- 1Department of Radiation Medicine, Georgetown University Hospital, Washington, DC, United States
- 2Division of Neuro-Oncology, Columbia University Irving Medical Center, New York, NY, United States
- 3Department of Radiation Oncology, Perlmutter Cancer Center, New York University (NYU), Langone, New York, NY, United States
- 4Department of Neurosurgery and Brain Repair, University of South Florida, Tampa, FL, United States
- 5Department of Pathology and Neurology, Georgetown University Hospital, Washington, DC, United States
- 6Department of Endocrinology, MedStar Washington Hospital Center, Washington, DC, United States
- 7Department of Neurosurgery, MedStar Washington Hospital Center, Washington, DC, United States
- 8Department of Neurosurgery, Georgetown University Hospital, Washington, DC, United States
- 9Department of Radiation Oncology, University of South Florida, Tampa, FL, United States
- 10Division of Neurosurgery, Lehigh Valley Fleming Neuroscience Institute, Allentown, PA, United States
Objective: Nonfunctioning macroadenoma is a commonly diagnosed pituitary tumor. Resection is the favored treatment, with radiosurgery often utilized for residual or progressing disease. Long-term outcomes are established in the literature for single-fraction frame-based radiosurgery, but mature outcomes are lacking for fractionated frameless radiosurgery. We report our institution’s 5-year efficacy and toxicity results for unfavorable nonfunctioning pituitary macroadenoma patients treated with 5-fraction robotic radiosurgery.
Methods: Between 2010 and 2020, patients who completed 5-fraction robotic radiosurgery for the treatment of unfavorable nonfunctioning pituitary macroadenomas were included. A tumor was considered unfavorable if the gross tumor volume (GTV) was larger than 5 cc or if it closely approached a critical structure (optic apparatus, brainstem, or pituitary gland). Local control was calculated using the Kaplan–Meier method.
Results: Twenty predominantly female patients (60%), ages 21–77 (median: 53 years), were included in this study. All underwent primary resection at the time of diagnosis. The indication for radiosurgery was tumor progression (n = 14, 70%) or residual tumor after subtotal resection (n = 6, 30%). Eighty-five percent of patients treated with radiosurgery (n = 17) had cavernous sinus involvement. Median GTV was 3.4 cm3 (range: 0.3–20.8 cm3), and 40% of the tumors had suprasellar extension. A mean dose of 28.8 Gy (range: 25–30 Gy) was delivered to a median isodose line of 80% (range: 75%–89%). The median optic chiasm maximum point dose was 21.8 Gy (range: 12.0–25.0 Gy). Acute toxicity was minimal with eight patients (40%) developing short-lived headaches and one patient (5%) developing a brief ipsilateral sixth nerve palsy. There was no late radiation-induced neurologic or optic dysfunction identified in this cohort. At a median follow-up of 5 years, local control was 94%. There was one in-field failure pathologically confirmed following surgery for pituitary hemorrhage and two radiographically confirmed out-of-field failures in patients with larger tumors (>20 cc).
Conclusions: The treatment of unfavorable nonfunctioning pituitary macroadenoma with 5-fraction robotic radiosurgery provides excellent local control to date, with acceptable toxicity. However, tumors with GTVs greater than 20 cc may still require conventionally fractionated treatment with a margin to optimize local control.
Introduction
Pituitary adenomas represent 10%–20% of intracranial tumors and can be hormonally active or non-functioning adenomas (NFAs) (1). Most NFAs are macroadenomas (i.e., measure greater than 1 cm in size at diagnosis) and are diagnosed after developing tumor-related headaches, vision loss, and/or cranial nerve deficit. Some cases are diagnosed incidentally when cranial imaging is performed for other purposes (2).
Multiple treatment options exist for patients with newly diagnosed NFAs, including observation, surgery, or radiation therapy. Transsphenoidal resection is the treatment of choice for patients suitable for surgery. Complete resection is achieved in more than 50% of patients with a low rate of complications including visual field deficits, pituitary dysfunction, cerebrospinal fluid (CSF) rhinorrhea, meningitis, diabetes insipidus, and severe bleeding (3). Unfortunately, complete resection is difficult to accomplish in patients presenting with parasellar extension. Such tumors often require radiation therapy following surgery (3–5).
Radiation therapy is an alternative to resection for patients ill-suited for surgery, an adjuvant treatment for those who have undergone a subtotal resection, or salvage treatment for those who experience tumor recurrence or growth of residual tumor. Multiple radiotherapy modalities are available for the treatment of pituitary macroadenomas, including stereotactic radiosurgery (SRS), multifraction SRS, also known as hypofractionated stereotactic radiotherapy (HSRT), or conventionally fractionated radiation therapy. SRS is a radiation therapy technique that precisely delivers a high dose of radiation in a single fraction while HSRT delivers treatment typically in 2–5 fractions. SRS is often delivered via the frame-based cobalt-60 Gamma Knife (GK) or the image guided CyberKnife (CK) robotic radiosurgery system. HSRT may be delivered using GK, CK, or a modified conventional radiotherapy machine (linear accelerator, LINAC) (6).
SRS is the favored treatment for small pituitary adenomas (<2.5 cm or 5 cc). Multiple retrospective series have demonstrated favorable control rates, often >90% at 5 years and toxicity is low (7). Furthermore, if the pituitary gland can be excluded from the irradiated volume, the risk of radiation-induced hypopituitarism may be negligible with this approach.
However, for unfavorable tumors, i.e., larger tumors, or those in close proximity to critical structures, conventional fractionated radiotherapy uses the advantage of the variance in biologically effective dose to tumors and normal tissue, a difference especially amplified with fractionation, allowing protection of the optic chiasm, proximal optic nerves, pituitary gland, and brainstem when irradiated. This highly effective treatment, with greater than 90% local control at 5 years, has typically been delivered in 25–28 fractions to a total dose of 45–50.4 Gy using a conventional LINAC (8–10).
However, a traditional LINAC has intrafraction uncertainty that necessitates a planning target volume (PTV) expansion, which increases the treatment volume, delivering dose to a larger component of the optic pathway, the pituitary gland, and brain (9, 11). As an alternative, the robotic CK system is a frameless radiosurgical technology with automated intrafraction kV imaging used to assess and automatically adjust to any minimal cranial motion in real time. This technique delivers a conformal radiation dose with a steep dose drop-off like that of the GK and eliminates the need for a margin accounting for intrafraction uncertainty. Additionally, because of the precise intrafraction positional correction, patients do not require frame-based immobilization, allowing for enhanced patient comfort (12–14).
A recent large meta-analysis confirmed that with single-fraction SRS, approximately 8% of patients developed vision loss and approximately 20% of all patients developed hypopituitarism. The authors concluded that multifraction SRS delivered in 3 to 5 fractions is promising with high rates of local control and low rates of treatment-related toxicity. However, they acknowledged that mature outcomes are lacking for multisession radiosurgery and therefore do not yet recommend it for routine clinical practice (7). To our knowledge, only two studies thus far have specifically looked at HSRT for non-functioning pituitary adenomas (15, 16).
Of these studies, one study reported outcomes using a NovalisTX radiotherapy system and the other utilized CK. Khattab and colleagues, treating on a NovalisTX radiotherapy system, reported their outcomes with HSRT for large nonfunctioning pituitary adenomas with chiasm involvement showing over 92% local control in the cohort treated with HSRT and with no optic neuropathies and similar endocrinopathies to the single-fraction cohort (15). Iwata and colleagues reported their outcomes on a CK treating 100 patients with NFPAs with HSRT with 98% local control and very low toxicity, with only 1 patient having grade 2 visual symptoms at a 3-year follow-up (16). In this small retrospective study, we report our promising 5-year efficacy and toxicity results for unfavorable NFPA patients treated with 5-fraction robotic radiosurgery.
Materials and methods
Study design, setting, and participants
The Medstar Health Research Institute–Georgetown University Oncology institutional review board approved this retrospective analysis of an established departmental treatment approach. A multidisciplinary neuro-oncology team evaluated patients. Patients with unfavorable nonfunctioning pituitary macroadenomas who had undergone surgical resection followed by 5-fraction robotic radiosurgery from 2010 to 2020 were included in the present analysis. A tumor was considered unfavorable if the gross tumor volume (GTV) was greater than 5 cc or if the tumor closely approached a critical structure (e.g., optic apparatus, pituitary gland, or brainstem).
Treatment planning
Prior to treatment, a custom thermoplastic mask was fabricated. A fine-cut (1.25 mm) contrast-enhanced treatment planning CT scan was obtained in the supine treatment position for each patient using a GE LightSpeed RT16. T1-weighted, contrast-enhanced magnetic resonance imaging (MRI) with 1.25-mm slice thickness was fused with the planning CT scans for target volume delineation. Pituitary adenomas and organs at risk (i.e., optic nerve, optic chiasm, and brainstem) were contoured without expansion on all visualized image slices of the planning CT scan and/or fused MRI. The pituitary gland was contoured as an organ at risk when identified. A treatment plan was generated using the Accuray Inc. MultiPlan 5.2.1 non-isocentric inverse-planning algorithm.
Delivery of stereotactic fractionated radiotherapy
Each patient underwent fractionated CK radiosurgery (Accuray Inc.) (17). Treatment plans were composed of hundreds of pencil beams delivered using a single 10- to 35-mm-diameter collimator. Radiation was delivered in 5 fractions of 5 to 6 Gy prescribed to an isodose line that covered at least 95% of the GTV. Patients were treated in the supine position with a custom aquaplast mask for immobilization and reproducible patient setup. The SRS treatment was routinely delivered over five consecutive business days. Organ at risk Dmax constraints were defined as 25 Gy for the optic chiasm, 28 Gy for optic nerves, and 31 Gy for the brainstem. There was no dose constraint placed on the pituitary gland.
Follow-up studies
Patients were followed with serial physical examination, pituitary function tests, and MRI per routine institutional practice. Routine visual field testing was not completed. Local tumor recurrence was defined as progression of the treated tumor based on radiological review of serial follow-up MRI. All cases involving a question of tumor progression were discussed at a weekly interdisciplinary CNS tumor conference. Progression was confirmed pathologically in those cases requiring surgical intervention. Toxicities were scored according to the National Cancer Institute Common Terminology Criteria for Adverse Events, Version 5.0 (18). A patient was considered to have radiation-induced pituitary dysfunction if they developed a new hormone deficiency requiring medical treatment following radiosurgery. A patient was considered to have radiation-induced vision loss if they developed a new visual field deficit following radiosurgery confirmed by formal visual field testing. Patients were followed until death. Cause of death analysis was completed by the interdisciplinary CNS tumor conference. Autopsy was not completed to confirm cause of death.
Statistical analysis
Data were analyzed and graphs were prepared with the SPSS 23 statistical package (IBM Corporation, Armonk, NY). The follow-up duration was defined as the time from the date of completion of radiosurgery treatment to the last date of follow-up or the date of death. The treatment parameters assessed included treatment isodose line, tumor coverage, and maximum radiation dose to organs at risk. The conformity index (CI) is the ratio of the treatment volume to the target volume.
The primary endpoint was local control defined as stable disease or partial response as evaluated on serial MRI. These were calculated using the Kaplan–Meier method. The secondary endpoints were radiation-induced neurologic or optic dysfunction.
Results
Patient, tumor, and treatment characteristics
Twenty predominantly female patients (60%), ages 21–77 (median: 53 years), were included in this study (Table 1). All underwent primary resection. One patient had panhypopituitarism, and two patients had visual field deficits prior to radiosurgery. The indication for radiosurgery was tumor progression in 70% of patients. The remainder were treated following subtotal resection (30%). Eighty-five percent of patients treated with radiosurgery had been deemed unresectable due to cavernous sinus involvement. Pituitary gland contouring for radiosurgery planning was feasible in eight patients (40%). The median tumor volume was 3.4 cc (range: 0.3–20.8 cc). Thirty percent of the tumors were greater than 5.00 cc and 40% of the tumors were suprasellar (Table 2).
Stereotactic fractionated radiosurgery
A mean dose of 28.8 Gy (range: 25–30 Gy) was delivered to a median isodose line of 80% (range: 75%–89%). The median tumor volume (GTV) treated was 3.8 cc (range: 0.3–20.8 cc). The mean GTV target coverage was 99.88% (range: 97%–100%) and average conformality index was of 1.63. The median optic nerve and optic chiasm maximum point doses were 22.9 Gy (range: 10.8–27.9 Gy) and 21.8 Gy (range: 12.0–25.0 Gy). The median pituitary gland maximum point dose was 30.5 Gy (range: 23.8–35.1 Gy) and mean dose was 20.2 Gy (range: 14.6–29.5 Gy) (Table 3).
Tumor control and survival
The 5-year tumor control rate was 94% at a median follow-up of 5 years (Figure 1). There were three local failures (Table 4). One was an early in-field failure for a 1-cc tumor that received a dose of 30 Gy (Figure 2). It was pathologically confirmed following surgery for pituitary hemorrhage (Figure 3). The other two local failures were late radiographically confirmed out-of-field failures in patients with large tumors (>20 cc) that had received a relatively low dose of 25 Gy (Figure 4). There were two reported deaths in this study that were unrelated to the pituitary tumor or its treatment.
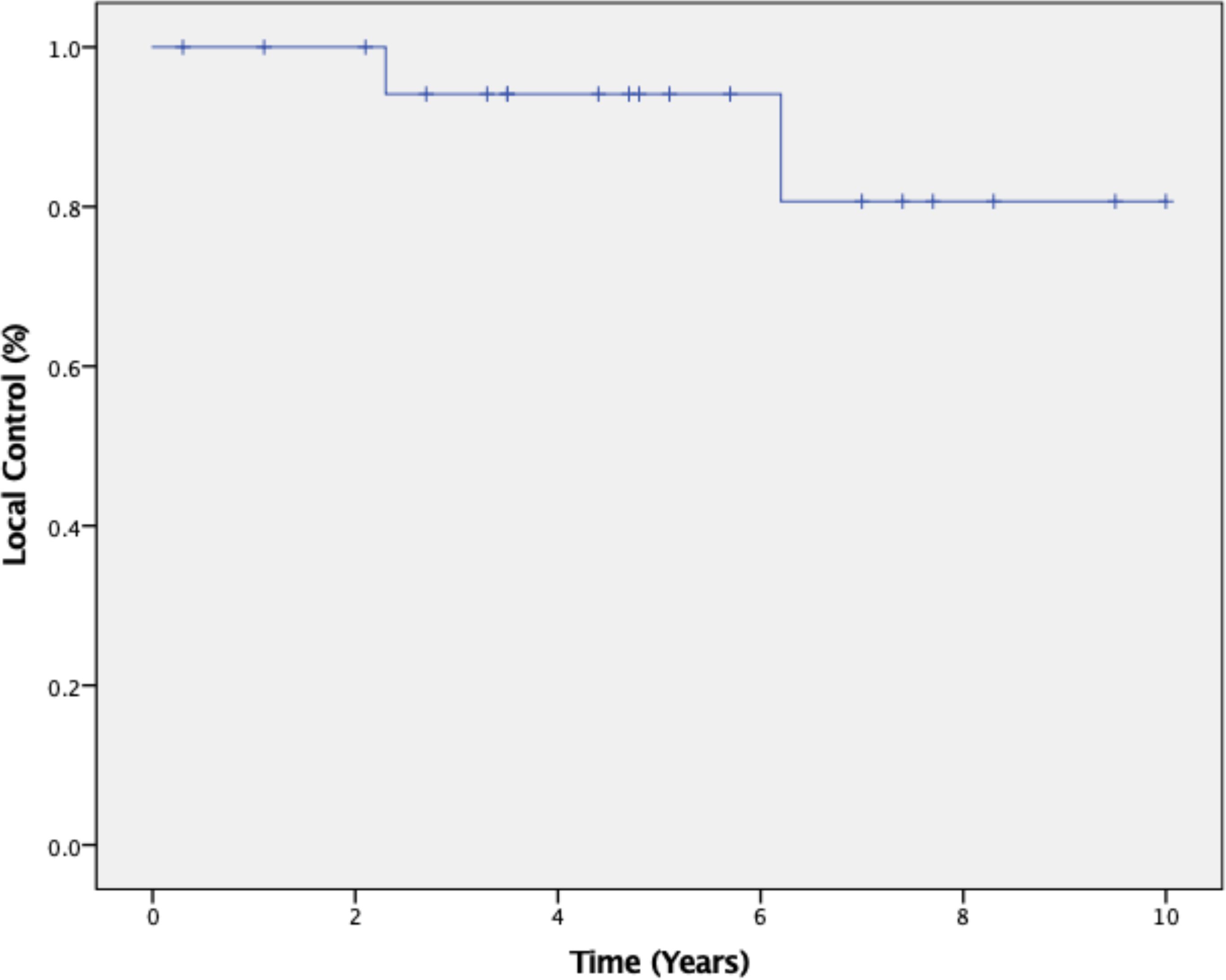
Figure 1. Kaplan–Meier 5-year local control was 94% at a median follow-up of 5 years from completion of SRS radiotherapy treatment.
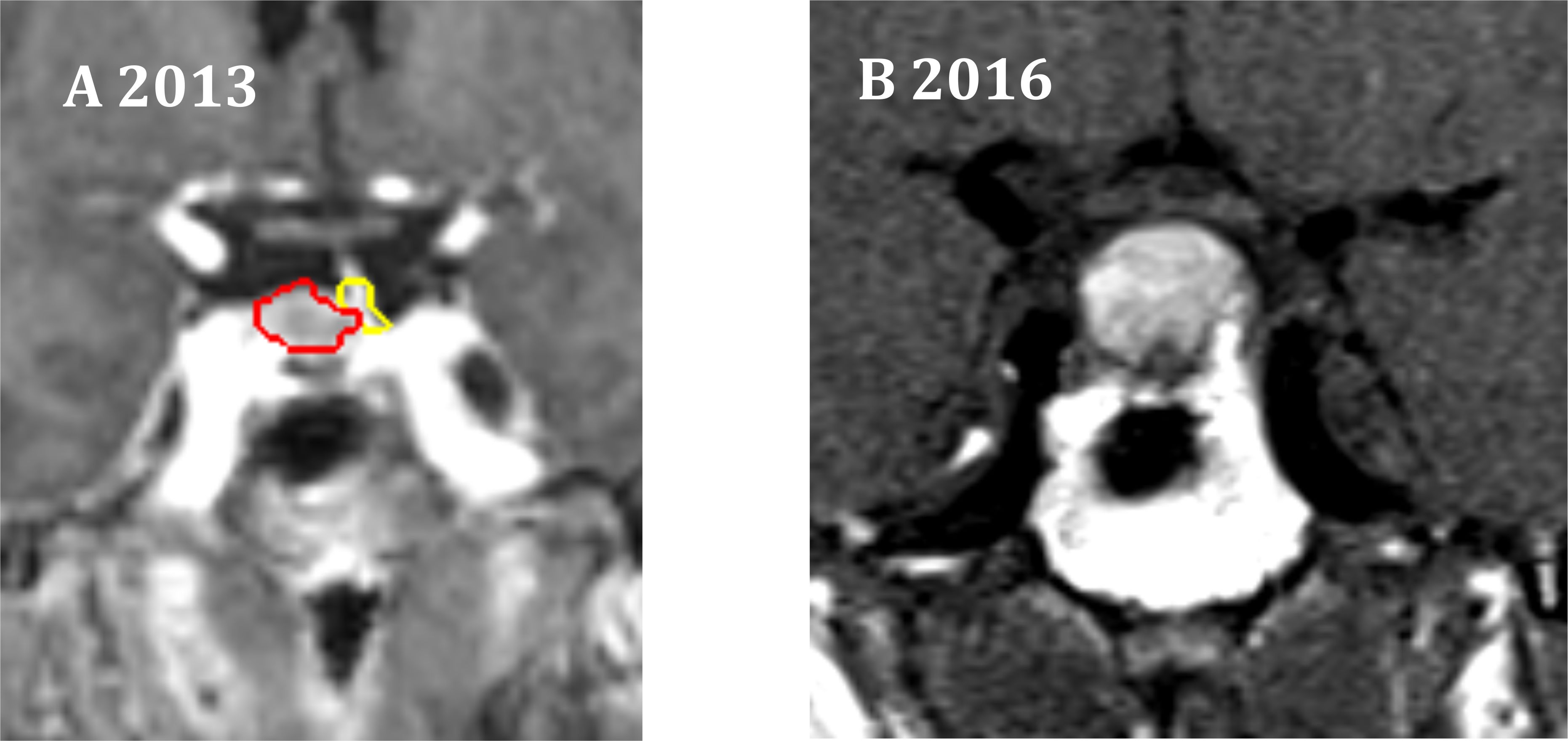
Figure 2. One in-field failure. (A) Coronal radiation treatment image demonstrating the treatment volumes for an NFA. Red represents the gross tumor volume (GTV), which is 1 cc in size. Yellow represents the pituitary gland. A prescription dose of 30 Gy was delivered to the 83% isodose line. (B) At 3 years, pituitary hemorrhage was observed.
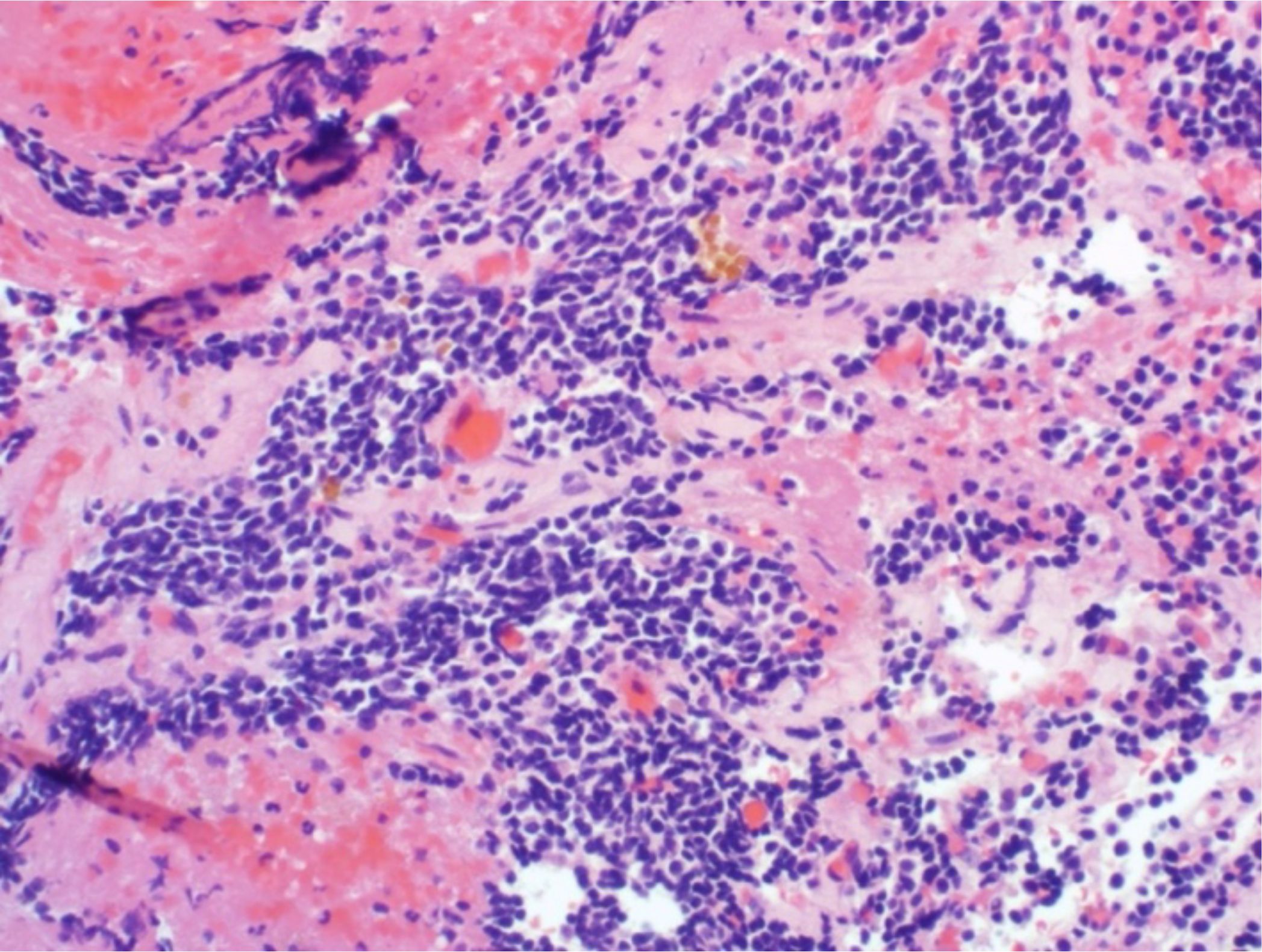
Figure 3. There was one early in-field failure pathologically confirmed following surgery for pituitary hemorrhage. Microscopic evaluation revealed residual pituitary adenoma with fibrosis and hemorrhage.
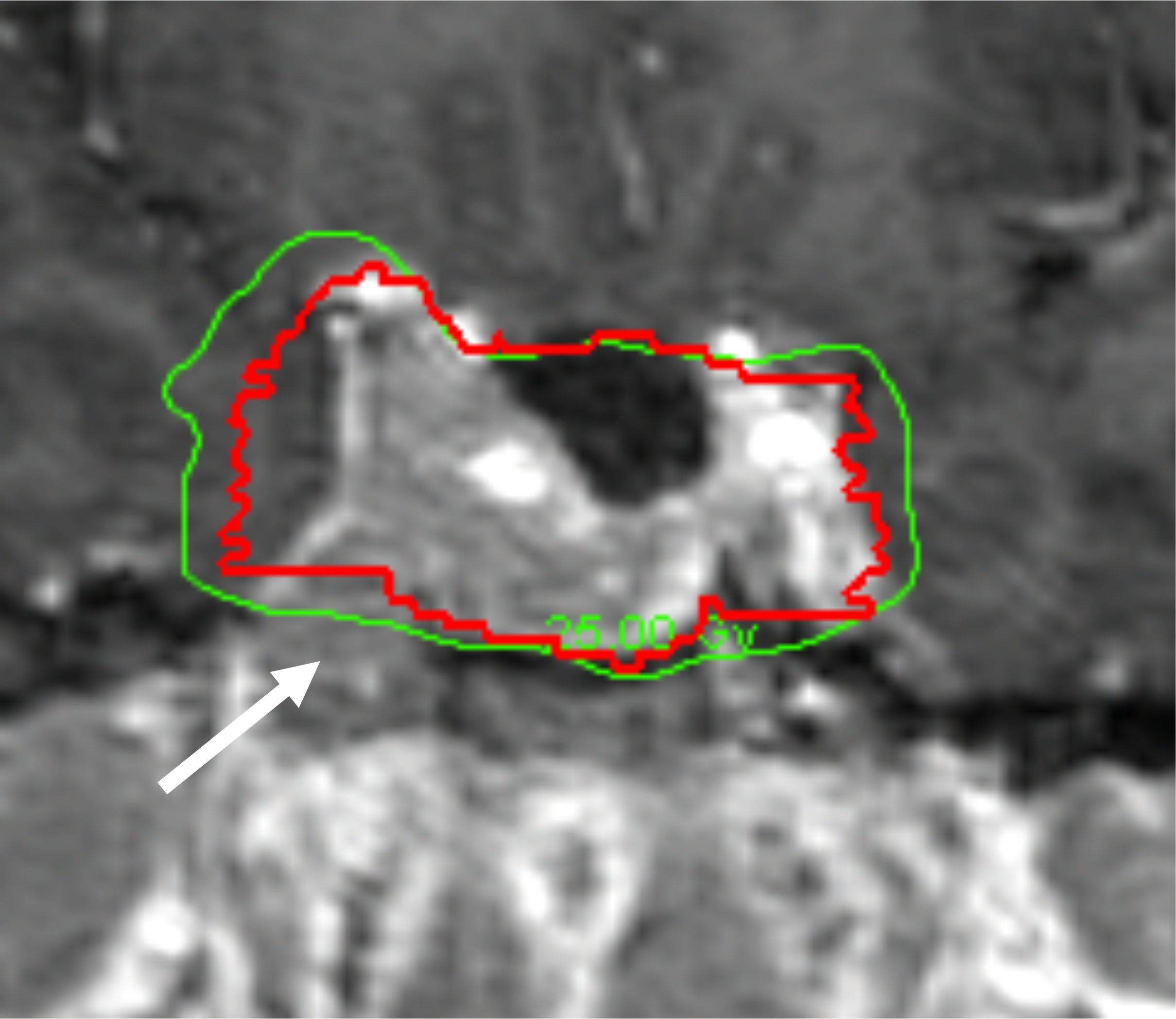
Figure 4. Example of a radiographically confirmed out-of-field failure in a patient with a large tumor (>20 cc) treated with a lower dose (25 Gy). Red: gross tumor volume (GTV) at the time of treatment. Green: isodose line that indicates the prescribed dose of 25 Gy. See tumor progression inferior to the prescribed dose of 25 Gy (white arrow).
Toxicity
Toxicity was acceptable with eight patients (40%) developing acute transient headaches and one patient (5%) developing an acute brief ipsilateral sixth nerve palsy (Table 4) Two patients developed chronic hypothyroidism, approximately 4 years after the completion of radiation treatment that required hormone replacement therapy (Table 4). The pituitary gland had been contoured in one of these patients and had received high maximum and mean pituitary doses of 31.28 and 26.35 Gy.
Discussion
Single-fraction SRS delivering doses of 14–16 Gy is a standard treatment option for small (<2.5 cm) residual, recurrent, or progressing NFAs, resulting in tumor control rates of 95% at 5 years and acceptable toxicity (i.e., ~20% chronic pituitary dysfunction) (7, 14). Our results suggest that SRS delivered in 5 fractions to a total dose of 25–30 Gy may represent an alternative treatment option for unfavorable nonfunctioning pituitary macroadenoma with similar 5-year local control rates.
Our reported toxicities were minimal. Multifraction SRS schemes allow for a high dose of conformal radiation to be delivered to a pituitary tumor with a steep dose fall-off. The pituitary gland was often in close proximity to the tumor and at times was not discernable. In this situation, limited fractionation allowed us to protect organs at risk including the pituitary gland. Hypopituitarism remains the most commonly reported late complication following pituitary tumor radiation treatment (7). In our routine radiosurgery practice, we have now adopted a 5-fraction treatment approach for all cases of pituitary macroadenomas to minimize radiation toxicity.
We observed one in-field failure for a 1-cc tumor that received a total dose of 30 Gy (Figure 2). It was pathologically confirmed following surgery for pituitary hemorrhage (Figure 3). Pituitary hemorrhage following single-fraction radiosurgery is not an uncommon phenomenon with a reported incidence of 7% (19–21). We do not believe that this toxicity was a result of fractionation. In fact, pituitary hemorrhage might also be decreased with fractionation. Only one patient experienced pituitary hemorrhage in our small series (i.e., 5% of patients). There were two radiographically confirmed out-of-field failures in patients with large tumors (>20 cc) treated with lower doses (25 Gy) (Table 3). See tumor progression inferior to the prescribed dose (Figure 4). Therefore, we believe tumors with GTVs greater than 20 cc may still require conventionally fractionated treatment with a margin to optimize local control (22).
Limitations of this study include the small patient population and its retrospective design. Because of its retrospective design, we recognize that we did not have baseline formal visual field testing or a comprehensive pituitary function evaluation, prior to and after treatment. Thus, our toxicity could be more significant than reported. Future prospective research should continue to investigate the optimal fractionation schedule for non-functioning pituitary macroadenoma.
Conclusion
Single-fraction radiosurgery is the favored treatment for small (<2.5 cm or 5 cc) residual, recurrent, or progressing NFAs. However, a recent large meta-analysis suggests that for tumors that are >2.5 cm or 5 cc, or located in close proximity to the optic apparatus, SRS delivered in 3 to 5 fractions may represent an alternative treatment to single-fraction SRS. However, the absence of mature outcome data has prevented this from becoming a routine approach (7). We present the first 5-year outcome data in a small series of patients that confirm that the treatment of unfavorable nonfunctioning pituitary macroadenoma with 5-fraction CK radiosurgery provides excellent local control, 94% at 5 years, with minimal acute toxicity and no late radiation-induced neurologic or optic deficits. However, our small series also suggests that tumors with GTVs greater than 20 cc may still require conventionally fractionated treatment with a margin to optimize local control. Prospective research is planned to confirm our preliminary findings.
Data availability statement
The raw data supporting the conclusions of this article will be made available by the authors, without undue reservation.
Ethics statement
The studies involving humans were approved by Georgetown-MedStar Institutional Review Board. The studies were conducted in accordance with the local legislation and institutional requirements.
Author contributions
AB: Conceptualization, Data curation, Formal analysis, Project administration, Resources, Validation, Visualization, Writing – original draft, Writing – review & editing. MM: Writing – original draft, Writing – review & editing. JL: Writing – original draft, Writing – review & editing. MJK: Writing – review & editing. SA: Writing – original draft, Writing – review & editing. SS: Data curation, Writing – original draft, Writing – review & editing. BH: Writing – original draft, Writing – review & editing. STS: Writing – original draft, Writing – review & editing. EA: Writing – original draft, Writing – review & editing. AA: Writing – original draft, Writing – review & editing. MHK: Writing – original draft, Writing – review & editing. SC: Writing – original draft, Writing – review & editing. BC: Writing – original draft, Writing – review & editing, Conceptualization, Investigation, Project administration, Resources, Supervision, Validation. WJ: Writing – original draft, Writing – review & editing, Conceptualization, Investigation, Project administration, Resources, Supervision, Validation.
Funding
The author(s) declare that no financial support was received for the research, authorship, and/or publication of this article.
Acknowledgments
We would like to thank Michael Carrasquilla, MD, for his valuable contributions to the manuscript.
Conflict of interest
The authors declare that the research was conducted in the absence of any commercial or financial relationships that could be construed as a potential conflict of interest.
Generative AI statement
The author(s) declare that no Generative AI was used in the creation of this manuscript.
Publisher’s note
All claims expressed in this article are solely those of the authors and do not necessarily represent those of their affiliated organizations, or those of the publisher, the editors and the reviewers. Any product that may be evaluated in this article, or claim that may be made by its manufacturer, is not guaranteed or endorsed by the publisher.
References
1. Ezzat S, Asa SL, Couldwell WT, Barr CE, Dodge WE, Vance ML, et al. The prevalence of pituitary adenomas: a systematic review. Cancer. (2004) 101:613–9. doi: 10.1002/cncr.20412
2. Drummond JB, Ribeiro-Oliveira A Jr., Soares BS. Non-Functioning Pituitary Adenomas. In: Feingold KR, Anawalt B, Blackman MR, et al, editors. Endotext. MDText.com, Inc (2000). Copyright © 2000-2024. Available at: https://www.ncbi.nlm.nih.gov/books/NBK534880/.
3. Mortini P, Losa M, Barzaghi R, Boari N, Giovanelli M. Results of transsphenoidal surgery in a large series of patients with pituitary adenoma. Neurosurgery. (2005) 56:1222–33. doi: 10.1227/01.neu.0000159647.64275.9d
4. Esposito D, Olsson DS, Ragnarsson O, Buchfelder M, Skoglund T, Johannsson G. Non-functioning pituitary adenomas: indications for pituitary surgery and post-surgical management. Pituitary. (2019) 22:422–34. doi: 10.1007/s11102-019-00960-0
5. Dekkers OM, Pereira AM, Romijn JA. Treatment and follow-up of clinically nonfunctioning pituitary macroadenomas. J Clin Endocrinol Metab. (2008) 93:3717–26. doi: 10.1210/jc.2008-0643
6. Minniti G, Osti MF, Niyazi M. Target delineation and optimal radiosurgical dose for pituitary tumors. Radiat Oncol. (2016) 11:135. doi: 10.1186/s13014-016-0710-y
7. Kotecha R, Sahgal A, Rubens M, Salles AD, Fariselli L, Pollock BE, et al. Stereotactic radiosurgery for non-functioning pituitary adenomas: meta-analysis and International Stereotactic Radiosurgery Society practice opinion. Neuro Oncol. (2020) 22:318–32. doi: 10.1093/neuonc/noz225
8. Purdy JA. Dose to normal tissues outside the radiation therapy patient’s treated volume: a review of different radiation therapy techniques. Health Phys. (2008) 95:666–76. doi: 10.1097/01.Hp.0000326342.47348.06
9. Milker-Zabel S, Debus J, Thilmann C, Schlegel W, Wannenmacher M. Fractionated stereotactically guided radiotherapy and radiosurgery in the treatment of functional and nonfunctional adenomas of the pituitary gland. Int J Radiat Oncol Biol Phys. (2001) 50:1279–86. doi: 10.1016/s0360-3016(01)01535-8
10. Kong DS, Lee JI, Lim DH, Kim KW, Shin HJ, Nam D-H, et al. The efficacy of fractionated radiotherapy and stereotactic radiosurgery for pituitary adenomas: long-term results of 125 consecutive patients treated in a single institution. Cancer. (2007) 110:854–60. doi: 10.1002/cncr.22860
11. Leber KA, Berglöff J, Pendl G. Dose-response tolerance of the visual pathways and cranial nerves of the cavernous sinus to stereotactic radiosurgery. J Neurosurg. (1998) 88:43–50. doi: 10.3171/jns.1998.88.1.0043
12. Chang SD, Main W, Martin DP, Gibbs IC, Heilbrun MP. An analysis of the accuracy of the CyberKnife: a robotic frameless stereotactic radiosurgical system. Neurosurgery. (2003) 52:140–6. doi: 10.1097/00006123-200301000-00018
13. Killory BD, Kresl JJ, Wait SD, Ponce FA, Porter R, White WL. Hypofractionated CyberKnife radiosurgery for perichiasmatic pituitary adenomas: early results. Neurosurgery. (2009) 64:A19–25. doi: 10.1227/01.Neu.0000341630.42160.18
14. Adler JR Jr., Gibbs IC, Puataweepong P, Chang SD. Visual field preservation after multisession cyberknife radiosurgery for perioptic lesions. Neurosurgery. (2006) 59:244–54. doi: 10.1227/01.Neu.0000223512.09115.3e
15. Khattab MH, Sherry AD, Xu MC, Kelly P, Anderson JL, Luo G, et al. Stereotactic radiosurgery and hypofractionated stereotactic radiotherapy for nonfunctioning pituitary adenoma. J Neurol Surg B Skull Base. (2021) 82:e51–8. doi: 10.1055/s-0040-1710518
16. Iwata H, Sato K, Tatewaki K, Yokota N, Inoue M, Baba Y, et al. Hypofractionated stereotactic radiotherapy with CyberKnife for nonfunctioning pituitary adenoma: high local control with low toxicity. Neuro Oncol. (2011) 13:916–22. doi: 10.1093/neuonc/nor055
17. Heilbrun MP. Cyberknife Radiosurgery: A Practical Guide. Sunnyvale, CA: CyberKnife Society (2003).
18. Institute NC. Common Terminology Criteria for Adverse Events (CTCAE). National Cancer Institute. Available online at: https://ctep.cancer.gov/protocoldevelopment/electronic_applications/docs/CTCAE_v5_Quick_Reference_5x7.pdf (Accessed October 29, 2024).
19. Fu J, Li Y, Wu L, Yang X, Quan T, Li X, et al. Pituitary hemorrhage in pituitary adenomas treated with gamma knife radiosurgery: incidence, risk factors and prognosis. J Cancer. (2021) 12:1365–72. doi: 10.7150/jca.52349
20. Weisberg LA. Pituitary apoplexy. Association of degenerative change in pituitary ademona with radiotherapy and detection by cerebral computed tomography. Am J Med. (1977) 63:109–15. doi: 10.1016/0002-9343(77)90122-x
21. Briet C, Salenave S, Bonneville JF, Laws ER, Chanson P. Pituitary apoplexy. Endocr Rev. (2015) 36:622–45. doi: 10.1210/er.2015-1042
Keywords: radiosurgery, fractionated stereotactic radiosurgery, nonfunctioning pituitary adenoma, local control, CyberKnife
Citation: Bhatnagar A, Marin MP, Lischalk JW, Koh MJ, Agazzi S, Suy S, Harris BT, Sharma ST, Aulisi E, Anaizi A, Khattab MH, Jean WC, Collins SP and Collins BT (2025) Fractionated robotic radiosurgery for unfavorable nonfunctioning pituitary macroadenoma: 5-year outcomes from a single institution protocol. Front. Oncol. 15:1519445. doi: 10.3389/fonc.2025.1519445
Received: 29 October 2024; Accepted: 07 January 2025;
Published: 04 February 2025.
Edited by:
Andrei Fodor, IRCCS San Raffaele Scientific Institute, ItalyReviewed by:
Marco Losa, Vita-Salute San Raffaele University, ItalySara Morlino, IRCCS Carlo Besta Neurological Institute Foundation, Italy
Copyright © 2025 Bhatnagar, Marin, Lischalk, Koh, Agazzi, Suy, Harris, Sharma, Aulisi, Anaizi, Khattab, Jean, Collins and Collins. This is an open-access article distributed under the terms of the Creative Commons Attribution License (CC BY). The use, distribution or reproduction in other forums is permitted, provided the original author(s) and the copyright owner(s) are credited and that the original publication in this journal is cited, in accordance with accepted academic practice. No use, distribution or reproduction is permitted which does not comply with these terms.
*Correspondence: Akrita Bhatnagar, YWtyaXRhLnJhZHNAZ21haWwuY29t; Min Ji Koh, bWluamlrb2gwMzIzQGdtYWlsLmNvbQ==
†These authors have contributed equally to this work and share senior authorship