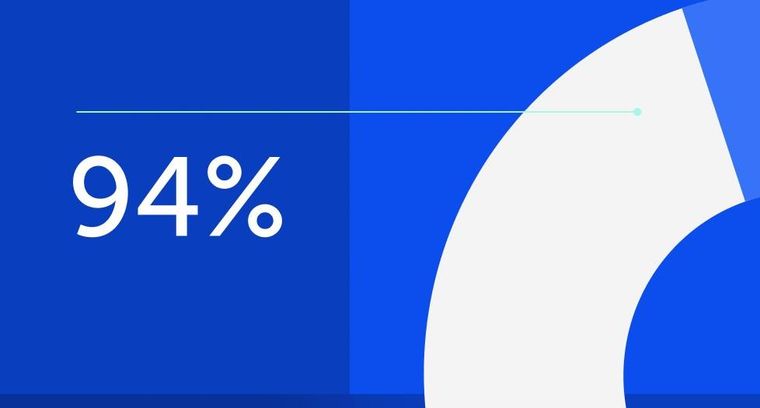
94% of researchers rate our articles as excellent or good
Learn more about the work of our research integrity team to safeguard the quality of each article we publish.
Find out more
MINI REVIEW article
Front. Ophthalmol., 01 August 2022
Sec. Inflammatory Eye Diseases
Volume 2 - 2022 | https://doi.org/10.3389/fopht.2022.948481
Dry eye syndrome, a form of ocular surface inflammation, and chronic ocular pain are common conditions impacting activities of daily living and quality of life. Oxytocin and secretin are peptide hormones that have been shown to synergistically reduce inflammation in various tissues and attenuate the pain response at both the neuron and brain level. The oxytocin receptor (OXTR) and secretin receptor (SCTR) have been found in a wide variety of tissues and organs, including the eye. We reviewed the current literature of in vitro experiments, animal models, and human studies that examine the anti-inflammatory and anti-nociceptive roles of oxytocin and secretin. This review provides an overview of the evidence supporting oxytocin and secretin as the basis for novel treatments of dry eye and ocular pain syndromes.
Dry eye syndrome (DES) affects up to 15% of the adult population, and up to 30% of patients over age 50, causing significant impairment in visual acuity, workplace functioning, and activities of daily living (1, 2). Originally thought to be a condition of reduced tear volume, subsequent research shows that the pathogenesis involves a complex interplay of multiple autoimmune, inflammatory, hormonal, and pain pathway influences (3, 4). Despite a better understanding into the mechanism of dry eye pathogenesis, currently available treatments are limited to symptomatic relief with artificial tears and non-specific immunosuppressive medications (5).
Oxytocin is a nine amino-acid neuropeptide hormone synthesized in the paraventricular and supraoptic nuclei of the hypothalamus and has well-studied roles in parturition, lactation, social bonding, and neuropsychological disorders (6). It binds to the oxytocin receptor (OXTR), which is a member of the rhodopsin-type (class I) G-protein coupled receptor family that contains seven transmembrane α-helices and is coupled to Gαq/11, Gαi/o, or Gαs heterotrimeric complexes (7–10). When coupled to Gαq/11 class GTP binding proteins, stimulation of this receptor activates phospholipase Cβ (PLCβ) catalyzing the hydrolysis of phosphatidylinositol 4,5-bisphosphate (PIP2) to generate inositol triphosphate (IP3) and 1,2-diacylglycerol (DAG), which in turn leads to intracellular calcium release and protein kinase C stimulation, respectively (11). In conjunction with calmodulin, intracellular calcium then activates protein kinases involved in myoepithelial cell contraction. While the oxytocin PLC/Ca2+ pathway is well known for its role in mammary gland and smooth muscle function, it has recently been shown to induce lacrimal gland myoepithelial cell contraction (7). OXTR can also be coupled to Gαi/o, resulting in inhibition of adenylate cyclase and a decrease of intracellular cyclic adenosine monophosphate (cAMP) (10). OXTR coupled with Gαs potentially increases cAMP; however, its physiologic existence is questionable with only low or insignificant coupling ever demonstrated (8–10). Depending on the tissue type, stimulation of OXTRs coupled with Gαq/11 or Gαi/o complexes can act synergistically, as in the case of myometrial cell contraction, or antagonistically as occurs with inward rectifier K + channel conductance in olfactory neurons (12) and cell growth in human embryonic kidney cells (13–15).
The OXTR can also signal via G protein-independent pathways. Stimulation of the OXTR and subsequent phosphorylation by G protein coupled receptor kinases induce the recruitment of β-arrestins (9, 16). While β-arrestins lead to desensitization of the OXTR, they also have been shown to simultaneously activate downstream signaling, including the mitogen-activated protein kinase (MAPK) pathway (16).
OXTR has been shown to be expressed in the tongue, nose, retina, gut, and brain (11, 17–20). Studies suggest anti-inflammatory roles for oxytocin in the heart (21), skin (22), and gastrointestinal tract (23), as well as pain mitigation in the setting of chronic low back pain (24), facial pain syndromes (25), and migraine headaches (26, 27).
The secretion of oxytocin and expression of OXTR have been shown to be influenced by expression of secretin, a peptide hormone that regulates water homeostasis and pancreatic secretions, and the secretin receptor (SCTR) in the hypothalamus (28, 29). Rodent model studies of inflammatory bowel disease demonstrate that oxytocin works synergistically with secretin to reduce inflammation in the gut (30). These results suggest that dual peptide treatment may modulate inflammation in tissues and organs that express both OXTR and SCTR and that secretin may potentiate the action of oxytocin. The presence of OXTR has been previously described in the retina, where it was proposed to have a role in paracrine signaling with the retinal pigment epithelium (19). OXTR had also been identified in human and mouse lacrimal glands with its expression decreased in the setting of dry eye mouse models (31). Additionally, OXTR and SCTR have been shown to be expressed on the corneal surface of humans and rats, although their function is unknown (32). Given their anti-inflammatory roles and presence on relevant ocular structures, these neuropeptide pathways potentially may be harnessed for novel treatments of dry eye and ocular pain syndromes.
PubMed was used to search for articles containing combinations of keywords: (oxytocin OR secretin) AND (inflammation OR anti-inflammatory OR pain OR nociception OR trigeminal neuralgia OR eye OR dry eye syndrome OR Sjögren’s syndrome). Available articles in English were reviewed and included in this review if found to be relevant to the anti-inflammatory or anti-nociceptive effects of OXTR and/or SCTR on the eye.
Chronic ocular pain is a significant component of DES that may be less responsive to conventional treatment including lubrication, topical corticosteroids, and immunomodulators (33). Multiple studies suggest that DES patients can experience symptoms consistent with neuropathic pain, including hypersensitivity to environmental triggers (wind, light, heat), spontaneous burning unresponsive to therapies, severe symptoms with minimal exam findings, co-existing psychologic distress, and increased pain sensitivity in regions other than the eye (34, 35). Patients with increased chronic pain-related syndromes exhibit more severe neuropathic-type dry eye symptoms, suggesting that a subset of dry eye patients resistant to conventional treatment may manifest an underlying central pain processing disorder (36).
There are several proposed pathways that modulate neuropathic pain. Nerve growth factor (NGF), released in chronic inflammation, increases sensitivity to painful stimuli by activating peripheral nociceptors via tyrosine kinase (trkA) receptors (37). A second proposed pathway is mediated by corneal nociceptors that transmit pain signals along the trigeminal nerve (V1) followed by the release of glutamate binding to AMPA (α-Amino-3-hydroxy-5-methyl-4-isoxazolepropionic acid) receptors for the sensation of acute pain and NMDA (N-methyl-D-aspartate) receptors for chronic pain, resulting in a continued transmission of pain signals to higher-order neurons (37). Additionally, in one genome-wide analysis of differentially expressed genes following dorsal root ganglion compression, several key genes that may play a role in neuropathic pain development were found, including syndecan 1 (Sdc1); phosphatidyloinositol-4,5-bisphosphate 3-kinase, catalytic subunit gamma (Pi3k); Janus kinase 2 (Jak2); Jun proto-oncogene, AP-1 transcription factor subunit (Jun); and interleukin 6 (IL-6) (38).
In addition to neuropathic pain, there is also a component of nociceptive pain in DES. Heightened sensitivity to pain and diminished pain tolerance are significantly associated with symptoms of dry eye disease (39). This type of pain involves several nociceptor pain fibers, such as transient receptor protein (TRP) channels, that are responsible for sensing tissue inflammation and damage (40). Environmental triggers can lead to damaged epithelial cells and corneal nerves, causing an upregulation of pro-inflammatory mediators within the cornea of dry eye patients. These inflammatory stimuli include prostaglandin 2 (PGE2), cyclooxygenase 2 (COX2), various interleukins (IL-2, IL-4, IL-5, IL-6, IL-8, IL-10, IL-17), tumor necrosis factors alpha (TNF-α), reactive oxygen species, nerve growth factor (NGF), and metalloproteinase-9 (MMP-9) (40). Pain hypersensitivity and central sensitization involving the trigeminal brainstem may result from persistent ocular surface damage, inflammation, and pain nerve stimulation, leading to chronic dry eye symptoms that are disconnected from the pathology seen in the eye (40, 41). Patients with greater dry eye and reported ocular pain symptoms also display hypersensitivity within corneal somatosensory pathways that suggest modulation of specific nerve pathways (42). Furthermore, dry eye symptoms have been correlated with measures of anxiety and pain sensation at distant locations in the forearm, suggesting diffuse somatosensory dysfunction beyond the trigeminal system (43).
It is well-documented that neuropathic pain similar to symptoms experienced by patients with DES is mediated by chronic inflammation in neurons and increased excitability in brain regions associated with somatosensory processing and stress (44). Table 1 outlines the anti-nociceptive roles of oxytocin and secretin. There is evidence from rat models that oxytocin modulates pain processing at the rostral angular insular cortex, a brain structure important in nociception (45). At the level of the spinal cord, animal models of neuropathic pain have shown that oxytocin inhibits nociceptive neurotransmission at the medullary dorsal horn by suppressing transmission from sensory Aδ and C-fibers (54) and increasing GABAergic inhibitory transmission to TRPV1 (transient receptor potential channel vanilloid 1), a non-selective cation channel that transmits painful stimuli in the nervous system (46). In addition, oxytocin suppresses the expression of inflammatory cytokines TLR-4, TNF-α, and IL-1β while down-regulating mechanical and thermal pain sensation in rat models (47). At the neuronal level, oxytocin inhibited activation of the trigeminocervical complex in animal models (48) by blocking the release of calcitonin gene-related peptide, a neuropeptide that regulates nociceptive signaling (27). The mechanism of oxytocin’s effects may be mediated by increasing intracellular chloride concentrations with the KNCC1 chloride co-transporter and depolarizing GABA on nociceptive trigeminal neurons (49) or increasing voltage-gated K+ channel currents in the trigeminal ganglia, decreasing the resting potential and activity of the neurons (25). One study showed that oxytocin was effective in alleviating vincristine-induced neurite damage in cultured primary dorsal root ganglion neurons in vitro and vincristine-induced hyperalgesia with an in vivo mouse model (50).
In several animal models, oxytocin attenuates trigeminal (26, 51) as well as visceral pain (51). At the neurophysiologic level, oxytocin receptors have been detected in the lateral capsular division of the central nucleus of the amygdala, with anxiolytic effects mediated by the depression of inwardly rectifying K+ channels (52, 55). Activation of parvocellular oxytocin neurons in the paraventricular nuclei can also inhibit spinal pain processing by repressing nociceptive transmission from Aδ and C-type afferent signaling pathways (56). In a rat model of neuropathic pain, oxytocin application to nerve-injured sites decreased the amount of TRPV1-immune reactive trigeminal neurons, key players in nociception, that innervated the whisker pad skin. This led to an attenuation of orofacial mechanical allodynia following infraorbital nerve injury (53). In another rat study of neuropathic pain involving infraorbital nerve injury, the combined effects of oxytocin application to the locally injured nerve and low-level laser therapy showed a decrease in cortical excitation in response to electrical stimulation of dental pulp, reflecting a reduction of neuropathic pain processed by the animals’ brains (57). In animal models of intestinal inflammation, the combination of secretin and oxytocin decreases the excitability of central neurons located in the hypothalamus, amygdala, and piriform cortex, areas of the brain critical for the emotional processing of pain (30), with similar findings in the frontal cortex, hippocampus, thalamus, and midbrain observed in separate models (26). In a double-blinded, placebo-controlled, cross-over design trial involving 30 healthy human subjects, a single dose of 40 IU/ml intranasal oxytocin was shown to enhance endogenous pain inhibition in the trigeminal distribution via conditioned pain modulation as well as improved mood (58). Another double-blinded, placebo-controlled trial of ten individuals with migraine showed a dose-dependent response of oxytocin in reducing headache pain with oxytocin levels positively correlated with measures of symptom severity (59).
Ocular surface inflammation is an important risk factor for and subsequent consequence of DES (3). Both the innate and adaptive immune system play key roles in the pathogenesis of DES. The innate immune response in DES has been shown to involve activation of interleukin and tumor necrosis factors through the MAPK pathway and upregulation of immune cells through Toll-like receptor signal transduction (60). The adaptive immune response in DES has been shown to involve CD4+ T cells and antigen presenting cells (APCs), which increase production of a multitude of cytokines, including IFN-gamma, IL-6, IL-17, IL-4, and IL-1, that contribute to further pro-inflammatory pathways, such as NF-κB and corneal epithelial caspase-1 pathways (60).
There is evidence supporting the anti-inflammatory roles of oxytocin and secretin in multiple organ systems, outlined in Table 2. Oxytocin has been shown in rodent models to decrease plasma concentrations of cytokines including TNF-α, IL-1, IL-4, and IL-6 in the setting of bacterial infection (61), wound healing (62), and intestinal inflammation (30, 63), where there was synergy with secretin. Rodent model studies suggest that oxytocin has widespread effects on leukocyte activation, expression, and migration, while decreasing stress-induced release of reactive oxygen species and corticosterone (64, 74–76). In an obesity mouse model, the administration of oxytocin decreased TNF-α secretion and the macrophage M1/M2 ratio, inducing an anti-inflammatory phenotype with improvements in glucose tolerance (65). In another obesity mouse model study, expression of adipose tissue OXTR was increased in obese mice compared to lean controls. Exposure to long-term oxytocin treatment led to reductions in visceral adipose tissue inflammation, with decreased adipocyte size, macrophage infiltration, IL-6 and TNF-α expression. Adiponectin, a key anti-inflammatory adipokine, was increased in both plasma and adipose tissue, while plasma levels of serum amyloid A, a marker of systemic inflammation, were decreased (66). In vivo and in vitro models of food allergy showed that oxytocin inhibits the production of multiple cytokines, including thymic stromal lymphopoietin (TSLP), IL-33, and IL-25, through suppression of NF-κB signaling and upregulation of B-arrestin2 expression, which decreased systemic anaphylactic responses and intestinal inflammation in mice (67). In a separate inflammatory bowel disease (IBD) murine model, OXTR knock-out mice displayed an increased number of pro-inflammatory cytokine transcripts encoding for TNF-α, IL-1β, and IL-6 and exhibited shorter villi and crypts in the intestinal mucosa, a sign of chronic inflammation. In addition, colonic tissue in OXTR knock-out mice was more susceptible to cholera toxin-induced inflammation compared to a blunted immune response observed in wild-type mice treated with oxytocin (68). Oxytocin-treated mice also had a reduction in neutrophils and macrophages observed in the mucosal and submucosal layers of the colon and decreased expression of TNF-α and IFN-γ, leading to clinical improvement in ulcerative disease (30). At the cellular level, in vitro experiments using gut epithelial cells have demonstrated that oxytocin modulates important molecules in the stress signaling pathway (69, 77) and plays a regulatory role in translation of proteins (69). In addition, oxytocin may protect epithelial cells from inflammation (70). Mouse models of blepharitis, Sjögren’s keratoconjunctivitis sicca, and DES have demonstrated elevated levels of inflammatory cytokines, including IL-1, IL-6, and TNF-α (78–80) although the role of oxytocin in modulating these inflammatory cytokines on the ocular surface is currently unknown.
There is evidence of synergistic activity between secretin and oxytocin, an interaction that may enhance their usefulness as anti-inflammatory agents. Indeed, the release of oxytocin is directly influenced by the levels of circulating secretin through stimulation of α1-adrenoreceptors and SCTR (81). Secretin has been shown to activate supraoptic oxytocin neurons, which express SCTR, and facilitate release of oxytocin from these nerve dendrites in mice (28). In rats, secretin can increase the firing rate of supraoptic oxytocin neurons through noradrenergic pathways, thereby increasing the plasma concentration of oxytocin (82). In a rat model of chronic colitis, combined intravenous (IV) administration of secretin and oxytocin led to decreased inflammatory infiltrates in the colon and reduced expression of TNF-α and IFN- γ in colonic tissue. Interestingly, IV administration of oxytocin or secretin alone did not produce a significant change in colonic inflammation. Combined IV administration of oxytocin and secretin also inhibited colitis-associated activation of forebrain neurons in the paraventricular nucleus of the hypothalamus, basolateral amygdala, central amygdala, and piriform cortex (30).
Oxytocin and OXTR are expressed in the myoepithelial cells of the lacrimal gland, stimulating the contraction of acinar cells to secrete tears. The number of oxytocin receptors and myoepithelial cells are significantly reduced in mouse models of Sjögren’s syndrome, suggesting oxytocin’s role in maintaining tear production. Stimulation of oxytocin receptors in the lacrimal glands of healthy, control mice resulted in contraction of acini and the production of tears that was not present when dry eye disease mice were exposed to oxytocin (31). The pro-inflammatory state of DES may also be driven by corneal epithelial dysfunction and increased sensitivity to bacterial metabolites produced by commensal organisms on the ocular surface. For example, in dry eye mouse models, bacterial lipopolysaccharides (LPS) increases the expression of inflammatory mediators including IL-1β, IL-6, CXCL10, IL-12a, and IFN-γ in the conjunctiva and IL-1β and CXCL10 in the cornea (83). In-vitro studies of anterior pituitary cell culture provided the first evidence that oxytocin can inhibit LPS and IL-1β stimulation of macrophages, T-cells, and B-cells and decrease IL-6 cytokine production (71).
Animal studies have demonstrated that secretin increases tear production but not when topical anesthesia is applied, suggesting a sensory-dependent mechanism of effect (73). Vasoactive intestinal peptide (VIP) and pituitary adenylate cyclase-activating polypeptide (PACAP), which are part of the same superfamily of structurally related peptide hormones that includes secretin, glucagon, glucagon-like peptides, gastric inhibitory peptide (GIP) and growth hormone-releasing hormone (GHRH), have been shown to increase tear production by upregulating cyclic adenosine monophosphate (cAMP) and cyclic guanosine monophosphate (cGMP) production and protein kinase A phosphorylation, resulting in downstream aquaporin protein expression stimulating fluid and protein flow (73, 84, 85).
Animal and human studies suggest that oxytocin can be administered safely for local treatment with little to no systemic side effects. In mouse, rabbit, and primate models, there was minimal systemic absorption of oxytocin through the nasal mucosa (86, 87) and ocular surface (88). In a mouse model of mild traumatic brain injury (TBI), intranasal oxytocin application led to an attenuation of reactive and ongoing non-reactive pain following mild TBI for at least 3-4 hours. Interestingly, IV administration of oxytocin did not have the same pain attenuating effects, and there were higher concentrations of oxytocin found in the trigeminal ganglion following intranasal application compared to IV administration of oxytocin (51). A 20-year review of intranasal oxytocin use in human research studies found that dosages of 18-40 IU oxytocin had no reliable side effect profile compared to placebo and no reported adverse outcomes (89).
Several analogs of oxytocin, including lipo-oxytocins and TGOT, have been studied in the basic science and clinical trial contexts for social anxiety, autism spectrum, and other neuropsychiatric disorders, and shown to be powerful agonists on OXTR (90–92). Carbetocin is another oxytocin analog that has been extensively studied as treatment for postpartum hemorrhage when peripherally administered (92, 93). Unlike oxytocin itself, recent evidence points to oxytocin analogs having differential abilities to activate the OXTR, and subsequent downstream effects, depending on which G protein with the OXTR is coupled (15), raising the potential of selective OXTR-based therapies. Recently, a non-peptide agonist of oxytocin receptor (LIT-001) was able to induce a durable reduction in inflammatory pain-induced hyperalgesia in a rat model (94). Several small molecules have shown evidence as allosteric modulators of the SCTR, expanding potential drug options beyond secretin and its peptide analogs (95, 96).
There has been evidence for oxytocin and secretin’s role in reducing inflammation within human corneal cells. In a cell culture model of ocular surface inflammation created through the exposure of TNF-α to human corneal cells, levels of ICAM-1 expression were measured following administration of oxytocin and/or secretin. Three hours after addition of oxytocin or secretin, ICAM-1 levels were reduced about 40% compared to TNF-α treatment alone. A combination treatment of both oxytocin and secretin did not result in further reduction of ICAM-1 levels. The effect of the hormonal treatment was transient, with a maximal change at 3-4 hours (32).
A recent prospective cohort study investigated the use of nebulized oxytocin compared to vitamin B12 for the treatment of dry eye disease. Thirty-eight patients with DES were enrolled, with half receiving oxytocin (OXT) nebulization and the other half receiving vitamin B12 (VB12) nebulization treatment twice weekly for 3 months. Several clinical measurements were taken at baseline, 1 month, and 3 months after starting treatment, including Ocular Surface Disease Index (OSDI) questionnaire, self-reported light sensitivity and dryness, tear meniscus height (TMH), tear break-up time (BUT), and corneal staining. In vivo confocal microscopy (IVCM) data of basal epithelial cell density, sub-basal dendritic cell density, nerve density, and nerve tortuosity were also measured (72). IVCM is used to identify structural features that are pathognomonic for DES, including decreased number of corneal epithelial cells, increased number of dendritic cells, reduced sub-basal nerve density, and increased nerve tortuosity (97).
There were no adverse events in either treatment group over the 3 months. The VB12 group showed statistically significant improvements in all clinical measurements and signs of DES, apart from nerve tortuosity, over the 3-month treatment. For the OXT group, all clinical and IVCM data showed significant improvement at 1 month, except for nerve tortuosity. Between the 1-month and 3-month timepoints, there were significant improvements in OSDI, TMH, BUT, and sub-basal nerve density; however, nerve tortuosity was increased. Like the VB12 group, the oxytocin group showed significant improvements at 3 months compared to baseline, except for nerve tortuosity and BUT. The OXT group IVCM data showed an increased basal epithelial cell density, decreased dendritic cell density, and increased sub-basal nerve density and tortuosity at 1 and 3 months, reflecting an overall improvement in DES conditions, apart from the increased nerve tortuosity (72). This study demonstrates a promising potential use of nebulized oxytocin as an effective treatment for DES.
There is a growing body of evidence supporting the role of oxytocin and secretin in ocular surface homeostasis, outlined in Figure 1. Oxytocin promotes tear production by the lacrimal gland and appears to reduce pain via both neuronal and central mechanisms; its intranasal use has shown promise in the treatment of trigeminal pain. There is compelling evidence showing the anti-inflammatory and anti-nociceptive potential of oxytocin, especially in synergism with secretin, in intestinal mucosa and other tissue types. There may be a similar role for these peptide hormones in reducing ocular surface inflammatory and pain syndromes. Further studies are necessary to determine the physiologic functions of ocular surface oxytocin and secretin receptors and how stimulation of these receptors modulate inflammatory and pain pathways.
Figure 1 Potential mechanistic relationships and biomarkers relating stimulation of the oxytocin and secretin receptors at the ocular surface with modulation of pain and inflammation.
All authors made substantial contributions to the conception of this mini-review and drafted or revised it critically for important intellectual content. They provide approval for publication of the content and agree to be accountable for all aspects of the work in ensuring that questions related to the accuracy or integrity of any part of the work are appropriately investigated and resolved.
This review was supported by funds from the National Institutes of Health (NIH-NEI P30 EY002162 – Core Grant for Vision Research; and R01EY032161 awarded to M.F.C.), Research to Prevent Blindness (an unrestricted grant to the UCSF Department of Ophthalmology), and All May See Foundation.
Thank you to Suling Wang and the UCSF Vision Core for assistance with creating the figure.
BW along with Columbia University filed a patent application for the use of oxytocin and secretin for the treatment of ocular surface disease.
All claims expressed in this article are solely those of the authors and do not necessarily represent those of their affiliated organizations, or those of the publisher, the editors and the reviewers. Any product that may be evaluated in this article, or claim that may be made by its manufacturer, is not guaranteed or endorsed by the publisher.
1. Moss SE. Prevalence of and risk factors for dry eye syndrome. Arch Ophthalmol (2000) 118(9):1264. doi: 10.1001/archopht.118.9.1264
2. Moss SE, Klein R, Klein BEK. Incidence of dry eye in an older population. Arch Ophthalmol (2004) 122(3):369–73. doi: 10.1001/archopht.122.3.369
3. Baudouin C. The pathology of dry eye. Survey Ophthalmol (2001) 45:S211–20. doi: 10.1016/S0039-6257(00)00200-9
4. Craig JP, Nichols KK, Akpek EK, Caffery B, Dua HS, Joo CK, et al. TFOS DEWS II definition and classification report. Ocul Surf (2017) 15(3):276–83. doi: 10.1016/j.jtos.2017.05.008
5. Valim V, Trevisani VFM, de Sousa JM, Vilela VS, Belfort R. Current approach to dry eye disease. Clinic Rev Allerg Immunol (2015) 49(3):288–97. doi: 10.1007/s12016-014-8438-7
6. Magon N, Kalra S. The orgasmic history of oxytocin: Love, lust, and labor. Indian J Endocrinol Metab (2011) 15(7):156. doi: 10.4103/2230-8210.84851
7. Gárriz A, Aubry S, Wattiaux Q, Bair J, Mariano M, Hatzipetrou G, et al. Role of the phospholipase c pathway and calcium mobilization in oxytocin-induced contraction of lacrimal gland myoepithelial cells. Invest Ophthalmol Visual Sci (2021) 62(14):25. doi: 10.1167/iovs.62.14.25s
8. Strakova Z, Soloff MS. Coupling of oxytocin receptor to G proteins in rat myometrium during labor: Gi receptor interaction. Am J Physiol Endocrinol Metab (1997) 272(5):E870–6. doi: 10.1152/ajpendo.1997.272.5.E870
9. Liu H, Gruber CW, Alewood PF, Möller A, Muttenthaler M. The oxytocin receptor signalling system and breast cancer: A critical review. Oncogene (2020) 39(37):5917–32. doi: 10.1038/s41388-020-01415-8
10. Busnelli M, Chini B. Molecular basis of oxytocin receptor signalling in the brain: What we know and what we need to know. In: Hurlemann R, Grinevich V, editors. Behavioral pharmacology of neuropeptides: Oxytocin. Cham: Springer International Publishing (2018). p. 3–29. doi: 10.1007/7854_2017_6
11. Gimpl G, Fahrenholz F. The oxytocin receptor system: Structure, function, and regulation. Physiol Rev (2001) 81(2):629–83. doi: 10.1152/physrev.2001.81.2.629
12. Gravati M, Busnelli M, Bulgheroni E, Reversi A, Spaiardi P, Parenti M, et al. Dual modulation of inward rectifier potassium currents in olfactory neuronal cells by promiscuous G protein coupling of the oxytocin receptor. J Neurochem (2010) 114(5):1424–35. doi: 10.1111/j.1471-4159.2010.06861.x
13. Guzzi F, Zanchetta D, Cassoni P, Guzzi V, Francolini M, Parenti M, et al. Localization of the human oxytocin receptor in caveolin-1 enriched domains turns the receptor-mediated inhibition of cell growth into a proliferative response. Oncogene (2002) 21(11):1658–67. doi: 10.1038/sj.onc.1205219
14. Rimoldi V, Reversi A, Taverna E, Rosa P, Francolini M, Cassoni P, et al. Oxytocin receptor elicits different EGFR/MAPK activation patterns depending on its localization in caveolin-1 enriched domains. Oncogene (2003) 22(38):6054–60. doi: 10.1038/sj.onc.1206612
15. Busnelli M, Saulière A, Manning M, Bouvier M, Galés C, Chini B. Functional selective oxytocin-derived agonists discriminate between individual G protein family subtypes. J Biol Chem (2012) 287(6):3617–29. doi: 10.1074/jbc.M111.277178
16. Grotegut CA, Feng L, Mao L, Heine RP, Murtha AP, Rockman HA. β-arrestin mediates oxytocin receptor signaling, which regulates uterine contractility and cellular migration. Am J Physiol Endocrinol Metab (2011) 300(3):E468–477. doi: 10.1152/ajpendo.00390.2010
17. Sinclair MS, Perea-Martinez I, Abouyared M, St. John SJ, Chaudhari N. Oxytocin decreases sweet taste sensitivity in mice. Physiol Behav (2015) 141:103–10. doi: 10.1016/j.physbeh.2014.12.048
18. Hammock E, Levitt P. Oxytocin receptor ligand binding in embryonic tissue and postnatal brain development of the C57BL/6J mouse. Front Behav Neurosci (2013) 7:195. doi: 10.3389/fnbeh.2013.00195
19. Halbach P, Pillers DAM, York N, Asuma MP, Chiu MA, Luo W, et al. Oxytocin expression and function in the posterior retina: A novel signaling pathway. Invest Ophthalmol Vis Sci (2015) 56(2):751–60. doi: 10.1167/iovs.14-15646
20. Grinevich V, Stoop R. Interplay between oxytocin and sensory systems in the orchestration of socio-emotional behaviors. Neuron (2018) 99(5):887–904. doi: 10.1016/j.neuron.2018.07.016
21. Nation DA, Szeto A, Mendez AJ, Brooks LG, Zaias J, Herderick EE, et al. Oxytocin attenuates atherosclerosis and adipose tissue inflammation in socially isolated ApoE–/– mice. Psychosomatic Med (2010) 72(4):376–82. doi: 10.1097/PSY.0b013e3181d74c48
22. Petersson M, Wiberg U, Lundeberg T, Uvnäs-Moberg K. Oxytocin decreases carrageenan induced inflammation in rats. Peptides (2001) 22(9):1479–84. doi: 10.1016/S0196-9781(01)00469-7
23. Işeri SO, Sener G, Sağlam B, Gedik N, Ercan F, Yeğen BC. Oxytocin ameliorates oxidative colonic inflammation by a neutrophil-dependent mechanism. Peptides (2005) 26(3):483–91. doi: 10.1016/j.peptides.2004.10.005
24. Goodin BR, Ness TJ, Robbins MT. Oxytocin – a multifunctional analgesic for chronic deep tissue pain. Curr Pharm Des (2015) 21(7):906–13. doi: 10.2174/1381612820666141027111843
25. Kubo A, Shinoda M, Katagiri A, Takeda M, Suzuki T, Asaka J, et al. Oxytocin alleviates orofacial mechanical hypersensitivity associated with infraorbital nerve injury through vasopressin-1A receptors of the rat trigeminal ganglia. PAIN (2017) 158(4):649–59. doi: 10.1097/j.pain.0000000000000808
26. Tzabazis A, Kori S, Mechanic J, Miller J, Pascual C, Manering N, et al. Oxytocin and migraine headache. Headache: J Head Face Pain (2017) 57(S2):64–75. doi: 10.1111/head.13082
27. Tzabazis A, Mechanic J, Miller J, Klukinov M, Pascual C, Manering N, et al. Oxytocin receptor: Expression in the trigeminal nociceptive system and potential role in the treatment of headache disorders. Cephalalgia (2016) 36(10):943–50. doi: 10.1177/0333102415618615
28. Takayanagi Y, Yoshida M, Takashima A, Takanami K, Yoshida S, Nishimori K, et al. Activation of supraoptic oxytocin neurons by secretin facilitates social recognition. Biol Psychiatry (2017) 81(3):243–51. doi: 10.1016/j.biopsych.2015.11.021
29. Welch MG, Welch-Horan TB, Anwar M, Anwar N, Ludwig RJ, Ruggiero DA. Brain effects of chronic IBD in areas abnormal in autism and treatment by single neuropeptides secretin and oxytocin. J Mol Neurosci (2005) 25(3):259–74. doi: 10.1385/JMN:25:3:259
30. Welch MG, Anwar M, Chang CY, Gross KJ, Ruggiero DA, Gershon MD. Combined administration of secretin and oxytocin inhibits chronic colitis and associated activation of forebrain neurons. Neurogastroenterol Motil (2010) 22(6):654–e202. doi: 10.1111/j.1365-2982.2010.01477.x
31. Hawley D, Tang X, Zyrianova T, Shah M, Janga S, Letourneau A, et al. Myoepithelial cell-driven acini contraction in response to oxytocin receptor stimulation is impaired in lacrimal glands of sjögren’s syndrome animal models | scientific reports. Sci Rep (2018) 8(9919). doi: 10.1038/s41598-018-28227-x
32. Abdelhakim A, Anwar M, Rosko L, Todaro J, Nagasaki T, Ludwig R, et al. The role of the oxytocin and secretin receptors in modulating inflammation in ocular surface tissues. Invest Ophthalmol Visual Sci (2017) 58(8). ARVO E-Abstract 3948.
33. Siedlecki AN, Smith SD, Siedlecki AR, Hayek SM, Sayegh RR. Ocular pain response to treatment in dry eye patients. Ocul Surf (2020) 18(2):305–11. doi: 10.1016/j.jtos.2019.12.004
34. Galor A, Moein HR, Lee C, Rodriguez A, Felix ER, Sarantopoulos KD, et al. Neuropathic pain and dry eye. Ocul Surf (2018) 16(1):31–44. doi: 10.1016/j.jtos.2017.10.001
35. Levitt AE, Galor A, Chowdhury AR, Felix ER, Sarantopoulos CD, Zhuang GY, et al. Evidence that dry eye represents a chronic overlapping pain condition. Mol Pain (2017) 13:1744806917729306. doi: 10.1177/1744806917729306
36. Galor A, Covington D, Levitt AE, McManus KT, Seiden B, Felix ER, et al. Neuropathic ocular pain due to dry eye is associated with multiple comorbid chronic pain syndromes. J Pain (2016) 17(3):310–8. doi: 10.1016/j.jpain.2015.10.019
37. Kalangara JP, Galor A, Levitt RC, Felix ER, Alegret R, Sarantopoulos CD. Burning eye syndrome: Do neuropathic pain mechanisms underlie chronic dry eye? Pain Med (2016) 17(4):746–55. doi: 10.1093/pm/pnv070
38. Du Z, Yin S, Song X, Zhang L, Yue S, Jia X, et al. Identification of differentially expressed genes and key pathways in the dorsal root ganglion after chronic compression. Front Mol Neurosci (2020) 13:71. doi: 10.3389/fnmol.2020.00071
39. Vehof J, Kozareva D, Hysi PG, Harris J, Nessa A, Williams FK, et al. Relationship between dry eye symptoms and pain sensitivity. JAMA Ophthalmol (2013) 131(10):1304–8. doi: 10.1001/jamaophthalmol.2013.4399
40. Galor A, Levitt RC, Felix ER, Martin ER, Sarantopoulos CD. Neuropathic ocular pain: An important yet underevaluated feature of dry eye. Eye (Lond) (2015) 29(3):301–12. doi: 10.1038/eye.2014.263
41. Rosenthal P, Borsook D. Ocular neuropathic pain. Br J Ophthalmol (2016) 100(1):128–34. doi: 10.1136/bjophthalmol-2014-306280
42. Spierer O, Felix ER, McClellan AL, Parel JM, Gonzalez A, Feuer WJ, et al. Corneal mechanical thresholds negatively associate with dry eye and ocular pain symptoms. Invest Ophthalmol Visual Sci (2016) 57(2):617–25. doi: 10.1167/iovs.15-18133
43. Galor A, Levitt RC, McManus KT, Kalangara JP, Seiden BE, Park JJ, et al. Assessment of somatosensory function in patients with idiopathic dry eye symptoms. JAMA Ophthalmol (2016) 134(11):1290–8. doi: 10.1001/jamaophthalmol.2016.3642
44. Li YX, An H, Wen Z, Tao ZY, Cao DY. Can oxytocin inhibit stress-induced hyperalgesia? Neuropeptides (2020) 79:101996. doi: 10.1016/j.npep.2019.101996
45. Gamal-Eltrabily M, Espinosa de Los Monteros-Zúñiga A, Manzano-García A, Martínez-Lorenzana G, Condés-Lara M, González-Hernández A. The rostral agranular insular cortex, a new site of oxytocin to induce antinociception. J Neurosci (2020) 40(29):5669–80. doi: 10.1523/JNEUROSCI.0962-20.2020
46. Sun W, Zhou Q, Ba X, Feng X, Hu X, Cheng X, et al. Oxytocin relieves neuropathic pain through GABA release and presynaptic TRPV1 inhibition in spinal cord. Front Mol Neurosci (2018) 11:248. doi: 10.3389/fnmol.2018.00248
47. Mou X, Fang J, Yang A, Du G. Oxytocin ameliorates bone cancer pain by suppressing toll-like receptor 4 and proinflammatory cytokines in rat spinal cord. J Neurogenet (2020) 34(2):216–22. doi: 10.1080/01677063.2019.1711077
48. García-Boll E, Martínez-Lorenzana G, Condés-Lara M, González-Hernández A. Inhibition of nociceptive dural input to the trigeminocervical complex through oxytocinergic transmission. Exp Neurol (2020) 323:113079. doi: 10.1016/j.expneurol.2019.113079
49. Mazzuca M, Minlebaev M, Shakirzyanova A, Tyzio R, Taccola G, Janackova S, et al. Newborn analgesia mediated by oxytocin during delivery. Front Cell Neurosci (2011) 5:3. doi: 10.3389/fncel.2011.00003
50. Zhu J, Li Y, Liang J, Li J, Huang K, Li J, et al. The neuroprotective effect of oxytocin on vincristine-induced neurotoxicity in mice. Toxicol Lett (2021) 340:67–76. doi: 10.1016/j.toxlet.2021.01.008
51. Meidahl AC, Eisenried A, Klukinov M, Cao L, Tzabazis AZ, Yeomans DC. Intranasal oxytocin attenuates reactive and ongoing, chronic pain in a model of mild traumatic brain injury. Headache (2018) 58(4):545–58. doi: 10.1111/head.13248
52. Hu B, Boyle CA, Lei S. Oxytocin receptors excite lateral nucleus of central amygdala by phospholipase cβ- and protein kinase c-dependent depression of inwardly rectifying k+ channels. J Physiol (2020) 598(16):3501–20. doi: 10.1113/JP279457
53. Ando M, Hayashi Y, Hitomi S, Shibuta I, Furukawa A, Oto T, et al. Oxytocin-dependent regulation of TRPs expression in trigeminal ganglion neurons attenuates orofacial neuropathic pain following infraorbital nerve injury in rats. Int J Mol Sci (2020) 21(23):9173. doi: 10.3390/ijms21239173
54. García-Boll E, Martínez-Lorenzana G, Condés-Lara M, González-Hernández A. Oxytocin inhibits the rat medullary dorsal horn Sp5c/C1 nociceptive transmission through OT but not V1A receptors. Neuropharmacology (2018) 129:109–17. doi: 10.1016/j.neuropharm.2017.11.031
55. Neugebauer V, Mazzitelli M, Cragg B, Ji G, Navratilova E, Porreca F. Amygdala, neuropeptides, and chronic pain-related affective behaviors. Neuropharmacology (2020) 170:108052. doi: 10.1016/j.neuropharm.2020.108052
56. Eliava M, Melchior M, Knobloch-Bollmann HS, Wahis J, da Silva Gouveia M, Tang Y, et al. A new population of parvocellular oxytocin neurons controlling magnocellular neuron activity and inflammatory pain processing. Neuron (2016) 89(6):1291–304. doi: 10.1016/j.neuron.2016.01.041
57. Noma D, Fujita S, Zama M, Mayahara K, Motoyoshi M, Kobayashi M. Application of oxytocin with low-level laser irradiation suppresses the facilitation of cortical excitability by partial ligation of the infraorbital nerve in rats: An optical imaging study. Brain Res (2020) 1728:146588. doi: 10.1016/j.brainres.2019.146588
58. Goodin BR, Anderson AJB, Freeman EL, Bulls HW, Robbins MT, Ness TJ. Intranasal oxytocin administration is associated with enhanced endogenous pain inhibition and reduced negative mood states. Clin J Pain (2015) 31(9):757–67. doi: 10.1097/AJP.0000000000000166
59. Wang YL, Yuan Y, Yang J, Wang CH, Pan YJ, Lu L, et al. The interaction between the oxytocin and pain modulation in headache patients. Neuropeptides (2013) 47(2):93–7. doi: 10.1016/j.npep.2012.12.003
60. Yu L, Yu C, Dong H, Mu Y, Zhang R, Zhang Q, et al. Recent developments about the pathogenesis of dry eye disease: Based on immune inflammatory mechanisms. Front Pharmacol (2021) 12:732887. doi: 10.3389/fphar.2021.732887
61. Clodi M, Vila G, Geyeregger R, Riedl M, Stulnig TM, Struck J, et al. Oxytocin alleviates the neuroendocrine and cytokine response to bacterial endotoxin in healthy men. Am J Physiol Endocrinol Metab (2008) 295(3):E686–691. doi: 10.1152/ajpendo.90263.2008
62. Poutahidis T, Kearney SM, Levkovich T, Qi P, Varian BJ, Lakritz JR, et al. Microbial symbionts accelerate wound healing via the neuropeptide hormone oxytocin. PLoS One (2013) 8(10):e78898. doi: 10.1371/journal.pone.0078898
63. Cetinel S, Hancioğlu S, Sener E, Uner C, Kiliç M, Sener G, et al. Oxytocin treatment alleviates stress-aggravated colitis by a receptor-dependent mechanism. Regul Pept (2010) 160(1–3):146–52. doi: 10.1016/j.regpep.2009.11.011
64. Wang Y, Zhao S, Liu X, Zheng Y, Li L, Meng S. Oxytocin improves animal behaviors and ameliorates oxidative stress and inflammation in autistic mice. BioMed Pharmacother (2018) 107:262–9. doi: 10.1016/j.biopha.2018.07.148
65. Garrido-Urbani S, Deblon N, Poher AL, Caillon A, Ropraz P, Rohner-Jeanrenaud F, et al. Inhibitory role of oxytocin on TNFα expression assessed in vitro and in vivo. Diabetes Metab (2018) 44(3):292–5. doi: 10.1016/j.diabet.2017.10.004
66. Szeto A, Cecati M, Ahmed R, McCabe PM, Mendez AJ. Oxytocin reduces adipose tissue inflammation in obese mice. Lipids Health Dis (2020) 19(1):188. doi: 10.1186/s12944-020-01364-x
67. Yu Y, Li J, Liu C. Oxytocin suppresses epithelial cell-derived cytokines production and alleviates intestinal inflammation in food allergy. Biochem Pharmacol (2022) 195:114867. doi: 10.1016/j.bcp.2021.114867
68. Welch MG, Margolis KG, Li Z, Gershon MD. Oxytocin regulates gastrointestinal motility, inflammation, macromolecular permeability, and mucosal maintenance in mice. Am J Physiol Gastrointest Liver Physiol (2014) 307(8):G848–862. doi: 10.1152/ajpgi.00176.2014
69. Klein BY, Tamir H, Welch MG. PI3K/Akt responses to oxytocin stimulation in Caco2BB gut cells. J Cell Biochem (2011) 112(11):3216–26. doi: 10.1002/jcb.23243
70. Klein BY, Tamir H, Hirschberg DL, Ludwig RJ, Glickstein SB, Myers MM, et al. Oxytocin opposes effects of bacterial endotoxin on ER-stress signaling in Caco2BB gut cells. Biochim Biophys Acta (2016) 1860(2):402–11. doi: 10.1016/j.bbagen.2015.10.025
71. Spangelo BL, deHoll PD, Kalabay L, Bond BR, Arnaud P. Neurointermediate pituitary lobe cells synthesize and release interleukin-6 in vitro: effects of lipopolysaccharide and interleukin-1 beta. Endocrinology (1994) 135(2):556–63. doi: 10.1210/endo.135.2.8033802
72. Yang J, Liu Y, Xu Y, Li X, Fu J, Jiang X, et al. A new approach of ocular nebulization with vitamin B12 versus oxytocin for the treatment of dry eye disease: an in vivo confocal microscopy study. Drug Des Devel Ther (2019) 13:2381–91. doi: 10.2147/DDDT.S203464
73. Gilbard JP, Rossi SR, Heyda KG, Dartt DA. Stimulation of tear secretion by topical agents that increase cyclic nucleotide levels. Invest Ophthalmol Vis Sci (1990) 31(7):1381–8.
74. Inoue T, Yamakage H, Tanaka M, Kusakabe T, Shimatsu A, Satoh-Asahara N. Oxytocin suppresses inflammatory responses induced by lipopolysaccharide through inhibition of the eIF-2-ATF4 pathway in mouse microglia. Cells (2019) 8(6):E527. doi: 10.3390/cells8060527
75. Li T, Wang P, Wang SC, Wang YF. Approaches mediating oxytocin regulation of the immune system. Front Immunol (2017) 7:693. doi: 10.3389/fimmu.2016.00693
76. Bordt EA, Smith CJ, Demarest TG, Bilbo SD, Kingsbury MA. Mitochondria, oxytocin, and vasopressin: Unfolding the inflammatory protein response. Neurotox Res (2019) 36(2):239–56. doi: 10.1007/s12640-018-9962-7
77. Klein BY, Tamir H, Hirschberg DL, Glickstein SB, Welch MG. Oxytocin modulates mTORC1 pathway in the gut. Biochem Biophys Res Commun (2013) 432(3):466–71. doi: 10.1016/j.bbrc.2013.01.121
78. Acera A, Rocha G, Vecino E, Lema I, Durán JA. Inflammatory markers in the tears of patients with ocular surface disease. Ophthal Res (2008) 40(6):315–21. doi: 10.1159/000150445
79. Pflugfelder SC, Jones D, Ji Z, Afonso A, Monroy D. Altered cytokine balance in the tear fluid and conjunctiva of patients with sjögren’s syndrome keratoconjunctivitis sicca. Curr Eye Res (1999) 19(3):201–11. doi: 10.1076/ceyr.19.3.201.5309
80. Yoon KC, Jeong IY, Park YG, Yang SY. Interleukin-6 and tumor necrosis factor-alpha levels in tears of patients with dry eye syndrome. Cornea (2007) 26(4):431–7. doi: 10.1097/ICO.0b013e31803dcda2
81. Bai JJ, Tan CD, Chow BKC. Secretin, at the hub of water-salt homeostasis. Am J Physiol Renal Physiol (2017) 312(5):F852–60. doi: 10.1152/ajprenal.00191.2015
82. Velmurugan S, Brunton PJ, Leng G, Russell JA. Circulating secretin activates supraoptic nucleus oxytocin and vasopressin neurons via noradrenergic pathways in the rat. Endocrinology (2010) 151(6):2681–8. doi: 10.1210/en.2009-1440
83. Simmons KT, Xiao Y, Pflugfelder SC, de Paiva CS. Inflammatory response to lipopolysaccharide on the ocular surface in a murine dry eye model. Invest Ophthalmol Visual Sci (2016) 57(6):2443–51. doi: 10.1167/iovs.15-18396
84. Nakamachi T, Ohtaki H, Seki T, Yofu S, Kagami N, Hashimoto H, et al. PACAP suppresses dry eye signs by stimulating tear secretion. Nat Commun (2016) 7:12034. doi: 10.1038/ncomms12034
85. Shioda S, Takenoya F, Hirabayashi T, Wada N, Seki T, Nonaka N, et al. Effects of PACAP on dry eye symptoms, and possible use for therapeutic application. J Mol Neurosci (2019) 68(3):420–6. doi: 10.1007/s12031-018-1087-1
86. Smith AS, Korgan AC, Young WS. Oxytocin delivered nasally or intraperitoneally reaches the brain and plasma of normal and oxytocin knockout mice. Pharmacol Res (2019) 146:104324. doi: 10.1016/j.phrs.2019.104324
87. Lee MR, Shnitko TA, Blue SW, Kaucher AV, Winchell AJ, Erikson DW, et al. Labeled oxytocin administered via the intranasal route reaches the brain in rhesus macaques | nature communications. Nat Commun (2020) 11(2783). doi: 10.1038/s41467-020-15942-1
88. Chiou GC, Shen ZF, Zheng YQ. Systemic absorption of oxytocin and vasopressin through eyes in rabbits. J Ocul Pharmacol (1991) 7(4):351–9. doi: 10.1089/jop.1991.7.351
89. MacDonald E, Dadds MR, Brennan JL, Williams K, Levy F, Cauchi AJ. A review of safety, side-effects and subjective reactions to intranasal oxytocin in human research. Psychoneuroendocrinology (2011) 36(8):1114–26. doi: 10.1016/j.psyneuen.2011.02.015
90. Mizuno A, Cherepanov SM, Kikuchi Y, Fakhrul AA, Akther S, Deguchi K, et al. Lipo-oxytocin-1, a novel oxytocin analog conjugated with two palmitoyl groups, has long-lasting effects on anxiety-related behavior and social avoidance in CD157 knockout mice. Brain Sci (2015) 5(1):3–13. doi: 10.3390/brainsci5010003
91. Ichinose W, Cherepanov SM, Shabalova AA, Yokoyama S, Yuhi T, Yamaguchi H, et al. Development of a highly potent analogue and a long-acting analogue of oxytocin for the treatment of social impairment-like behaviors. J Med Chem (2019) 62(7):3297–310. doi: 10.1021/acs.jmedchem.8b01691
92. Cid-Jofré V, Moreno M, Reyes-Parada M, Renard GM. Role of oxytocin and vasopressin in neuropsychiatric disorders: Therapeutic potential of agonists and antagonists. Int J Mol Sci (2021) 22(21):12077. doi: 10.3390/ijms222112077
93. Chao YS, McCormack S. Carbetocin for the prevention of post-partum hemorrhage: A Review of Clinical Effectiveness, Cost-Effectiveness, and Guidelines [Internet]. Ottawa (ON): Canadian Agency for Drugs and Technologies in Health. Available from: https://www.ncbi.nlm.nih.gov/books/NBK546425/
94. Hilfiger L, Zhao Q, Kerspern D, Inquimbert P, Andry V, Goumon Y, et al. A nonpeptide oxytocin receptor agonist for a durable relief of inflammatory pain. Sci Rep (2020) 10(1):3017. doi: 10.1038/s41598-020-59929-w
95. Dengler DG, Sun Q, Holleran J, Pollari S, Beutel J, Brown BT, et al. Development of a testing funnel for identification of small-molecule modulators targeting secretin receptors. SLAS Discov (2021) 26(1):1–16. doi: 10.1177/2472555220945284
96. Dengler DG, Harikumar KG, Pollari S, Sun Q, Brown BT, Shinoki-Iwaya A, et al. Discovery of small molecule positive allosteric modulators of the secretin receptor. Biochem Pharmacol (2021) 185:114451. doi: 10.1016/j.bcp.2021.114451
Keywords: oxytocin, secretin, ocular pain, anti-inflammatory, dry eye syndrome
Citation: Lopez JB, Chang C-C, Kuo Y-M, Chan MF and Winn BJ (2022) Oxytocin and secretin receptors – implications for dry eye syndrome and ocular pain. Front. Ophthalmol. 2:948481. doi: 10.3389/fopht.2022.948481
Received: 19 May 2022; Accepted: 11 July 2022;
Published: 01 August 2022.
Edited by:
Driss Zoukhri, Tufts University School of Medicine, United StatesReviewed by:
Luping Wang, Capital Medical University, ChinaCopyright © 2022 Lopez, Chang, Kuo, Chan and Winn. This is an open-access article distributed under the terms of the Creative Commons Attribution License (CC BY). The use, distribution or reproduction in other forums is permitted, provided the original author(s) and the copyright owner(s) are credited and that the original publication in this journal is cited, in accordance with accepted academic practice. No use, distribution or reproduction is permitted which does not comply with these terms.
*Correspondence: Bryan J. Winn, QnJ5YW4uV2lubkB1Y3NmLmVkdQ==
†ORCID: Bryan J. Winn, orcid.org/0000-0002-5609-3723
Disclaimer: All claims expressed in this article are solely those of the authors and do not necessarily represent those of their affiliated organizations, or those of the publisher, the editors and the reviewers. Any product that may be evaluated in this article or claim that may be made by its manufacturer is not guaranteed or endorsed by the publisher.
Research integrity at Frontiers
Learn more about the work of our research integrity team to safeguard the quality of each article we publish.