- 1First Center Clinical College, Tianjin Medical University, Tianjin, China
- 2Nankai University School of Medicine, Nankai University, Tianjin, China
- 3Department of Emergency, Tianjin First Central Hospital, Tianjin, China
- 4Department of Hematology, Tianjin First Central Hospital, Tianjin, China
Introduction: CD7 chimeric antigen receptor T-cell (CAR-T cell) therapy is an emerging method for treating hematological malignancies, and is another breakthrough in CAR-T cell therapy.
Methods: This study summarizes the currently published clinical research results on CD7 CAR-T cells and evaluates the safety and effectiveness of CD7 CAR-T cell therapy.
Results: Among the 13 studies included in this study, a total of 200 patients received CD7 CAR-T cell therapy, including 88 patients who received autologous CAR-T cells, 112 patients who received donor derived CAR-T cells. 87% (80% -94%, I2=29.65%) of patients achieved complete remission. The incidence of cytokine release syndrome (CRS) was 94% (88% -98%, I2 =32.71%, p=0.12), while the incidence of severe CRS (grade ≥ 3) was 12% (5% -20%, I2=41.04%, p=0.06). As for the incidence of immune effector cell-associated neurotoxicity syndrome (ICANS), it is 4% (1% -7%, I2=0, p=0.72). Through analysis of the key clinical issues, we found that consolidation allogeneic hematopoietic stem cell transplantation (allo-HSCT) after CAR-T cell therapy can significantly improve survival and avoid recurrence. Therefore, we believe that the consolidation allo-HSCT after CD7 CAR-T cell therapy should be advocated. And patients who received CD7 CAR-T cell therapy without gene editing had significantly longer overall survival than those who received CD7 CAR-T cell therapy with gene editing. This suggests that gene edited CD7 CAR-T cells may pose some potential risks that limit the long-term survival of patients.
Conclusion: Our study confirms the efficacy and safety of CD7 CAR-T cells and provides research directions for the subsequent treatment.
Systematic Review Registration: https://www.crd.york.ac.uk/PROSPERO/display_record.php?RecordID=502896, identifier CRD42024502896.
Introduction
Acute T-lymphoblastic leukemia accounts for approximately 15% of childhood acute lymphoblastic leukemia (ALL) and 25% of adult ALL (1). T-cell lymphoblastic lymphoma (T-LBL) is diagnosed when T lymphoblastic cells present as a mass but do not infiltrate the bone marrow. The two diseases are similar, both caused by lymphoblasts. The disease molecular markers in T-LBL and T-ALL patients are basically consistent, which both have high invasiveness. The overall survival (OS) rate of pediatric T-ALL patients at 5 years is about 63% and that of adult patients is 40% to 55% (2) (3). Moreover, T-ALL recurrence is mostly early (within 2 years after diagnosis) (4). After recurrence, the prognosis of T-ALL patients is extremely poor. The OS rate in children with recurrent T-ALL is less than 25% (5). The 5-year OS rate of adult T-ALL patients is only 7% (6). The proportion of recurrent T-ALL patients achieving complete remission (CR) again is significantly reduced. At present, the treatment options for T-ALL/LBL (especially relapsing/refractory T-ALL/LBL) are relatively limited, mainly relying on high intensity chemotherapy and hematopoietic stem cell transplantation, which need to be optimized (7).
With the rise of immunotherapy in the field of tumor treatment, CAR-T cell therapy has received great attention from researchers and healthcare workers. The preparation of CAR-T cells usually requires collecting peripheral blood mononuclear cells (PBMCs) from patients or donors, selecting T cells from them, and activating T cells with CD3/CD28 magnetic beads (8). And methods such as lentivirus or electroporation are used to make the surface of T cells carry CAR structures that can recognize tumor specific targets, which makes it different from T cell receptor-T (TCR-T) cells and breaks free from major histocompatibility complex (MHC) limitations, thus allowing for broader application space (9). The single-chain variable fragment (scfv) confers CAR-T cells with precise identification capability, whereas the intracellular signaling domains can trigger CAR-T cell-induced cytotoxicity (10). In the field of B-cell malignancies, CD19 CAR-T cell therapy has achieved remarkable success and sparked the enthusiasm of researchers to explore more targets for the treatment of other hematological malignancies and solid tumors. The potential for CAR-T cell therapy to revolutionize cancer treatment is immense.
Up till now, three targets have received attention among numerous targets in hematological tumors and relevant clinical trials have been widely conducted in multiple centers. They are CD19 CAR-T cells used for the treatment of B-cell malignancies, BCMA CAR-T cells used for the treatment of multiple myeloma, and CD7 CAR-T cells used for the treatment of T-lymphocyte hematologic malignancies and some acute myeloid leukemia (AML). Following extensive research proving their efficacy and safety, the Food and Drug Administration (FDA) has now authorized CAR-T cell products that target the first two targets (11–13). Therefore, using a systematic review and meta-analysis of previous published cases, our study investigates the safety and efficacy of CD7 CAR-T cells. We included 13 clinical studies with 200 patients who received CD7 CAR-T cell products (Table 1). Among the 13 studies, a total of 10 reported relatively accurate survival times, involving 112 patients. Therefore, we conducted subgroup analyses based on 112 patients who reported survival time and evaluated the differences in OS and progression free survival (PFS) using Kaplan Meier survival curves.
During the application of CD7 CAR-T cell therapy, some issues have arisen. Researchers have proposed some potential treatment options for these issues. Firstly, the fratricide phenomenon in CD7 CAR-T cell therapy. Research has shown that more than 95% of T-lymphocyte hematologic malignancies (including T-ALL and T-LBL) express CD7 on the surface, making it a good therapeutic target (14). Meanwhile, approximately 30% of patients with acute myeloid leukemia have high expression of CD7 in their tumor cells (15). However, due to the expression of CD7 on the surface of CAR-T cells, fratricide often occurs, which affects the efficacy of CD7 CAR-T cells. In order to avoid the occurrence of self-killing behavior, researchers have proposed two types of strategies. One method is to use gene-editing techniques (including base editors) to knock out the CD7 gene of CD7 CAR-T cells so that the CD7 protein is not expressed on the cell membrane (16, 17). Another method is to reduce fratricide of CD7 CAR-T cells without gene editing techniques, such as utilizing a CD7 protein expression blocker (PEBL) and naturally selecting T cell (18). In addition, some other methods for preparing CD7 CAR-T cells have also been used in preclinical and clinical trials. Secondly, because of the unique biology of T-lymphocyte hematologic malignancies, it is hard to get a lot of healthy cells from patients. This makes choosing autologous or donor T cells even more important. At the moment, a lot of studies are using gene editing to remove the TRAC and CD52 genes from healthy donor T cells. This is done to create universal CD7 CAR-T cells that don’t have graft-versus-host disease (GVHD), which achieve good therapeutic effects (19). In addition, how to avoid recurrence in patients receiving CD7 CAR-T cell therapy is an important issue. Consolidating allo-HSCT after CD19 CAR-T cell therapy is a good strategy for prolonging patient survival and avoiding recurrence (20). We are curious whether consolidating allo-HSCT after CD7 CAR-T therapy can achieve the same good therapeutic effect as consolidating transplantation after CD19 CAR-T cell therapy.
Emerging technologies such as nanobody-derived CAR-T cells are also challenging the position of traditional scFv-derived CAR-T cells. Unlike scFv with two variable domains of variable heavy chain (VH) and variable light chain (VL), nanoantibodies derived from alpacas and sharks only have the VHH domain, which is not only small in structure but also very stable (21). Compared to traditional scFv derived CAR-T cells, CAR-T cells prepared by emerging technologies such as nanoantibodies may have more potential (Figure 1).
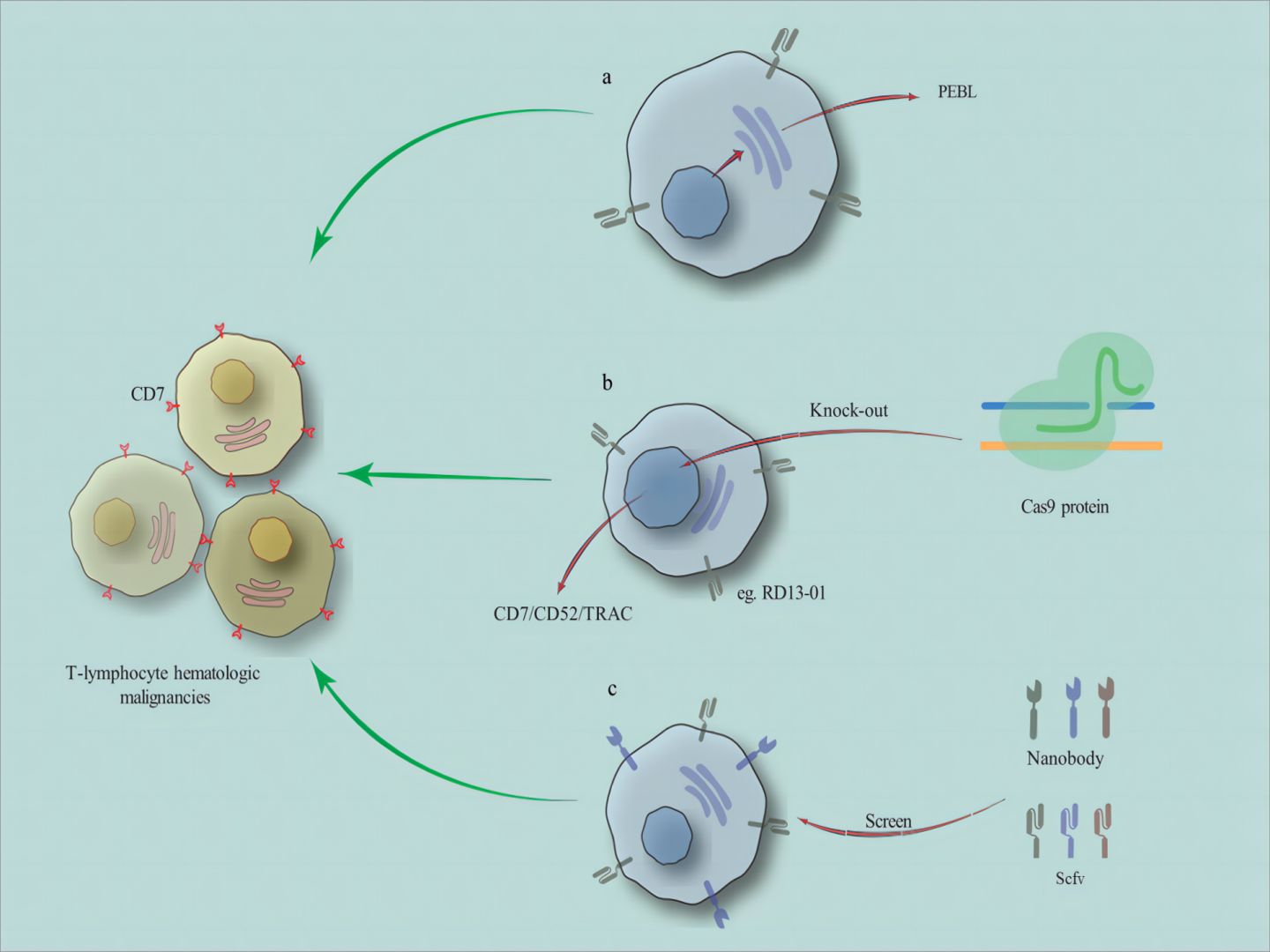
Figure 1. The related methods for preparing CD7 CAR-T cells. (A) reducing fratricide of CD7 CAR-T cells without gene editing techniques, such as utilizing a CD7 protein expression blocker (PEBL). (as well as natural selection of T cells and other methods) (B) using gene editing (eg. CRISPR-Cas9) to remove the TRAC and CD52 genes from healthy donor T cells to prepare universal CD7 CAR-T cells. (C) the CAR structures include nanobody-derived or scFv-derived.
Therefore, we also focused on exploring the clinical hotspots of CD7 CAR-T cells. Through subgroup analysis, we evaluated effectiveness of the therapy using different survival curves, including consolidating transplantation or not, gene-editing or non-gene-editing CAR-T cells, autologous or allogeneic CAR-T cells, nanobody-derived or scFv-derived CAR-T cells, providing some assistance for subsequent CD7 CAR-T therapy research.
Methods
Searching strategy and selection criteria
Our study is the first systematic review and meta-analysis of the safety and efficacy of CD7 CAR-T cell therapy for hematological malignancies in the world. The relevant clinical studies included in this study were retrieved through PubMed and conference abstracts published by the American Society of Clinical Oncology (ASCO), American Society of Hematology (ASH), and European Hematology Association (EHA). All clinical studies were registered on Clinicaltrials.gov (NCTnumber) or the Chinese Clinical Trial Registry (ChiCTRnumber). The searching keywords are “CD7”, “CD7 CAR-T”, or “CD7 chimeric antigen receptor T cells”. Due to the first public report on CD7 CAR-T cell therapy occurring in 2017, the searching time of this study was set from January 1, 2017 to February 29, 2024. Patient data was completely extracted from publicly available clinical reports, there were no additional requirements for raw patient data for the authors of these studies. Database searching and data collection were independently conducted by two authors (Jile Liu and Yuxin An). If there is no consensus on opinions, another author (Rui Sun) will determine whether to omit or retain it. The PRISMA fow diagram in Figure 2 describes the searching strategy followed by this study.
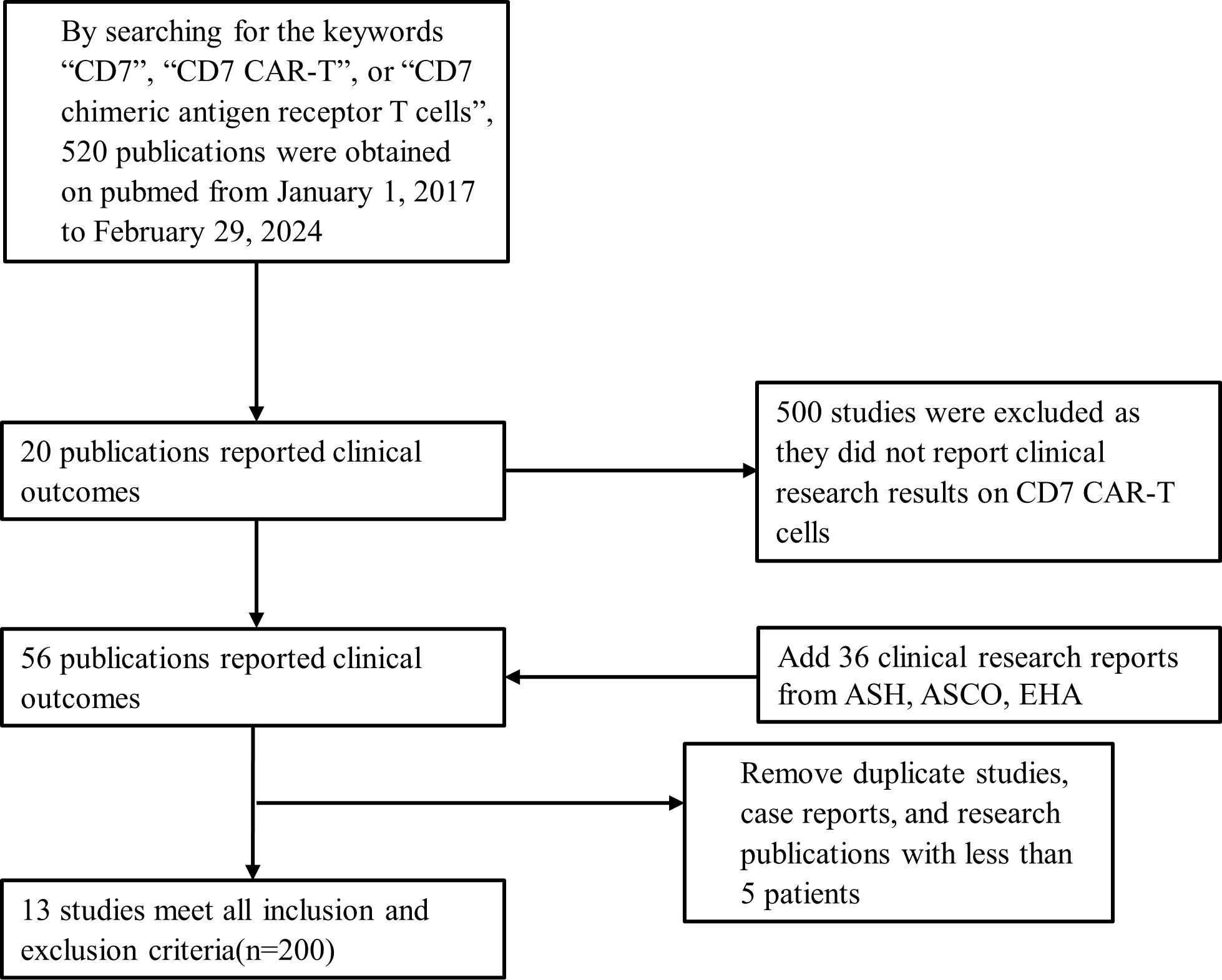
Figure 2. Searching strategy and study selection. CAR-T, chimeric antigen receptor of T cell; ASH, American Society of Hematology; ASCO, American Society of Clinical Oncology; EHA, European Hematology Association.
Data analysis
We have retrieved a total of 13 clinical research reports on the treatment of hematological malignancies with CD7 CAR-T products. Based on the clinical trial registration number, main author and affiliation, as well as CD7 CAR-T product types, independent clinical studies were identified. Also, clinical studies and case reports with duplicate data and small sample sizes were excluded. For example, clinical studies on base-edited CD7 CAR-T cells with only three cases were excluded.
We evaluated the CR, CRS, and ICANS of various studies. Due to the diversity between the studies, we adopted a random effects model. Heterogeneity was determined by the forest plots and I2. The results are reported with a 95% confidence interval (CI). Subgroup analyses were conducted to assess the differences between each group of studies. P values were calculated based on the heterogeneity between subgroups. By subgrouping whether to consolidate transplantation after infusion, gene-editing or non-gene-editing, donor or autologous derived T cells, as well as scFv-derived or nanobody-derived CAR structure, the differences in OS and PFS were evaluated using Kaplan-Meier survival curves. The forest plots are made by Stata 14.0, and the Kaplan-Meier survival curves are plotted by GraphPad Prism 8.0. This study has been registered with PROSPERO (CRD42024502896).
Results
Analysis of the efficacy and safety of CD7 CAR-T cells
A total of 13 clinical trial reports were included, involving 200 patients receiving CD7 CAR-T cell therapy. Among the 200 patients, except for 1 patient with AML and 11 patients with mixed phenotype acute leukemia (MPAL), all other patients had T lymphocyte hematological malignancy. All patients were diagnosed with recurrent/refractory hematological malignancies and received multi line treatment. All patients meet the CD7 CAR-T trial criteria. Table 1 provides a detailed presentation of the patient baseline, clinical efficacy, and side effects reported in various clinical reports. 167 patients achieved CR, with a rate of 87% (80%-94%, I2 = 29.65%, p=0.15). (Figure 3) The CR rate of T lymphocyte hematological malignancies was 83% (156/188), while the CR rate of AML and MPAL were 92% (11/12). Regarding the safety of CD7 CAR-T cells, we evaluated the common side effects of CRS and ICANS after CD7 CAR-T cell infusion. CRS, the most common adverse effect of CAR-T treatment, presents with symptoms such as fever, fatigue and anorexia. It can be assessed by detecting the level of various cytokines in the patient’s serum, including interleukin-6/10, interferon-γ and so on (22). Severe CRS (grade ≥ 3) often affect the survival and prognosis of patients. ICANS, the second most likely side effect after CAR-T cell therapy, is characterized by aphasia, changes in consciousness levels, impaired cognitive function, epilepsy, and cerebral edema (23). Among the 200 patients included in the study, the incidence of CRS was 94% (88% -98%, I2 = 32.71%, p=0.12), while the incidence of severe CRS (grade ≥ 3) was 12% (5% -20%, I2 = 41.04%, p=0.06). (Supplementary Figures 1A, B) As for the incidence of ICANS, it is 4% (1% -7%, I2 = 0, p=0.72). (Supplementary Figure 1C) Therefore, combining the above statistical data, we can conclude that CD7 CAR-T cell therapy for hematologic malignancies is a safe and effective treatment approach. Perhaps in the near future, we can choose to use CD7 CAR-T cell therapy earlier in the treatment of T lymphocyte malignancies and AML patients with high CD7 expression.
Subgroup analysis
Based on four major clinical practice controversies—including whether consolidation allo-HSCT was used after CD7 CAR-T cell therapy, whether gene-editing methods were used to make CD7 CAR-T cells, the source of T cells used to make CAR-T cells, and whether CAR-T cells were nanobody-derived or single chain variable regions (scFv)-derived—we compared OS and PFS between groups based on cases that had been published.
An increasing number of studies indicate that consolidating allo-HSCT after receiving CAR-T cell therapy is a significant therapeutic approach to prevent recurrence and prolong disease-free survival in patients (24). Our study included 27 cases of consolidation allogeneic hematopoietic stem cell transplantation after CD7 CAR-T cell therapy (25). Through subgroup analysis with non-transplant patients, it was found that those who consolidated allo-HSCT showed significant improvements in OS and PFS, suggesting that consolidating allo-HSCT after CD7 CAR-T cell therapy is an active treatment option for prolonging patient survival and consolidating therapeutic efficacy (Figures 4A, B).
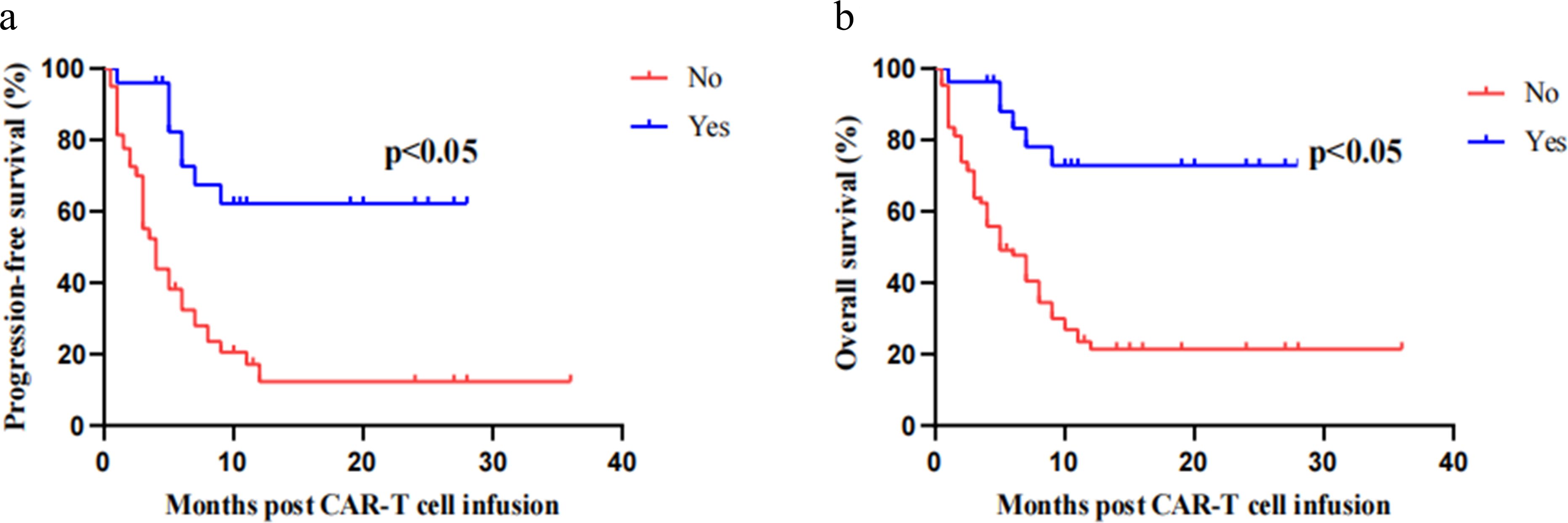
Figure 4. Kaplan–Meier progression-free survival curves and overall survival curves. Patients who consolidated allo-HSCT after CD7 CAR-T cell therapy showed significant improvements in OS (A) and PFS (B).
The application of gene-editing to avoid fratricide and GVHD is currently one of the mainstream methods for preparing CD7 CAR-T cells (17). However, genetic toxicity is an issue that cannot be ignored in gene-editing. Although there are currently no reports proving the potential threat of gene edited CAR-T cells, we still need to evaluate the safety of gene edited CD7 CAR-T cells. Therefore, we evaluated the survival of gene-edited CD7 CAR-T cells. Among the included cases, survival curve analysis was performed on the OS and PFS of 60 patients who received non-gene-edited CD7 CAR-T cell therapy and 52 patients who received gene-edited CD7 CAR-T cell therapy to determine whether there were statistical differences. The Kaplan-Meier survival curve showed that there was no statistically significant difference in PFS between patients receiving non-gene-edited CD7 CAR-T cells and gene-edited CD7 CAR-T cells. However, the OS of patients receiving non-gene-edited CD7 CAR-T cell therapy was longer than the other group, and the difference was statistically significant (P=0.016). Therefore, we believe that there may be potential threats that may occur in patients receiving gene edited CD7 CAR-T cell therapy (Supplementary Figures 2A, B).
Due to the patient’s malignant tumor of T lymphocytes, there is a risk of tumor contamination of patients’ peripheral blood T cells. Meanwhile, after multiple rounds of chemotherapy, the quality of T cells in patients generally deviates from that of healthy individuals. Therefore, in the field of CD7 CAR-T cells, it is more common to use donor and healthy human-derived T cells to prepare CD7 CAR-T cells than CD19 CAR-T cells and BCMA CAR-T cells. Therefore, based on existing data, we divided into subgroups for Kaplan Meier survival. We found that there was no statistically significant difference both OS and PFS in the existing data (Supplementary Figures 2C, D).
Research has found that compared to traditional scFv-derived CARs, nanobody-derived CARs have greater advantages (23). First, nanobody-derived CAR-T cells are not easily exhausted. Second, nanobody-derived CAR-T cells have low immunogenicity, avoiding the production of related antibodies in the body. Third, the smaller structure of CAR makes it easy to produce CAR-T and is beneficial for the preparation of dual target CAR-T cells (23). Among the 13 studies we included, only 2 clinical studies reported using nanobody-derived CD7 CAR-T cells to treat patients. A total of 15 patients received nanobody-derived CD7 CAR-T therapy, of which 14 patients achieved CR. We conducted a subgroup survival analysis and discovered no statistically significant differences in OS and PFS between patients who received scFv-derived and nanobody-derived CD7 CAR-T cells. (Supplementary Figures 2E, F).
Discussion
Our study confirms the clinical efficacy and safety of CD7 CAR-T cells in patients with hematologic malignancies through systematic review and meta-analysis. Among the 200 patients included in this study, the CR rate after CD7 CAR-T cell infusion was as high as 87%, with only an 8% and 4% probability of severe CRS and ICANS affecting prognosis. Due to the small number of patients receiving CD7 CAR-T cell therapy and a lack of specific data from various conference sources, we combined patients with T-cell malignancies and AML patients to conduct a comprehensive evaluation of the effectiveness and safety of CD7 CAR-T cells. We suggest that in the future, when more comprehensive data is available, separate evaluations should be conducted for patients with T-cell malignancies and AML patients.
At the same time, we also analyzed the issues that clinical doctors are more concerned. Through the analysis of the survival curve, consistent with the conclusion of Li Z et al. (25), consolidation allo-HSCT after CD7 CAR-T cell therapy can significantly improve OS and PFS. This conclusion is of great significance for clinical guidance. We suggest that for patients assessed as suitable for allo-HSCT after CD7 CAR-T cell infusion, allo-HSCT should be recommended to consolidate and improve treatment outcomes. This is also beneficial for the recovery of T cells in patients after CD7 CAR-T therapy.
In addition, we found that patients treated with CD7 CAR-T cells prepared using gene-editing technology had significantly lower overall survival compared to patients treated with CD7 CAR-T cells without gene-editing, although there was no significant difference in progression free survival between the two groups. We believe that this may be due to the potential genotoxic effects of gene-editing technology. For example, one of the common toxic side effect of gene-editing is the “off-target” effect. Due to the possibility of random similarity between base sequences at other positions of DNA and the target gene, if the cleavage enzyme identifies sites incorrectly, new genes may be knocked into other positions, leading to gene editing errors. It is worthy our attention. We should actively explore measures to reduce the risk and harm of genetic toxicity. The emergence of base editing technology is also worth looking forward to see whether it can reduce the risk of gene toxicity in the field of universal CAR-T cells (26). For the selection of autologous and donor T cells, we found no statistically significant difference in OS and PFS between the two groups, which is consistent with the conclusion of Zhang YQ et al. Therefore, when dealing with patients who have undergone multiple rounds of chemotherapy and have poor T cell status, we can choose donor T cells to prepare CD7 CAR-T cells to improve the success rate of preparation. For the nanobody-derived CD7 CAR-T cells, survival analysis did not show any statistical differences compared to traditional CAR-T cells. Although some preliminary conclusions have been drawn from this study, the number of patient cases is still relatively small. We look forward to more research reports being published in the future to obtain more accurate results and guide clinical applications.
At present, the CAR-T immunotherapy treating T-lymphocyte hematologic malignancies field is developing rapidly. Studies have reported the activity of dual target CAR-T against T-lymphocyte hematologic malignancies, such as CD5/CD7 CAR-T cells (27). Meanwhile, in the field of basic research, there are other options for preparing CD7 CAR-T cells, such as selecting CD7 negative T cells to prepare CAR-T cells and using antibodies to reduce the occurrence of fratricide effects during in vitro preparation (28, 29). Studies have shown that approximately 30% of patients with AML have CD7 antigen expression on leukemia cells (15, 30). Lu Y et al. have validated the inhibitory effect of CD7 CAR-T cells on CD7 positive AML tumors in vitro experiments and animal models (15). Currently, there are many studies on targets of CAR-T cell therapy for patients with refractory/relapsed AML, such as CLL1, CD123 etc. (31) However, these CAR-T cells often lead to severe neutropenia, which can easily lead to infection and affect patient prognosis (15). CD7 is not expressed in normal myeloid cells, so CD7 CAR-T cells will not lead to severe neutropenia. Therefore, we suggest that for AML patients with strong positive CD7 antigen expression, treatment with CD7 CAR-T cells can be attempted. We can believe that CD7 CAR-T products play a significant role in the treatment of hematologic malignancies with high malignancy and limited treatment options.
Data availability statement
The original contributions presented in the study are included in the article/Supplementary Material. Further inquiries can be directed to the corresponding authors.
Author contributions
JL: Conceptualization, Data curation, Formal analysis, Investigation, Methodology, Writing – original draft. YA: Data curation, Formal analysis, Investigation, Methodology, Writing – original draft. RS: Data curation, Formal analysis, Validation, Writing – original draft. XZ: Data curation, Formal analysis, Writing – original draft. SG: Data curation, Formal analysis, Writing – original draft. XG: Methodology, Supervision, Validation, Writing – review & editing. MZ: Conceptualization, Funding acquisition, Resources, Software, Supervision, Validation, Writing – review & editing.
Funding
The author(s) declare financial support was received for the research, authorship, and/or publication of this article. This work was supported by grants from the General Project of the National Natural Science Foundation of China (81970180), the Science and Technology Project of Tianjin Municipal Health Committee (TJWJ2022QN030), the Key projects of Tianjin Applied Basic Research and Multi-Investment Fund (21JCZDJC01240), the Science and Technology Project of Tianjin Municipal Health Committee (TJWJ2022XK018), Tianjin Key Medical Discipline (Specialty) Construction Project (TJYXZDXK-056B), Tianjin Municipal Natural Science Foundation (22JCQNJC00820), Tianjin Municipal Science and Technology Commission Grant (21JCQNJC00070), Tianjin Health Research Project (TJWJ2023QN027), Tianjin Health Bureau Project (ZC20074), and Tianjin Key Medical Discipline(Specialty) Construction Project (TJWJ2023XK010).
Conflict of interest
The authors declare that the research was conducted in the absence of any commercial or financial relationships that could be construed as a potential conflict of interest.
Publisher’s note
All claims expressed in this article are solely those of the authors and do not necessarily represent those of their affiliated organizations, or those of the publisher, the editors and the reviewers. Any product that may be evaluated in this article, or claim that may be made by its manufacturer, is not guaranteed or endorsed by the publisher.
Supplementary material
The Supplementary Material for this article can be found online at: https://www.frontiersin.org/articles/10.3389/fonc.2024.1478888/full#supplementary-material
Abbreviations
CAR-T, chimeric antigen receptor T; CRS, cytokine release syndrome; ICANS, immune effector cell-associated neurotoxicity syndrome; allo-HSCT, allogeneic hematopoietic stem cell transplantation; ALL, acute lymphoblastic leukemia; T-LBL, T-cell lymphoblastic lymphoma; OS, overall survival; CR, complete response; PBMC, peripheral blood mononuclear cell; TCR, T cell receptor; MHC, major histocompatibility complex; scFv, single-chain variable fragment; AML, acute myeloid leukemia; FDA, Food and Drug Administration; PEBL, protein expression blocker; GVHD, graft versus host disease; VL, variable light chain; VH, variable heavy chain; ASCO, American Society of Clinical Oncology; ASH, American Society of Hematology; EHA, European Hematology Association; CI, confidence interval; PFS, progression-free survival; MPAL, mixed phenotype acute leukemia.
References
1. Vadillo E, Dorantes-Acosta E, Pelayo R, Schnoor M. T cell acute lymphoblastic leukemia (T-ALL): New insights into the cellular origins and infiltration mechanisms common and unique among hematologic Malignancies. Blood Rev. (2018) 32:36–51. doi: 10.1016/j.blre.2017.08.006
2. Liu XM, Chen XJ, Zou Y, Wang SC, Wang M, Zhang L, et al. Outcome of children with T cell acute lymphoblastic leukemia treated with Chinese Children Leukemia Group acute lymphoblastic leukemia (CCLG-ALL) 2008 protocol. Zhonghua Er Ke Za Zhi. (2019) 57:761–6. doi: 10.3760/cma.j.issn.0578-1310.2019.10.007
3. Marks DI, Paietta EM, Moorman AV, Richards SM, Buck G, DeWald G, et al. T-cell acute lymphoblastic leukemia in adults: clinical features, immunophenotype, cytogenetics, and outcome from the large randomized prospective trial (UKALL XII/ECOG 2993). Blood. (2009) 114:5136–45. doi: 10.1182/blood-2009-08-231217
4. Wu J, Lu AD, Zhang LP, Zuo YX, Jia YP. Study of clinical outcome and prognosis in pediatric core binding factor-acute myeloid leukemia. Zhonghua Xue Ye Xue Za Zhi. (2019) 40:52–7. doi: 10.3760/cma.j.issn.0253-2727.2019.01.010
5. Hunger SP, Raetz EA. How I treat relapsed acute lymphoblastic leukemia in the pediatric population. Blood. (2020) 136:1803–12. doi: 10.1182/blood.2019004043
6. Fielding AK, Richards SM, Chopra R, Lazarus HM, Litzow MR, Buck G, et al. Outcome of 609 adults after relapse of acute lymphoblastic leukemia (ALL); an MRC UKALL12/ECOG 2993 study. Blood. (2006), 109:944–50. doi: 10.1182/blood-2006-05-018192
7. Zhao Y, Guo R, Cao X, Zhang Y, Sun R, Lu W, et al. Role of chemokines in T-cell acute lymphoblastic Leukemia: From pathogenesis to therapeutic options. Int Immunopharmacol. (2023) 121:110396. doi: 10.1016/j.intimp.2023.110396
8. Wang H, Tsao ST, Gu M, Fu C, He F, Li X, et al. A simple and effective method to purify and activate T cells for successful generation of chimeric antigen receptor T (CAR-T) cells from patients with high monocyte count. J Transl Med. (2022) 20:608. doi: 10.1186/s12967-022-03833-6
9. Haslauer T, Greil R, Zaborsky N, Geisberger R. CAR T-cell therapy in hematological Malignancies. Int J Mol Sci. (2021) 22(16):8996. doi: 10.3390/ijms22168996
10. Baker DJ, Arany Z, Baur JA, Epstein JA, June CH. CAR T therapy beyond cancer: the evolution of a living drug. Nature. (2023) 619:707–15. doi: 10.1038/s41586-023-06243-w
11. Roex G, Timmers M, Wouters K, Campillo-Davo D, Flumens D, Schroyens W, et al. Safety and clinical efficacy of BCMA CAR-T-cell therapy in multiple myeloma. J Hematol Oncol. (2020) 13:164. doi: 10.1186/s13045-020-01001-1
12. Cook MR, Dorris CS, Makambi KH, Luo Y, Munshi PN, Donato M, et al. Toxicity and efficacy of CAR T-cell therapy in primary and secondary CNS lymphoma: a meta-analysis of 128 patients. Blood Adv. (2023) 7:32–9. doi: 10.1182/bloodadvances.2022008525
13. Grigor EJM, Fergusson D, Kekre N, Montroy J, Atkins H, Seftel MD, et al. Risks and benefits of chimeric antigen receptor T-cell (CAR-T) therapy in cancer: A systematic review and meta-analysis. Transfus Med Rev. (2019) 33:98–110. doi: 10.1016/j.tmrv.2019.01.005
14. Reinhold U, Abken H, Kukel S, Moll M, Müller R, Oltermann I, et al. CD7- T cells represent a subset of normal human blood lymphocytes. J Immunol. (1993) 150:2081–9. doi: 10.4049/jimmunol.150.5.2081
15. Lu Y, Liu Y, Wen S, Kuang N, Zhang X, Li J, et al. Naturally selected CD7 CAR-T therapy without genetic editing demonstrates significant antitumour efficacy against relapsed and refractory acute myeloid leukaemia (R/R-AML). J Transl Med. (2022) 20:600. doi: 10.1186/s12967-022-03797-7
16. Cooper ML, Choi J, Staser K, Ritchey JK, Devenport JM, Eckardt K, et al. An “off-the-shelf” fratricide-resistant CAR-T for the treatment of T cell hematologic Malignancies. Leukemia. (2018) 32:1970–83. doi: 10.1038/s41375-018-0065-5
17. Chiesa R, Georgiadis C, Syed F, Zhan H, Etuk A, Gkazi SA, et al. Base-edited CAR7 T cells for relapsed T-cell acute lymphoblastic leukemia. N Engl J Med. (2023) 389:899–910. doi: 10.1056/NEJMoa2300709
18. Pan J, Tan Y, Wang G, Deng B, Ling Z, Song W, et al. Donor-derived CD7 chimeric antigen receptor T cells for T-cell acute lymphoblastic leukemia: first-in-human, phase I trial. J Clin Oncol. (2021) 39:3340–51. doi: 10.1200/jco.21.00389
19. Hu Y, Zhou Y, Zhang M, Zhao H, Wei G, Ge W, et al. Genetically modified CD7-targeting allogeneic CAR-T cell therapy with enhanced efficacy for relapsed/refractory CD7-positive hematological Malignancies: a phase I clinical study. Cell Res. (2022) 32:995–1007. doi: 10.1038/s41422-022-00721-y
20. Huang R, Wang X, Zhang X. Unity brings strength: Combination of CAR-T cell therapy and HSCT. Cancer Lett. (2022) 549:215721. doi: 10.1016/j.canlet.2022.215721
21. Safarzadeh Kozani P, Naseri A, Mirarefin SMJ, Salem F, Nikbakht M, Evazi Bakhshi S, et al. Nanobody-based CAR-T cells for cancer immunotherapy. biomark Res. (2022) 10:24. doi: 10.1186/s40364-022-00371-7
22. Cosenza M, Sacchi S, Pozzi S. Cytokine release syndrome associated with T-cell-based therapies for hematological Malignancies: pathophysiology, clinical presentation, and treatment. Int J Mol Sci. (2021) 22(14):7652. doi: 10.3390/ijms22147652
23. Sheth VS, Gauthier J. Taming the beast: CRS and ICANS after CAR T-cell therapy for ALL. Bone Marrow Transplant. (2021) 56:552–66. doi: 10.1038/s41409-020-01134-4
24. Li Z, Yang K, Song Y, Zhao Y, Wu F, Wen X, et al. CAR-T therapy followed by allogeneic hematopoietic stem cell transplantation for refractory/relapsed acute B lymphocytic leukemia: Long-term follow-up results. Front Oncol. (2022) 12:1048296. doi: 10.3389/fonc.2022.1048296
25. Li Z, An N, Yang K, Meng F, Xu T, Peng X, et al. Donor CD7 chimeric antigen receptor T cell bridging to allogeneic hematopoietic stem cell transplantation for T cell hematologic Malignancy. Transplant Cell Ther. (2023) 29:167–73. doi: 10.1016/j.jtct.2022.11.013
26. Georgiadis C, Rasaiyaah J, Gkazi SA, Preece R, Etuk A, Christi A, et al. Base-edited CAR T cells for combinational therapy against T cell Malignancies. Leukemia. (2021) 35:3466–81. doi: 10.1038/s41375-021-01282-6
27. Dai Z, Mu W, Zhao Y, Cheng J, Lin H, Ouyang K, et al. T cells expressing CD5/CD7 bispecific chimeric antigen receptors with fully human heavy-chain-only domains mitigate tumor antigen escape. Signal Transduct Target Ther. (2022) 7:85. doi: 10.1038/s41392-022-00898-z
28. Ye J, Jia Y, Tuhin IJ, Tan J, Monty MA, Xu N, et al. Feasibility study of a novel preparation strategy for anti-CD7 CAR-T cells with a recombinant anti-CD7 blocking antibody. Mol Ther Oncolytics. (2022) 24:719–28. doi: 10.1016/j.omto.2022.02.013
29. Freiwan A, Zoine JT, Crawford JC, Vaidya A, Schattgen SA, Myers JA, et al. Engineering naturally occurring CD7- T cells for the immunotherapy of hematological Malignancies. Blood. (2022) 140:2684–96. doi: 10.1182/blood.2021015020
30. Venditti A, Del Poeta G, Buccisano F, Tamburini A, Cox-Froncillo MC, Aronica G, et al. Prognostic relevance of the expression of Tdt and CD7 in 335 cases of acute myeloid leukemia. Leukemia. (1998) 12:1056–63. doi: 10.1038/sj.leu.2401067
31. Wei W, Yang D, Chen X, Liang D, Zou L, Zhao X. Chimeric antigen receptor T-cell therapy for T-ALL and AML. Front Oncol. (2022) 12:967754. doi: 10.3389/fonc.2022.967754
32. Zhang M, Fu X, Meng H, Wang M, Wang Y, Zhang L, et al. A single-arm, open-label, pilot trial of autologous CD7-CAR-T cells for CD7 positive relapsed and refractory T-lymphoblastic leukemia/lymphoma. Blood. (2021) 138:3829–9. doi: 10.1182/blood-2021-149999
33. Zhang M, Chen D, Fu X, Meng H, Nan F, Sun Z, et al. Autologous nanobody-derived fratricide-resistant CD7-CAR T-cell therapy for patients with relapsed and refractory T-cell acute lymphoblastic leukemia/lymphoma. Clin Cancer Res. (2022) 28:2830–43. doi: 10.1158/1078-0432.Ccr-21-4097
34. Zhang X, Zhou Y, Yang J, Li J, Qiu L, Ge W, et al. A novel universal CD7-targeted CAR-T cell therapy for relapsed or refractory T-cell acute lymphoblastic leukemia and T-cell lymphoblastic lymphoma. Blood. (2022) 140:4566–7. doi: 10.1182/blood-2022-165733
35. Tan Y, Pan J, Deng B, Ling Z, Weiliang S, Tian Z, et al. Efficacy and safety of donor-derived CD7 CAR T cells for r/r T-cell acute lymphoblastic leukemia/lymphoma: interim analysis from a phase 2 trial. Blood. (2022) 140:4602–3. doi: 10.1182/blood-2022-165819
36. Li S, Wang X, Liu L, Liu J, Rao J, Yuan Z, et al. CD7 targeted “off-the-shelf” CAR-T demonstrates robust in vivo expansion and high efficacy in the treatment of patients with relapsed and refractory T cell Malignancies. Leukemia. (2023) 37:2176–86. doi: 10.1038/s41375-023-02018-4
37. Zhang X, Yang J, Li J, Qiu L, Zhang J, Lu Y, et al. Analysis of 60 patients with relapsed or refractory T-cell acute lymphoblastic leukemia and T-cell lymphoblastic lymphoma treated with CD7-targeted chimeric antigen receptor-T cell therapy. Am J Hematol. (2023) 98:1898–908. doi: 10.1002/ajh.27094
38. Tan Y, Shan L, Zhao L, Deng B, Ling Z, Zhang Y, et al. Long-term follow-up of donor-derived CD7 CAR T-cell therapy in patients with T-cell acute lymphoblastic leukemia. J Hematol Oncol. (2023) 16:34. doi: 10.1186/s13045-023-01427-3
39. Chen W, Shi H, Liu Z, Yang F, Liu J, Zhang L, et al. Single-cell transcriptomics reveals immune reconstitution in patients with R/R T-ALL/LBL treated with donor-derived CD7 CAR-T therapy. Clin Cancer Res. (2023) 29:1484–95. doi: 10.1158/1078-0432.Ccr-22-2924
40. Zhang Y, Li C, Du M, Jiang H, Luo W, Tang L, et al. Allogenic and autologous anti-CD7 CAR-T cell therapies in relapsed or refractory T-cell Malignancies. Blood Cancer J. (2023) 13:61. doi: 10.1038/s41408-023-00822-w
41. Zhang X, Yang J, Li J, Qiu L, Lu P, et al. P1389: A novel and successful cd7-targeted chimeric antigen receptor (Car)-T cell therapy for refractory/relapsed mixed phenotype acute leukemia (Mpal). Hemasphere. (2023) 7:e7872701. doi: 10.1097/01.HS9.0000972444.78727.01
42. Cheng J, Wang J, Mao X, Mu W, Sun S, Zhou X, et al. Phase 1 dose escalation study of the anti-CD7 CAR-T therapy in relapsed/refractory T-cell acute leukemia and lymphoblastic lymphoma. Blood. (2023) 142:6863–3. doi: 10.1182/blood-2023-184370
43. Ghobadi A, Aldoss I, Maude SL, Bhojwani D, Wayne AS, Bajel A, et al. Phase 1/2 dose-escalation/dose-expansion study of anti-CD7 allogeneic CAR-T cells (WU-CART-007) in relapsed or refractory (R/R) T-cell acute lymphoblastic leukemia/lymphoblastic lymphoma (T-ALL/LBL). Blood. (2023) 142:770–0. doi: 10.1182/blood-2023-178723
Keywords: CD7 CAR-T cell, chimeric antigen receptor T cells, T-lymphocyte hematologic malignancies, gene editing, allogeneic stem cell transplantation
Citation: Liu J, An Y, Sun R, Zhang X, Guo S, Gao X and Zhao M (2025) The efficacy and safety of CD7 chimeric antigen receptor T-cell therapy for hematologic malignancies: a systematic review and meta-analysis. Front. Oncol. 14:1478888. doi: 10.3389/fonc.2024.1478888
Received: 11 August 2024; Accepted: 10 December 2024;
Published: 07 January 2025.
Edited by:
Swami P Iyer, University of Texas MD Anderson Cancer Center, United StatesReviewed by:
Carlos Ramos, Baylor College of Medicine, United StatesPraveen Kumar Kaudlay Sathyanarayana, University of Birmingham, United Kingdom
Copyright © 2025 Liu, An, Sun, Zhang, Guo, Gao and Zhao. This is an open-access article distributed under the terms of the Creative Commons Attribution License (CC BY). The use, distribution or reproduction in other forums is permitted, provided the original author(s) and the copyright owner(s) are credited and that the original publication in this journal is cited, in accordance with accepted academic practice. No use, distribution or reproduction is permitted which does not comply with these terms.
*Correspondence: Mingfeng Zhao, bWluZ2Zlbmd6aGFvQHNpbmEuY29t; Xuejin Gao, a3Jpc3RhcG9lcmljQDE2My5jb20=
†These authors have contributed equally to this work and share first authorship