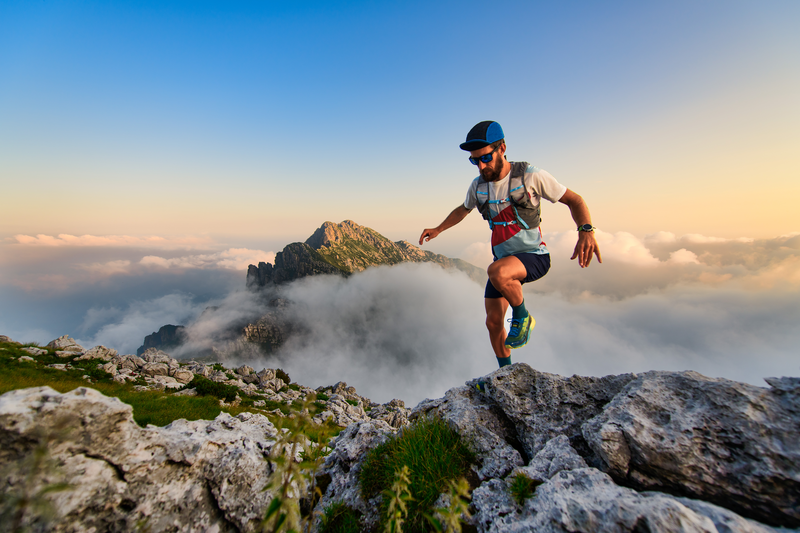
94% of researchers rate our articles as excellent or good
Learn more about the work of our research integrity team to safeguard the quality of each article we publish.
Find out more
EDITORIAL article
Front. Oncol. , 11 February 2025
Sec. Cancer Molecular Targets and Therapeutics
Volume 15 - 2025 | https://doi.org/10.3389/fonc.2025.1550672
This article is part of the Research Topic Drug Repurposing for Cancer Treatment: Current and Future Directions View all 5 articles
Editorial on the Research Topic
Drug repurposing for cancer treatment: current and future directions
Cancer is a leading cause of morbidity and death worldwide, with approximately 20 million new cancer cases reported annually (1). Chemotherapy, surgery, and radiotherapy are the mainstay of cancer treatment (2). Despite significant progress in the development of novel chemotherapy agents, the process of developing new chemotherapy agents is time- and money-consuming. In specific, it is estimated that in average, the development of a new drug requires 10 years from discovery to market delivery and approximately 1 billion dollars, while only a minority of the initially promising drugs receive market approval (3). Drug repurposing for cancer treatment is an optimal alternative since promising repurposed drugs have been previously tested for their safety and pharmacokinetic properties and may be directly tested in phase 3 clinical trials (4).
Antipsychotic drugs are psychotropic medications utilized primarily for the treatment of psychotic disorders, including schizophrenia and bipolar disorders (5). These drugs act primarily by mediating the positive psychotic symptoms by inhibiting dopamine receptors in the mesolimbic dopaminergic pathway (5, 6). The first generation of antipsychotic drugs, also called typical antipsychotics, act by inhibiting D2 dopamine receptors (DRD2), while the second-generation antipsychotic drugs act by inhibiting DRD2 and serotonin, 5-HT2A receptors. The observation that the incidence of cancer was reduced in patients receiving chemotherapeutic medications compared to general population led to the assumption that antipsychotic medication may have anti-cancer properties. To date, several studies have been performed to study the potential anti-cancer mechanisms of the antipsychotic medications (6).
Haloperidol is the most frequently utilized first-generation antipsychotic medication and today is primarily utilized for the treatment of acute psychosis and delirium (7). It is a non-selective inhibitor of DRD2 and has an affinity for multiple receptors, including anti-muscarinic, anti-adrenergic, and anti-histaminic properties (7, 8). Regarding its role as a repurposing drug, it has been tested for its anti-cancer and anti-microbiological effects (8). Haloperidol has been found to induce apoptosis, mediate autophagy, and cause cell cycle arrest in several cancer types, including glioblastoma and pancreatic cancer (9). Several potential mechanisms have been described, including the reduction in the expression of adhesion molecules in cancer cells, the downregulation of Sonic Hedgehog Pathway (SHH), and the Extracellular signal-regulated kinase pathway (ERK) (6). Moreover, haloperidol has a synergic effect with the alkylating agent temozolamide, the primary chemotherapeutic agent utilized in patients with glioblastoma. In a recent study, Shi et al. reported that this synergic effect was caused by increasing endoplasmic reticulum stress, which leads to autophagy and ferroptosis (10).
Trifluoperazine is another typical antipsychotic that has been found to have anti-neoplastic properties and suppress tumor growth in several types of cancer, including glioblastoma, hepatocellular carcinoma, breast, colorectal, and lung cancer (6). Regarding its effect on glioblastoma, trifluoperazine has been found to enhance the radiosensitivity of glioma cells in vitro via decreasing the radiation-induced-Nanog expression (11). Moreover, trifluoperazine was found to induce the activation of FOXO1 transcription factor in lung cancer, hepatocellular carcinoma, and glioma cell lines that suppress the expression of multi-drug resistance (MDR) genes, including P glycoprotein, and to increase the sensitivity of these cancer cells in chemotherapy agents (12–14).
Chlorpromazine is a phenothiazine class antipsychotic drug associated with anti-cancer effects in glioblastoma, white blood cell malignancies, oral cancer, Ewing sarcoma, and colorectal cancer (6, 15–17). Specifically, chlorpromazine has been found to decrease cell growth and induce autophagic cell death and apoptosis (15, 16) In glioma cell lines, chlorpromazine was found to downregulate the expression of stemness genes such as NANOG and promote autophagy via inhibition of the Akt/mTOR pathway (16, 17). Additionally, chlorpromazine induces apoptosis in various leukemia types, including B and T cell malignancies, by inhibiting the mitochondrial DNA polymerase (15). Finally, chlorpromazine induced a mitotic arrest via inhibition of mitotic kinesin in-vivo and in-vitro in colorectal cell lines (18).
The repurposing role of penfluridol as an anti-cancer medication has been experimentally studied in multiple studies in various cancer types, including melanoma, glioblastoma, breast, pancreatic, and lung cancer (6, 19). Mechanistically, penfluridol induces apoptosis and autophagy and inhibits cancer cell invasion. Interestingly, penfluridol has been found to downregulate integrin expression, thus reducing cancer cells’ invasiveness and metastatic potential (20, 21). Moreover, penfluridol has been found to reduce the expression of GLI-1, a transcription factor overexpressed in glioma cells involved in chromatin regulation. GLI-1 downregulates the expression of transcription factors such as SOX2 and reduces the expression of proteins involved in cell invasion, including integrins and epithelial to mesenchymal transition (22). Penfluridol also suppresses the HER2/b-catenin signaling pathway in breast cancer cell lines. HER2/-catenin is an important pathway involved in cell survival, and this pathway is the pharmaceutic target of paclitaxel, a widely utilized chemotherapeutic drug. Of note, Gupta et al. found that penfluridol enhanced the efficacy of paclitaxel in in-vivo breast cancer models (23).
Thioridazine is a phenothiazine antipsychotic drug that is probably the most studied antipsychotic drug regarding its role in multiple cancer types, including breast, lung, gastrointestinal, hepatocellular, prostate, renal, breast, testicular, hematological, and gynecological cancers (6, 24–26). The anti-tumor effect of thioridazine seems to be mediated through the suppression of angiogenesis, cell cycle arrest, induction of autophagy, and apoptosis (6, 24). Suppression of angiogenesis is mediated by the inhibition of Vascular Endothelial Growth Factor (VEGF) via various mechanisms, including downregulation of PI3/Akt and VEGF/PIEK/mTOR pathway and inhibition of Hypoxia Inducing Factor (HIF)-1a (24, 27, 28). Thioridazine-induced downregulation of PI3/Akt pathway in cancer cell lines has also induced autophagy, apoptosis, and cell cycle arrest. The promising results of experimental studies led to the conduction of a Phase 1 clinical trial evaluating the effect of the combination of thioridazine and cytarabine in acute myeloid leukemia, in which a reduction of about 50% in the blast cells was found in most patients (29).
Several other typical and atypical antipsychotics have been studied regarding their potential role in cancer treatment. To date, the main body of research is currently focused on typical antipsychotics compared to atypical antipsychotics. The latter is probably explained by the increased affinity of typical antipsychotics for DRD2 receptors, which are implicated in tumorigenesis. However, during the last decade, increased reports regarding the potential role of atypical antipsychotics in cancer treatment have been accumulated. Olanzapine is a first-line atypical antipsychotic agent widely utilized for various psychiatric diseases worldwide. Olanzapine has been reported to enhance the therapeutic effect of temozolomide in glioblastoma cell lines (30). A similar synergic effect of temozolomide with quetiapine in glioma cell lines, another first-line antipsychotic drug, has also been observed (31). Preliminary promising results were also reported for various cancer types for other atypical antipsychotics, including risperidone for prostate (32) and breast cancer (33), aripiprazole for breast (34), and lung cancer (35). Finally, clozapine, a very effective antipsychotic medication for resistant psychosis but with an unfavorable safety profile because it is associated with significant adverse effects, including neutropenia and cardiomyopathy, has been found to induce G0/G1 cell cycle arrest in lung cancer (36) and melanoma cell lines (37).
Except for their experimental study as sole anti-cancer medication, antipsychotics are frequently studied for their synergic effect with chemotherapy drugs (6) Temozolomide (TMZ) is an alkylating agent broadly utilized as an adjuvant glioblastoma treatment (6, 10). Haloperidol, penfliridol, quetiapine, and olanzapine are among the antipsychotic drugs that have been found to have a synergic effect with TMZ in the treatment of GBM (10, 38). Several mechanisms have been proposed to explain this effect, including inhibition of the extracellular signal-related kinase (ERK), Cco subunit 4 isoform 1 (COX4-1), and Cx43, a molecule essential in DNA repair (22, 31, 38). Doxorubicin is an anthracycline-based chemotherapy medication widely used to treat hematologic and solid cancers. The cytotoxic effect of doxorubicin seems to be enhanced when combined with trifluoperazine via a mechanism that involves transnuclear translocation of FOXO1, a transcription factor that suppresses the expression of multidrug resistance (MDR) genes (13, 39).
Despite the efficacy of antipsychotic medication in the treatment of various psychiatric disorders, their use is associated with significant side effects (40). First-generation antipsychotics, or typical antipsychotics, cause significant sedation and extrapyramidal symptoms mediated via inhibition of the D2 receptors. Thus, their broad use in the past caused impairment in the quality of life of patients with psychiatric disorders (41). The development of the second generation, or atypical antipsychotics, which have a more favorable side effect profile with minimal extrapyramidal symptoms, led to the replacement of the typical antipsychotics (42). However, atypical antipsychotics also cause some degree of sedation and are associated with metabolic disturbances, including weight gain and risk for insulin resistance and diabetes development (6, 42). These factors, in addition to the antipsychotic-related stigma in society, may be potential factors that could limit the enrollment of cancer patients in future promising clinical trials (43). Future studies should consider all the limitation and toxicity associated with the use of antipsychotics as anti-cancer medication. However, the pharmacokinetic properties and the toxicity of antipsychotic drugs are well recognized by both clinicians and researchers due to their broad use (44). Hence, optimal dose adjustment and symptoms management may not be a significant obstacle. Moreover, recent data support that the use of typical antipsychotics may improve the anorexia of patients with advanced cancer and may be utilized as a part of palliative treatment (45).
Over the last two decades, mounting evidence from experimental studies has indicated the promising role of antipsychotics in cancer treatment. Antipsychotics seem to have a dual role in cancer treatment: They directly inhibit tumor growth, induce apoptosis, and enhance the sensitivity of chemotherapy agents. However, despite the increased pre-clinical evidence, only three early-phase (I/II) clinical trials (NCT04224441, NCT02096289) have been conducted so far. One potential reason for the latter is that typical antipsychotics, the leading group of antipsychotics targeted for cancer repurposing, have significant side effects such as extrapyramidal symptoms, sedation, and anticholinergic effects, that may not be optimal for cancer patients with an increased morbidity. Hence, more pre-clinical and clinical studies should be performed both in first and second-generation antipsychotics to clarify the exact role of antipsychotics as repurposing drugs for cancer treatment.
ML: Conceptualization, Writing – original draft, Writing – review & editing. NV: Conceptualization, Writing – original draft. GL: Conceptualization, Writing – original draft, Writing – review & editing. CB: Conceptualization, Writing – review & editing. GA: Supervision, Validation, Writing – review & editing.
The authors declare that the research was conducted in the absence of any commercial or financial relationships that could be construed as a potential conflict of interest.
All claims expressed in this article are solely those of the authors and do not necessarily represent those of their affiliated organizations, or those of the publisher, the editors and the reviewers. Any product that may be evaluated in this article, or claim that may be made by its manufacturer, is not guaranteed or endorsed by the publisher.
1. Sung H, Ferlay J, Siegel RL, Laversanne M, Soerjomataram I, Jemal A, et al. Global cancer statistics 2020: GLOBOCAN estimates of incidence and mortality worldwide for 36 cancers in 185 countries. CA Cancer J Clin. (2021) 71:209–49. doi: 10.3322/caac.21660
2. DeVita VT Jr, Rosenberg SA. Two hundred years of cancer research. N Engl J Med. (2012) 366:2207–14. doi: 10.1056/NEJMra1204479
3. Ciociola AA, Cohen LB, Kulkarni P, Kefalas C, Buchman A, Burke C, et al. How drugs are developed and approved by the FDA: current process and future directions. Am J Gastroenterol. (2014) 109:620–3. doi: 10.1038/ajg.2013.407
4. Xia Y, Sun M, Huang H, Jin WL. Drug repurposing for cancer therapy. Sig Transduct Target Ther. (2024) 9:92. doi: 10.1038/s41392-024-01808-1
5. Huhn M, Nikolakopoulou A, Schneider-Thoma J, Krause M, Samara M, Peter N, et al. Comparative efficacy and tolerability of 32 oral antipsychotics for the acute treatment of adults with multi-episode schizophrenia: a systematic review and network meta-analysis [published correction appears in Lancet. Lancet. (2019) 394:939–51. doi: 10.1016/S0140-6736(19)31135-3
6. Vlachos N, Lampros M, Voulgaris S, Alexiou GA. Repurposing antipsychotics for cancer treatment. Biomedicines. (2021) 9:1785. doi: 10.3390/biomedicines9121785
7. Rahman S, Marwaha R. Haloperidol. In: StatPearls. StatPearls Publishing, Treasure Island (FL (2023).
8. Hoertel N, Sánchez-Rico M, Vernet R, Jannot A-S, Neuraz A, Blanco C, et al. Observational study of haloperidol in hospitalized patients with COVID-19. PloS One. (2021) 16:e0247122. doi: 10.1371/journal.pone.0247122
9. Papadopoulos F, Isihou R, Alexiou GA, Tsalios T, Vartholomatos E, Markopoulos GS, et al. Haloperidol induced cell cycle arrest and apoptosis in glioblastoma cells. Biomedicines. (2020) 8:595. doi: 10.3390/biomedicines8120595
10. Shi L, Chen H, Chen K, Zhong C, Song C, Huang Y, et al. The DRD2 antagonist haloperidol mediates autophagy-induced ferroptosis to increase temozolomide sensitivity by promoting endoplasmic reticulum stress in glioblastoma. Clin Cancer Res. (2023) 29:3172–88. doi: 10.1158/1078-0432.CCR-22-3971
11. Bhat K, Saki M, Vlashi E, Cheng F, Duhachek-Muggy S, Alli C, et al. The dopamine receptor antagonist trifluoperazine prevents phenotype conversion and improves survival in mouse models of glioblastoma. Proc Natl Acad Sci USA. (2020) 117:11085–96. doi: 10.1073/pnas.1920154117
12. Jiang J, Huang Z, Chen X, Luo R, Cai H, Wang H, et al. Trifluoperazine activates FOXO1-related signals to inhibit tumor growth in hepatocellular carcinoma. DNA Cell Biol. (2017) 36:813–21. doi: 10.1089/dna.2017.3790
13. Chen X, Luo X, Cheng Y. Trifluoperazine prevents FOXO1 nuclear excretion and reverses doxorubicin-resistance in the SHG44/DOX drug-resistant glioma cell line. Int J Mol Med. (2018) 42:3300–8. doi: 10.3892/ijmm.2018.3885
14. Yeh C-T, Wu A, Chang PM-H, Chen K-Y, Yang C-N, Yang S-C, et al. Trifluoperazine, an antipsychotic agent, inhibits cancer stem cell growth and overcomes drug resistance of lung cancer. Am J Respir Crit Care Med. (2012) 186:1180–8. doi: 10.1164/rccm.201207-1180OC
15. Zhelev Z, Ohba H, Bakalova R, Hadjimitova V, Ishikawa M, Shinohara Y, et al. Phenothiazines suppress proliferation and induce apoptosis in cultured leukemic cells without any influence on the viability of normal lymphocytes. Cancer Chemother Pharmacol. (2004) 53:267–75. doi: 10.1007/s00280-003-0738-1
16. Shin SY, Lee KS, Choi Y-K, Lim HJ, Lee HG, Lim Y, et al. The antipsychotic agent chlorpromazine induces autophagic cell death by inhibiting the Akt/mTOR pathway in human U-87MG glioma cells. Carcinogenesis. (2013) 34:2080–9. doi: 10.1093/carcin/bgt169
17. Matteoni S, Matarrese P, Ascione B, Buccarelli M, Ricci-Vitiani L, Pallini R, et al. Anticancer properties of the antipsychotic drug chlorpromazine and its synergism with temozolomide in restraining human glioblastoma proliferation in vitro. Front Oncol. (2021) 11:635472. doi: 10.3389/fonc.2021.635472
18. Martins AS, Ordóñez JL, Amaral AT, Prins F, Floris G, Debiec-Rychter M, et al. IGF1R signaling in ewing sarcoma is shaped by clathrin-/caveolin-dependent endocytosis. PloS One. (2011) 6:e19846. doi: 10.1371/journal.pone.0019846
19. Tuan NM, Lee CH. Penfluridol as a candidate of drug repurposing for anticancer agent. Molecules. (2019) 24:3659. doi: 10.3390/molecules24203659
20. Ranjan A, Gupta P, Srivastava SK. Penfluridol: an antipsychotic agent suppresses metastatic tumor growth in triple-negative breast cancer by inhibiting integrin signaling axis. Cancer Res. (2015) 76:877–90. doi: 10.1158/0008-5472.CAN-15-1233
21. Hedrick E, Li X, Safe S. Penfluridol represses integrin expression in breast cancer through induction of reactive oxygen species and downregulation of sp transcription factors. Mol Cancer Ther. (2016) 16:205–16. doi: 10.1158/1535-7163.MCT-16-0451
22. Kim H, Chong K, Ryu BK, Park KJ, Yu MO, Lee J, et al. Repurposing penfluridol in combination with temozolomide for the treatment of glioblastoma. Cancers. (2019) 11:1310. doi: 10.3390/cancers11091310
23. Gupta N, Gupta P, Srivastava SK. Penfluridol overcomes paclitaxel resistance in metastatic breast cancer. Sci Rep. (2019) 9:5066. doi: 10.1038/s41598-019-41632-0
24. Cheng HW, Liang YH, Kuo YL, Chuu CP, Lin CY, Lee MH, et al. Identification of thioridazine, an antipsychotic drug, as an antiglioblastoma and anticancer stem cell agent using public gene expression data. Cell Death Dis. (2015) 6:e1753. doi: 10.1038/cddis.2015.77
25. Tegowski M, Fan C, Baldwin AS. Thioridazine inhibits self-renewal in breast cancer cells via DRD2-dependent STAT3 inhibition, but induces a G1 arrest independent of DRD2. J Biol Chem. (2018) 293:15977–90. doi: 10.1074/jbc.RA118.003719
26. Shen J, Ma B, Zhang X, Sun X, Han J, Wang Y, et al. Thioridazine has potent antitumor effects on lung cancer stem-like cells. Oncol Lett. (2017) 13:1563–8. doi: 10.3892/ol.2017.5651
27. Yong M, Yu T, Tian S, Liu S, Xu J, Hu J, et al. DR2 blocker thioridazine: A promising drug for ovarian cancer therapy. Oncol Lett. (2017) 14:8171–7. doi: 10.3892/ol.2017.7184
28. Meng Q, Sun X, Wang J, Wang Y, Wang L. The important application of thioridazine in the endometrial cancer. Am J Transl Res. (2016) 8:2767–75. ISSN:1943-8141/AJTR0025191
29. Aslostovar L, Boyd AL, Almakadi M, Collins TJ, Leong DP, Tirona RG, et al. A phase 1 trial evaluating thioridazine in combination with cytarabine in patients with acute myeloid leukemia. Blood Adv. (2018) 2:1935–45. doi: 10.1182/bloodadvances.2018015677
30. Karpel-Massler G, Kast RE, Westhoff M-A, Dwucet A, Welscher N, Nonnenmacher L, et al. Olanzapine inhibits proliferation, migration and anchorage-independent growth in human glioblastoma cell lines and enhances temozolomide’s antiproliferative effect. J Neuro-Oncol. (2014) 122:21–33. doi: 10.1007/s11060-014-1688-7
31. Wang Y, Huang N, Li H, Liu S, Chen X, Yu S, et al. Promoting oligodendroglial-oriented differentiation of glioma stem cell: A repurposing of quetiapine for the treatment of Malignant glioma. Oncotarget. (2017) 8:37511–24. doi: 10.18632/oncotarget.16400
32. Dilly SJ, Clark AJ, Marsh A, Mitchell DA, Cain R, Fishwick CW, et al. A chemical genomics approach to drug reprofiling in oncology: Antipsychotic drug risperidone as a potential adenocarcinoma treatment. Cancer Lett. (2017) 393:16–21. doi: 10.1016/j.canlet.2017.01.042
33. Wang J-S, Zhu H-J, Markowitz JS, Donovan JL, Yuan H-J, DeVane CL. Antipsychotic drugs inhibit the function of breast cancer resistance protein. Basic Clin Pharmacol Toxicol. (2008) 103:336–41. doi: 10.1111/j.1742-7843.2008.00298.x
34. Badran A, Tul-Wahab A, Zafar H, Mohammad N, Imad R, Khan MA, et al. Antipsychotics drug aripiprazole as a lead against breast cancer cell line (MCF-7) in vitro. PloS One. (2020) 15:e0235676. doi: 10.1371/journal.pone.0235676
35. Suzuki S, Okada M, Kuramoto K, Takeda H, Sakaki H, Watarai H, et al. Aripiprazole, an antipsychotic and partial dopamine agonist, inhibits cancer stem cells and reverses chemoresistance. Anticancer Res. (2016) 36:5153–62. doi: 10.21873/anticanres.11085
36. Yin Y-C, Lin C-C, Chen T-T, Chen J-Y, Tsai H-J, Wang C-Y, et al. Clozapine induces autophagic cell death in non-small cell lung cancer cells. Cell Physiol Biochem. (2015) 35:945–56. doi: 10.1159/000369751
37. Massari NA, Medina VA, Cricco GP, Lamas DJM, Sambuco L, Pagotto R, et al. Antitumor activity of histamine and clozapine in a mouse experimental model of human melanoma. J Dermatol Sci. (2013) 72:252–62. doi: 10.1016/j.jdermsci.2013.07.012
38. Matarrese P, Signore M, Ascione B, Fanelli G, Paggi MG, Abbruzzese C. Chlorpromazine overcomes temozolomide resistance in glioblastoma by inhibiting Cx43 and essential DNA repair pathways. J Transl Med. (2024) 22:667. doi: 10.1186/s12967-024-05501-3
39. Angelini A, Ciofani G, Conti P. Antipsychotics reverse p-glycoprotein-mediated doxorubicin resistance in human uterine sarcoma MES-SA/Dx5 cells: A novel approach to cancer chemotherapy. J Biol Regul Homeost Agents. (2015) 29.2:357–65.
40. Muench J, Hamer AM. Adverse effects of antipsychotic medications. Am Fam Phys. (2010) 81:617–22.
41. Chow RTS, Whiting D, Favril L, Ostinelli E, Cipriani A, Fazel S. An umbrella review of adverse effects associated with antipsychotic medications: the need for complementary study designs. Neurosci Biobehav Rev. (2023) 155:105454. doi: 10.1016/j.neubiorev.2023.105454
42. Achtyes E, Simmons A, Skabeev A, Levy N, Jiang Y, Marcy P, et al. Patient preferences concerning the efficacy and side-effect profile of schizophrenia medications: a survey of patients living with schizophrenia. BMC Psychiatry. (2018) 18:292. doi: 10.1186/s12888-018-1856-y
43. Townsend M, Pareja K, Buchanan-Hughes A, Worthington E, Pritchett D, Brubaker M, et al. Antipsychotic-related stigma and the impact on treatment choices: A systematic review and framework synthesis. Patient Prefer Adherence. (2022) 16:373–401. doi: 10.2147/PPA.S343211
44. Lianos GD, Alexiou GA, Rausei S, Galani V, Mitsis M, Kyritsis AP. Repurposing antipsychotic drugs for cancer treatment: current evidence and future perspectives. Expert Rev Anticancer Ther. (2022) 22:131–4. doi: 10.1080/14737140.2022.1987221
45. Sandhya L, Devi Sreenivasan N, Goenka L, Dubashi B, Kayal S, Solaiappan M, et al. Randomized double-blind placebo-controlled study of olanzapine for chemotherapy-related anorexia in patients with locally advanced or metastatic gastric, hepatopancreaticobiliary, and lung cancer. J Clin Oncol. (2023) 41:2617. doi: 10.1200/JCO.22.01997
Keywords: antipsychotics - treatment, cancer treatment, gastrointestinal cancer, glioblastoma, chemotherapy agents
Citation: Lampros M, Vlachos N, Lianos GD, Bali C and Alexiou GA (2025) Editorial: Drug repurposing for cancer treatment: current and future directions. Front. Oncol. 15:1550672. doi: 10.3389/fonc.2025.1550672
Received: 23 December 2024; Accepted: 21 January 2025;
Published: 11 February 2025.
Edited and Reviewed by:
Massimo Broggini, Mario Negri Institute for Pharmacological Research (IRCCS), ItalyCopyright © 2025 Lampros, Vlachos, Lianos, Bali and Alexiou. This is an open-access article distributed under the terms of the Creative Commons Attribution License (CC BY). The use, distribution or reproduction in other forums is permitted, provided the original author(s) and the copyright owner(s) are credited and that the original publication in this journal is cited, in accordance with accepted academic practice. No use, distribution or reproduction is permitted which does not comply with these terms.
*Correspondence: Georgios D. Lianos, Z2Vvcmdpb2xpYW5vc0B5YWhvby5ncg==
Disclaimer: All claims expressed in this article are solely those of the authors and do not necessarily represent those of their affiliated organizations, or those of the publisher, the editors and the reviewers. Any product that may be evaluated in this article or claim that may be made by its manufacturer is not guaranteed or endorsed by the publisher.
Research integrity at Frontiers
Learn more about the work of our research integrity team to safeguard the quality of each article we publish.