- 1Department of Neurosurgery, Charité – Universitätsmedizin Berlin, Corporate Member of Freie Universität Berlin, Humboldt-Universität zu Berlin, and Berlin Institute of Health, Berlin, Germany
- 2Department of Neurosurgery, Städtisches Klinikum Karlsruhe, Karlsruhe, Germany
- 3Department of Neurosurgery, University of Foggia, Foggia, Italy
- 4Department of Neurosurgery, University of Messina, Messina, Italy
- 5Cluster of Excellence: “Matters of Activity. Image Space Material,” Humboldt University, Berlin, Germany
- 6Berlin Institute of Health at Charité – Universitätsmedizin Berlin, Berlin Institute of Health (BIH) Biomedical Innovation Academy, BIH Charité Digital Clinician Scientist Program, Berlin, Germany
Accurate preoperative mapping is crucial for maximizing tumor removal while minimizing damage to critical brain functions during brain tumor surgery. Navigated transcranial magnetic stimulation (nTMS), magnetoencephalography (MEG), and functional magnetic resonance imaging (fMRI) are established methods for assessing motor and language function. Following PRISMA guidelines, this systematic review analyzes the reliability, clinical utility, and accessibility of these techniques. A total of 128 studies (48 nTMS, 56 fMRI, 24 MEG) were identified from various databases. The analysis finds nTMS to be a safe, standardized method with high accuracy compared to direct cortical stimulation for preoperative motor mapping. Combining nTMS with tractography allows for preoperative assessment of short-term and long-term motor deficits, which may not be possible with fMRI. fMRI data interpretation requires careful consideration of co-activated, non-essential areas (potentially leading to false positives) and situations where neural activity and blood flow are uncoupled (potentially leading to false negatives). These limitations restrict fMRI’s role in preoperative planning for both motor and language functions. While MEG offers high accuracy in motor mapping, its high cost and technical complexity contribute to the limited number of available studies. Studies comparing preoperative language mapping techniques with direct cortical stimulation show significant variability across all methods, highlighting the need for larger, multicenter studies for validation. Repetitive nTMS speech mapping offers valuable negative predictive value, allowing clinicians to evaluate whether a patient should undergo awake or asleep surgery. Language function monitoring heavily relies on the specific expertise and experience available at each center, making it challenging to establish general recommendations.
1 Introduction
Gliomas represent the most common intracranial malignant pathology in pediatric and adult populations (1). The extent of resection (EOR) represents an independent prognostic factor predicting the survival and quality of life of these patients (2–6). Therefore, preoperative planning as well as intraoperative mapping of cortical and subcortical hotspots is of utmost importance in neuro-oncological surgery (7, 8).
Intraoperative direct electrical stimulation (DES) is considered the gold standard for creating a map of the functional areas within and around the lesion and is commonly used to map language and sensorimotor function (9–11). The suitability for an awake surgery depends on various factors such as the patient’s personality and preoperative general/neurological condition, so not every patient is eligible for an awake surgery.
In recent years, significant advancements have been made in the field of preoperative functional mapping, with numerous international experts exploring the clinical applications of instruments such as navigated transcranial magnetic stimulation (nTMS), functional magnetic resonance imaging (fMRI), and magnetoencephalography (MEG) (12–14). The objective of this systematic review is to comprehensively and critically assess the role, advantages, and limitations of nTMS, fMRI, and MEG in preoperative mapping for managing patients with motor- and language-eloquent gliomas, and how these techniques may impact the extent of resection (EOR) and functional outcomes. In detail, the accuracy, resolution, reliability, and accessibility were investigated. Furthermore, new insights into the procedural intricacies of these methods are provided to enhance understanding of the current non-invasive solutions for sensorimotor and language mapping.
2 Materials and methods
2.1 Search strategy and selection criteria
A comprehensive, systematic search of the literature was performed in compliance with the updated Preferred Reporting Items for Systematic Reviews and Meta-Analyses (PRISMA) 2020 guidelines as shown in Figure 1. The literature review for articles was conducted in January 2023 using electronic databases including MEDLINE/PubMed, EMBASE, PLOS, and the Cochrane Library. Human studies published in English between 1997 and January 2024 were considered for inclusion. The primary search terms used were “fMRI”, “functional Magnetic Resonance Imaging”, “nTMS”, “[navigated] Transcranial Magnetic Stimulation”, “MEG”, “Magnetoencephalography”, “MSI”, and “magnetic source imaging”, “glioma”, and “preoperative mapping” in the titles and abstracts of articles using various MeSH combinations. Eligible articles consisted of original studies and experiences involving cohorts of more than 10 patients. Exclusion criteria were applied to case reports, publications detailing technical notes, and studies where gliomas made up less than 20% of the overall cohort.
2.2 Data extraction
Two authors independently reviewed all abstracts to recognize articles that warranted a full-text review. The abstracts were assessed against predetermined eligibility criteria, and all included studies were reviewed with a third author. The following information was obtained: the author’s name, country, publication year, number of patients, type of lesion, tumor localization, histology, and type of preoperative mapping. In particular, details on accuracy, resolution, reliability, accessibility, and patient comfort techniques were extracted for all three mapping techniques. The gathered data were stored in a centralized database using Microsoft Excel. We evaluated the methodological quality of the studies (to assess bias risk) using the JBI Critical Appraisal Checklist for Case Series. This system assigns a quality rating to case series ranging from 0 (poor methodological quality) to 10 (optimal methodological quality) (15, 16).
3 Results
A total of 907 records were identified and were then subdivided into three groups according to the described technique: nTMS (Figure 1), fMRI (Figure 2), and MEG (Figure 3). Any irrelevant research, review articles, meeting abstracts/summaries, editorials, and studies lacking data on post-operative neurological outcomes were excluded. Thus, 229 full texts were assessed for eligibility, with 99 studies being excluded for various reasons: inappropriate study design (n = 50), inappropriate setting (n = 5), inappropriate patient population (n = 14), insufficient population size (n = 7), inappropriate intervention (n = 12), inappropriate outcomes (n = 3), through an automated system (Covidence) and one paper was not retrieved. Finally, 128 publications were included in the qualitative analysis: 48 nTMS studies (Table 1), 56 fMRI studies (Table 2), and 24 MEG studies (Table 3).
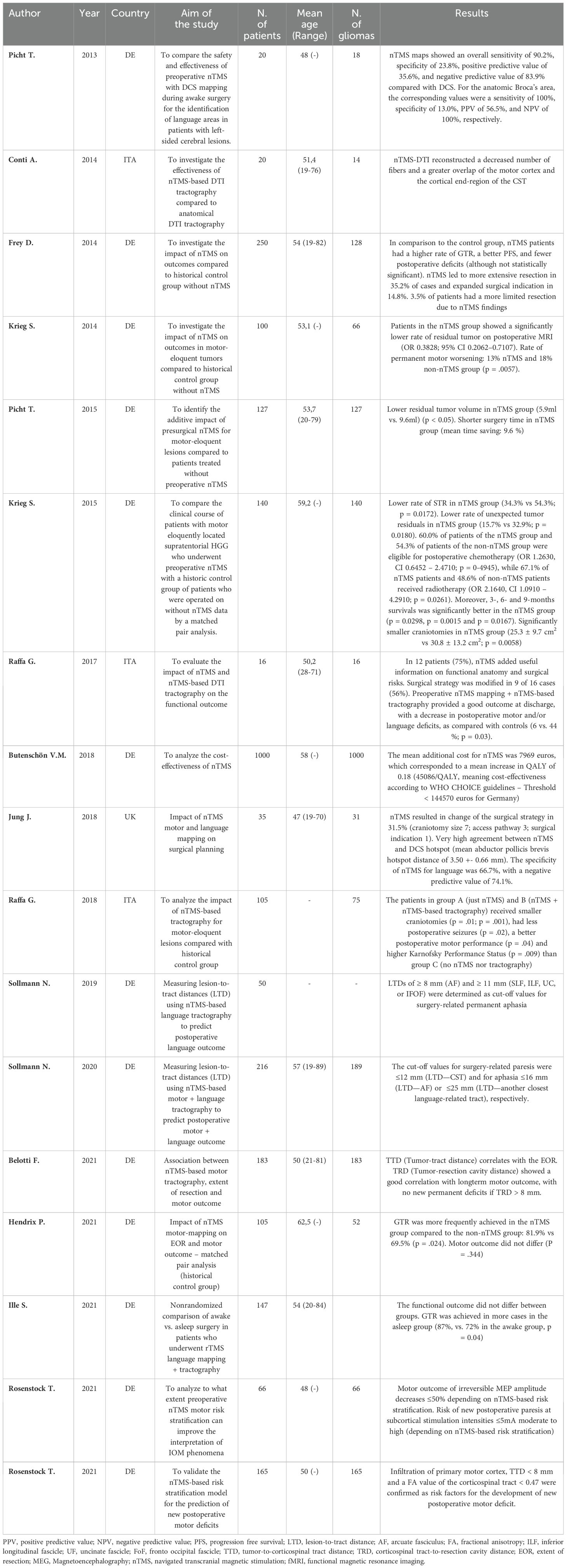
Table 1. Extraction of the most significant studies investigating nTMS's role as a preoperative mapping tool.
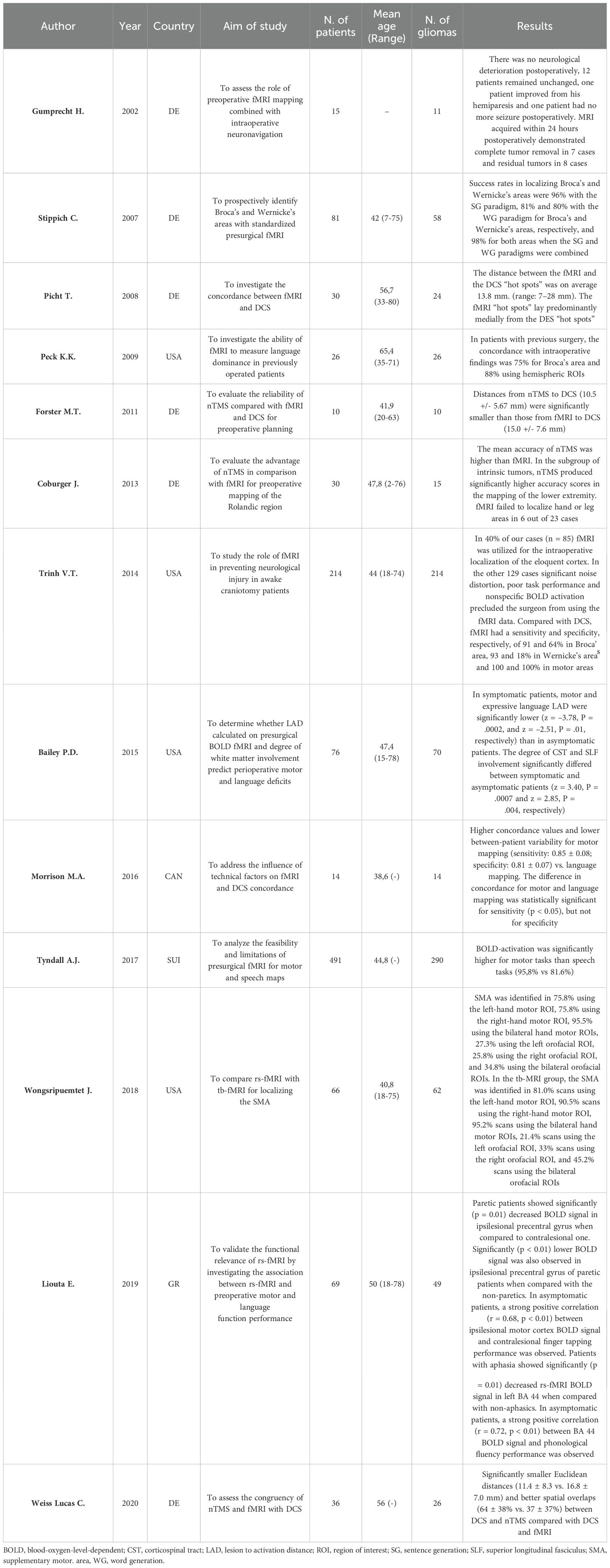
Table 2. Extraction of the most significant studies investigating fMRI’s role as a preoperative mapping tool.
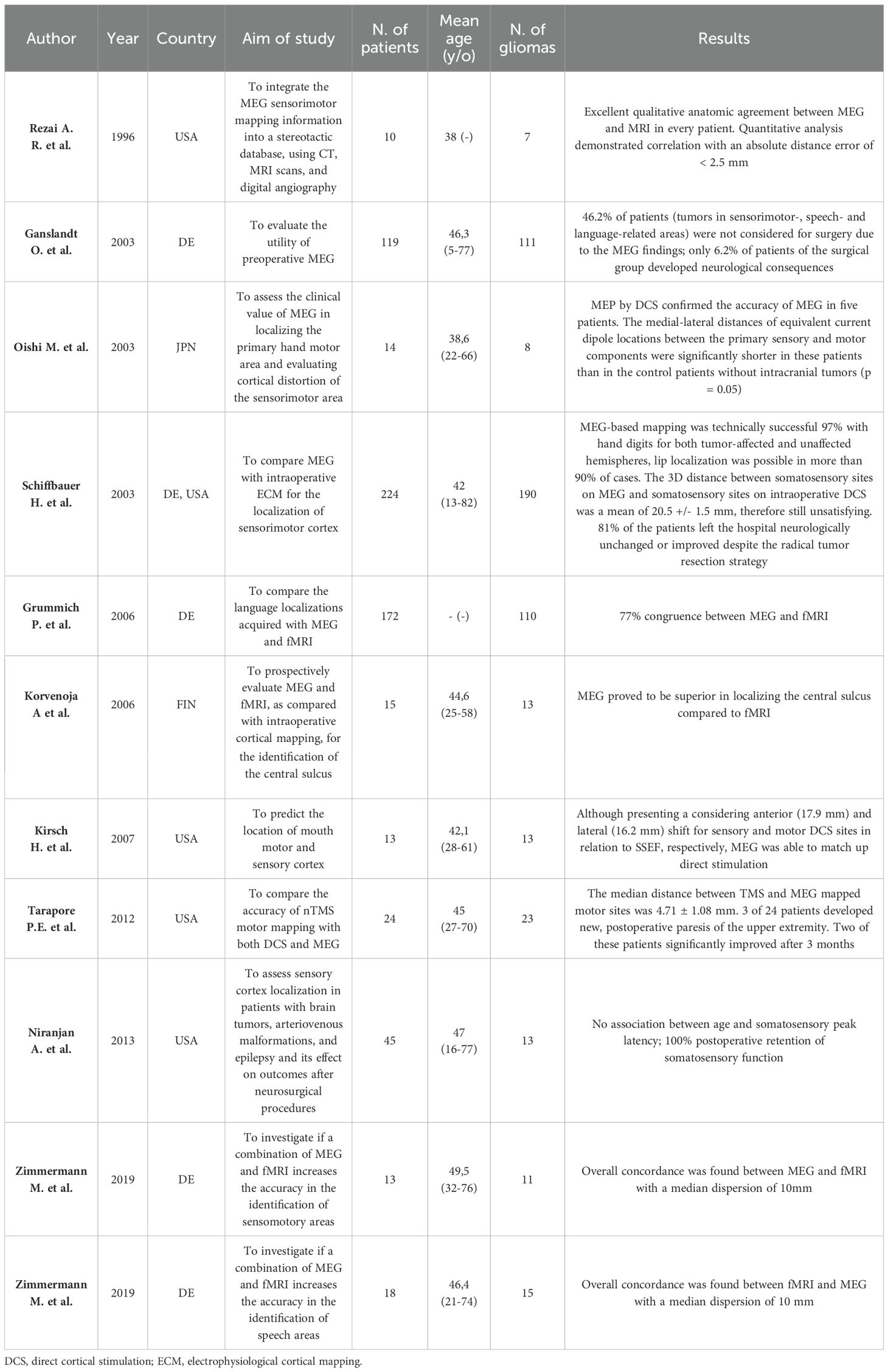
Table 3. Extraction of the most significant studies investigating MEG’s role as a preoperative mapping tool.
3.1 nTMS
Since its introduction in 1985 by Barker et al., nTMS has been used in specialized neurosurgical centers to improve tumor resections in eloquent brain areas without increasing the risk of postoperative neurological deficits. The safety and tolerability of nTMS for neurosurgical planning was demonstrated by Tarapore et al., who reported no seizures or other adverse events in a multicenter study of 733 brain tumor patients (17).
This non-invasive brain stimulation technique uses a coil to generate a variable magnetic field (strength ranging between 2-3 Tesla), inducing changing electrical currents in the brain through electromagnetic induction (Figure 4). (18–20) Due to the brain’s non-uniform structure, current distributions may be distorted, leading to the development of technical methods to reduce spatial errors. Various techniques, including stereotactic positioning with optical navigation systems, have been employed for precise targeting specific brain regions, resulting in navigated (n)TMS (18–22).
The motor resting threshold (RMT) is a well-known standardized parameter in neurophysiology and describes the individual excitability of the motor system (23–25). It varies between individuals (e.g. due to anatomical differences that influence the distance between the coil and cortex). The RMT is often used, for example, to assess the intraindividual excitability between the hemispheres or to adjust the stimulation intensity for motor and language mappings (26, 27).
3.1.1 nTMS motor mapping
In 2009, Picht et al. reported one of the earliest uses of an electromagnetic navigation system to position the nTMS coil in a larger, prospective patient cohort (28). In this and other studies, the accuracy was validated in comparison with direct cortical stimulation (DCS) (mean deviation from DCS 2-4mm) (29–32). A direct comparison between nTMS- and fMRI-determined motor areas with DCS showed better agreement for the nTMS motor mapping (33). When using nTMS, it is important to consider the calculated stimulation accuracy after co-registration with MRI and to accept only a calculated tolerance range of ≤2 mm. Failure to comply has been shown in a study to result in individual cases with significant deviations compared to DCS (34).
Many institutions have explored the clinical benefits of nTMS in surgical planning due to its spatial accuracy in creating three-dimensional functional maps (8, 35, 36). In a study by Rizzo et al., neurosurgeons reported that nTMS motor mapping provided valuable anatomo-functional information in 71% of cases and influenced operative techniques in 29% of surgeries, leading to changes in surgical strategy (37). Frey et al. also observed changes in surgical approaches for over half of patients mapped with nTMS, resulting in a significant increase in gross total resections from 42% to 59% (38). A comprehensive/summarizing meta-analysis by Raffa et al. showed a reduction in postoperative motor deficits (OR = 0.54), an improvement in the extent of resection (GTR OR: OR = 2.32) and an optimization of craniotomy size (-6cm2) and operation duration (-10min) in motor eloquent brain tumors (39).
3.1.2 nTMS-based diffusion tensor imaging fiber tracking of the CST
The idea behind combining nTMS motor maps and DTI tractography was to provide a function-based, individual tractography of the essential tracts of the CST. Conventional tractography algorithms often failed to visualize the CST due to tumor mass effects and peritumoral edema, which has now become possible through the integration of functional nTMS data (40, 41). In detail, Frey et al. defined the term “FA threshold” which enables an individually tailored representation of the CST (40).
In 2014, Conti et al. introduced a technique for somatotopic DTI tractography based on the somatotopic nTMS motor mapping of different muscles (42). The preoperative tractography analyses of 20 patients were integrated into the intraoperative neuronavigation and the accuracy was confirmed using subcortical stimulation. Thus, nTMS-based tractography provides a reliable anatomic and functional characterization of the motor pathway. Rosenstock et al. proposed a manual for standardized nTMS-based tractography confirming the reliability and user-independence of nTMS-based tractography. Even inexperienced users are able to perform the tractography and determine the tumor-tract distance reliably (ICC > 0.9) (43). The superiority in terms of accuracy of nTMS-based tractography compared with conventional tractography was confirmed with direct stimulation (44). In comparison to fMRI as another method for function-based tractography, nTMS-based tractography showed higher plausibility rate, whereas fMRI-based tractography falsely visualized posterior pathways that are presumably functionally more associated with the sensory system (45).
3.1.3 Prognostic value of nTMS mapping/nTMS-based tractography for the motor function
Takakura et al. found a correlation between hotspot-tumor distances and postoperative upper-extremity motor function recovery (46). Patients with greater distances (>10mm) showed better grip strength recovery at 3 months. Krieg et al. demonstrated the prognostic value of nTMS functional mapping in influencing surgical strategy and reducing iatrogenic damage (47, 48). They compared a cohort of 100 nTMS-guided tumor resections with a historical control group and found lower residual tumor rates, improved motor function, smaller craniotomies, and increased eligibility for chemotherapy and radiation therapy in the nTMS group.
Moser et al. and Hendrix et al. further validated the prognostic significance of nTMS positive motor areas anterior to the motor cortex but achieved conflicting results (12, 49). However, when simulating premotor areas care must be taken to ensure that the motor cortex is not stimulated incidentally, which can lead to false-positive results (50).
Several authors investigated the white matter integrity of the CST by analyzing the tumor-tract distance (TTD) as well as the fractional anisotropy (FA) and the apparent diffusion coefficient (ADC). Interestingly, Rosenstock et al. demonstrated that a lower average FA within the affected CST as well as a higher average ADC were associated with deteriorated postoperative motor function (43). In a prospective cohort of 113 patients, no new postoperative motor deficit occurred when the TTD was greater than 8 mm and the precentral gyrus was not infiltrated (27). The relevance of the TTD was confirmed by Sollmann et al. who found that no patient with a TTD ≥ 12 mm suffered from new surgery-induced permanent paresis (51).
Finally, Rosenstock et al. proposed a bicentric-validated risk stratification model using nTMS motor maps and nTMS-based tractography to predict postoperative motor outcomes (short-term and long-term after 3 months) in glioma patients (27, 52). In a study of 278 patients, they found no new permanent deficits when the tumor was > 8 mm distant from the CST and did not infiltrate the precentral gyrus. By assessing the integrity of the CST (by measuring the fractional anisotropy) and of the motor system (by determining the RMT), they could calculate the patients’ individual risk of a new postoperative motor deficit. Further studies demonstrated the clinical and prognostic value of nTMS-based DTI for motor eloquent tumors (53–56). Ivren et al. demonstrated that nTMS-based prediction outperforms an anatomy-based risk assessment (only using structural MRI data) and better predicts the extent of resection (57).
Despite the many published (prospective) studies, no RCT data is yet available since the analysis of the first RCT on the use of preoperative nTMS mapping is pending (NCT02879682).
3.1.4 nTMS language mapping
One of the first experiences of repetitive navigated transcranial magnetic stimulation (rnTMS) was reported by George et al., Herwig et al., and Lioumis et al. for language mapping by inducing temporary disruptions in specific brain regions to identify language-related areas (19, 58, 59). Several stimulation algorithms have been studied over the years, resulting in a recommendation on rnTMS language mapping stated by an international consortium of experts (26): stimulation frequency 5-7 Hz, applied pulses 5-7, picture presentation time 500-700ms, time between picture presentation and stimulation 0-300ms. However, rnTMS language mapping with stimulation frequencies up to 50 Hz was also evaluated in healthy subjects to improve the reliability (60).
In 2013, Picht et al. conducted the first study comparing the use of rnTMS for functional mapping of language areas in combination with direct electrical stimulation (DES) during awake surgery to analyze its reliability in identifying language regions in patients with left-sided lesions (61). The overall evaluation of the following studies showed a high (and therefore clinically significant) sensitivity (range: 63%-97%) and negative predictive value (range: 74%-99%) (62–64). The accuracy appears to be higher for the frontal language regions (Broca’s area), where a sensitivity and NPV of 100% were achieved in two independent studies (47, 61). Thus, tumor resections in rnTMS language-negative areas can be performed with a very low risk for the occurrence of new postoperative language deficits. Some centers even decide not to perform awake craniotomies in these cases, however, the proximity to language-associated tracts must be taken into account (65).
Disadvantages of rnTMS language mapping are the average and highly variable specificity (13%-98%) and positive predictive value (24%-69%). Schwarzer et al. showed a very high rate of false-positive mapping results in patients with neurocognitive deficits and preoperative language impairment, so that rnTMS language mapping should not be performed in cases with baseline error rates >28% (66).
Similar to the nTMS-based DTI FT of the CST, the combination of rnTMS language mapping with DTI studies has been utilized to visualize the language network by emphasizing the rnTMS-associated functional cortico-subcortical tracts. Several studies have explored the integration of rnTMS language mapping and rnTMS-based DTI FT for surgical planning, showing consistent results (67–70). In conclusion, rnTMS-based DTI FT has proven to be valuable in guiding surgical decisions and reducing residual tumor volume (71). However, it should be noted that tractography solely based on rnTMS seeds is not recommended since this will lead to unplausible tractography maps (72). According to the current state of clinically available tractography algorithms, it is rather recommended to first identify pathways based on anatomical ROIs and then integrate the rnTMS information to investigate functional corticosubcortical pathways (67, 73).
3.2 fMRI
fMRI is a crucial preoperative mapping tool that identifies cortical and subcortical activity by detecting changes in brain vascular flow through the BOLD (blood-oxygen-level-dependent) effect, which associates cerebral blood flow with neural activity via neurovascular coupling (Figure 5) (74). Since the introduction of the BOLD protocol in 1990 by Ogawa and colleagues (75), fMRI has become a valuable tool in neurosurgery and functional neuroscience due to its ability to accurately show brain regions involved in processing internal or external stimuli (76). This oxygenation-sensitive imaging technique provides precise spatio-temporal accuracy by capturing changes in oxyhemoglobin and deoxyhemoglobin levels linked to neuronal activity (77). While other protocols have been suggested, discussing their advantages and disadvantages is beyond the scope of this review (78).
3.2.1 Task-based fMRI motor mapping
In 1998, Pujol et al. reported the first cases of using three-dimensional fMRI mapping to identify the central sulcus as part of preoperative planning for patients with space-occupying lesions (79). The most commonly used task-based motor mapping fMRI methodology is based on conscious, repetitive motor activations in response to the examiner’s instructions (7, 80–82). Such protocols are based on BOLD signal fluctuations between task-induced and control states, whose correlation with intraoperative mapping data has been shown in several studies (83).
Several studies confirmed the clinical utility of tb-fMRI motor mappings, e.g. in selecting the appropriate surgical approach, minimizing craniotomy size (and thus brain exposure), and reducing postoperative neurological complications caused by tumor-induced alterations in expected anatomy and function (84). The studies on neuroplasticity showed varying- and partially contradictory - results. For instance, Baciu et al. (85) and Alkadhi et al. (86) used a block paradigm to study neural plasticity in patients with primary brain lesions, focusing on hand, leg, and mouth movements. They observed that the most common tumor-induced neural plasticity involves inter-hemispheric reorganization, with only few cases of intra-hemispheric reorganization or activation outside the primary motor area. In contrast, Nelson et al. (87) demonstrated varying levels of neural reorganization in the supplementary motor area (SMA), including ipsilateral SMA activation, bilateral activation, and different grades of reorganization. In the small cohort of 12 patients, they found a significant association between the distance between the tumor and SMA region (5mm) and the occurrence of new postoperative neurological deficits (paralysis and speech impairment/mutism).
Although many studies on the clinical applicability of task-based fMRI motor mappings exist, this technology has two significant drawbacks. Firstly, there is no established risk stratification that can differentiate between eloquent-activated areas (where resection leads to neurological deficits) and co-activated areas (where resection results in no or transient deficits). Secondly, several studies have shown that fMRI signal analysis near brain tumors is distorted due to neurovascular uncoupling (i.e. associated with vasogenic edema) and has led to inaccuracies compared to the gold standard (DCS) (88–93). Thus, the motor cortex could not be identified in 18% of patients and the distance between the DCS hotspot and fMRI hotspot was frequently >1cm, which hindered adequate surgical planning (94–96). Ultimately, the sensitivity and specificity of fMRI were 61.7% and 93.7% (94, 97, 98). In DCS-based comparative studies, fMRI was significantly inferior compared to nTMS in terms of identifying motor eloquent areas (33, 95, 99). In an effort to improve subcortical spatial orientation during surgical resection, fMRI-identified areas were used as ROIs for DTI tractography of the corticospinal tract (100, 101). In a comparative study, fMRI-based tractography was significantly inferior to nTMS-based tractography in terms of plausibility and occurrence of aberrant fiber pathways. Particularly when the tumor was in close proximity to functional areas, changes in BOLD signal physiology occurred, highlighting nTMS-based tractography as the method of choice (45).
3.2.2 Resting-state-fMRI motor mapping
Dierker and colleagues were among the first to compare rs-fMRI with tb-fMRI acquisition (102). By detecting task-negative states, rs-fMRI enables researchers to identify spontaneous fluctuations in BOLD signals, forming resting-state networks (RSN) (103). The main benefit of rs-fMRI is the ability to generate functional maps even in uncooperative individuals (such as children or incompliant adults), as long as they remain calm during the scan (104–108). rs-fMRI tends to additionally detect network-based associated regions (such as the sensory cortex) (109), resulting in the detection of larger areas but also raising further questions about their surgical relevance and usability.
The aforementioned disadvantages of tb-fMRI are even more pronounced here, as rs-fMRI mapping relies essentially on identifying preserved anatomical landmarks that may be altered by the lesion. Furthermore, the acquisition times for rs-fMRI can still be too long at times, although interesting new advances through machine learning (ML) and artificial intelligence show promising results (110).
3.2.3 fMRI language mapping
Despite the complexity of the language network, the initial experiences date back to 1999 by Ruge et al., where a good concordance between tb-fMRI activated areas and DCS was found in 5 patients (111). Current standard paradigms include picture-naming tasks and word-listening tasks, which are also utilized in assessing neuroplasticity (112, 113). Although many studies have reported positive experiences with fMRI for investigating the language network (13, 114, 115), very similar limitations exist as with fMRI motor mapping. Particularly when the tumor is located very close to eloquent areas (<1cm), involves high-grade gliomas with blood-brain barrier disruption and perifocal edema, or well-vascularized tumors, authors have found significant inaccuracies (sensitivity: 37.1%, specificity: 83.4%) (116, 117). In another study involving 50 patients with left-hemispheric gliomas, in some cases, a right-hemispheric language dominance was mistakenly indicated, which was explained by a reduced perilesional fMRI signal due to lesion-induced neurovascular uncoupling (118).
Studies on rsfMRI have shown that even uncooperative patients could be examined, leading to the detection of co-activated areas and thus overcoming this intrinsic limitation of tb-fMRI. However, this also complicates the interpretation of results, as it remains difficult to differentiate between eloquent and non-eloquent, co-activated areas (119–121). rsfMRI analyses should be interpreted with caution, especially when tumors alter the anatomical landmarks as well as the functionality of the RSN, leading to incorrect results (122).
3.3 MEG
Since its inception in 1968 by Illinois physicist David Cohen, MEG is a relatively new technology used in cognitive brain sciences, oncological neurosurgery, and neurotherapy with real-time neurofeedback (123, 124). It operates on high-sensitivity arrays of SQUIDs (superconducting quantum interference devices) magnetometers to capture and map synchronized electrical brain activities by recording magnetic fields from activated synapses (Figure 6). Despite limited use in neurosurgery due to cost and infrastructure requirements, MEG-based brain mapping offers advantages over other techniques, such as less distortion compared to EEG and superior temporal resolution compared to fMRI (125). While MEG mapping is not widely studied as a standalone technique in medical literature, it is often combined or compared with other methods like nTMS and fMRI for preoperative planning in neuro-oncology.
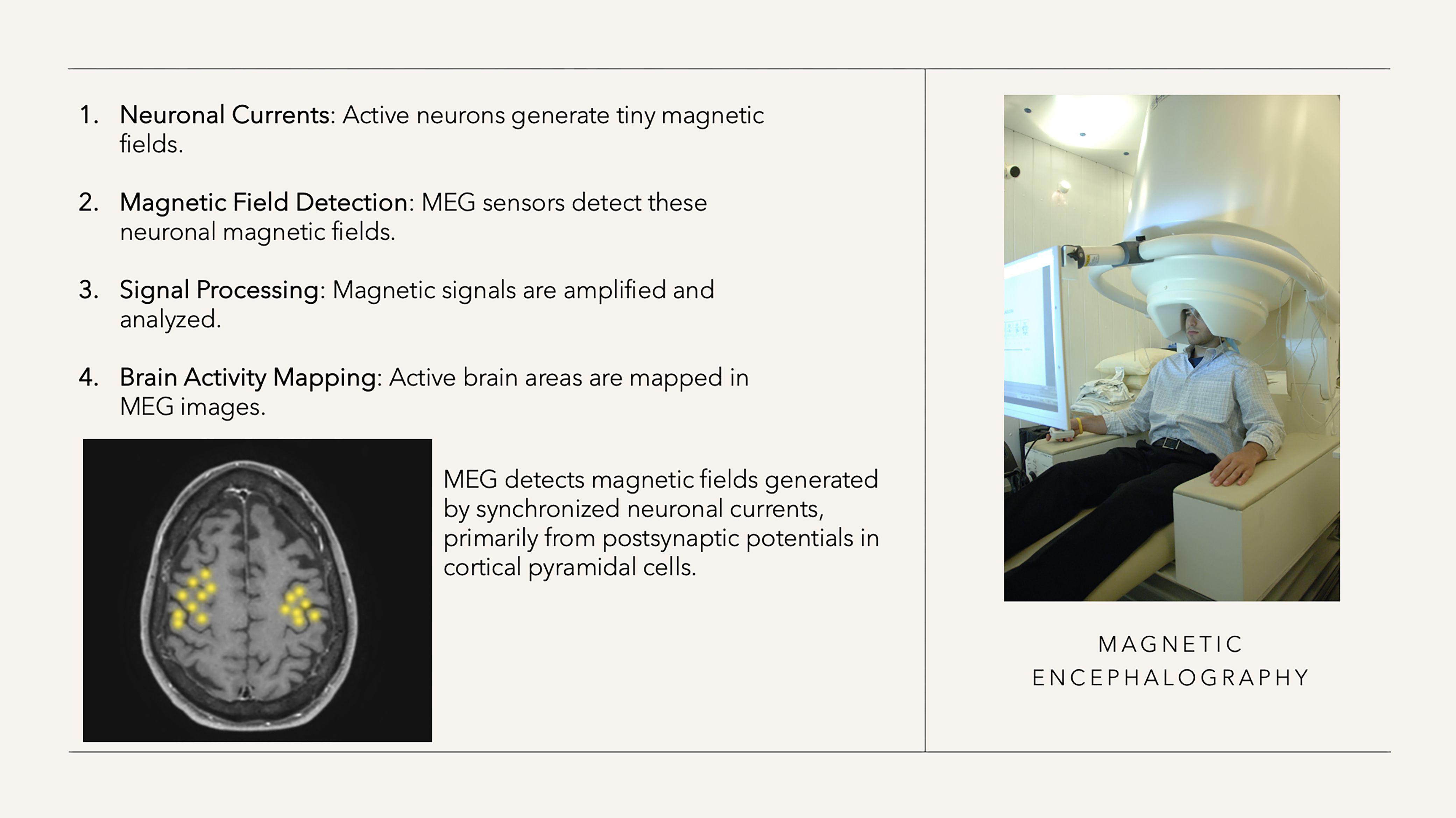
Figure 6. MEG characteristics (right-sided figure approved by the National Institute of Mental Health, Department of Health and Human Services).
3.3.1 MEG motor mapping
The first clinical experience with magnetoencephalography (MEG) dates back to 1995, when Gallen et al. attempted to utilize somatosensory evoked potentials to predict the pattern of phase reversals observable intraoperatively on the cortical surface in six patients with various pathologies. They identified a correlation across the entire cohort, with an average deviation of 8 mm compared to the intraoperative findings (126). In 1996, Rezai et al. reported on the integration of MEG-derived brain mapping with various imaging techniques to develop a preoperative algorithm for navigating during the resection of eloquent lesions in the sensorimotor cortex (127). Schiffbauer et al. used MEG to investigate the spatial relationship between functional eloquent areas and tumors, finding functional activity in 18% of grade 2 tumors, 17% of grade 3 tumors, and 8% of grade 4 tumors (128).
In numerous studies, MEG results have been compared with fMRI analyses and validated in a few studies using intraoperative DES. Overall, there was good agreement (and partial superiority) with fMRI analyses (129–131), but a mean deviation of DES-identified motor hotspots of 12.5mm, which poses a significant limitation for neurosurgical operation planning (132–135). This spatial deviation poses a particular challenge for precise neurosurgical planning. MEG localization often relies on the mapping of somatosensory evoked fields (SEFs) rather than signals from the motor cortex. SEFs localize the somatosensory cortex, with sources located deeper in the central sulcus, while direct electrical stimulation (DES) targets the crown of the precentral gyrus to identify motor hotspots. This anatomical variance between somatosensory and motor cortex activation contributes to the observed spatial discrepancies and must be taken into account (136, 137). The reliability of motor mapping for planning brain tumor resections is not compromised in centers with experience in MEG analysis, as the deviation between the activity shown by MEG and the DES stimulation was generally ≤10 mm (133).
Tarapore et al. compared nTMS with MEG and DES for cortical motor mapping in patients with malignant lesions near the central region. While a direct comparison between MEG and DES was not conducted, the mean distance between nTMS and MEG imaging motor sites was 5 mm, and between nTMS and DES motor sites was 2 mm, suggesting a strong alignment between MEG and nTMS (34). In one case of the study, no MEPs could be elicited by preoperative nTMS or intraoperative DCS; however, there was motor-functional MEG activity within the lesion. Postoperatively, a permanent motor deficit was evident in this case. This individual case suggests that MEG may be valuable in situations where neither nTMS nor DCS is able to induce MEPs.
In a study of 119 patients with gliomas, the relative spatial relationship between the tumor and eloquent brain areas (specifically sensory-motor, visual, and language areas) was analyzed (138). In high-risk cases with distances <5mm, surgery was avoided, which occurred in 46% of patients. Although the rate of postoperative neurological deficits was low at 6%, it raises questions about whether some non-operated patients may have been suitable for resection, potentially missing the opportunity for longer overall survival.
In a feasibility study, the language associated areas detected by MEG were used as ROI and combined with diffusion tensor imaging (DTI) to visualize the arcuate fasciculus in patients with lesions in language regions. However, the clinical relevance and benefits for surgical planning and the correlation with postoperative outcomes have yet to be investigated (139).
3.3.2 MEG language mapping
In contrast to MEG motor mapping, there are very few studies available on MEG-based language mapping, which primarily focus on interhemispheric language lateralization. In one of the initial studies, Grummich et al. combined fMRI and MEG to investigate language localization in 172 patients with brain lesions (140). They found a high level of agreement between the two techniques in identifying language sites (77% congruence), with only a small percentage showing differences (4%). MEG mapping was shown to be superior in nearly half of the cases (in which the BOLD signal was suppressed, particularly in glioma patients). Another study investigating language localization was conducted by Szymanski et al. in 2001, who demonstrated a general left asymmetry in right-handed neurosurgical patients through MEG mapping, as well as a right asymmetry in two patients with confirmed right hemispheric language dominance via the amobarbital test (141).
While the Wada test is nowadays used by only 12% of epilepsy centers for language lateralization assessment, it has been considered the “gold standard” for preoperative determination of language dominance (142). Doss et al. validated MEG-based language localization with the Wada test, reporting a relatively low concordance rate (69%) between the two methods (143). Ota et al. (144) compared MEG and fMRI with NIRS (near-infrared spectroscopy) and the Wada test for language lateralization, finding that fMRI and NIRS had higher sensitivity and specificity in patients with typical hemispheric dominance, while NIRS showed better specificity in patients with right language lateralization (144). However, the authors emphasized the individual, high technical requirements (device differences, patient characteristics such as brain edema and movement artifacts) associated with MEG, fMRI and NIRS, which make (routine) use difficult (145). In a comparative study between MEG and nTMS language mapping for determining language dominance, a 64% agreement was found. However, no validation with the WADA test was performed which limits the significance of the study (146).
4 Discussion
The management of CNS tumors involves intricate preoperative planning that considers individual patient characteristics, especially their functional neuroanatomy, to achieve maximal resection while preventing new neurological deficits. Most studies on preoperative mapping were fMRI-based (n=56), followed by nTMS (n=48), with significantly fewer studies on MEG (n=26). Motor mapping is well established in routine practice, with nTMS-based motor mapping proving to be the most accurate. Studies on preoperative language mapping showed highly heterogeneous results with varying levels of agreement with direct electrical stimulation (DES) during awake craniotomies (Table 4).
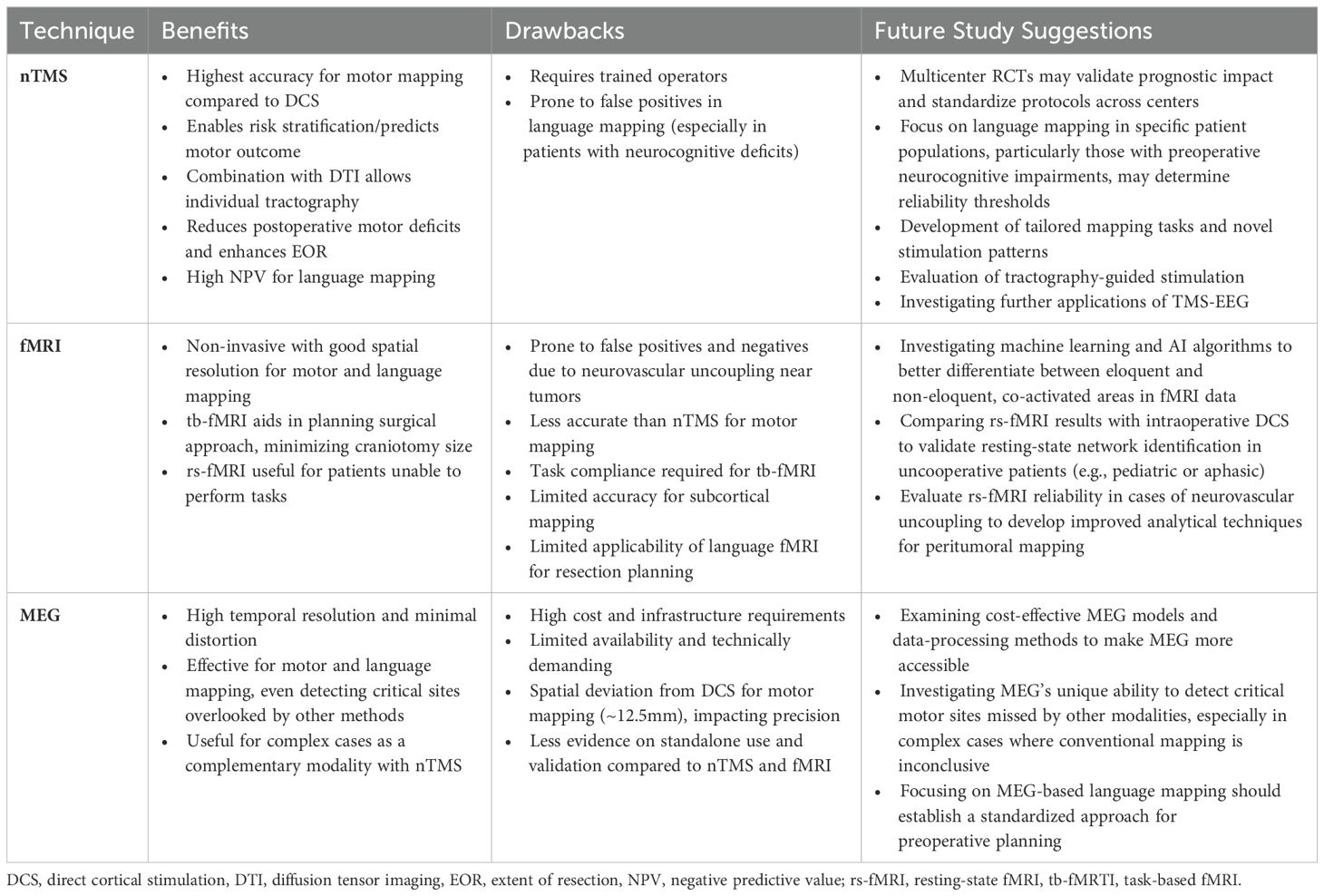
Table 4. Benefits, drawbacks and potential future studies of nTMS, fMRI, and MEG in preoperative mapping.
4.1 Preoperative motor mapping
In previous studies, nTMS-based motor mapping was found to be the most accurate method compared to DCS for both upper and lower extremities. In contrast, fMRI-based mapping was not as precise and exhibited a greater deviation from the DCS-based motor hotspot (95, 99, 147, 148). The nTMS motor mapping is based on single-pulse stimulation, and only if the stimulation induces a motor-evoked potential is it considered positive. In contrast, with fMRI and MEG, areas are also considered activated that would be assigned to the secondary motor system (such as movement planning in prefrontal areas). However, resections in the co-activated areas do not necessarily lead to motor deficits, which must be taken into account when interpreting fMRI- and MEG-based motor maps to avoid unnecessarily restrictive resections and compromising oncological outcomes.
Besides the high standardization and interrater reliability, an nTMS risk stratification has been developed based on motor mapping and tractography, which further strengthened its application in routine practice (27, 52). In detail, the risk for a new or aggravated motor deficit can be predicted preoperatively, allowing for individual patient counseling and surgical planning. No new motor deficits occurred in patients with a TTD > 8mm and without motor cortex infiltration, so in these cases, gross total resection (GTR) is recommended, and the use of IOM is not necessarily required. Although intraoperative validation studies have shown high agreement with subcortical stimulation, the use of IOM is strongly recommended for resecting motor eloquent gliomas with a tumor tract distance (TTD) ≤ 8 mm to preserve the integrity of the motor system, as it is not possible to account for all intraoperative influences like brain shift (149).
In addition to improving clinical outcomes and preoperative planning, the cost-effectiveness of nTMS motor mapping has been demonstrated (145). Corresponding calculations for fMRI- and MEG-based motor mappings are not available.
The acquisition costs for an MEG system are substantial and comparable to those of a 3T MRI scanner; however, recent technological advancements, such as helium recycling, have contributed to reducing long-term operating costs. Nevertheless, the acquisition and operating costs still exceed those of an nTMS system (150). Furthermore, the process of analyzing dipole sources in MEG is automated. The operation (and associated costs) of an MEG device after its installation can be streamlined just like that of fMRI, which could enhance its feasibility and accessibility in hospitals with adequate infrastructure (151).
In addition to SEFs, alternative MEG-based methods such as corticomotor coherence (CMC) and beta band suppression (event-related desynchronization or ERD) have been investigated for mapping motor cortex function. While these techniques provide valuable insights into the dynamics of the motor network, they often result in complex data analysis and frequently yield spatially dispersed activation patterns. In contrast, it has been shown that the nTMS motor mapping provides high spatial resolution with excellent intra- and interindividual reliability (152).
Finally, ML algorithms were recently applied to detect previously unrecognized patterns. For example, Shams et al. recently developed an ML algorithm to predict motor deficits in glioma patients based on quantitative parameters such as FA, ADC, axial, and radial diffusivity examined along the tract statistics (153). However, these AI-supported methods are highly technical and require considerable technical expertise with promising - but still insufficient - accuracy, which has so far significantly limited their routine use and dissemination in patient care.
4.2 Preoperative language mapping
Preoperative language mapping is known to be more challenging to investigate, because the language network is significantly more complex than the motor system and because there is a greater variety of possible language deficits (154). Studies comparing the concordance of the mapping methods with DCS did not show consistent results, thus a standardized preoperative language mapping method or protocol has not been established across centers. In addition to a few studies comparing different mapping methods, there are no multicenter, larger studies demonstrating the superiority or inferiority of the respective techniques. The treatment of patients with language-eloquent brain tumors is characterized by center-specific experience and expertise in the respective mapping method available. The decision-making process between language eloquent brain areas and tumor tissue to be resected is therefore far from standardized.
In studies on rTMS language mapping, a high specificity with a very high negative predictive value was predominantly observed (61, 63, 64, 66, 155–157). Centers with extensive experience in rTMS language mapping decide on the basis of the rTMS mapping results (so-called negative mapping) whether patients should undergo awake surgery. rTMS-negative areas can therefore be safely resected, but care must be taken at the resection margins to avoid damaging the subcortical language network. In a direct comparison between rTMS and fMRI, rTMS mapping showed a higher negative predictive value (rTMS 100% vs. 73% fMRI), indicating a better suitability for preoperative mapping (158–162). In cases of severe neurocognitive impairments or advanced aphasia, rTMS language mapping should not be performed due to increased false-positive results (66). Interpretation of fMRI language mapping is particularly difficult due to frequent coactivation of areas leading to false-positive activations and peritumoral false-negative signal suppression (due to neurovascular uncoupling caused by the lesion).
The mapping technologies can also be used to determine hemispheric language dominance (115, 118, 142, 146). However, as the majority of patients have a left hemispheric dominance, the results of the examination rarely have an impact on the treatment strategy.
5 Conclusion
nTMS motor mapping has proven to be superior to fMRI in terms of accuracy (compared to DCS) and clinical applicability. nTMS with nTMS-based tractography allows for standardized preoperative risk stratification to predict short-term and long-term motor outcomes. MEG demonstrates high accuracy in motor mapping; however, its use is limited due to the high costs and technical demands, similar to fMRI. Mapping language function remains challenging with a wide range of agreement with DCS for all mapping methods, thus no standard has been established in clinical routine. rTMS stands out for its high negative predictive value across studies, allowing it to evaluate patients for an awake craniotomy. When interpreting fMRI results, attention must be paid to co-activated but non-eloquent areas (false positives) as well as false negatives due to neurovascular uncoupling, significantly limiting its utility in preoperative planning for both motor and language functions.
Author contributions
AL: Conceptualization, Data curation, Formal analysis, Investigation, Writing – original draft. FC: Formal analysis, Writing – original draft. US: Supervision, Writing – review & editing. PV: Supervision, Writing – review & editing, Funding acquisition, Resources. GR: Conceptualization, Supervision, Validation, Writing – review & editing. FA: Supervision, Writing – review & editing. AG: Supervision, Writing – review & editing. ME: Conceptualization, Writing – review & editing. TP: Conceptualization, Funding acquisition, Methodology, Project administration, Resources, Supervision, Validation, Writing – review & editing. AC: Supervision, Writing – review & editing. TR: Conceptualization, Formal analysis, Investigation, Methodology, Project administration, Resources, Supervision, Writing – original draft, Writing – review & editing.
Funding
The author(s) declare financial support was received for the research, authorship, and/or publication of this article. The authors acknowledge the support of the Cluster of Excellence Matters of Activity. Image Space Material funded by the Deutsche Forschungsgemeinschaft (DFG, German Research Foundation) under Germany´s Excellence Strategy – EXC 2025. TR is participant in the BIH Charité Digital Clinician Scientist Program funded by the Charité – Universitätsmedizin Berlin, and the Berlin Institute of Health at Charité (BIH).
Conflict of interest
The authors declare that the research was conducted in the absence of any commercial or financial relationships that could be construed as a potential conflict of interest.
Publisher’s note
All claims expressed in this article are solely those of the authors and do not necessarily represent those of their affiliated organizations, or those of the publisher, the editors and the reviewers. Any product that may be evaluated in this article, or claim that may be made by its manufacturer, is not guaranteed or endorsed by the publisher.
Supplementary material
The Supplementary Material for this article can be found online at: https://www.frontiersin.org/articles/10.3389/fonc.2024.1481430/full#supplementary-material
References
1. Ostrom QT, Gittleman H, Stetson L, Virk SM, Barnholtz-Sloan JS. Epidemiology of gliomas. Cancer Treat Res. (2015) 163:1–14. doi: 10.1007/978-3-319-12048-5_1
2. Jakola AS, Myrmel KS, Kloster R, Torp SH, Lindal S, Unsgård G, et al. Comparison of a strategy favoring early surgical resection vs a strategy favoring watchful waiting in low-grade gliomas. JAMA. (2012) 308:1881. doi: 10.1001/jama.2012.12807
3. Almenawer SA, Badhiwala JH, Alhazzani W, Greenspoon J, Farrokhyar F, Yarascavitch B, et al. Biopsy versus partial versus gross total resection in older patients with high-grade glioma: a systematic review and meta-analysis. Neuro-Oncol. (2015) 17:868–81. doi: 10.1093/neuonc/nou349
4. Lee CH, Kim DG, Kim JW, Han JH, Kim YH, Park CK, et al. The role of surgical resection in the management of brain metastasis: a 17-year longitudinal study. Acta Neurochir (Wien). (2013) 155:389–97. doi: 10.1007/s00701-013-1619-y
5. Zeppa P, De Marco R, Monticelli M, Massara A, Bianconi A, Di Perna G, et al. Fluorescence-guided surgery in glioblastoma: 5-ALA, SF or both? Differences between fluorescent dyes in 99 consecutive cases. Brain Sci. (2022) 12:555. doi: 10.3390/brainsci12050555
6. Palmieri G, Cofano F, Salvati LF, Monticelli M, Zeppa P, Perna GD, et al. Fluorescence-guided surgery for high-grade gliomas: state of the art and new perspectives. Technol Cancer Res Treat. (2021) 20:153303382110216. doi: 10.1177/15330338211021605
7. Tyndall AJ, Reinhardt J, Tronnier V, Mariani L, Stippich C. Presurgical motor, somatosensory and language fMRI: Technical feasibility and limitations in 491 patients over 13 years. Eur Radiol. (2017) 27:267–78. doi: 10.1007/s00330-016-4369-4
8. Picht T, Schulz J, Hanna M, Schmidt S, Suess O, Vajkoczy P. Assessment of the influence of navigated transcranial magnetic stimulation on surgical planning for tumors in or near the motor cortex. Neurosurgery. (2012) 70:1248–57. doi: 10.1227/NEU.0b013e318243881e
9. Yingling CD, Ojemann S, Dodson B, Harrington MJ, Berger MS. Identification of motor pathways during tumor surgery facilitated by multichannel electromyographic recording. J Neurosurg. (1999) 91:922–7. doi: 10.3171/jns.1999.91.6.0922
10. Roux FE, Durand JB, Djidjeli I, Moyse E, Giussani C. Variability of intraoperative electrostimulation parameters in conscious individuals: language cortex. J Neurosurg. (2017) 126:1641–52. doi: 10.3171/2016.4.JNS152434
11. Morshed RA, Young JS, Lee AT, Hervey-Jumper SL. Functional mapping for glioma surgery, part 2. Neurosurg Clin N Am. (2021) 32:75–81. doi: 10.1016/j.nec.2020.09.001
12. Hendrix P, Dzierma Y, Burkhardt BW, Simgen A, Wagenpfeil G, Griessenauer CJ, et al. Preoperative navigated transcranial magnetic stimulation improves gross total resection rates in patients with motor-eloquent high-grade gliomas: A matched cohort study. Neurosurgery. (2021) 88:627–36. doi: 10.1093/neuros/nyaa486
13. Gumprecht H, Ebel GK, Auer DP, Lumenta CB. Neuronavigation and functional MRI for surgery in patients with lesion in eloquent brain areas. Min - Minim Invasive Neurosurg. (2002) 45:151–3. doi: 10.1055/s-2002-34341
14. Yousry T. Localization of the motor hand area to a knob on the precentral gyrus. A New landmark. Brain. (1997) 120:141–57. doi: 10.1093/brain/120.1.141
15. Munn Z, Barker TH, Moola S, Tufanaru C, Stern C, McArthur A, et al. Methodological quality of case series studies: an introduction to the JBI critical appraisal tool. JBI Evid Synth. (2020) 18(10):2127–33. doi: 10.11124/JBISRIR-D-19-00099
16. Page MJ, McKenzie JE, Bossuyt PM, Boutron I, Hoffmann TC, Mulrow CD, et al. The PRISMA 2020 statement: an updated guideline for reporting systematic reviews. BMJ. (2021) 72:n71. doi: 10.1136/bmj.n71
17. Tarapore PE, Picht T, Bulubas L, Shin Y, Kulchytska N, Meyer B, et al. Safety and tolerability of navigated TMS for preoperative mapping in neurosurgical patients. Clin Neurophysiol. (2016) 127:1895–900. doi: 10.1016/j.clinph.2015.11.042
18. Ettinger GJ, Leventon ME, Grimson WEL, Kikinis R, Gugino L, Cote W, et al. Experimentation with a transcranial magnetic stimulation system for functional brain mapping. Med Image Anal. (1998) 2(2):133–42. doi: 10.1016/s1361-8415(98)80008-x
19. Herwig U, Schönfeldt-Lecuona C, Wunderlich AP, von Tiesenhausen C, Thielscher A, Walter H, et al. The navigation of transcranial magnetic stimulation. Psychiatry Res Neuroimaging. (2001) 108:123–31. doi: 10.1016/S0925-4927(01)00121-4
20. Yang S, Xu G, Wang L, Chen Y, Wu H, Li Y, et al. 3D realistic head model simulation based on transcranial magnetic stimulation. Conf Proc IEEE Eng Med Biol Soc. (2006). p. 6469–72. doi: 10.1109/IEMBS.2006.260877
21. Zhang H, Julkunen P, Schröder A, Kelm A, Ille S, Zimmer C, et al. Short-interval intracortical facilitation improves efficacy in nTMS motor mapping of lower extremity muscle representations in patients with supra-tentorial brain tumors. Cancers. (2020) 12:3233. doi: 10.3390/cancers12113233
22. Sollmann N, Zhang H, Kelm A, Schröder A, Meyer B, Pitkänen M, et al. Paired-pulse navigated TMS is more effective than single-pulse navigated TMS for mapping upper extremity muscles in brain tumor patients. Clin Neurophysiol. (2020) 131:2887–98. doi: 10.1016/j.clinph.2020.09.025
23. McConnell KA, Nahas Z, Shastri A, Lorberbaum JP, Kozel FA, Bohning DE, et al. The transcranial magnetic stimulation motor threshold depends on the distance from coil to underlying cortex: a replication in healthy adults comparing two methods of assessing the distance to cortex. Biol Psychiatry. (2001) 49:454–9. doi: 10.1016/S0006-3223(00)01039-8
24. Sollmann N, Tanigawa N, Bulubas L, Sabih J, Zimmer C, Ringel F, et al. Clinical factors underlying the inter-individual variability of the resting motor threshold in navigated transcranial magnetic stimulation motor mapping. Brain Topogr. (2017) 30:98–121. doi: 10.1007/s10548-016-0536-9
25. Sollmann N, Bulubas L, Tanigawa N, Zimmer C, Meyer B, Krieg SM. The variability of motor evoked potential latencies in neurosurgical motor mapping by preoperative navigated transcranial magnetic stimulation. BMC Neurosci. (2017) 18:5. doi: 10.1186/s12868-016-0321-4
26. Krieg SM, Lioumis P, Mäkelä JP, Wilenius J, Karhu J, Hannula H, et al. Protocol for motor and language mapping by navigated TMS in patients and healthy volunteers; workshop report. Acta Neurochir (Wien). (2017) 159:1187–95. doi: 10.1007/s00701-017-3187-z
27. Rosenstock T, Grittner U, Acker G, Schwarzer V, Kulchytska N, Vajkoczy P, et al. Risk stratification in motor area–related glioma surgery based on navigated transcranial magnetic stimulation data. J Neurosurg. (2017) 126:1227–37. doi: 10.3171/2016.4.JNS152896
28. Picht T, Mularski S, Kuehn B, Vajkoczy P, Kombos T, Suess O. Navigated transcranial magnetic stimulation for preoperative functional diagnostics in brain tumor surgery. Oper Neurosurg. (2009) 65:ons93–9. doi: 10.1227/01.NEU.0000348009.22750.59
29. Krieg SM, Shiban E, Buchmann N, Meyer B, Ringel F. Presurgical navigated transcranial magnetic brain stimulation for recurrent gliomas in motor eloquent areas. Clin Neurophysiol. (2013) 124:522–7. doi: 10.1016/j.clinph.2012.08.011
30. Picht T, Schulz J, Vajkoczy P. The preoperative use of navigated transcranial magnetic stimulation facilitates early resection of suspected low-grade gliomas in the motor cortex. Acta Neurochir (Wien). (2013) 155:1813–21. doi: 10.1007/s00701-013-1839-1
31. Jung J, Lavrador JP, Patel S, Giamouriadis A, Lam J, Bhangoo R, et al. First United Kingdom experience of navigated transcranial magnetic stimulation in preoperative mapping of brain tumors. World Neurosurg. (2019) 122:e1578–87. doi: 10.1016/j.wneu.2018.11.114
32. Picht T, Schmidt S, Brandt S, Frey D, Hannula H, Neuvonen T, et al. Preoperative functional mapping for rolandic brain tumor surgery: comparison of navigated transcranial magnetic stimulation to direct cortical stimulation. Neurosurgery. (2011) 69:581–9. doi: 10.1227/NEU.0b013e3182181b89
33. Weiss Lucas C, Nettekoven C, Neuschmelting V, Oros-Peusquens AM, Stoffels G, Viswanathan S, et al. Invasive versus non-invasive mapping of the motor cortex. Hum Brain Mapp. (2020) 41:3970–83. doi: 10.1002/hbm.25101
34. Tarapore PE, Tate MC, Findlay AM, Honma SM, Mizuiri D, Berger SM, et al. Preoperative multimodal motor mapping: a comparison of magnetoencephalography imaging, navigated transcranial magnetic stimulation, and direct cortical stimulation: Clinical article. J Neurosurg. (2012) 117:354–62. doi: 10.3171/2012.5.JNS112124
35. Lavrador JP, Ghimire P, Brogna C, Furlanetti L, Patel S, Gullan R, et al. Pre- and intraoperative mapping for tumors in the primary motor cortex: decision-making process in surgical resection. J Neurol Surg Part Cent Eur Neurosurg. (2021) 82:333–43. doi: 10.1055/s-0040-1709729
36. Picht T, Frey D, Thieme S, Kliesch S, Vajkoczy P. Presurgical navigated TMS motor cortex mapping improves outcome in glioblastoma surgery: a controlled observational study. J Neurooncol. (2016) 126:535–43. doi: 10.1007/s11060-015-1993-9
37. Rizzo V, Terranova C, Conti A, Germanò A, Alafaci C, Raffa G, et al. Preoperative functional mapping for rolandic brain tumor surgery. Neurosci Lett. (2014) 583:136–41. doi: 10.1016/j.neulet.2014.09.017
38. Frey D, Schilt S, Strack V, Zdunczyk A, Rösler J, Niraula B, et al. Navigated transcranial magnetic stimulation improves the treatment outcome in patients with brain tumors in motor eloquent locations. Neuro-Oncol. (2014) 16:1365–72. doi: 10.1093/neuonc/nou110
39. Raffa G, Scibilia A, Conti A, Ricciardo G, Rizzo V, Morelli A, et al. The role of navigated transcranial magnetic stimulation for surgery of motor-eloquent brain tumors: a systematic review and meta-analysis. Clin Neurol Neurosurg. (2019) 180:7–17. doi: 10.1016/j.clineuro.2019.03.003
40. Frey D, Strack V, Wiener E, Jussen D, Vajkoczy P, Picht T. A new approach for corticospinal tract reconstruction based on navigated transcranial stimulation and standardized fractional anisotropy values. NeuroImage. (2012) 62:1600–9. doi: 10.1016/j.neuroimage.2012.05.059
41. Krieg SM, Buchmann NH, Gempt J, Shiban E, Meyer B, Ringel F. Diffusion tensor imaging fiber tracking using navigated brain stimulation—a feasibility study. Acta Neurochir (Wien). (2012) 154:555–63. doi: 10.1007/s00701-011-1255-3
42. Conti A, Raffa G, Granata F, Rizzo V, Germanò A, Tomasello F. Navigated transcranial magnetic stimulation for “Somatotopic” Tractography of the corticospinal tract. Oper Neurosurg. (2014) 10:542–54. doi: 10.1227/NEU.0000000000000502
43. Rosenstock T, Giampiccolo D, Schneider H, Runge SJ, Bährend I, Vajkoczy P, et al. Specific DTI seeding and diffusivity-analysis improve the quality and prognostic value of TMS-based deterministic DTI of the pyramidal tract. NeuroImage Clin. (2017) 16:276–85. doi: 10.1016/j.nicl.2017.08.010
44. Forster MT, Hoecker AC, Kang JS, Quick J, Seifert V, Hattingen E, et al. Does navigated transcranial stimulation increase the accuracy of tractography? A prospective clinical trial based on intraoperative motor evoked potential monitoring during deep brain stimulation. Neurosurgery. (2015) 76:766–76. doi: 10.1227/NEU.0000000000000715
45. Weiss Lucas C, Tursunova I, Neuschmelting V, Nettekoven C, Oros-Peusquens AM, Stoffels G, et al. Functional MRI vs. navigated TMS to optimize M1 seed volume delineation for DTI tractography. A prospective study in patients with brain tumours adjacent to the corticospinal tract. NeuroImage Clin. (2017) 13:297–309. doi: 10.1016/j.nicl.2016.11.022
46. Takakura T, Muragaki Y, Tamura M, Maruyama T, Nitta M, Niki C, et al. Navigated transcranial magnetic stimulation for glioma removal: prognostic value in motor function recovery from postsurgical neurological deficits. J Neurosurg. (2017) 127:877–91. doi: 10.3171/2016.8.JNS16442
47. Krieg SM, Sabih J, Bulubasova L, Obermueller T, Negwer C, Janssen I, et al. Preoperative motor mapping by navigated transcranial magnetic brain stimulation improves outcome for motor eloquent lesions. Neuro-Oncol. (2014) 16:1274–82. doi: 10.1093/neuonc/nou007
48. Krieg SM, Sollmann N, Obermueller T, Sabih J, Bulubas L, Negwer C, et al. Changing the clinical course of glioma patients by preoperative motor mapping with navigated transcranial magnetic brain stimulation. BMC Cancer. (2015) 15:231. doi: 10.1186/s12885-015-1258-1
49. Moser T, Bulubas L, Sabih J, Conway N, Wildschutz N, Sollmann N, et al. Resection of navigated transcranial magnetic stimulation-positive prerolandic motor areas causes permanent impairment of motor function. Neurosurgery. (2017) 81:99–110. doi: 10.1093/neuros/nyw169
50. Mirbagheri A, Schneider H, Zdunczyk A, Vajkoczy P, Picht T. NTMS mapping of non-primary motor areas in brain tumour patients and healthy volunteers. Acta Neurochir (Wien). (2020) 162:407–16. doi: 10.1007/s00701-019-04086-x
51. Sollmann N, Wildschuetz N, Kelm A, Conway N, Moser T, Bulubas L, et al. Associations between clinical outcome and navigated transcranial magnetic stimulation characteristics in patients with motor-eloquent brain lesions: a combined navigated transcranial magnetic stimulation–diffusion tensor imaging fiber tracking approach. J Neurosurg. (2018) 128:800–10. doi: 10.3171/2016.11.JNS162322
52. Rosenstock T, Häni L, Grittner U, Schlinkmann N, Ivren M, Schneider H, et al. Bicentric validation of the navigated transcranial magnetic stimulation motor risk stratification model. J Neurosurg. (2022) 136:1194–206. doi: 10.3171/2021.3.JNS2138
53. Belotti F, Tuncer MS, Rosenstock T, Ivren M, Vajkoczy P, Picht T. Predicting the extent of resection of motor-eloquent gliomas based on TMS-guided fiber tracking. Brain Sci. (2021) 11:1517. doi: 10.3390/brainsci11111517
54. Raffa G, Conti A, Scibilia A, Cardali SM, Esposito F, Angileri FF, et al. The impact of diffusion tensor imaging fiber tracking of the corticospinal tract based on navigated transcranial magnetic stimulation on surgery of motor-eloquent brain lesions. Neurosurgery. (2018) 83:768–82. doi: 10.1093/neuros/nyx554
55. Sollmann N, Zhang H, Fratini A, Wildschuetz N, Ille S, Schröder A, et al. Risk assessment by presurgical tractography using navigated TMS maps in patients with highly motor- or language-eloquent brain tumors. Cancers. (2020) 12:1264. doi: 10.3390/cancers12051264
56. Rosenstock T, Tuncer MS, Münch MR, Vajkoczy P, Picht T, Faust K. Preoperative nTMS and intraoperative neurophysiology - A comparative analysis in patients with motor-eloquent glioma. Front Oncol. (2021) 11:676626. doi: 10.3389/fonc.2021.676626
57. Ivren M, Grittner U, Khakhar R, Belotti F, Schneider H, Pöser P, et al. Comparison of anatomical-based vs. nTMS-based risk stratification model for predicting postoperative motor outcome and extent of resection in brain tumor surgery. NeuroImage Clin. (2023) 38:103436. doi: 10.1016/j.nicl.2023.103436
58. Lioumis P, Zhdanov A, Mäkelä N, Lehtinen H, Wilenius J, Neuvonen T, et al. A novel approach for documenting naming errors induced by navigated transcranial magnetic stimulation. J Neurosci Methods. (2012) 204:349–54. doi: 10.1016/j.jneumeth.2011.11.003
59. George MS, Lisanby SH, Sackeim HA. Transcranial magnetic stimulation: applications in neuropsychiatry. Arch Gen Psychiatry. (1999) 56(4):300–11. doi: 10.1001/archpsyc.56.4.300
60. Nettekoven C, Pieczewski J, Neuschmelting V, Jonas K, Goldbrunner R, Grefkes C, et al. Improving the efficacy and reliability of rTMS language mapping by increasing the stimulation frequency. Hum Brain Mapp. (2021) 42:5309–21. doi: 10.1002/hbm.25619
61. Picht T, Krieg SM, Sollmann N, Rösler J, Niraula B, Neuvonen T, et al. A comparison of language mapping by preoperative navigated transcranial magnetic stimulation and direct cortical stimulation during awake surgery. Neurosurgery. (2013) 72:808–19. doi: 10.1227/NEU.0b013e3182889e01
62. Jeltema HR, Ohlerth AK, De Wit A, Wagemakers M, Rofes A, Bastiaansee R, et al. Comparing navigated transcranial magnetic stimulation mapping and “gold standard” direct cortical stimulation mapping in neurosurgery: a systematic review. Neurosurg Rev. (2021) 44:1903–20. doi: 10.1007/s10143-020-01397-x
63. Tarapore PE, Findlay AM, Honma SM, Mizuiri D, Houde JF, Berger MS, et al. Language mapping with navigated repetitive TMS: Proof of technique and validation. NeuroImage. (2013) 82:260–72. doi: 10.1016/j.neuroimage.2013.05.018
64. Ille S, Schroeder A, Albers L, Kelm A, Droese D, Meyer B, et al. Non-invasive mapping for effective preoperative guidance to approach highly language-eloquent gliomas—A large scale comparative cohort study using a new classification for language eloquence. Cancers. (2021) 13:207. doi: 10.3390/cancers13020207
65. Ille S, Sollmann N, Butenschoen VM, Meyer B, Ringel F, Krieg SM. Resection of highly language-eloquent brain lesions based purely on rTMS language mapping without awake surgery. Acta Neurochir (Wien). (2016) 158:2265–75. doi: 10.1007/s00701-016-2968-0
66. Schwarzer V, Bährend I, Rosenstock T, Dreyer FR, Vajkoczy P, Picht T. Aphasia and cognitive impairment decrease the reliability of rnTMS language mapping. Acta Neurochir (Wien). (2018) 160:343–56. doi: 10.1007/s00701-017-3397-4
67. Raffa G, Conti A, Scibilia A, Sindorio C, Quattropani MC, Visocchi M, et al. Functional reconstruction of motor and language pathways based on navigated transcranial magnetic stimulation and DTI fiber tracking for the preoperative planning of low grade glioma surgery: A new tool for preservation and restoration of eloquent networks. In: Visocchi M, Mehdorn HM, Katayama Y, von Wild KRH, editors. Trends in Reconstructive Neurosurgery. Springer International Publishing (2017). p. 251–61. p. 124. doi: 10.1007/978-3-319-39546-3_37
68. Sollmann N, Negwer C, Ille S, Maurer S, Hauck T, Kirschke JS, et al. Feasibility of nTMS-based DTI fiber tracking of language pathways in neurosurgical patients using a fractional anisotropy threshold. J Neurosci Methods. (2016) 267:45–54. doi: 10.1016/j.jneumeth.2016.04.002
69. Raffa G, Quattropani MC, Scibilia A, Conti A, Angileri FF, Esposito F, et al. Surgery of language-eloquent tumors in patients not eligible for awake surgery: the impact of a protocol based on navigated transcranial magnetic stimulation on presurgical planning and language outcome, with evidence of tumor-induced intra-hemispheric plasticity. Clin Neurol Neurosurg. (2018) 168:127–39. doi: 10.1016/j.clineuro.2018.03.009
70. Sollmann N, Kelm A, Ille S, Schröder A, Zimmer C, Ringel F, et al. Setup presentation and clinical outcome analysis of treating highly language-eloquent gliomas via preoperative navigated transcranial magnetic stimulation and tractography. Neurosurg Focus. (2018) 44:E2. doi: 10.3171/2018.3.FOCUS1838
71. Reisch K, Böttcher F, Tuncer MS, Schneider H, Vajkoczy P, Picht T, et al. Tractography-based navigated TMS language mapping protocol. Front Oncol. (2022) 12:1008442. doi: 10.3389/fonc.2022.1008442
72. Silva LL, Tuncer MS, Vajkoczy P, Picht T, Rosenstock T. Distinct approaches to language pathway tractography: comparison of anatomy-based, repetitive navigated transcranial magnetic stimulation (rTMS)–based, and rTMS-enhanced diffusion tensor imaging–fiber tracking. J Neurosurg. (2022) 136:589–600. doi: 10.3171/2020.12.JNS204028
73. Fekonja L, Wang Z, Bährend I, Rosenstock T, Rösler J, Wallmeroth L, et al. Manual for clinical language tractography. Acta Neurochir (Wien). (2019) 161:1125–37. doi: 10.1007/s00701-019-03899-0
74. Logothetis NK, Pauls J, Augath M, Trinath T, Oeltermann A. Neurophysiological investigation of the basis of the fMRI signal. Nature (2001) 412(6843):150–7. doi: 10.1038/35084005
75. Ogawa S, Lee TM, Nayak AS, Glynn P. Oxygenation-sensitive contrast in magnetic resonance image of rodent brain at high magnetic fields. Magn Reson Med. (1990) 14:68–78. doi: 10.1002/mrm.1910140108
76. Wunderlich AP, Grön G, Braun V. Funktionelle MR-Bildgebung des Arbeitsgedächtnisses vor neurochirurgischen Eingriffen. Z Für Med Phys. (2007) 17:250–7. doi: 10.1016/j.zemedi.2007.06.006
77. Frahm J, Bruhn H, Merboldt KD, Hänicke W. Dynamic MR imaging of human brain oxygenation during rest and photic stimulation. J Magn Reson Imaging. (1992) 2:501–5. doi: 10.1002/jmri.1880020505
78. Yablonskiy DA, Haacke EM. Theory of NMR signal behavior in magnetically inhomogeneous tissues: The static dephasing regime. Magn Reson Med. (1994) 32:749–63. doi: 10.1002/mrm.1910320610
79. Pujol J, Conesa G, Deus J, López-Obarrio L, Isamat F, Capdevila A. Clinical application of functional magnetic resonance imaging in presurgical identification of the central sulcus. J Neurosurg. (1998) 88:863–9. doi: 10.3171/jns.1998.88.5.0863
80. Schulder M, Maldjian JA, Liu WC, Holodny AI, Kalnin AT, Mun IK, et al. Functional image—guided surgery of intracranial tumors located in or near the sensorimotor cortex. J Neurosurg. (1998) 89:412–8. doi: 10.3171/jns.1998.89.3.0412
81. Kannurpatti SS, Rypma B, Biswal BB. Prediction of Task-Related BOLD fMRI with Amplitude Signatures of Resting-State fMRI. Front Syst Neurosci. (2012) 6:7. doi: 10.3389/fnsys.2012.00007
82. Zacà D, Jovicich J, Nadar SR, Voyvodic JT, Pillai JJ. Cerebrovascular reactivity mapping in patients with low grade gliomas undergoing presurgical sensorimotor mapping with BOLD fMRI: BOLD CVR Mapping in Low Grade Gliomas. J Magn Reson Imaging. (2014) 40:383–90. doi: 10.1002/jmri.24406
83. Mahvash M, Maslehaty H, Jansen O, Mehdorn HM, Petridis AK. Functional magnetic resonance imaging of motor and language for preoperative planning of neurosurgical procedures adjacent to functional areas. Clin Neurol Neurosurg. (2014) 123:72–7. doi: 10.1016/j.clineuro.2014.05.011
84. Möller M, Freund M, Greiner C, Schwindt W, Gaus C, Heindel W. Real time fMRI: a tool for the routine presurgical localisation of the motor cortex. Eur Radiol. (2005) 15:292–5. doi: 10.1007/s00330-004-2513-z
85. Baciu M, Le Bas JF, Segebarth C, Benabid AL. Presurgical fMRI evaluation of cerebral reorganization and motor deficit in patients with tumors and vascular malformations. Eur J Radiol. (2003) 46:139–46. doi: 10.1016/S0720-048X(02)00083-9
86. Alkadhi H, Kollias SS, Crelier GR, Golay X, Hepp-Reymond MC, Valavanis A. Plasticity of the human motor cortex in patients with arteriovenous malformations: a functional MR imaging study. AJNR Am J Neuroradiol. (2000). 21(8):1423–33
87. Nelson L, Lapsiwala S, Haughton VM, Noyes J, Sadrzadeh AH, Moritz CH, et al. Preoperative mapping of the supplementary motor area in patients harboring tumors in the medial frontal lobe. J Neurosurg. (2002) 97:1108–14. doi: 10.3171/jns.2002.97.5.1108
88. Wang L, Chen D, Olson J, Ali S, Fan T, Mao H. Re-examine tumor-induced alterations in hemodynamic responses of BOLD fMRI: implications in presurgical brain mapping. Acta Radiol. (2012) 53:802–11. doi: 10.1258/ar.2012.120118
89. Hou BL, Bradbury M, Peck KK, Petrovich NM, Gutin PH, Holodny AI. Effect of brain tumor neovasculature defined by rCBV on BOLD fMRI activation volume in the primary motor cortex. NeuroImage. (2006) 32:489–97. doi: 10.1016/j.neuroimage.2006.04.188
90. Xie J, Chen XZ, Jiang T, Li SW, Li ZX, Zhang Z, et al. Preoperative blood oxygen level-dependent functional magnetic resonance imaging in patients with gliomas involving the motor cortical areas. Chin Med J (Engl). (2008) 121:631–5. doi: 10.1097/00029330-200804010-00011
91. Lu JF, Zhang H, Wu JS, Yao CJ, Zhuang DX, Qiu TM, et al. Awake” intraoperative functional MRI (ai-fMRI) for mapping the eloquent cortex: Is it possible in awake craniotomy? NeuroImage Clin. (2013) 2:132–42. doi: 10.1016/j.nicl.2012.12.002
92. Dorward NL, Alberti O, Velani B, Gerritsen FA, Harkness WF, Kitchen ND, et al. Postimaging brain distortion: magnitude, correlates, and impact on neuronavigation. J Neurosurg. (1998) 88(4):656–62. doi: 10.3171/jns.1998.88.4.0656
93. Liu YT, Yang TC, Jung SM, Lee CC. Probable pathogenesis, diagnosis, and management of untreated arteriovenous malformation with cyst formation: case report and literature review. Acta Neurol Belg. (2018) 118:603–5. doi: 10.1007/s13760-018-1011-z
94. Morrison MA, Tam F, Garavaglia MM, Here GM, Cusimano MD, Schweizer TA, et al. Sources of variation influencing concordance between functional MRI and direct cortical stimulation in brain tumor surgery. Front Neurosci. (2016) 10:461. doi: 10.3389/fnins.2016.00461
95. Coburger J, Musahl C, Henkes H, Horvath-Rizea D, Bittl M, Weissbach C, et al. Comparison of navigated transcranial magnetic stimulation and functional magnetic resonance imaging for preoperative mapping in rolandic tumor surgery. Neurosurg Rev. (2013) 36:65–76. doi: 10.1007/s10143-012-0413-2
96. Picht T, Wachter D, Mularski S, Kuehn B, Brock M, Kombos T, et al. Functional magnetic resonance imaging and cortical mapping in motor cortex tumor surgery: complementary methods. Zentralblatt Für Neurochir - Cent Eur Neurosurg. (2008) 69:1–6. doi: 10.1055/s-2007-993138
97. Qiu T, Gong F, Gong X, Wu JS, Lin CP, Biswal BB, et al. Real-time motor cortex mapping for the safe resection of glioma: an intraoperative resting-state fMRI study. Am J Neuroradiol. (2017) 38:2146–52. doi: 10.3174/ajnr.A5369
98. Fang S, Liang J, Qian T, Wang Y, Liu X, Fan X, et al. Anatomic location of tumor predicts the accuracy of motor function localization in diffuse lower-grade gliomas involving the hand knob area. Am J Neuroradiol. (2017) 38:1990–7. doi: 10.3174/ajnr.A5342
99. Forster MT, Hattingen E, Senft C, Gasser T, Seifert V, Szelényi A. Navigated transcranial magnetic stimulation and functional magnetic resonance imaging: advanced adjuncts in preoperative planning for central region tumors. Neurosurgery. (2011) 68:1317–25. doi: 10.1227/NEU.0b013e31820b528c
100. Spena G, Nava A, Cassini F, Pepoli A, Bruno M, D'Agata F, et al. Preoperative and intraoperative brain mapping for the resection of eloquent-area tumors. A prospective analysis of methodology, correlation, and usefulness based on clinical outcomes. Acta Neurochir (Wien). (2010) 152:1835–46. doi: 10.1007/s00701-010-0764-9
101. Kumar A, Chandra PS, Sharma BS, Garg A, Rath GK, Bithal PK, et al. The role of neuronavigation-guided functional MRI and diffusion tensor tractography along with cortical stimulation in patients with eloquent cortex lesions. Br J Neurosurg. (2014) 28:226–33. doi: 10.3109/02688697.2013.835370
102. Dierker D, Roland JL, Kamran M, Rutlin J, Hacker CD, Marcus DS, et al. Resting-state functional magnetic resonance imaging in presurgical functional mapping. Neuroimaging Clin N Am. (2017) 27:621–33. doi: 10.1016/j.nic.2017.06.011
103. Raichle ME. The restless brain: how intrinsic activity organizes brain function. Philos Trans R Soc B Biol Sci. (2015) 370:20140172. doi: 10.1098/rstb.2014.0172
104. Roder C, Charyasz-Leks E, Breitkopf M, Decker K, Ernemann U, Klose U, et al. Resting-state functional MRI in an intraoperative MRI setting: proof of feasibility and correlation to clinical outcome of patients. J Neurosurg. (2016) 125:401–9. doi: 10.3171/2015.7.JNS15617
105. Liouta E, Katsaros VK, Stranjalis G, Leks E, Klose U, Bisdas S. Motor and language deficits correlate with resting state functional magnetic resonance imaging networks in patients with brain tumors. J Neuroradiol. (2019) 46:199–206. doi: 10.1016/j.neurad.2018.08.002
106. Schneider FC, Pailler M, Faillenot I, Vassal F, Guyotat J, Barral FG, et al. Presurgical assessment of the sensorimotor cortex using resting-state fMRI. Am J Neuroradiol. (2016) 37:101–7. doi: 10.3174/ajnr.A4472
107. Voets NL, Plaha P, Parker Jones O, Pretorius P, Bartsch A. Presurgical localization of the primary sensorimotor cortex in gliomas: when is resting state FMRI beneficial and sufficient? Clin Neuroradiol. (2021) 31:245–56. doi: 10.1007/s00062-020-00879-1
108. Metwali H, Samii A. Seed-based connectivity analysis of resting-state fMRI in patients with brain tumors: A feasibility study. World Neurosurg. (2019) 128:e165–76. doi: 10.1016/j.wneu.2019.04.073
109. Wongsripuemtet J, Tyan AE, Carass A, Agarwal S, Gujar SK, Pillai JJ, et al. Preoperative mapping of the supplementary motor area in patients with brain tumor using resting-state fMRI with seed-based analysis. Am J Neuroradiol. (2018) 39(8):1493–8. doi: 10.3174/ajnr.A5709
110. Luckett PH, Park KY, Lee JJ, Lenze EJ, Wetherell JL, Eyler LT, et al. Data-efficient resting-state functional magnetic resonance imaging brain mapping with deep learning. J Neurosurg. (2023) 139(5):1258–69. doi: 10.3171/2023.3.JNS2314
111. Ruge MI, Victor J, Hosain S, Correa DD, Relkin NR, Tabar V, et al. Concordance between functional magnetic resonance imaging and intraoperative language mapping. Stereotact Funct Neurosurg. (1999) 72:95–102. doi: 10.1159/000029706
112. Gębska-Kośla K, Bryszewski B, Jaskólski DJ, Fortuniak J, Niewodniczy M, Stefańczyk L, et al. Reorganization of language centers in patients with brain tumors located in eloquent speech areas – A pre- and postoperative preliminary fMRI study. Neurol Neurochir Pol. (2017) 51:403–10. doi: 10.1016/j.pjnns.2017.07.010
113. Stippich C, Rapps N, Dreyhaupt J, Durst A, Kress B, Nennig E, et al. Localizing and lateralizing language in patients with brain tumors: feasibility of routine preoperative functional MR imaging in 81 consecutive patients 1. Radiology. (2007) 243:828–36. doi: 10.1148/radiol.2433060068
114. Bailey PD, Zacà D, Basha MM, Agarwal S, Gujar SK, Sair HI, et al. Presurgical fMRI and DTI for the prediction of perioperative motor and language deficits in primary or metastatic brain lesions: presurgical mapping prediction of perioperative deficits. J Neuroimaging. (2015) 25:776–84. doi: 10.1111/jon.12273
115. Peck KK, Bradbury M, Petrovich N, Hou BL, Ishill N, Brennan C, et al. Presurgical evaluation of language using functional magnetic resonance imaging in brain tumor patients with previous surgery. Neurosurgery. (2009) 64:644–53. doi: 10.1227/01.NEU.0000339122.01957.0A
116. Kundu B, Penwarden A, Wood JM, Gallagher TA, Andreoli MJ, Voss J, et al. Association of functional magnetic resonance imaging indices with postoperative language outcomes in patients with primary brain tumors. Neurosurg Focus. (2013) 34:E6. doi: 10.3171/2013.2.FOCUS12413
117. Kuchcinski G, Mellerio C, Pallud J, Dezamis E, Turc G, Rigaux-Viodé O, et al. Three-tesla functional MR language mapping: Comparison with direct cortical stimulation in gliomas. Neurology. (2015) 84:560–8. doi: 10.1212/WNL.0000000000001226
118. Ulmer JL, Hacein-Bey L, Mathews VP, Mueller WM, DeYoe EA, Prost RW, et al. Lesion-induced pseudo-dominance at functional magnetic resonance imaging: implications for preoperative assessments. Neurosurgery. (2004) 55:569–81. doi: 10.1227/01.NEU.0000134384.94749.B2
119. Lemée J, Berro DH, Bernard F, Chinier E, Leiber LM, Menei P, et al. Resting-state functional magnetic resonance imaging versus task-based activity for language mapping and correlation with perioperative cortical mapping. Brain Behav. (2019) 9(10):e01362. doi: 10.1002/brb3.1362
120. Kumar VA, Heiba IM, Prabhu SS, Chen MM, Colen RR, Young AL, et al. The role of resting-state functional MRI for clinical preoperative language mapping. Cancer Imaging. (2020) 20:47. doi: 10.1186/s40644-020-00327-w
121. Sair HI, Yahyavi-Firouz-Abadi N, Calhoun VD, Airan RD, Agarwal S, Intrapiromkul J, et al. Presurgical brain mapping of the language network in patients with brain tumors using resting-state f MRI : Comparison with task f MRI. Hum Brain Mapp. (2016) 37:913–23. doi: 10.1002/hbm.23075
122. Cochereau J, Deverdun J, Herbet G, Charroud C, Boyer A, Moritz-Gasser S, et al. Comparison between resting state fMRI networks and responsive cortical stimulations in glioma patients: Resting State fMRI in Preoperative Mapping. Hum Brain Mapp. (2016) 37:3721–32. doi: 10.1002/hbm.23270
123. Dabiri M, Dehghani Firouzabadi F, Yang K, Barker PB, Lee RR, Yousem DM. Neuroimaging in schizophrenia: A review article. Front Neurosci. (2022) 16:1042814. doi: 10.3389/fnins.2022.1042814
124. Foldes ST, Weber DJ, Collinger JL. MEG-based neurofeedback for hand rehabilitation. J NeuroEngineering Rehabil. (2015) 12:85. doi: 10.1186/s12984-015-0076-7
125. Glover GH. Deconvolution of impulse response in event-related BOLD fMRI1. NeuroImage. (1999) 9:416–29. doi: 10.1006/nimg.1998.0419
126. Gallen CC, Schwartz BJ, Bucholz RD, Malik G, Barkley GL, Smith J, et al. Presurgical localization of functional cortex using magnetic source imaging. J Neurosurg. (1995) 82:988–94. doi: 10.3171/jns.1995.82.6.0988
127. Rezai AR, Hund M, Kronberg E, Zonenshayn M, Cappell J, Ribary U, et al. The interactive use of magnetoencephalography in stereotactic image-guided neurosurgery. Neurosurgery. (1996) 39:92–102. doi: 10.1097/00006123-199607000-00018
128. Schiffbauer H, Ferrari P, Rowley HA, Berger MS, Roberts TPL. Functional activity within brain tumors: A magnetic source imaging study. Neurosurgery. (2001) 49:1313–21. doi: 10.1097/00006123-200112000-00005
129. Zimmermann M, Rössler K, Kaltenhäuser M, Grummich P, Brandner N, Buchfelder M, et al. Comparative fMRI and MEG localization of cortical sensorimotor function: Bimodal mapping supports motor area reorganization in glioma patients. PLoS One. (2019) 14:e0213371. doi: 10.1371/journal.pone.0213371
130. Jannin P, Morandi X, Fleig OJ, Le Rumeur E, Toulouse P, Gibaud B, et al. Integration of sulcal and functional information for multimodal neuronavigation. J Neurosurg. (2002) 96:713–23. doi: 10.3171/jns.2002.96.4.0713
131. Korvenoja A, Kirveskari E, Aronen HJ, Avikainen S, Brander A, Huttunen J, et al. Sensorimotor cortex localization: comparison of magnetoencephalography, functional MR imaging, and intraoperative cortical mapping. Radiology. (2006) 241:213–22. doi: 10.1148/radiol.2411050796
132. Willemse RB, Hillebrand A, Ronner HE, Peter Vandertop W, Stam CJ. Magnetoencephalographic study of hand and foot sensorimotor organization in 325 consecutive patients evaluated for tumor or epilepsy surgery. NeuroImage Clin. (2016) 10:46–53. doi: 10.1016/j.nicl.2015.11.002
133. Schiffbauer H, Freudenstein D, Roberts TPL. Preoperative magnetic source imaging for brain tumor surgery: a quantitative comparison with intraoperative sensory and motor mapping. Neurosurg Focus. (2003) 15:10. doi: 10.3171/foc.2003.15.1.7
134. Firsching R, Bondar I, Heinze HJ, Hinrichs H, Hagner T, Heinrich J, et al. Practicability of magnetoencephalography-guided neuronavigation. Neurosurg Rev. (2002) 25:73–8. doi: 10.1007/s101430100161
135. Kirsch HE, Zhu Z, Honma S, Findlay A, Berger MS, Nagarajan SS. Predicting the location of mouth motor cortex in patients with brain tumors by using somatosensory evoked field measurements. J Neurosurg. (2007) 107:481–7. doi: 10.3171/JNS-07/09/0481
136. Steinmetz MP, Lüders J, Benzel EC. Magnetoencephalography. In: Barnett GH, editor. High-grade Gliomas: Diagnosis and Treatment. Humana Press (2007). p. 187–95. doi: 10.1007/978-1-59745-185-7_10
137. Grèzes J, Decety J. Functional anatomy of execution, mental simulation, observation, and verb generation of actions: A meta-analysis. Hum Brain Mapp. (2001) 12:1–19. doi: 10.1002/1097-0193(200101)12:1<1::AID-HBM10>3.0.CO;2-V
138. Ganslandt O, Buchfelder M, Hastreiter P, Grummich P, Fahlbusch R, Nimsky C. Magnetic source imaging supports clinical decision making in glioma patients. Clin Neurol Neurosurg. (2004) 107:20–6. doi: 10.1016/j.clineuro.2004.02.027
139. Kamada K, Todo T, Masutani Y, Aoki S, Ino K, Morita A, et al. Visualization of the frontotemporal language fibers by tractography combined with functional magnetic resonance imaging and magnetoencephalography. J Neurosurg. (2007) 106:90–8. doi: 10.3171/jns.2007.106.1.90
140. Grummich P, Nimsky C, Pauli E, Buchfelder M, Ganslandt O. Combining fMRI and MEG increases the reliability of presurgical language localization: A clinical study on the difference between and congruence of both modalities. NeuroImage. (2006) 32:1793–803. doi: 10.1016/j.neuroimage.2006.05.034
141. Szymanski MD, Perry DW, Gage NM, Rowley HA, Walker J, Berger MS, et al. Magnetic source imaging of late evoked field responses to vowels: toward an assessment of hemispheric dominance for language. J Neurosurg. (2001) 94:445–53. doi: 10.3171/jns.2001.94.3.0445
142. Qadri S, Dave H, Das R, Alick-Lindstrom S. Beyond the Wada: An updated approach to pre-surgical language and memory testing. Epilepsy Res. (2021) 174:106673. doi: 10.1016/j.eplepsyres.2021.106673
143. Doss RC, Zhang W, Risse GL, Dickens DL. Lateralizing language with magnetic source imaging: Validation based on the Wada test. Epilepsia. (2009) 50:2242–8. doi: 10.1111/j.1528-1167.2009.02242.x
144. Ota T, Kamada K, Kawai K, Yumoto M, Aoki S, Saito N. Refined analysis of complex language representations by non-invasive neuroimaging techniques. Br J Neurosurg. (2011) 25:197–202. doi: 10.3109/02688697.2010.505986
145. Roldán M, Kyriacou PA. Near-infrared spectroscopy (NIRS) in traumatic brain injury (TBI). Sensors. (2021) 21:1586. doi: 10.3390/s21051586
146. Rezaie R, Schiller KK, Embury L, Boop FA, Wheless JW, Narayana S. The clinical utility of transcranial magnetic stimulation in determining hemispheric dominance for language: A magnetoencephalography comparison study. J Clin Neurophysiol. (2020) 37:90–103. doi: 10.1097/WNP.0000000000000499
147. Krieg SM, Shiban E, Buchmann N, Gempt J, Foerschler A, Meyer B, et al. Utility of presurgical navigated transcranial magnetic brain stimulation for the resection of tumors in eloquent motor areas: Clinical article. J Neurosurg. (2012) 116:994–1001. doi: 10.3171/2011.12.JNS111524
148. Mangraviti A, Casali C, Cordella R, Legnani FG, Mattei L, Prada F, et al. Practical assessment of preoperative functional mapping techniques: navigated transcranial magnetic stimulation and functional magnetic resonance imaging. Neurol Sci. (2013) 34:1551–7. doi: 10.1007/s10072-012-1283-7
149. Münnich T, Klein J, Hattingen E, Noack A, Herrmann E, Seifert V, et al. Tractography verified by intraoperative magnetic resonance imaging and subcortical stimulation during tumor resection near the corticospinal tract. Oper Neurosurg. (2019) 16:197–210. doi: 10.1093/ons/opy062
150. Wang C, Sun L, Lichtenwalter B, Zerkle B, Okada Y. Compact, ultra-low vibration, closed-cycle helium recycler for uninterrupted operation of MEG with SQUID magnetometers. Cryogenics. (2016) 76:16–22. doi: 10.1016/j.cryogenics.2016.03.007
151. Babajani-Feremi A, Pourmotabbed H, Schraegle WA, Calley CS, Clarke DF, Papanicolaou AC. MEG language mapping using a novel automatic ECD algorithm in comparison with MNE, dSPM, and DICS beamformer. Front Neurosci. (2023) 17:1151885. doi: 10.3389/fnins.2023.1151885
152. Pitkänen M, Yazawa S, Airaksinen K, Lioumis P, Nurminen J, Pekkonen E, et al. Localization of sensorimotor cortex using navigated transcranial magnetic stimulation and magnetoencephalography. Brain Topogr. (2019) 32:873–81. doi: 10.1007/s10548-019-00716-w
153. Shams B, Wang Z, Roine T, Aydogan DB, Vajkoczy P, Lippert C, et al. Machine learning-based prediction of motor status in glioma patients using diffusion MRI metrics along the corticospinal tract. Brain Commun. (2022) 4:fcac141. doi: 10.1093/braincomms/fcac141
154. Mandonnet E, Sarubbo S, Duffau H. Proposal of an optimized strategy for intraoperative testing of speech and language during awake mapping. Neurosurg Rev. (2017) 40:29–35. doi: 10.1007/s10143-016-0723-x
155. Muir M, Patel R, Traylor J, de Almeida Bastos DC, Prinsloo S, Liu HL, et al. Validation of non-invasive language mapping modalities for eloquent tumor resection: A pilot study. Front Neurosci. (2022) 16:833073. doi: 10.3389/fnins.2022.833073
156. Motomura K, Takeuchi H, Nojima I, et al. Navigated repetitive transcranial magnetic stimulation as preoperative assessment in patients with brain tumors. Sci Rep. (2020) 10:9044. doi: 10.1038/s41598-020-65944-8
157. Bährend I, Muench MR, Schneider H, Aoki K, Chalise L, Iijima K, et al. Incidence and linguistic quality of speech errors: a comparison of preoperative transcranial magnetic stimulation and intraoperative direct cortex stimulation. J Neurosurg. (2021) 134:1409–18. doi: 10.3171/2020.3.JNS193085
158. Ille S, Sollmann N, Hauck T, Moshourab R, Dreyer FR, Vajkoczy P, et al. Impairment of preoperative language mapping by lesion location: a functional magnetic resonance imaging, navigated transcranial magnetic stimulation, and direct cortical stimulation study. J Neurosurg. (2015) 123:314–24. doi: 10.3171/2014.10.JNS141582
159. Ille S, Sollmann N, Hauck T, Maurer S, Tanigawa N, Obermueller T, et al. Combined noninvasive language mapping by navigated transcranial magnetic stimulation and functional MRI and its comparison with direct cortical stimulation. J Neurosurg. (2015) 123:212–25. doi: 10.3171/2014.9.JNS14929
160. Groppa S, Oliviero A, Eisen A, Quartarone A, Cohen LG, Mall V, et al. A practical guide to diagnostic transcranial magnetic stimulation: Report of an IFCN committee. Clin Neurophysiol. (2012) 123:858–82. doi: 10.1016/j.clinph.2012.01.010
161. Rossi S, Antal A, Bestmann S, Bikson M, Brewer C, Brockmöller J, et al. Safety and recommendations for TMS use in healthy subjects and patient populations, with updates on training, ethical and regulatory issues: Expert Guidelines. Clin Neurophysiol. (2021) 132:269–306. doi: 10.1016/j.clinph.2020.10.003
Keywords: navigated transcranial magnetic stimulation (nTMS), magnetoencephalography (MEG), fMRI, brain mapping, preoperative mapping, brain tumor surgery, motor eloquent tumors, language eloquent tumor
Citation: Leone A, Carbone F, Spetzger U, Vajkoczy P, Raffa G, Angileri F, Germanó A, Engelhardt M, Picht T, Colamaria A and Rosenstock T (2025) Preoperative mapping techniques for brain tumor surgery: a systematic review. Front. Oncol. 14:1481430. doi: 10.3389/fonc.2024.1481430
Received: 15 August 2024; Accepted: 10 December 2024;
Published: 07 January 2025.
Edited by:
Domenico La Torre, University of Catanzaro, ItalyReviewed by:
Jyrki Mäkelä, Hospital District of Helsinki and Uusimaa, FinlandTeodor Svedung Wettervik, Uppsala University, Sweden
Copyright © 2025 Leone, Carbone, Spetzger, Vajkoczy, Raffa, Angileri, Germanó, Engelhardt, Picht, Colamaria and Rosenstock. This is an open-access article distributed under the terms of the Creative Commons Attribution License (CC BY). The use, distribution or reproduction in other forums is permitted, provided the original author(s) and the copyright owner(s) are credited and that the original publication in this journal is cited, in accordance with accepted academic practice. No use, distribution or reproduction is permitted which does not comply with these terms.
*Correspondence: Tizian Rosenstock, dGl6aWFuLnJvc2Vuc3RvY2tAY2hhcml0ZS5kZQ==