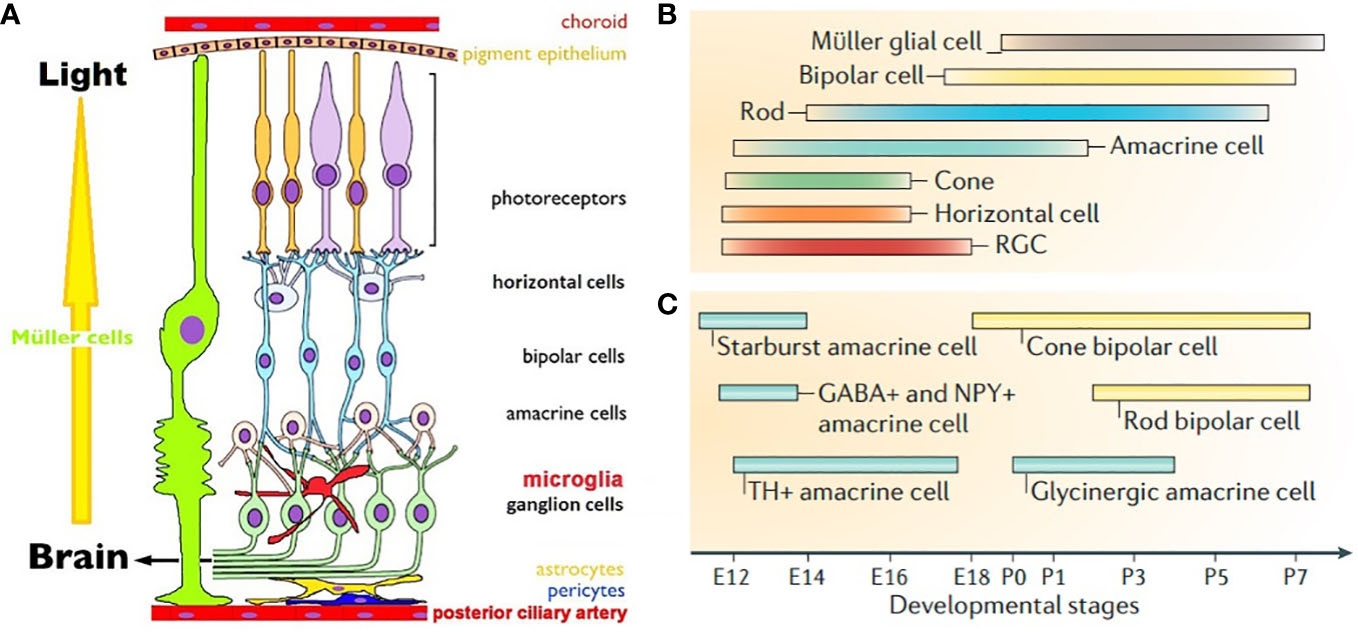
Figure 1 (A). Schematic view of mammalian retina. Displaced RGCs, ACs and intra retinal blood vessels are not shown (7). (B). Timeline of birth of retinal cells (C). GABAergic ACs are born earlier than glycinergic ACs. Cone BPs are born before rod BPs. (B, C) are from Cepko, 2014 (8).
Extracellular glutamate in eye diseases
At higher extracellular concentration glutamate is neurotoxic; excitotoxicity happens due to impairment in glutamate uptake by EAATs or blockade of metabolic pathways involving glutamate (17). Reduced synaptic glutamate, observed in retinitis pigmentosa and macular degeneration, is caused by mutations in one or multiple genes including iGluRs, mGluRs, exocytosis machinery, voltage gated and TRP channels, retinal pigment epithelium (RPE) and phototransduction related genes (18). Impaired glutamatergic neurotransmission impacts outer retinal lamination and initiates PR death. Moderate to dramatic changes are noticed in glutamate hyperexcitability conditions like ischemia, glaucoma, hyperglycemia induced diabetic retinopathy, migraine, schizophrenia, mood disorders, pathological pain, epilepsy, cerebellar ataxias, amyotrophic lateral sclerosis, Alzheimer’s and Huntington’s diseases (17, 19). In most of these diseases glutamate excitotoxicity initiates RGC death. In the retina of Alzheimer’s disease mice models, hyperexcitability was reported in early developmental stages, indicating early sign of pathogenesis.
Development of the retina
Eye development in vertebrates follows a common theme across species. Optic vesicles of future eyes evaginate out bilaterally from diencephalon to form the optic cups. RPE and neural retina (NR) are generated from the outer and inner neural layers of optic cups. Cascades of mitotic cell division form clones of retinal progenitor cells (RPCs) competent to become a specific cell type at a given time during development. Early stages of development predominantly depend on extrinsic signals and switch to intrinsic during cell cycle exit (20). Intrinsic signals are typically transcription factors, protein inhibitors and microRNAs; extrinsic signals include neurotransmitters, growth factors and diffusible molecules (8, 20, 21). Extrinsic factors signal end of proliferation by transcriptional reduction of mitogens. There seems to be a predetermined probability of a constant percentage of RPCs to timely exit cell cycle at every phase of neurogenesis. Post-mitotic cells may become insensitive to the extrinsic signals as differentiation is guided by transcriptional auto-activation of cell specific markers. Differentiating cells secrete factors that prohibit more multipotent RPCs from exiting cell cycle, thereby controlling their own density. These processes ensure sequential birth of seven retinal cell types. RGCs are the first cells to be born, followed by HCs, cones, ACs, rods, BPs and Müller glia (Figure 1B) (8). Neurogenesis is followed by migration in the apico-basal space (apical: close to RPE, basal: close to posterior ciliary artery) to achieve correct mosaic and lamination pattern. Final stage of retinal development involves synapse formation and pruning, until each cell type achieves adult like morphology, stratification pattern and functional synapses. Synaptic partners are brought together by cell adhesion molecules and extracellular matrix proteins. Neurons with no functional synapses undergo programmed cell death.
Glutamate in prenatal retinal development
Earliest works on the role of glutamate in retinal development concentrated on detecting glutamate in developing retina and evaluating effects of glutamate agonists and antagonists (22). Extracellular glutamate concentration is very high in prenatal retina, dropping abruptly at birth. Exogenous kainate acts as a trophic factor at low concentration and promotes HCs neurite sprouting into INL; higher concentration causes ablation of HCs in adult and developing rodent retina (23, 24). At P1, exogenous KCl causes nearly 2-fold increase in extracellular glutamate. This is even before glutamatergic synapses formed in OPL (P2-P5) and IPL (P6-P8) suggesting presence of extrasynaptic glutamate release mechanisms in the embryonic retina (25). VGLUT2 is detected in CNS beyond E9.5 and is critically required for prenatal development. Embryonic VGLUT1 transcripts were detected nowhere except olfactory cortex (26). The developmental role of PRs specific splice variant VGLUT1v is yet to be determined (27). Although VGLUT3 expression is limited to a subset of ACs in the retina, it is the primary VGLUT in inner ear. VGLUT3 expression is seen at E18 and beyond in CNS. Whether VGLUT2 or another VGLUT transiently expresses in embryonic RPCs and their expression switches at birth, similar to developmental switch from VGLUT2/VGLUT3 to VGLUT1 in the pyramidal cells of cerebellum, is not known.
iGluRs and mGluRs express at E14.5 and beyond; neuron-specific EAAT2 and EAAT3 express E14 onwards (28). Embryonic AMPA/KA receptors mediate exit from cell cycle by activating cyclin dependent kinase2 (cdk) inhibitor pathway; in contrast, mGluRs support proliferation. NMDA receptors mediate the neurotoxic effects of glutamate in visual pathways and cerebellum. In the developing chick retina NMDA receptors mediate choice between neuroprotection and programmed cell death by switching phosphorylation state of CREB protein (Figure 2) (29). In ischemia EAATs dependent release of glutamate into the extracellular space causes NMDA receptor mediated apoptosis. Taken together, EAAT2-3, iGluRs and mGluRs drive prenatal glutamatergic extrinsic signaling that influence choice between proliferation vs. differentiation and cell survival vs. programmed cell death.
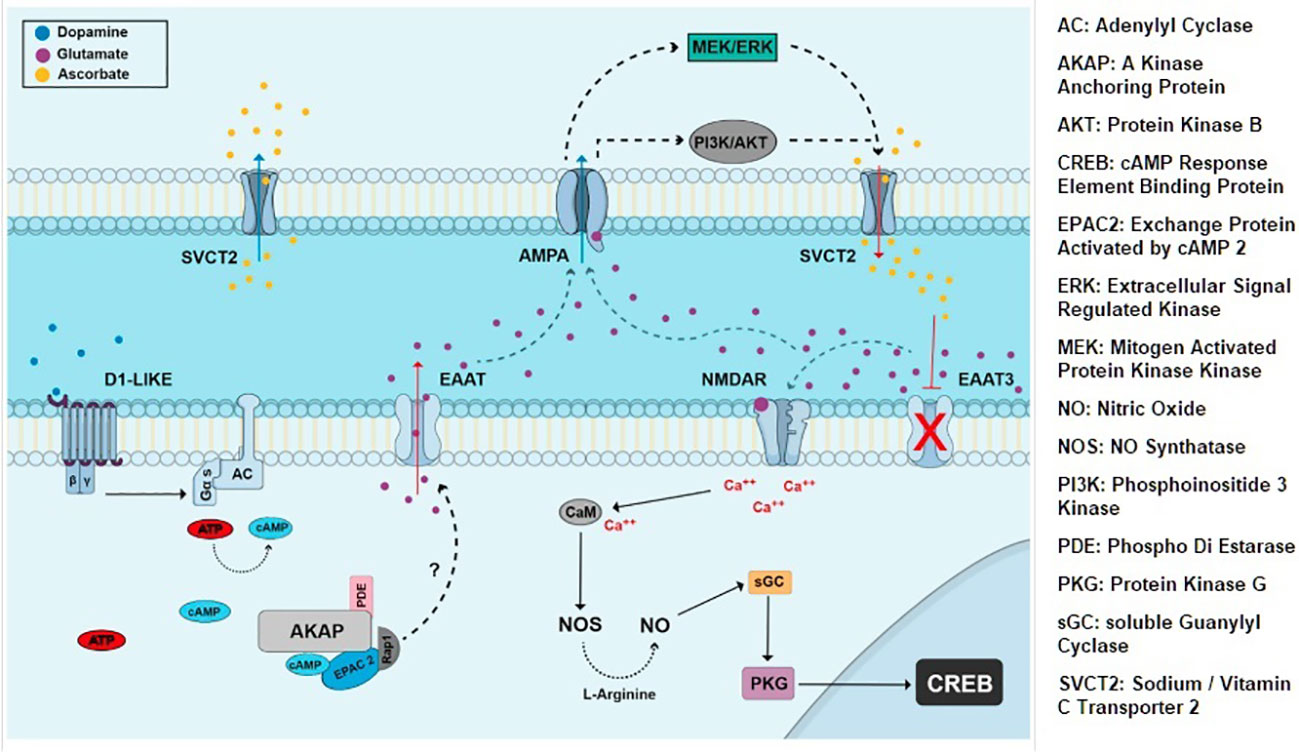
Figure 2 Extrinsic signaling involving dopamine, glutamate, EAATs and NMDA iGluRs. CREB is activated by NMDA and NO dependent cGMP/PKG pathway and affects neuronal proliferation and survival choices in chick retina (29).
RGCs axons project to LGN, SCN, OP and SC prenatally. Glutamate’s role in RGC axon guidance is not yet understood. A recent study reports that postsynaptic axon guidance effector abl2 kinase reduces iGluR currents. Abl2 knockdown causes dynamic reduction in the number of distal excitatory synapses in hippocampal cell cultures (30). Similar study has not been done in the retina. Number of axon projections to SC is altered in acetylcholine receptor beta2 knockout, presumably by tip-over glutamatergic excitation (31). This claim needs to be further validated.
Glutamate in postnatal retinal development
Retinal morphogenesis and lamination starts prenatally and continues postnatally, followed by synaptogenesis and network maturation that continues even after eye opening in rodents. At birth cones, some rods, HCs, RGCs and GABAergic ACs are already undergoing differentiation (Figures 1B, C). At P0 neuroblast layers have some rudimentary laminar structure; by P14 adult like laminar organization becomes apparent (21). Reese and colleagues showed that cones, HCs, ACs and RGCs may migrate tangentially, in addition to radially during mosaic formation (32). Rods, BPs and Müller glia are born from a single RPC that creates clonal radial columns in the retina, requiring postnatally born cells to migrate minimally. Migration effector protein reelin is expressed in RGCs, HCs, OPL and IPL and enhances Ca2+ conductance of NMDA receptors. In reeler mice rod BPs density is reduced, presumably due to altered NMDA receptor mediated choice of cell survival vs. apoptosis (33). Precise role of extracellular glutamate as an attractant or repellent in retinal migration is yet to be elucidated.
It is debated whether synapses form first and axo-dendritic arbors of pre and postsynaptic partners move together to find their desired strata. Paracrine glutamatergic signaling facilitates synapse formation by acting onto GluN2B extrasynaptic NMDA receptors in developing retina (34). Formation of ON pathway is delayed in dark reared retina, or in absence of glutamatergic input from mipRGCs (35). Synaptic targeting of ON BPs and ON RGCs dendrites does not depend on glutamate release from PRs and BPs, but fewer synapses form in reduced glutamate conditions (36). Thus, excitatory synapse formation in ON pathway requires glutamatergic signal. Role of glutamate in OFF pathway formation remains inconclusive.
Waves of transient, retina-wide correlated bursts of spiking are seen in developing GCL. Gap junctions mediate these waves in E17-P1 (stage I), acetylcholine in P2-P9 (stage II) and glutamate in P10-P14 (stage III) rodent retinas. Glutamatergic waves are not detected in VGLUT1 knockout (37). Ablating VGLUT2 expressing mipRGCs causes incomplete segregation of ipsi and contralateral RGC projections in LGN (38). Precocious glutamatergic wave causes purely ON or OFF projections in SC, whereas ON and OFF projections overtly segregate in LGN. RGCs dendrites diffusely stratify at P1. Segregation of their dendrites into ON and OFF sublamina of IPL requires cessation of group III mGluR signaling, as exogenous application of selective agonist APB severely delays this process (39). These indicate glutamate’s role in RGCs network maturation. Recent evidences suggest that mipRGCs influence VEGF and oxytocin dependent vasculature and synapse formation, implying bigger impact of light mediated glutamatergic signaling on brain and behavior (40).
Conclusion
Retinal cells are extremely diverse in their morphology and function. To this date 1 rod, 2 or more cones, 1 rod BP, 3 HCs, 1 Müller glia, 9 cone BPs, 30-60 ACs, 3-5 mipRGCs and 20-30 RGCs have been described. Establishing a multifunctional network containing millions of neurons in a 0.2 mm thick retina requires timed interplay between extrinsic, intrinsic and trophic signals. Extrinsic glutamatergic signaling is crucial for developmental choice between proliferation and cell cycle exit, as well as survival and apoptosis; in postnatal retina it affects synapse formation and network maturation. Role of glutamate in neural migration and axon guidance hasn’t been clearly identified.
Author contributions
SM reviewed the relevant articles, conceptualized the review and wrote the manuscript. The author confirms being the sole contributor of this work and has approved it for publication.
Acknowledgments
I am thankful to the editors for this opportunity to contribute in Professor Massey’s festschrift, Professors Krizaj, Cepko and Paes-de-Carvalho for permission to reproduce their graphics and Santa Clara University to cover the publication expenses.
Conflict of interest
The author declares that the research was conducted in the absence of any commercial or financial relationships that could be construed as a potential conflict of interest.
Publisher’s note
All claims expressed in this article are solely those of the authors and do not necessarily represent those of their affiliated organizations, or those of the publisher, the editors and the reviewers. Any product that may be evaluated in this article, or claim that may be made by its manufacturer, is not guaranteed or endorsed by the publisher.
References
1. Brosnan JT, Brosnan ME. Glutamate: a truly functional amino acid. Amino Acids (2013) 45(3):413–8. doi: 10.1007/s00726-012-1280-4
2. Fremeau RT Jr, Voglmaier S, Seal RP, Edwards RH. VGLUTs define subsets of excitatory neurons and suggest novel roles for glutamate. Trends Neurosci (2004) 27(2):98–103. doi: 10.1016/j.tins.2003.11.005
3. Kovermann P, Engels M, Müller F, Fahlke C. Cellular physiology and pathophysiology of EAAT anion channels. Front Cell Neurosci (2022) 15:815279. doi: 10.3389/fncel.2021.815279
4. Kew JNC, Kemp JA. Ionotropic and metabotropic glutamate receptor structure and pharmacology. Psychopharmacology (2005) 179:4–29. doi: 10.1007/s00213-005-2200-z
5. Hutmacher F. Why is there so much more research on vision than on any other sensory modality? Front Psychol (2019) 10:2246. doi: 10.3389/fpsyg.2019.02246
6. Wässle H. Parallel processing in the mammalian retina. Nat Rev Neurosci (2004) 5(10):747–57. doi: 10.1038/nrn1497
7. Ryskamp DA, Redmon S, Jo AO, Križaj D. TRPV1 and endocannabinoids: Emerging molecular signals that modulate mammalian vision. Cells (2014) 3(3):914–38. doi: 10.3390/cells3030914
8. Cepko C. Intrinsically different retinal progenitor cells produce specific types of progeny. Nat Rev Neurosci (2014) 15(9):615–27. doi: 10.1038/nrn3767
9. Ingram NT, Sampath AP, Fain GL. Why are rods more sensitive than cones? J Physiol (2016) 594(19):5415–26. doi: 10.1113/JP272556
10. McNeill DS, Sheely CJ, Ecker JL, Badea TC, Morhardt D, Guido W, et al. Development of melanopsin-based irradiance detecting circuitry. Neural Dev (2011) 6:8. doi: 10.1186/1749-8104-6-8
11. Brandstätter JH, Koulen P, Wässle H. Diversity of glutamate receptors in the mammalian retina. Vision Res (1998) 38(10):1385–97. doi: 10.1016/s0042-6989(97)00176-4
12. Dhingra A, Vardi N. “mGlu receptors in the retina” - WIREs membrane transport and signaling. Wiley Interdiscip Rev Membr Transp Signal (2012) 1(5):641–53. doi: 10.1002/wmts.43
13. Delwig A, Majumdar S, Ahern K, LaVail MM, Edwards R, Hnasko TS, et al. Glutamatergic neurotransmission from melanopsin retinal ganglion cells is required for neonatal photoaversion but not adult pupillary light reflex. PloS One (2013) 8(12):e83974. doi: 10.1371/journal.pone.0083974
14. Marshak DW. A tale of two neurotransmitters. Vis Neurosci (2016) 33:E017. doi: 10.1017/S0952523816000146
15. Massey SC. Connexins in the mammalian retina. In: Harris AL, Locke D, editors. Connexins. Humana Press (2009): 397–417. doi: 10.1007/978-1-59745-489-6_19
16. Wässle H, Regus-Leidig H, Haverkamp S. Expression of the vesicular glutamate transporter vGluT2 in a subset of cones of the mouse retina. J Comp Neurol (2006) 496(4):544–55. doi: 10.1002/cne.20942
17. Nakagawa T, Kaneko S. SLC1 glutamate transporters and diseases: psychiatric diseases and pathological pain. Curr Mol Pharmacol (2013) 6(2):66–73. doi: 10.2174/18744672113069990033
18. Marc RE, Jones BW, Anderson JR, Kinard K, Marshak DW, Wilson JH, et al. Neural reprogramming in retinal degeneration. Invest Ophthalmol Vis Sci (2007) 48(7):3364–71. doi: 10.1167/iovs.07-0032
19. Ishikawa M. Abnormalities in glutamate metabolism and excitotoxicity in the retinal diseases. Sci (Cairo) (2013) 2013:528940. doi: 10.1155/2013/528940
20. Edlund T, Jessell TM. Progression from extrinsic to intrinsic signaling in cell fate specification a view from the nervous system. Cell (1999) 96:211–24. doi: 10.1016/s0092-8674(00)80561-9
21. Burger CA, Jiang D, Mackin RD, Samuel MA. Development and maintenance of vision’s first synapse. Dev Biol (2021) 476:218–39. doi: 10.1016/j.ydbio.2021.04.001
22. Haberecht, Michael F, Dianna Ammons R. “High levels of extracellular glutamate are present in retina during neonatal development. Neurochemical Research (1996) 21:285–91. doi: 10.1007/BF02529146
23. Peichl L, Bolz J. Kainic acid induces sprouting of retinal neurons. Science (1984) 223(4635):503–4. doi: 10.1126/science.6691162
24. Redburn DA, Agarwal SH, Messersmith EK, Mitchell CK. Development of the glutamate system in rabbit retina. Neurochem Res (1992) 17:61–6. doi: 10.1007/BF00966865
25. Sherry DM, Wang MM, Bates J, Frishman LJ. Expression of vesicular glutamate transporter 1 in the mouse retina reveals temporal ordering in development of rod vs. cone and ON vs. OFF circuits. J Comp Neurol (2003) 465(4):480–98. doi: 10.1002/cne.10838
26. Boulland JL, Qureshi T, Seal RP, Rafiki A, Gundersen V, Bergersen LH, et al. Expression of the vesicular glutamate transporters during development indicates the widespread corelease of multiple neurotransmitters. J Comp Neurol (2004) 480(3):264–80. doi: 10.1002/cne.20354
27. Moriyama S, Iharada M, Omote H, Moriyama Y, Hiasa M. Function and expression of a splicing variant of vesicular glutamate transporter 1. Biochim Biophys Acta Biomembr (2017) 1859(5):931–40. doi: 10.1016/j.bbamem.2017.02.002
28. Martins RA, Linden R, Dyer MA. Glutamate regulates retinal progenitors cells proliferation during development. Eur J Neurosci (2006) 24(4):969–80. doi: 10.1111/j.1460-9568.2006.04966.x
29. Duarte-Silva AT, Ximenes LGR, Guimarães-Souza M, Domith I, Paes-de-Carvalho R. Chemical signaling in the developing avian retina: Focus on cyclic AMP and AKT-dependent pathways. Front Cell Dev Biol (2022) 10:1058925. doi: 10.3389/fcell.2022.1058925
30. Kabirova M, Reichenstein M, Borovok N, Sheinin A, Gorobets D, Michaelevski I. Abl2 kinase differentially regulates iGluRs current activity and synaptic localization. Cell Mol Neurobiol (2023). doi: 10.1007/s10571-023-01317-9
31. Chandrasekaran AR, Plas DT, Gonzalez E, Crair MC. Evidence for an instructive role of retinal activity in retinotopic map refinement in the superior colliculus of the mouse. J Neurosci (2005) 25(29):6929–38. doi: 10.1523/JNEUROSCI.1470-05.2005
32. Reese BE, Harvey AR, Tan SS. Radial and tangential dispersion patterns in the mouse retina are cell-class specific. Proc Natl Acad Sci USA (1995) 92(7):2494–8. doi: 10.1073/pnas.92.7.2494
33. Rice DS, Nusinowitz S, Azimi AM, Martínez A, Soriano E, Curran T. The reelin pathway modulates the structure and function of retinal synaptic circuitry. Neuron (2001) 31(6):929–41. doi: 10.1016/s0896-6273(01)00436-6
34. Andreae LC, Burrone J. The role of neuronal activity and transmitter release on synapse formation. Curr Opin Neurobiol (2014) 27(100):47–52. doi: 10.1016/j.conb.2014.02.008
35. Majumdar S, Bredl D, David R. Copenhagen; melanopsin controls early postnatal retinal development. Invest Ophthalmol Vis Sci (2012) 53(14):2724.
36. Kerschensteiner D. Glutamatergic retinal waves. Front Neural Circuits (2016) 10:38. doi: 10.3389/fncir.2016.00038
37. Blankenship AG, Ford KJ, Johnson J, Seal RP, Edwards RH, Copenhagen DR, et al. Synaptic and extrasynaptic factors governing glutamatergic retinal waves. Neuron (2009) 62(2):230–41. doi: 10.1016/j.neuron.2009.03.015
38. Chew KS, Renna JM, McNeill DS, Fernandez DC, Keenan WT, Thomsen MB, et al. A subset of ipRGCs regulates both maturation of the circadian clock and segregation of retinogeniculate projections in mice. Elife (2017) 6:e22861. doi: 10.7554/eLife.22861
39. D’Orazi FD, Suzuki SC, Wong RO. Neuronal remodeling in retinal circuit assembly, disassembly, and reassembly. Trends Neurosci (2014) 37(10):594–603. doi: 10.1016/j.tins.2014.07.009
Keywords: retina, glutamate, development, synapse, extrinsic, neurogenesis, synapse formation
Citation: Majumdar S (2023) Role of glutamate in the development of visual pathways. Front. Ophthalmol. 3:1147769. doi: 10.3389/fopht.2023.1147769
Received: 19 January 2023; Accepted: 20 February 2023;
Published: 03 March 2023.
Edited by:
Christophe P. Ribelayga, University of Houston, United StatesReviewed by:
Salvatore Lucia Stella, Jr., College of Medicine, The Pennsylvania State University, United StatesCopyright © 2023 Majumdar. This is an open-access article distributed under the terms of the Creative Commons Attribution License (CC BY). The use, distribution or reproduction in other forums is permitted, provided the original author(s) and the copyright owner(s) are credited and that the original publication in this journal is cited, in accordance with accepted academic practice. No use, distribution or reproduction is permitted which does not comply with these terms.
*Correspondence: Sriparna Majumdar, smajumdar2@scu.edu