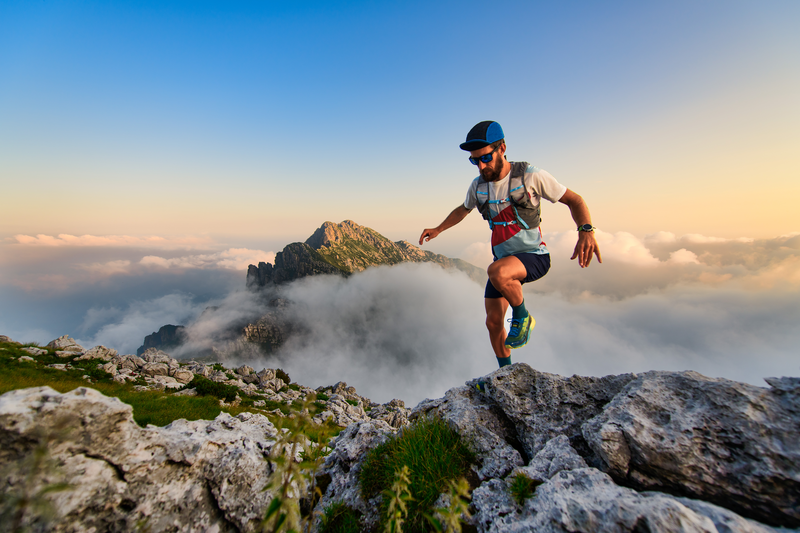
95% of researchers rate our articles as excellent or good
Learn more about the work of our research integrity team to safeguard the quality of each article we publish.
Find out more
ORIGINAL RESEARCH article
Front. Oncol. , 06 February 2025
Sec. Cancer Molecular Targets and Therapeutics
Volume 15 - 2025 | https://doi.org/10.3389/fonc.2025.1499650
Introduction: In patients with cancer, sarcopenia is an indicator of poor prognosis and is associated with an increased risk of chemotherapy-related adverse events. Skeletal muscle interacts with the immune system, and sarcopenia is associated with immune senescence. However, the association between sarcopenia and the response to immune checkpoint inhibitor (ICI) therapy remains unclear.
Methods: This retrospective study included patients with advanced or recurrent non-small cell lung cancer treated with nivolumab or pembrolizumab monotherapy. The association between the psoas muscle index (PMI) and both clinical response and immune-related adverse events (irAEs) was assessed using logistic regression. The PMI was calculated as the cross-sectional area of the psoas muscle divided by the square of the height based on computed tomography scans performed before the initial administration of ICI therapy.
Results: A total of 67 patients were included in the analysis. Logistic regression analysis showed that PMI was associated with the overall response (odds ratio [OR]: 1.52; 95% confidence interval [CI]: 1.04–2.22; p = 0.030) and the risk of severe irAEs (OR: 1.72; 95% CI: 1.05–2.80; p = 0.031).
Conclusion: These findings suggest that PMI is both an indicator of prognosis and a surrogate marker of immunocompetence in predicting the clinical response to ICI therapy.
Immune checkpoint inhibitors (ICIs) have demonstrated clinical benefits in patients with advanced cancer, including those with non-small cell lung cancer (NSCLC). Despite these advancements, the number of patients who benefit from ICIs remains limited, and no reliable predictive biomarkers have been identified. The mechanism of action of ICIs, which involves acting on the host immunity and stimulating the immune system against cancer cells, is fundamentally different from that of conventional cytotoxic chemotherapy. Hence, the effectiveness of ICIs is strongly dependent on patient immunocompetence, necessitating a comprehensive evaluation of patient immune status to predict responsiveness to ICI therapy.
Sarcopenia is defined as a loss of skeletal muscle mass and function. Its prevalence increases with age, and >40% of patients with advanced cancer are sarcopenic (1). In patients with cancer, sarcopenia is not only an independent factor associated with poor prognosis but is also associated with an increased incidence of adverse events caused by cytotoxic chemotherapy (2). Recent studies have demonstrated that skeletal muscle interacts with the immune system through various surface molecules and cytokines known as myokines, potentially linking sarcopenia to immune senescence (3, 4). Despite recent reports that sarcopenia is associated with a reduced clinical benefit of ICIs, it is unclear whether sarcopenia is merely a prognostic indicator or if it is associated with reduced effectiveness of ICI therapy through influencing the host immune system (5–11).
This study aimed to assess the relationship between skeletal muscle mass and the immune response to ICI therapy in patients with NSCLC. Skeletal muscle mass was evaluated using the psoas muscle index (PMI) measured on computed tomography (CT), a reliable indicator of skeletal muscle mass and sarcopenia status (12, 13).
This study had a retrospective cohort design. This study was designed as a retrospective cohort study. Patients diagnosed with advanced or recurrent non-small cell lung cancer (NSCLC) at our institution between 2016 and 2023 were included. The inclusion criteria consisted of patients with histologically confirmed advanced or recurrent NSCLC who received nivolumab or pembrolizumab monotherapy. Exclusion criteria included patients who did not have a pre-treatment abdominal CT scan within 4 weeks of initiating ICI therapy, as well as patients with poor-quality CT scans that were not suitable for PMI calculation, to minimize measurement errors. Baseline demographics, histology, prior chemotherapy, programmed cell death-ligand 1 (PD-L1) expression (evaluated using 28-8 or 22C3 pharmDx assays), serum albumin level, lymphocyte count, neutrophil-to-lymphocyte ratio, clinical outcomes, and adverse events were extracted from medical records. Many patients did not have an Eastern Cooperative Oncology Group Performance Status (PS) recorded; therefore, the PS was not included in the statistical analysis. Data collection was censored on May 1, 2023. This study was conducted in accordance with the principles of the Declaration of Helsinki. The Institutional Review Board, the Ethics Committee of Showa University School of Medicine, approved the study on March 3, 2023 (approval number: 22-058-A) and waived the requirement for informed consent because only medical records and images were reviewed.
The response to ICIs was evaluated using the Response Evaluation Criteria in Solid Tumors (version 1.1). Patients who showed complete response (CR) or partial response (PR) as the best overall response were defined as responders, and the others were defined as non-responders. Immune-related adverse events (irAEs) were diagnosed clinically, and the information was extracted from medical records. Severe irAEs were defined as any irAE that led to permanent discontinuation of ICIs. In the statistical analyses, age was considered as a potential confounding factor that influences both PMI and the outcomes. Using the “ten events per variable” rule and assuming an overall response rate (ORR) of 30% based on previous clinical trials, the minimal sample size was determined to be 60 in order to perform logistic regression analysis including PMI and age as variables (14–16). Sensitivity analyses were performed by substituting sex and albumin level for age as independent variables as they might influence both PMI and clinical outcomes. The Prognostic Nutritional Index (PNI) and Geriatric Nutritional Risk Index (GNRI) were also assessed as nutritional indices that may influence treatment outcomes. PNI was calculated using the formula: 10 × serum albumin level (g/dL) + 0.005 × total lymphocyte count (per mm³). GNRI was calculated using the formula: 14.89 × serum albumin level (g/dL) + 41.7 × current body weight/ideal body weight (17). These indices were analyzed in relation to the overall response rate (ORR), irAE incidence, progression-free survival (PFS), and overall survival (OS).
PMI was calculated as the cross-sectional area of the psoas muscle at the third lumbar vertebra divided by the square of the patient’s height. This method has been previously reported for evaluating sarcopenia in oncology patients (5–7). CT images used for PMI measurement were obtained before the initial administration of ICIs. The measurements were performed using SYNAPSE VINCENT software (Fujifilm Medical, Tokyo, Japan), which automatically identifies and measures the psoas muscle at the L3 level, minimizing the potential for operator error. For reference, we used established PMI cutoff values: 3.92 cm²/m² for female patients and 6.36 cm²/m² for male patients, as previously reported in healthy Asian populations without cancer (18). The study cohort was then categorized into “high-PMI” and “low-PMI” groups based on whether their PMI was above or below the median PMI, respectively, allowing for an unbiased division of the study population.
Two-group comparisons were performed using the chi-square test and Student’s t-test for categorical and continuous variables, respectively. Multivariable logistic regression was used to assess the association between the overall response and the incidence of irAEs. Progression-free survival (PFS) and overall survival (OS) were calculated using the Kaplan–Meier method, and group differences were analyzed using the log-rank test. Cox regression was used to analyze the association between PMI and survival. There were no missing data on the variables used in the statistical analyses. All statistical analyses were performed using JMP version 17.0.0 (SAS Institute Inc., Cary, NC, USA) and R software version 4.1.0 (R Foundation for Statistical Computing, Vienna, Austria). Two-tailed p values < 0.05 were considered statistically significant.
Sixty-seven patients (52 male and 15 female) were identified and confirmed eligible for inclusion in the analysis. The baseline characteristics of the low- and high-PMI groups are summarized in Table 1. The patients’ mean age was 65.4 years, and their mean PMI was 4.62 cm2/m2. Both PMI and body mass index were significantly higher in the high-PMI group than in the low-PMI group (p < 0.001 and p = 0.002, respectively). PNI and GNRI were not different between two groups. No differences were observed in histopathological characteristics or the prevalence of liver or brain metastases based on the PMI status. Pembrolizumab was administered to patients in the high-PMI group more frequently than to those in the low-PMI group (41% vs. 15%; p = 0.018).
The distribution of PMI in female and male patients is shown in Figure 1. The median PMI was 3.63 cm²/m² for female patients and 4.825 cm²/m² for male patients. Using the previously reported PMI cutoff values for healthy individuals without cancer (3.92 cm²/m² for female patients and 6.36 cm²/m² for male patients), 13 female patients (87%) and 43 male patients (83%) were classified as sarcopenic.
Figure 1. Density plot showing distribution of the psoas muscle index (PMI) according to sex. The dashed line represents the median PMI (females: 3.63 cm2/m2, and males: 4.825 cm2/m2). PMI, psoas muscle index.
Overall, 25 patients (37%) responded to ICI therapy. PMI was associated with the overall response in the unadjusted logistic regression analysis (odds ratio [OR]: 1.52; 95% confidence interval [CI]: 1.04–2.22; p = 0.030), and the analysis adjusted for age (OR: 1.49; 95% CI: 1.01–2.21; p = 0.046). Sensitivity analysis with adjustment for sex and albumin level resulted in similar findings (Supplementary Table 1). In addition, PMI was independently associated with a better response to PD-L1 expression (adjusted OR for PD-L1 expression: 1.54; 95% CI: 1.04–2.27; p = 0.030). Responders (CR or PR) had a significantly higher mean PMI than that of non-responders (5.13 cm2/m2 vs. 4.31 cm2/m2; p = 0.024) (Figure 2). The high-PMI group had an ORR of 44%, whereas the low-PMI group had an ORR of 30% (OR: 0.55; 95% CI: 0.20–1.50). The disease control rates (DCR) were 79% and 67% in the high- and low-PMI groups, respectively (OR: 0.52; 95% CI: 0.17–1.56). However, ORR was not correlated with PNI (unadjusted OR: 1.07; 95% CI: 0.98-1.17; p = 0.144) or GNRI (unadjusted OR: 1.04; 95% CI: 0.99-1.08; p = 0.101).
Figure 2. Clinical response to immune checkpoint inhibitors according to the psoas muscle index (PMI). (A) PMI in responders who showed complete response (CR) or partial response (PR) and in non-responders. (B) Best overall response (BOR) in the high- and low-PMI groups. BOR, best overall response; CR, complete response; PD, progressive disease; PMI, psoas muscle index; PR, partial response; SD, stable disease.
Nineteen patients (28%) experienced irAEs, and 10 (13%) experienced severe irAEs leading to ICI discontinuation. Logistic regression analysis showed a significant association between PMI and the incidence of severe irAEs (OR: 1.72; 95% CI: 1.05–2.80; p = 0.031), but did not show a significant association between PMI and all-grade irAEs (OR: 1.24; 95% CI: 0.853–1.80; p = 0.261). Multivariable logistic regression analysis also showed that PMI was independently associated with severe irAEs (Table 2). The mean PMI was significantly higher in patients with severe irAEs than in those without irAEs (5.56 cm2/m2 vs. 4.45 cm2/m2; p = 0.024) (Figure 3). The incidence of severe irAEs was 23.5% and 6% in the high- and low-PMI groups, respectively (OR: 0.21; 95% CI: 0.04–1.08). PNI was not associated with the incidence of severe irAEs (unadjusted OR: 1.12; 95% CI: 0.98-1.28; p = 0.098) nor was GNRI (unadjusted OR: 1.03; 95% CI: 0.98-1.09; p = 0.240).
Table 2. Multivariable logistic regression model of the association between PMI and the incidence of severe irAEs, adjusted for age.
Figure 3. Incidence of severe immune-related adverse events (irAEs) according to the psoas muscle index (PSI). PMI, psoas muscle index; irAE, immune-related adverse event.
The median PFS and OS for all patients were 252 and 858 days, respectively. Patients in the high-PMI group had a longer median PFS and OS than those in the low-PMI group, but these differences were not statistically significant (PFS: 386 days vs. 186 days, p = 0.173; OS: 878 days vs. 551 days; p = 0.059) (Supplementary Figure 1). Cox regression analysis did not show a significant association between PMI and survival (hazard ratio [HR] for PFS: 0.95; 95% CI: 0.80–1.14; p = 0.594, HR for OS: 0.95; 95% CI: 0.78−1.16; p = 0.616). In contrast, nutritional indices such as PNI and GNRI were correlated with both PFS and OS. Higher PNI values were associated with longer PFS (HR: 0.95; 95% CI: 0.91-1.00; p = 0.030) and OS (HR: 0.93; 95% CI: 0.88-0.98; p = 0.008). Similarly, higher GNRI values showed a association with longer PFS (HR: 0.97; 95% CI: 0.95-1.00; p = 0.051) and OS (HR: 0.96; 95% CI: 0.93-0.99; p = 0.015). Multivariable analysis with adjustment for age resulted in similar findings (Supplementary Table 2).
Further analyses were performed to examine the association between the presence of irAEs and clinical outcomes. Multivariable logistic regression analysis showed that ORR in patients with irAEs was higher than in those without (63% vs. 27%; OR adjusted for age: 5.14; 95% CI: 1.61–16.4; p = 0.006). The log-rank test revealed that the presence of irAEs was strongly associated with both PFS (median PFS: 625 days with irAEs and 146 days without irAEs; p = 0.011) and OS (median OS: 1,030 days with irAEs and 523 days without irAEs; p = 0.031) (Supplementary Figure 2). Cox regression analysis also revealed that the presence of irAEs was independently associated with both PFS and OS (Supplementary Table 3).
This study demonstrated a significant association between the ORR to ICIs and PMI. Notably, patients with a higher PMI had a higher incidence of irAEs, which are thought to be caused by ICI-induced immune cells. These findings indicate that PMI can serve not only as a prognostic indicator in patients with cancer but also as a predictor of their response to ICI therapy.
First, PMI was correlated with the ORR to ICIs, with responders having a significantly higher mean PMI than non-responders. Grouping patients based on the cutoff value of the median PMI showed higher ORR and DCR in the high-PMI group than in the low-PMI group. Furthermore, the mean PFS and OS in the high PMI group were double those in the low-PMI group, although these differences were not statistically significant. The lack of statistical significance is attributable to the small sample size. Recently, several parameters have been evaluated as biomarkers for predicting the response to ICI therapy. Of these, skeletal muscle mass is correlated with survival in patients with advanced NSCLC treated with nivolumab or a combination of ICIs and cytotoxic chemotherapy (5, 6). Additionally, PMI is correlated with the response to PD-1 inhibitors in patients with NSCLC (7). Similar associations have also been reported in patients receiving ICIs for gastric cancer and renal cell carcinoma (8–11). Two independent meta-analyses have shown that sarcopenia has a negative effect on the overall response and survival in patients treated with ICIs for various types of cancer, consistent with the results of this study.
Second, this study revealed a strong association between the PMI and the incidence of irAEs. This contrasts with previous reports on conventional cytotoxic chemotherapy, in which patients with sarcopenia tend to have more adverse events and higher discontinuation rates (1, 19–22). The difference in the effect of PMI on response to ICIs and cytotoxic chemotherapy can be attributed to fundamental differences in the mechanisms of these adverse events. Biologically, irAEs are inflammatory toxicities induced by overactivated T cells reinvigorated by ICIs (23). In a mouse model of ICI-induced myocarditis, clonally expanded cytotoxic CD8+ T cells infiltrated the myocardium and were necessary for the development of myocarditis (24). Similar findings have been reported for ICI-induced colitis. Single-cell RNA sequencing of colon biopsies from patients with ICI-induced colitis revealed an expansion of cytotoxic CD8+ T cells (25).
These findings suggest that the antitumor effects of ICIs and irAEs have a common underlying mechanism. In other words, the incidence of irAEs is a sign of sufficient activation of the host immune system by ICIs, and patients who experience irAEs can be deemed to be “responders” to ICIs. The presence of irAEs was strongly associated with both the clinical response of ICIs and survival in the present study. Several studies have had similar findings in NSCLC and in other types of cancer (26–29). Furthermore, severity and the number of irAEs were also associated with better survival in patients with NSCLC (30–32). This suggests that irAEs may not only reflect immune system activation but also act as an indicator of the degree of immune engagement, which could contribute to improved long-term outcomes.
The associations between PMI and both clinical response and irAEs suggest that PMI is not only a prognostic indicator but can also be used as a surrogate marker for patient immunocompetence, which predicts the clinical response to ICIs. Skeletal muscle cells secrete various cytokines called myokines, which communicate with other organs, such as the liver, pancreas, cardiovascular system, brain, and bones (33). Recently, skeletal muscle has been shown to regulate both innate and adaptive immune responses via surface molecules and myokines, and loss of skeletal muscle mass and sarcopenia may cause immune senescence (3, 4). Preclinical research has shown that skeletal muscle also interacts with the gut microbiota, which affect the tumor microenvironment via their metabolites (34–36). A clinical study of patients with lung cancer has shown that sarcopenia is associated with changes in the gut microbiota and their metabolites (37). These connections between skeletal muscle and the immune system may explain the greater potential utility of PMI for predicting the effectiveness of ICIs than for predicting response to conventional cytotoxic chemotherapy. In our study, PMI was independently associated with a better response to PD-L1 expression. Our findings suggest that PMI complements and enhances the prediction of clinical outcomes alongside PD-L1 expression, an established biomarker. While PD-L1 expression primarily focuses on tumor characteristics, this result further supports the idea that PMI reflects the patient’s overall immune status, which influences their response to ICIs. However, a recent meta-analysis, which found that sarcopenia had a negative effect on the clinical response to ICIs, was inconclusive regarding the relationship between sarcopenia and irAEs (38, 39). Considering that this lack of clarity is possibly due to the limited number of studies on irAEs, additional large studies are necessary to reach a conclusion.
Although PMI was significantly associated with both clinical response (ORR) and the incidence of severe irAEs, nutritional indices such as PNI and GNRI were not correlated with these factors but were linked to longer PFS and OS. This suggests that PMI is more closely related to immune response and may serve as a marker for immunocompetence, influencing both the clinical response to ICIs and the occurrence of irAEs. In contrast, PNI and GNRI appear to function more as prognostic scores, reflecting long-term survival outcomes rather than immediate treatment response. This distinction underscores the multifaceted relationship between muscle mass, nutrition, and immune status in patients treated with immune checkpoint inhibitors. Further research is needed to elucidate the mechanisms by which these indices influence immunotherapy outcomes.
Importantly, one strength of this study is that it focuses exclusively on patients treated with ICI monotherapy. By excluding combination therapies, we could better isolate the impact of ICI therapy alone, without the confounding effects of chemotherapy, which can also influence both objective response rates (ORR) and survival outcomes. However, this study has several limitations. First, it was conducted at a single institution with a relatively small sample size, which may limit the statistical power and generalizability of our findings. Additionally, the homogeneity of our cohort, composed exclusively of Asian patients, may restrict the applicability of our results to more diverse populations. The study also included relatively few female patients, which may limit the generalizability of the findings with regard to sex-based differences in sarcopenia or immune response. Furthermore, the use of previously reported cut-off values for PMI, which were established in healthy individuals without cancer, may not fully reflect the optimal thresholds for sarcopenia in cancer patients. These reference values (3.92 cm²/m² for female patients and 6.36 cm²/m² for male patients) led to a high proportion of patients in our cohort being classified as sarcopenic (87% of female patients and 83% of male patients), potentially limiting the generalizability of these thresholds to cancer populations. The optimal cut-off points for sarcopenia, particularly in the context of cancer treatment, remain an area of ongoing research. Moreover, as this was a retrospective study, we were unable to perform immune profiling, such as evaluating effector and regulatory T cells, myeloid-derived suppressor cells, natural killer cells, or monocyte activity, which may have provided deeper insights into the relationship between skeletal muscle mass and immune competence. Future prospective studies incorporating detailed immune profiling will be essential to elucidate the mechanisms underlying the observed associations between PMI and clinical outcomes in patients treated with ICIs. Additionally, the question of whether interventions to improve the sarcopenic status of patients with cancer can improve ICI response and overcome resistance to ICIs remains unanswered. Several interventions such as nutritional support, physical exercise, and anamorelin have been investigated, but none of these interventions have demonstrated clear clinical benefits on the survival of patients with cancer (1, 40). An ongoing trial including patients with melanoma receiving ICI therapy, with the intervention group undergoing diet modulation and regular exercise, is assessing the effect of exercise on response to ICI therapy (NCT04866810). Finally, the retrospective nature of this study means that we cannot infer causality, and the design inherently introduces selection bias, as well as the potential for residual confounders not accounted for in the analysis. Therefore, prospective clinical trials with well-defined interventions to increase patients’ PMI are required to provide conclusive evidence on the relationship between PMI and response to ICI therapy.
In conclusion, this study demonstrated a significant association between PMI and response to ICI therapy in patients with NSCLC. The incidence of irAEs, which are thought to be a sign of immune cell reinvigoration by ICIs, was also associated with PMI. These findings suggest that PMI may serve as a prognostic indicator and a biomarker of immunocompetence that can predict response to ICI therapy.
The raw data supporting the conclusions of this article will be made available by the authors, without undue reservation.
The studies involving humans were approved by the Institutional Review Board, the Ethics Committee of Showa University School of Medicine. The studies were conducted in accordance with the local legislation and institutional requirements. The ethics committee/institutional review board waived the requirement of written informed consent for participation from the participants or the participants’ legal guardians/next of kin because only medical records and images were reviewed.
ToT: Formal analysis, Investigation, Visualization, Writing – original draft. KH: Data curation, Methodology, Writing – review & editing. EM: Data curation, Writing – review & editing. RS: Data curation, Writing – review & editing. NI: Data curation, Writing – review & editing. TI: Data curation, Writing – review & editing. YH: Data curation, Writing – review & editing. RO: Data curation, Writing – review & editing. MS: Data curation, Writing – review & editing. HA: Data curation, Writing – review & editing. YK: Data curation, Writing – review & editing. AH: Data curation, Writing – review & editing. SW: Data curation, Writing – review & editing. KY: Data curation, Writing – review & editing. MT: Supervision, Writing – review & editing. YK: Supervision, Writing – review & editing. TaT: Conceptualization, Supervision, Writing – review & editing.
The author(s) declare that no financial support was received for the research, authorship, and/or publication of this article.
We thank Professor Eisuke Inoue, Showa University Research Administration Center, Showa University, for providing advice on the statistical analysis.
The authors declare that the research was conducted in the absence of any commercial or financial relationships that could be construed as a potential conflict of interest.
All claims expressed in this article are solely those of the authors and do not necessarily represent those of their affiliated organizations, or those of the publisher, the editors and the reviewers. Any product that may be evaluated in this article, or claim that may be made by its manufacturer, is not guaranteed or endorsed by the publisher.
The Supplementary Material for this article can be found online at: https://www.frontiersin.org/articles/10.3389/fonc.2025.1499650/full#supplementary-material
1. Bozzetti F. Forcing the vicious circle: Sarcopenia increases toxicity, decreases response to chemotherapy and worsens with chemotherapy. Ann Oncol. (2017) 28:2107–18. doi: 10.1093/annonc/mdx271
2. Shachar SS, Williams GR, Muss HB, Nishijima TF. Prognostic value of sarcopenia in adults with solid tumours: A meta-analysis and systematic review. Eur J Cancer. (2016) 57:58–67. doi: 10.1016/j.ejca.2015.12.030
3. Nelke C, Dziewas R, Minnerup J, Meuth SG, Ruck T. Skeletal muscle as potential central link between sarcopenia and immune senescence. EBiomedicine. (2019) 49:381–8. doi: 10.1016/j.ebiom.2019.10.034
4. Afzali AM, Müntefering T, Wiendl H, Meuth SG, Ruck T. Skeletal muscle cells actively shape (auto)immune responses. Autoimmun Rev. (2018) 17:518–29. doi: 10.1016/j.autrev.2017.12.005
5. Tsukagoshi M, Yokobori T, Yajima T, Maeno T, Shimizu K, Mogi A, et al. Skeletal muscle mass predicts the outcome of nivolumab treatment for non-small cell lung cancer. Med (Baltimore). (2020) 99:e19059. doi: 10.1097/MD.0000000000019059
6. Bolte FJ, McTavish S, Wakefield N, Shantzer L, Hubbard C, Krishnaraj A, et al. Association of sarcopenia with survival in advanced NSCLC patients receiving concurrent immunotherapy and chemotherapy. Front Oncol. (2022) 12:986236. doi: 10.3389/fonc.2022.986236
7. Shiroyama T, Nagatomo I, Koyama S, Hirata H, Nishida S, Miyake K, et al. Impact of sarcopenia in patients with advanced non–small cell lung cancer treated with PD-1 inhibitors: A preliminary retrospective study. Sci Rep. (2019) 9:2447. doi: 10.1038/s41598-019-39120-6
8. Kim YY, Lee J, Jeong WK, Kim ST, Kim JH, Hong JY, et al. Prognostic significance of sarcopenia in microsatellite-stable gastric cancer patients treated with programmed death-1 inhibitors. Gastric Cancer. (2021) 24:457–66. doi: 10.1007/s10120-020-01124-x
9. Kano M, Hihara J, Tokumoto N, Kohashi T, Hara T, Shimbara K, et al. Association between skeletal muscle loss and the response to nivolumab immunotherapy in advanced gastric cancer patients. Int J Clin Oncol. (2021) 26:523–31. doi: 10.1007/s10147-020-01833-4
10. Herrmann T, Mione C, Montoriol PF, Molnar I, Ginzac A, Durando X, et al. Body mass index, sarcopenia, and their variations in predicting outcomes for patients treated with nivolumab for metastatic renal cell carcinoma. Oncology. (2022) 100:114–23. doi: 10.1159/000520833
11. Ueki H, Hara T, Okamura Y, Bando Y, Terakawa T, Furukawa J, et al. Association between sarcopenia based on psoas muscle index and the response to nivolumab in metastatic renal cell carcinoma: A retrospective study. Investig Clin Urol. (2022) 63:415–24. doi: 10.4111/icu.20220028
12. Miller BS, Ignatoski KM, Daignault S, Lindland C, Gauger PG, Doherty GM, et al. A quantitative tool to assess degree of sarcopenia objectively in patients with hypercortisolism. Surgery. (2011) 150:1178–85. doi: 10.1016/j.surg.2011.09.020
13. Yajima T, Arao M, Yajima K. Psoas muscle index and psoas muscle density as predictors of mortality in patients undergoing hemodialysis. Sci Rep. (2022) 12:10496. doi: 10.1038/s41598-022-14927-y
14. Carbone DP, Reck M, Paz-Ares L, Creelan B, Horn L, Steins M, et al. First-line nivolumab in Stage IV or recurrent non–small-cell lung cancer. N Engl J Med. (2017) 376:2415–26. doi: 10.1056/NEJMoa1613493
15. Herbst RS, Baas P, Kim DW, Felip E, Pérez-Gracia JL, Han JY, et al. Pembrolizumab versus docetaxel for previously treated, PD-L1-positive, advanced non-small-cell lung cancer (KEYNOTE-010): A randomised controlled trial. Lancet. (2016) 387:1540–50. doi: 10.1016/S0140-6736(15)01281-7
16. Reck M, Rodríguez-Abreu D, Robinson AG, Hui R, Csőszi T, Fülöp A, et al. Pembrolizumab versus chemotherapy for PD-L1–positive non–small-cell lung cancer. N Engl J Med. (2016) 375:1823–33. doi: 10.1056/NEJMoa1606774
17. Kollu K, Akbudak Yerdelen E, Duran S, Kabatas B, Karakas F, Kizilarslanoglu MC. Comparison of nutritional risk indices (PNI, GNRI, mNUTRIC) and HALP score in predicting adverse clinical outcomes in older patients staying in an intensive care unit. Med (Baltimore). (2024) 103:e38672. doi: 10.1097/MD.0000000000038672
18. Hamaguchi Y, Kaido T, Okumura S, Kobayashi A, Hammad A, Tamai Y, et al. Proposal for new diagnostic criteria for low skeletal muscle mass based on computed tomography imaging in Asian adults. Nutrition. (2016) 32:1200–5. doi: 10.1016/j.nut.2016.04.003
19. Tan BHL, Brammer K, Randhawa N, Welch NT, Parsons SL, James EJ, et al. Sarcopenia is associated with toxicity in patients undergoing neo-adjuvant chemotherapy for oesophago-gastric cancer. Eur J Surg Oncol. (2015) 41:333–8. doi: 10.1016/j.ejso.2014.11.040
20. Prado CMM, Baracos VE, McCargar LJ, Reiman T, Mourtzakis M, Tonkin K, et al. Sarcopenia as a determinant of chemotherapy toxicity and time to tumor progression in metastatic breast cancer patients receiving capecitabine treatment. Clin Cancer Res. (2009) 15:2920–6. doi: 10.1158/1078-0432.CCR-08-2242
21. Prado CMM, Baracos VE, McCargar LJ, Mourtzakis M, Mulder KE, Reiman T, et al. Body composition as an independent determinant of 5-fluorouracil-based chemotherapy toxicity. Clin Cancer Res. (2007) 13:3264–8. doi: 10.1158/1078-0432.CCR-06-3067
22. Ali R, Baracos VE, Sawyer MB, Bianchi L, Roberts S, Assenat E, et al. Lean body mass as an independent determinant of dose-limiting toxicity and neuropathy in patients with colon cancer treated with FOLFOX regimens. Cancer Med. (2016) 5:607–16. doi: 10.1002/cam4.621
23. Wang SJ, Dougan SK, Dougan M. Immune mechanisms of toxicity from checkpoint inhibitors. Trends Cancer. (2023) 9:543–53. doi: 10.1016/j.trecan.2023.04.002
24. Axelrod ML, Meijers WC, Screever EM, Qin J, Carroll MG, Sun X, et al. T cells specific for α-myosin drive immunotherapy-related myocarditis. Nature. (2022) 611:818–26. doi: 10.1038/s41586-022-05432-3
25. Luoma AM, Suo S, Williams HL, Sharova T, Sullivan K, Manos M, et al. Molecular pathways of colon inflammation induced by cancer immunotherapy. Cell. (2020) 182:655–671.e22. doi: 10.1016/j.cell.2020.06.001
26. Lin L, Liu Y, Chen C, Wei A, Li W. Association between immune-related adverse events and immunotherapy efficacy in non-small-cell lung cancer: A meta-analysis. Front Pharmacol. (2023) 14:1190001. doi: 10.3389/fphar.2023.1190001
27. Socinski MA, Jotte RM, Cappuzzo F, Nishio M, Mok TSK, Reck M, et al. Association of immune-related adverse events with efficacy of atezolizumab in patients with non-small cell lung cancer: Pooled analyses of the Phase 3 IMpower130, IMpower132, and IMpower150 randomized clinical trials. JAMA Oncol. (2023) 9:527–35. doi: 10.1001/jamaoncol.2022.7711
28. Amoroso V, Gallo F, Alberti A, Paloschi D, Ferrari Bravo W, Esposito A, et al. Immune-related adverse events as potential surrogates of immune checkpoint inhibitors’ efficacy: A systematic review and meta-analysis of randomized studies. ESMO Open. (2023) 8:100787. doi: 10.1016/j.esmoop.2023.100787
29. Das S, Johnson DB. Immune-related adverse events and anti-tumor efficacy of immune checkpoint inhibitors. J Immunother Cancer. (2019) 7:306. doi: 10.1186/s40425-019-0805-8
30. Guezour N, Soussi G, Brosseau S, Abbar B, Naltet C, Vauchier C, et al. Grade 3-4 immune-related adverse events induced by immune checkpoint inhibitors in non-small-cell lung cancer (NSCLC) patients are correlated with better outcome: A real-life observational study. Cancers (Basel). (2022) 14:3878. doi: 10.3390/cancers14163878
31. Olsson Ladjevardi C, Koliadi A, Rydén V, El-Naggar AI, Digkas E, Valachis A, et al. Multiple immune-related adverse events secondary to checkpoint inhibitor therapy in patients with advanced cancer: association with treatment effectiveness. Front Oncol. (2024) 14:1399171. doi: 10.3389/fonc.2024.1399171
32. Shankar B, Zhang J, Naqash AR, Forde PM, Feliciano JL, Marrone KA, et al. Multisystem immune-related adverse events associated with immune checkpoint inhibitors for treatment of non-small cell lung cancer. JAMA Oncol. (2020) 6:1952–6. doi: 10.1001/jamaoncol.2020.5012
33. Giudice J, Taylor JM. Muscle as a paracrine and endocrine organ. Curr Opin Pharmacol. (2017) 34:49–55. doi: 10.1016/j.coph.2017.05.005
34. Lahiri S, Kim H, Garcia-Perez I, Reza MM, Martin KA, Kundu P, et al. The gut microbiota influences skeletal muscle mass and function in mice. Sci Transl Med. (2019) 11:eean5662. doi: 10.1126/scitranslmed.aan5662
35. Kim HJ, Kim YJ, Kim YJ, Baek JH, Kim HS, Kim IY, et al. Microbiota influences host exercise capacity via modulation of skeletal muscle glucose metabolism in mice. Exp Mol Med. (2023) 55:1820–30. doi: 10.1038/s12276-023-01063-4
36. Mancin L, Wu GD, Paoli A. Gut microbiota–bile acid–skeletal muscle axis. Trends Microbiol. (2023) 31:254–69. doi: 10.1016/j.tim.2022.10.003
37. Ni Y, Lohinai Z, Heshiki Y, Dome B, Moldvay J, Dulka E, et al. Distinct composition and metabolic functions of human gut microbiota are associated with cachexia in lung cancer patients. ISME J. (2021) 15:3207–20. doi: 10.1038/s41396-021-00998-8
38. Takenaka Y, Oya R, Takemoto N, Inohara H. Predictive impact of sarcopenia in solid cancers treated with immune checkpoint inhibitors: A meta-analysis. J Cachexia Sarcopenia Muscle. (2021) 12:1122–35. doi: 10.1002/jcsm.12755
39. Li S, Wang T, Tong G, Li X, You D, Cong M. Prognostic impact of sarcopenia on clinical outcomes in Malignancies treated with immune checkpoint inhibitors: A systematic review and meta-analysis. Front Oncol. (2021) 11:726257. doi: 10.3389/fonc.2021.726257
Keywords: immune checkpoint inhibitors, immunotherapy, lung neoplasms, sarcopenia, retrospective study
Citation: Tsurui T, Hamada K, Mura E, Suzuki R, Iriguchi N, Ishiguro T, Hirasawa Y, Ohkuma R, Shimokawa M, Ariizumi H, Kubota Y, Horiike A, Wada S, Yoshimura K, Tsuji M, Kiuchi Y and Tsunoda T (2025) Evaluation of patient immunocompetence for immune checkpoint inhibitor therapy using the psoas muscle index: a retrospective cohort study. Front. Oncol. 15:1499650. doi: 10.3389/fonc.2025.1499650
Received: 23 September 2024; Accepted: 16 January 2025;
Published: 06 February 2025.
Edited by:
Apostolos Zaravinos, European University Cyprus, CyprusReviewed by:
Kwong Tsang, Precision Biologics, Inc., United StatesCopyright © 2025 Tsurui, Hamada, Mura, Suzuki, Iriguchi, Ishiguro, Hirasawa, Ohkuma, Shimokawa, Ariizumi, Kubota, Horiike, Wada, Yoshimura, Tsuji, Kiuchi and Tsunoda. This is an open-access article distributed under the terms of the Creative Commons Attribution License (CC BY). The use, distribution or reproduction in other forums is permitted, provided the original author(s) and the copyright owner(s) are credited and that the original publication in this journal is cited, in accordance with accepted academic practice. No use, distribution or reproduction is permitted which does not comply with these terms.
*Correspondence: Toshiaki Tsurui, dC50c3VydWlAbWVkLnNob3dhLXUuYWMuanA=
Disclaimer: All claims expressed in this article are solely those of the authors and do not necessarily represent those of their affiliated organizations, or those of the publisher, the editors and the reviewers. Any product that may be evaluated in this article or claim that may be made by its manufacturer is not guaranteed or endorsed by the publisher.
Research integrity at Frontiers
Learn more about the work of our research integrity team to safeguard the quality of each article we publish.