- 1Department of Urology, Shanghai Tenth People’s Hospital, Clinical Medical College of Nanjing Medical University, Shanghai, China
- 2Department of Urology, Shanghai Tenth People’s Hospital, School of Medicine, Tongji University, Shanghai, China
- 3Urologic Cancer Institute, School of Medicine, Tongji University, Shanghai, China
- 4Department of Breast and Thyroid Surgery, Shanghai Tenth People’s Hospital, Tongji University School of Medicine, Shanghai, China
- 5Shanghai Center of Thyroid Diseases, Shanghai Tenth People’s Hospital, Tongji University School of Medicine, Shanghai, China
Purpose: Studies have shown that gut microbiota is involved in the tumorigenesis and development of prostate cancer. We aimed to perform a comprehensive analysis of causal associations of gut microbiota, metabolites, and cytokines with prostate cancer in the Asian population.
Patients and methods: Genome-wide association study (GWAS) summary datasets were collected from the public databases. There were 418 bacterial traits, 452 metabolites, 91 cytokines, 5408 cases of prostate cancer from East Asia, and 109,347 controls included. Mendelian randomization (MR) analyses were performed to investigate their causal relationships. Sensitivity analyses were conducted to test the reliability of MR results. Furthermore, the FinnGen database was used to assess the generalizability of our findings based on Asians.
Results: There were a total of 17 bacterial traits, 28 metabolites (including 2 microbiota-associated metabolites), and 9 cytokines to be significantly associated with prostate cancer in Asians (P < 0.05). Further MR analyses of these positive results indicated that G_Ruminococcaceae UCG014/TNFSF10 axis, G_Anaerofilum/TNFRSF14 axis, G_Erysipelotrichaceae UCG003/TNFSF10 axis, and P_Proteobacteria/cholesterol axis were key signaling pathways involved in the progression of prostate cancer. Notably, G_Ruminococcaceae UCG014/TNFSF10 axis and G_Anaerofilum/TNFRSF14 axis were found to act as protective factors, while the other two signaling axes played a crucial role in promoting the progression of prostate cancer. Sensitivity analyses further confirmed the reliability of our findings. Using the European population as outcome, we further assessed the generalizability of our conclusions and found limited applicability to Europeans.
Conclusions: We found that there were causal associations of gut microbiota, metabolites, and cytokines with prostate cancer in Asians. The causal effects of gut microbiota on prostate cancer were partially mediated by metabolites and cytokines. These findings might contribute to the development of new therapeutic strategies for prostate cancer.
Introduction
Prostate cancer is one of the most common malignancies among men. The Global Cancer Statistics 2020 indicated that there were approximately 1,414,259 new cases of prostate cancer, which were second in frequency, after lung cancer, for men worldwide (1). Patients with early-stage prostate cancer are usually associated with an overall favorable prognosis, while those with advanced prostate cancer exhibit worse clinical outcomes (2, 3). Although androgen deprivation therapy (ADT), the most classic treatment, showed promising therapeutic effects for prostate cancer, 10%-20% of cases can progress into castration-resistant prostate cancer (CRPC) within five years (4). Therefore, there is an urgent need for in-depth understanding the mechanisms of tumorigenesis and development of prostate cancer to develop new therapeutic options.
Accumulating evidence suggests gut microbiota is involved in diverse aspects of tumorigenesis and development of malignancies, including prostate cancer (5–7). Some commensal bacteria (e.g. Ruminococcus sp. DSM_100440) mediated the process of androgen activation, eventually leading to the accelerated development of CRPC (8). Makoto et al. (9) collected swab samples (96 cases and 56 controls) and found that patients with high-risk prostate cancer possessed specific microflora. However, the close relationships between gut microbiota and prostate cancer do not always imply causal relationships. Mendelian randomization (MR) methods provide suitable tools for investigating causal relationships between gut microbiota and prostate cancer. Wang et al. (10) included 211 bacterial traits and large prostate cancer cohorts in the European population, and identified the causal relationships between gut microbiota and prostate cancer. Similarly, Wei et al. (7) also performed the MR analyses using the 211 bacterial traits and genome-wide association study (GWAS) data of prostate cancer, and yielded positive results. Nevertheless, compared with thousands of bacterial taxa, 211 bacterial traits seem relatively few. On the other hand, the identity of 211 bacterial traits is not established down to the species, which poses an obstacle for the accurate understanding the roles of specific bacteria. Recently, the number of bacterial traits was updated to 418 and information on the species level was also added. Therefore, we performed comprehensive MR analyses using the latest GWAS summary data on gut microbiota, metabolites, cytokines, and prostate cancer from East Asia. These would broaden the understanding of the roles of gut microbiota in prostate cancer and contribute to the development of new therapeutic strategies.
Methods
Study design
The study design was illustrated in Figure 1. The MR analysis investigated the causal effects of gut microbiota, metabolites, and cytokines on the risk of prostate cancer. Sensitivity analysis was conducted to assess MR results and ensure their reliability.
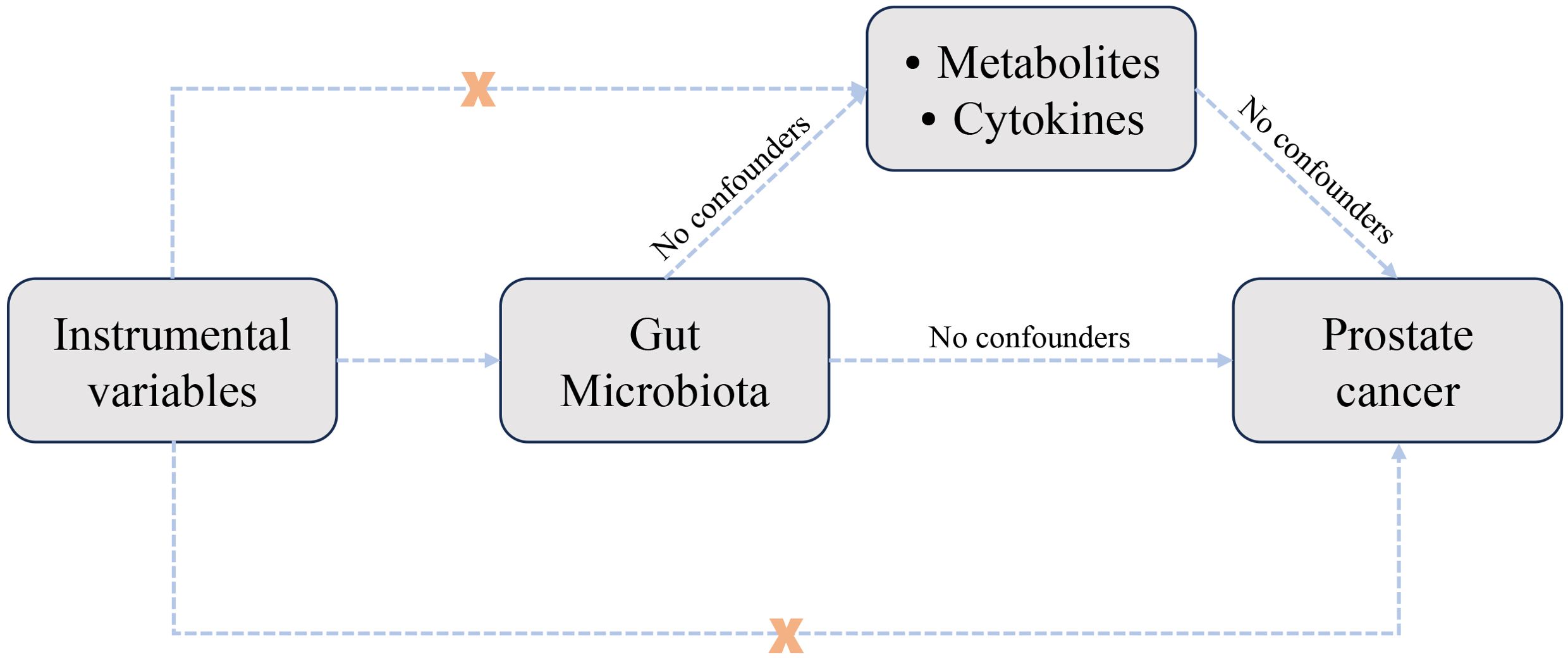
Figure 1. Study design used to investigate the causal relationships between gut microbiota, metabolites, cytokines, and prostate cancer through Mendelian randomization analysis.
Data sources
The GWAS summary datasets of gut microbiota were downloaded from MiBioGen (https://mibiogen.gcc.rug.nl), which were the most common datasets in the gut microbiota-associated MR analysis. According to taxonomic categories, there were a total of 418 bacterial traits, including 14 phylum, 26 class, 33 order, 58 family, 160 genus, and 112 species. Fifteen bacterial traits with unknown taxonomic categories were excluded. The summary datasets of metabolites were provided by Shin et al. (11). Gut microbiota-associated metabolites were identified using the Human Metabolome Database (HMDB). A total of 91 cytokines were obtained from the previous publication (12), including 11 large cohorts and 14,824 European participants.
To screen valid single nucleotide polymorphisms (SNPs), P-value of genome-wide significance was set as P < 10-5 based on the general practice of microbial consortia. To rule out the influence of linkage disequilibrium (LD), two filter conditions were set as follows: (1) the physical distance between two SNPs should be greater than 10,000 kb; (2) The statistical indicator R2 for LD should be less than 0.001. To ensure strong relationships between instrumental variables and exposure, SNPs with the F statistic greater than 10 were retained. The F statistic was calculated using the formula: F = (Beta/SE)2.
The prostate cancer coded as bbj-a-148 in the IEU OpenGWAS project (https://gwas.mrcieu.ac.uk/) was selected as outcome, which was the unique summary dataset form East Asia. Its sample size is sufficiently large to conduct a genome-wide association study, and the release time (2019) is also sufficiently new to reach a reliable conclusion. To further assess the generalizability of our findings based on Asian individuals, we also included the European population (11,590 cases and 110,189 controls) from the FinnGen database (https://storage.googleapis.com/finngen-public-data-r8/summary_stats). The above data was publicly available information and additional ethical approval was not required. Our study was approved by the Ethical Committee of Shanghai Tenth People’s Hospital.
MR analysis
Three common MR methods [MR Egger, weighted median, and inverse variance weighted (IVW)] were used to investigate the causal relationships between exposure and outcome.The primary analytical method is IVW method because it provides more accurate and reliable estimation results (13). When heterogeneity or pleiotropy exists, other MR methods were applied to correct for the bias (14). Sensitivity analysis included three primary methods: (1) Cochrans Q-statistic was applied to detect the heterogeneity; (2) MR Egger intercept test was applied to detect the pleiotropy; (3) The leave-one-out method was applied to detect the presence of outlier SNPs. “TwoSampleMR” (version 0.5.7) was the primary R package and all the statistical analyses were done in R software (version 4.3.1). P-value less than 0.05 was considered statistically significant.
Results
Causal relationships between gut microbiota and prostate cancer
The sample sizes were 1,531-14,306 and 114,755 (5408 cases and 109,347 controls) in the exposure and outcome dataset, respectively (Table 1). According to the IVW method, there were a total of 17 bacterial traits to be significantly associated with prostate cancer (Figure 2, Supplementary Figure 1, P < 0.05). Detailed list of causal relationships between each bacterial trait and prostate cancer was provided in Supplementary Table 1. Out of these 17 traits, five were negatively associated with prostate cancer, and the causal relationship between F_Peptostreptococcaceae (ebi-a-GCST90016946) and prostate cancer was further confirmed by the weighted median method (Supplementary Table 1, OR = 0.70, 95% CI = 0.51-0.98, P = 0.035). Nine bacterial traits were positively associated with prostate cancer, and three were further confirmed by the weighted median method (Supplementary Table 1), including G_Erysipelotrichaceae UCG003 (ebi-a-GCST90016994, OR = 1.81, 95% CI = 1.06-3.09, P = 0.030), G_Gordonibacter (ebi-a-GCST90027692, OR = 1.20, 95% CI = 1.01-1.42, P = 0.037), and S_Gordonibacter_pamelaeae (ebi-a-GCST90027761, OR = 1.20, 95% CI = 1.00-1.43, P = 0.044).
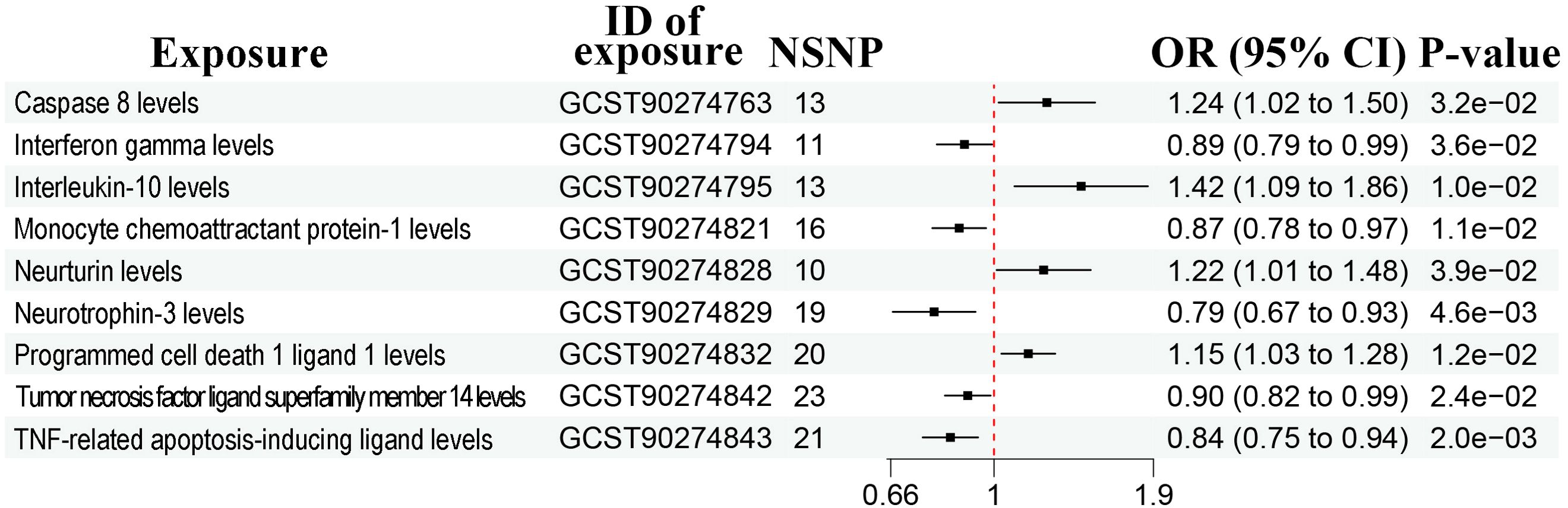
Figure 2. Forest plots showing causal relationships between gut microbiota and prostate cancer. An OR value below 1 (red dashed line) indicates a protective effect, while an OR value above 1 indicates an increased risk. NSNP, number of single nucleotide polymorphisms; OR, odds ratio; 95% CI, 95% confidence interval.
The heterogeneity test and MR-Egger regression (MR-Egger) showed no evidence of heterogeneity and pleiotropy (Supplementary Table 2, P > 0.05). The leave-one-out method suggested no outliers (Supplementary Figure 2).
Causal relationships between metabolites and prostate cancer
A total of 452 metabolites were included for MR analysis and there were 28 metabolites significantly associated with prostate cancer according to the IVW method (Figure 3, Supplementary Figure 3). Detailed list of causal relationships between each metabolite and prostate cancer was provided in Supplementary Table 3. We extracted 81 gut microbiota-associated metabolites in the Human Metabolome Database (HMDB) (Supplementary Table 4). Out of these 28 metabolites, two gut microbiota-associated metabolites (phenyllactate and serum total cholesterol) were shown to be positively associated with prostate cancer (Figure 3, phenyllactate, OR = 2.42, 95% CI = 1.26-4.66, P = 0.008; cholesterol, OR = 1.15, 95% CI = 1.02-1.30). The Q test revealed no heterogeneity (Supplementary Table 2, phenyllactate, Q = 9.640, P = 0.788; cholesterol, Q = 18.600, P = 0.885). MR-Egger regression also revealed no pleiotropy (Supplementary Table 2, phenyllactate, Egger intercept = 0.010, P = 0.592; cholesterol, Egger intercept = 0.016, P = 0.111). The leave-one-out analysis indicated no outliers (Supplementary Figure 4).
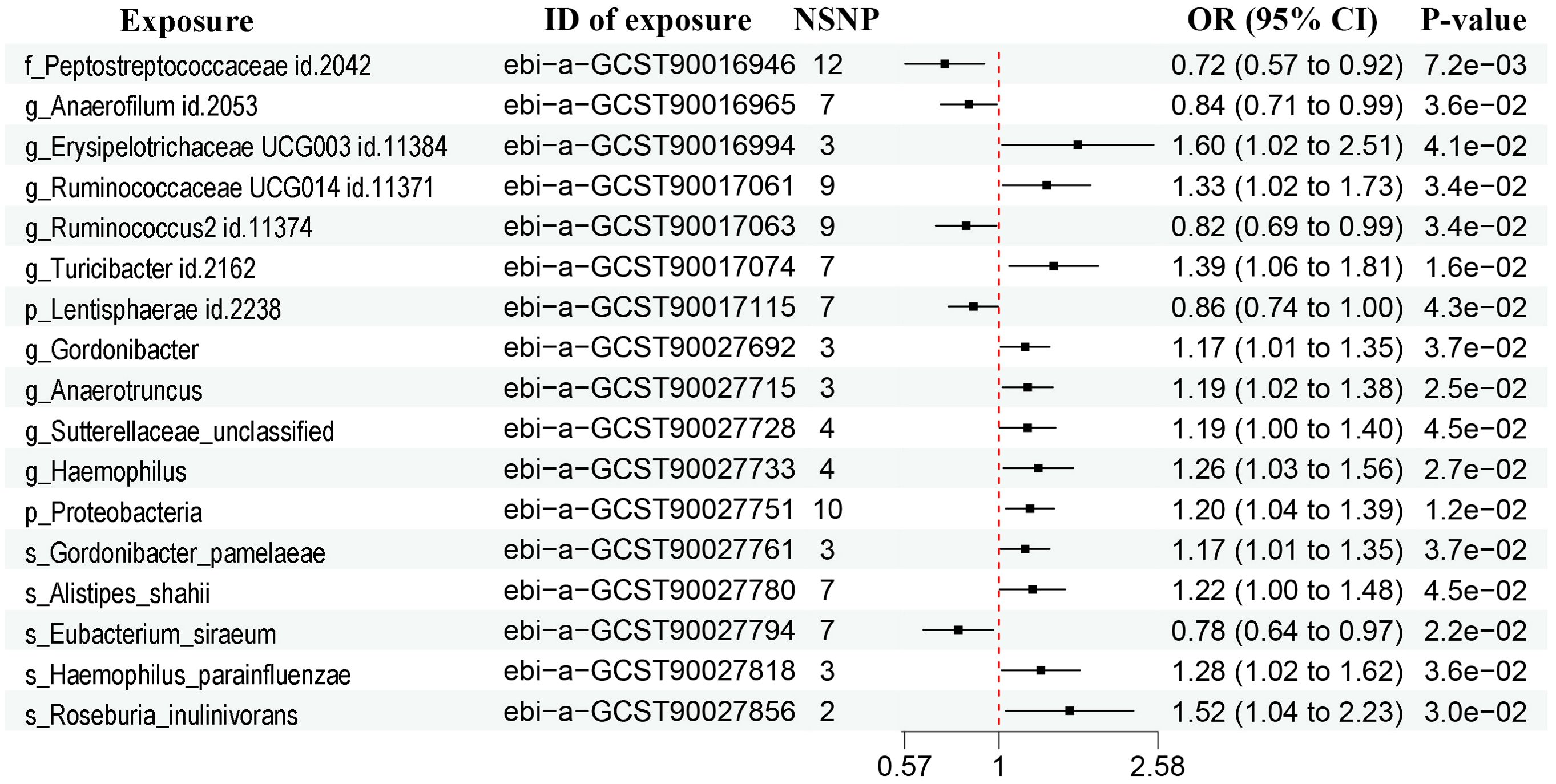
Figure 3. Forest plots showing causal relationships between metabolites and prostate cancer. An OR value below 1 (red dashed line) indicates a protective effect, while an OR value above 1 indicates an increased risk. NSNP, number of single nucleotide polymorphisms; OR, odds ratio; 95% CI, 95% confidence interval.
Causal relationships between cytokines and prostate cancer
A total of 91 cytokines were included for MR analysis and there were nine significantly associated with prostate cancer according to the IVW method (Figure 4, Supplementary Figure 5). Detailed list of causal relationships between each cytokine and prostate cancer was provided in Supplementary Table 5. Out of these 9 cytokines, four were positively associated with prostate cancer, while five were negatively associated. In particular, the causal effect of three cytokines were further confirmed by the weighted median method [Supplementary Table 5, monocyte chemoattractant protein-1 levels, OR = 0.85, 95% CI = 0.74-0.98, P = 0.027; TNF-related apoptosis-inducing ligand levels (TNFSF10), OR = 0.81, 95% CI = 0.68-0.95, P = 0.009; tumor necrosis factor ligand superfamily member 14 levels (TNFRSF14); OR = 0.87, 95% CI = 0.77-0.99, P = 0.043].
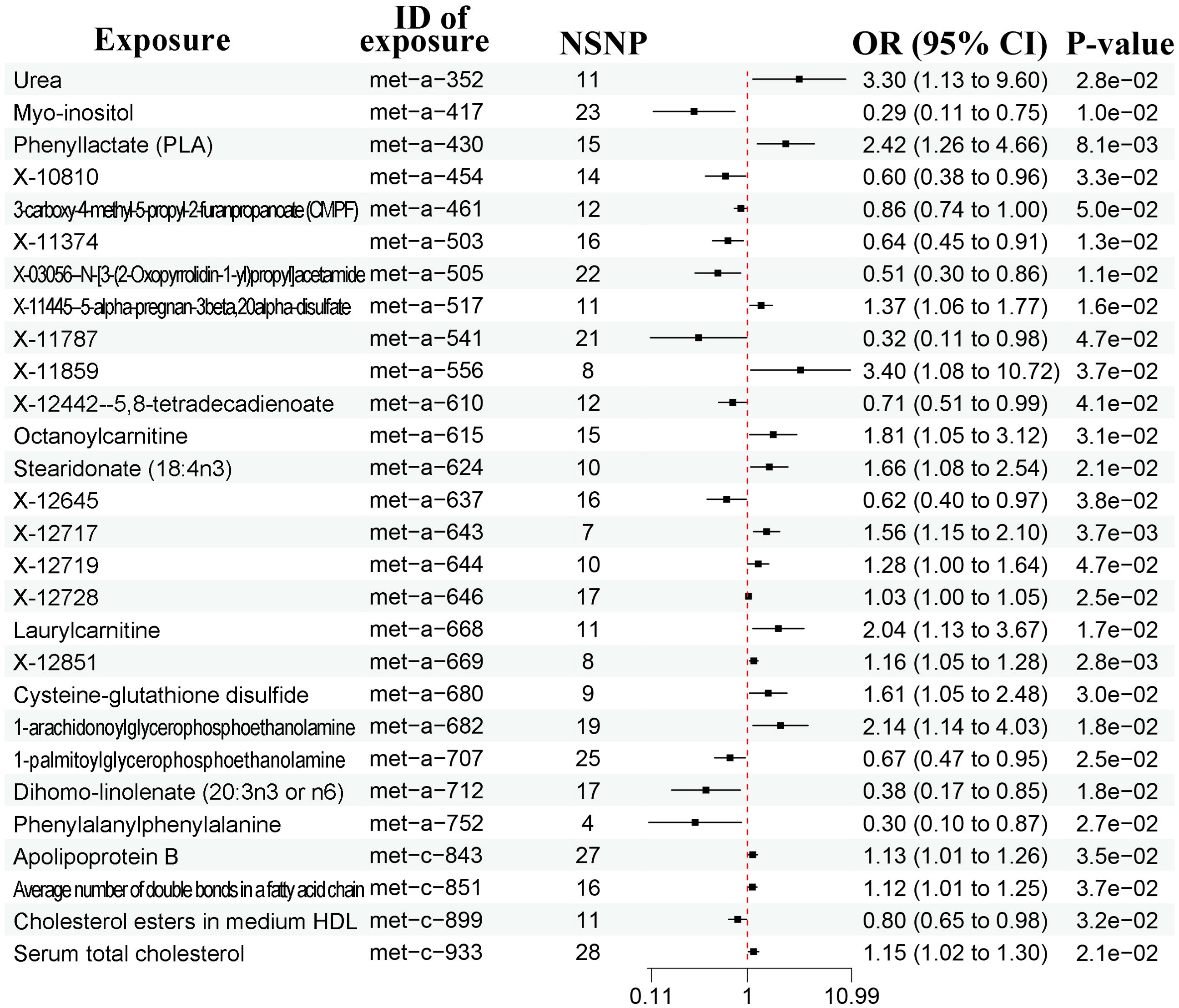
Figure 4. Forest plots showing causal relationships between cytokines and prostate cancer. An OR value below 1 (red dashed line) indicates a protective effect, while an OR value above 1 indicates an increased risk. NSNP, number of single nucleotide polymorphisms; OR, odds ratio; 95% CI, 95% confidence interval.
There were no evidence of heterogeneity and pleiotropy (Supplementary Table 2, P > 0.05). No outliers existed based on the leave-one-out method (Supplementary Figure 6).
Causal relationships between gut microbiota, metabolites, and cytokines
Considering the potential causal effects of gut microbiota on metabolites and cytokines, we included the aforementioned positive results to perform MR analyses. There were five bacterial traits significantly associated with cytokines (Table 2). However, ebi-a-GCST90027780 (S_Alistipes_shahii) was negatively associated with GCST90274763 (caspase 8 levels). Given the positive relationship between caspase 8 and prostate cancer, S_Alistipes_shahii should be associated with a decreased risk of prostate cancer, which contradicted the MR results between gut microbiota and prostate cancer (Figure 2). Therefore, S_Alistipes_shahii/caspase 8/prostate cancer may be not a reliable causal inference. The other four causal inferences were consistent with the MR results between gut microbiota and prostate cancer: G_Ruminococcus2 (ebi-a-GCST90017063)/neurturin levels (NTRN, GCST90274828)/prostate cancer, G_Anaerofilum (ebi-a-GCST90016965)/TNFRSF14 (GCST90274842)/prostate cancer, G_Ruminococcaceae UCG014 (ebi-a-GCST90017061)/TNFSF10 (GCST90274843)/prostate cancer, and G_Erysipelotrichaceae UCG003 (ebi-a-GCST90016994)/TNFSF10 (GCST90274843)/prostate cancer. Additionally, ebi-a-GCST90027751 (P_Proteobacteria) was positively with met-c-933 (serum total cholesterol), which was consistent with both the positive causal effect of cholesterol on prostate cancer and the positive causal effect of P_Proteobacteria on prostate cancer (Table 2).
Assessment of the generalizability of our conclusions
The aforementioned positive findings regarding gut microbiota, metabolites, and cytokines were treated as exposures, while European individuals were considered outcomes. We further performed MR analyses to assess the generalizability of our conclusions. As shown in Supplementary Table 6, a few gut microbiota and metabolites were significantly associated with prostate cancer in both Asian and European population.
Discussion
Using large GWAS summary datasets, we found that 17 bacterial traits, 28 metabolites, and 9 cytokines had causal relationships with prostate cancer in Asians. As is well known, gut microbiota also affects the occurrence and development of malignancies via metabolites and cytokines (15, 16). Further MR analyses indicated that G_Ruminococcus2/NTRN/prostate cancer, G_Anaerofilum/TNFRSF14/prostate cancer, G_Ruminococcaceae UCG014/TNFSF10/prostate cancer, and G_Erysipelotrichaceae UCG003/TNFSF10/prostate cancer, and P_Proteobacteria/cholesterol/prostate cancer were potential protective or risk signaling pathways. These findings suggest potential therapeutic targets for prostate cancer in Asia. However, given the significant genetic disparities in prostate cancer between Asian and European populations, the generalizability of our conclusions may be limited within Europeans. Studies have shown that Asian populations exhibit higher mutation rates of SPOP and FOXA1 in prostate cancer, while European populations are more characterized by ERG fusion mutations and PTEN loss (17). Even for the same SNP (e.g. ESR2 rs1256049), its association with prostate cancer susceptibility may exhibit opposite effects across different ethnic populations (18). These inter-ethnic genetic differences may also explain why only a small subset of gut microbiota and metabolites showed significant causal relationships with prostate cancer in both Asian and European populations according to our findings.
Both F_Peptostreptococcaceae and G_Anaerofilum were protective factors based on our analysis. These results were consistent with the study conducted by Makoto et al. (9), who identified a cluster of gut microbiota including the two bacteria negatively associated with high-risk prostate cancer. However, they showed that low-risk prostate cancer shared similar bacterial abundance with healthy control population, which may be caused by indolent biological behavior of low-risk prostate cancer (19). In another fecal microbiome analysis of 64 prostate cancer patients (20), Liss et al. reported that the abundance of Bacteroides and Streptococcus species was significantly different in prostate cancer from those without cancer, which was not in accord with our findings. A possible explanation for the inconsistency may be the small sample size. Increasing the sample size may make the findings tend to be consistent. Additionally, our analysis indicated that G_Anaerofilum may decrease the risk of prostate cancer via TNFRSF14 pathway. TNFRSF14, also called CD270 or herpes virus entry mediator (HVEM), is an immune checkpoint regulatory molecule and mainly expressed on various immune cells (21). It played a crucial role in the tumorigenesis, tumor development, and immunotherapy of a variety of malignancies, including prostate cancer (22, 23). Prostate cancer is usually considered as a “cold tumor”, which does not respond favorably to immunotherapy (24). G_Anaerofilum/TNFRSF14 axis might serve as a promising target for immunotherapy in prostate cancer.
Proteobacteria is a facultative anaerobe and generally predicts the presence of dysbiosis (25). In prostate cancer, Proteobacteria was significantly positively associated with lymph node metastasis and distant metastasis (26), which was consistent with our results of the positive causal relationship between P_Proteobacteria and prostate cancer. Our data also demonstrated that P_Proteobacteria may promote the progression of prostate cancer by regulating cholesterol metabolism. High cholesterol levels were tightly related to an increased risk of prostate cancer, which has been demonstrated by considerable epidemiological evidence (27). A randomized controlled trial showed that lifestyle factors (e.g. high-fat diet and obesity), well established risk factors for prostate cancer, might influence the progression of prostate cancer through gut microbiota including Proteobacteria (28). These findings largely supported our conclusions of the P_Proteobacteria/cholesterol/prostate cancer axis. However, more clinical and laboratory data are needed for confirmation.
There were a few limitations in the study. First, there were ethnic discrepancies between the exposure and outcome, which might bring a selection bias. However, the bias seemed clinically acceptable as our MR results were partially consistent with previous studies, including extensive 16S rRNA and metagenomic sequencing data. Second, this was short of experimental validation although we identified several signaling pathways involved in prostate cancer. Third, we performed the study using the latest and most comprehensive GWAS summary datasets of bacterial traits, metabolites, and cytokines. Nevertheless, the data depth and breadth were still relatively limited to reach more reliable and stable conclusions.
Conclusion
In summary, we found that there were causal associations of gut microbiota, metabolites, and cytokines with prostate cancer in Asians. The causal effects of gut microbiota on prostate cancer were partially mediated by metabolites and cytokines. These findings might contribute to the development of new therapeutic strategies for prostate cancer.
Data availability statement
The original contributions presented in the study are included in the article/Supplementary Material. Further inquiries can be directed to the corresponding authors.
Ethics statement
The studies involving humans were approved by the Ethical Committee of Shanghai Tenth People’s Hospital. The studies were conducted in accordance with the local legislation and institutional requirements. Written informed consent for participation was not required from the participants or the participants’ legal guardians/next of kin in accordance with the national legislation and institutional requirements.
Author contributions
ZW: Data curation, Formal analysis, Investigation, Validation, Visualization, Writing – original draft. HC: Investigation, Methodology, Resources, Software, Writing – original draft. YL: Investigation, Resources, Software, Visualization, Writing – original draft. LZ: Methodology, Software, Writing – original draft. ZZ: Data curation, Investigation, Resources, Writing – original draft. ZY: Project administration, Validation, Writing – review & editing. SM: Formal Analysis, Investigation, Supervision, Writing – original draft. CG: Data curation, Methodology, Project administration, Software, Writing – original draft. BY: Investigation, Validation, Visualization, Writing – review & editing. PW: Conceptualization, Resources, Supervision, Validation, Writing – review & editing. XY: Conceptualization, Funding acquisition, Supervision, Validation, Visualization, Writing – review & editing.
Funding
The author(s) declare financial support was received for the research, authorship, and/or publication of this article. The work was supported by National Key Research and Development Program of China (No.2022YFB3804504) and Experimental Animal Fund of Shanghai Science and Technology Commission (No.22140903800).
Conflict of interest
The authors declare that the research was conducted in the absence of any commercial or financial relationships that could be construed as a potential conflict of interest.
Correction note
A correction has been made to this article. Details can be found at: 10.3389/fonc.2025.1647090.
Publisher’s note
All claims expressed in this article are solely those of the authors and do not necessarily represent those of their affiliated organizations, or those of the publisher, the editors and the reviewers. Any product that may be evaluated in this article, or claim that may be made by its manufacturer, is not guaranteed or endorsed by the publisher.
Supplementary material
The Supplementary Material for this article can be found online at: https://www.frontiersin.org/articles/10.3389/fonc.2024.1466190/full#supplementary-material
Supplementary Figure 1 | Scatter plots showing causal relationships between gut microbiota and prostate cancer.
Supplementary Figure 2 | Leave-one-out sensitivity analysis for causal relationships between gut microbiota and prostate cancer.
Supplementary Figure 3 | Scatter plots showing causal relationships between metabolites and prostate cancer.
Supplementary Figure 4 | Leave-one-out sensitivity analysis for causal relationships between gut microbiota-associated metabolites and prostate cancer.
Supplementary Figure 5 | Scatter plots showing causal relationships between cytokines and prostate cancer.
Supplementary Figure 6 | Leave-one-out sensitivity analysis for causal relationships between cytokines and prostate cancer.
Supplementary Table 6 | Gut microbiota, metabolites, and cytokines that had significant causal relationships with prostate cancer in both Asian and European populations.
References
1. Sung H, Ferlay J, Siegel RL, Laversanne M, Soerjomataram I, Jemal A, et al. Global cancer statistics 2020: GLOBOCAN estimates of incidence and mortality worldwide for 36 cancers in 185 countries. CA Cancer J Clin. (2021) 71:209–49. doi: 10.3322/caac.21660
2. Mamouni K, Zhang S, Li X, Chen Y, Yang Y, Kim J, et al. A novel flavonoid composition targets androgen receptor signaling and inhibits prostate cancer growth in preclinical models. Neoplasia. (2018) 20:789–99. doi: 10.1016/j.neo.2018.06.003
3. Genkinger JM, Wu K, Wang M, Albanes D, Black A, van den Brandt PA, et al. Measures of body fatness and height in early and mid-to-late adulthood and prostate cancer: risk and mortality in The Pooling Project of Prospective Studies of Diet and Cancer. Ann Oncol. (2020) 31:103–14. doi: 10.1016/j.annonc.2019.09.007
4. Hooijman EL, Chalashkan Y, Ling SW, Kahyargil FF, Segbers M, Bruchertseifer F, et al. Development of [(225)Ac]Ac-PSMA-I&T for targeted alpha therapy according to GMP guidelines for treatment of mCRPC. Pharmaceutics. (2021) 13:715. doi: 10.3390/pharmaceutics13050715
5. Maddern AS, Coller JK, Bowen JM, Gibson RJ. The association between the gut microbiome and development and progression of cancer treatment adverse effects. Cancers (Basel). (2023) 15:4301. doi: 10.3390/cancers15174301
6. Sun J, Chen F, Wu G. Potential effects of gut microbiota on host cancers: focus on immunity, DNA damage, cellular pathways, and anticancer therapy. ISME J. (2023) 17:1535–51. doi: 10.1038/s41396-023-01483-0
7. Wei Z, Yang B, Tang T, Xiao Z, Ye F, Li X, et al. Gut microbiota and risk of five common cancers: A univariable and multivariable Mendelian randomization study. Cancer Med. (2023) 12:10393–405. doi: 10.1002/cam4.5772
8. Pernigoni N, Zagato E, Calcinotto A, Troiani M, Mestre RP, Cali B, et al. Commensal bacteria promote endocrine resistance in prostate cancer through androgen biosynthesis. Science. (2021) 374:216–24. doi: 10.1126/science.abf8403
9. Matsushita M, Fujita K, Motooka D, Hatano K, Fukae S, Kawamura N, et al. The gut microbiota associated with high-Gleason prostate cancer. Cancer Sci. (2021) 112:3125–35. doi: 10.1111/cas.14998
10. Mingdong W, Xiang G, Yongjun Q, Mingshuai W, Hao P. Causal associations between gut microbiota and urological tumors: a two-sample mendelian randomization study. BMC Cancer. (2023) 23:854. doi: 10.1186/s12885-023-11383-3
11. Shin SY, Fauman EB, Petersen AK, Krumsiek J, Santos R, Huang J, et al. An atlas of genetic influences on human blood metabolites. Nat Genet. (2014) 46:543–50. doi: 10.1038/ng.2982
12. Zhao JH, Stacey D, Eriksson N, Macdonald-Dunlop E, Hedman AK, Kalnapenkis A, et al. Genetics of circulating inflammatory proteins identifies drivers of immune-mediated disease risk and therapeutic targets. Nat Immunol. (2023) 24:1540–51. doi: 10.1038/s41590-023-01588-w
13. Cong R, Zhang X, Song Z, Chen S, Liu G, Liu Y, et al. Assessing the causal effects of adipokines on uric acid and gout: A two-sample mendelian randomization study. Nutrients. (2022) 14:1091. doi: 10.3390/nu14051091
14. Kamiza AB, Fatumo S, Singini MG, Yeh CC, Chikowore T. Hepatitis B infection is causally associated with extrahepatic cancers: A Mendelian randomization study. EBioMedicine. (2022) 79:104003. doi: 10.1016/j.ebiom.2022.104003
15. Hu X, Xu X, Zeng X, Jin R, Wang S, Jiang H, et al. Gut microbiota dysbiosis promotes the development of epithelial ovarian cancer via regulating Hedgehog signaling pathway. Gut Microbes. (2023) 15:2221093. doi: 10.1080/19490976.2023.2221093
16. Fong W, Li Q, Ji F, Liang W, Lau HCH, Kang X, et al. Lactobacillus gallinarum-derived metabolites boost anti-PD1 efficacy in colorectal cancer by inhibiting regulatory T cells through modulating IDO1/Kyn/AHR axis. Gut. (2023) 72:2272–85. doi: 10.1136/gutjnl-2023-329543
17. Zhu Y, Mo M, Wei Y, Wu J, Pan J, Freedland SJ, et al. Epidemiology and genomics of prostate cancer in Asian men. Nat Rev Urol. (2021) 18:282–301. doi: 10.1038/s41585-021-00442-8
18. Chang X, Wang H, Yang Z, Wang Y, Li J, Han Z. ESR2 polymorphisms on prostate cancer risk: A systematic review and meta-analysis. Med (Baltimore). (2023) 102:e33937. doi: 10.1097/MD.0000000000033937
19. Ho AS, Daskivich TJ, Sacks WL, Zumsteg ZS. Parallels between low-risk prostate cancer and thyroid cancer: A review. JAMA Oncol. (2019) 5:556–64. doi: 10.1001/jamaoncol.2018.5321
20. Liss MA, White JR, Goros M, Gelfond J, Leach R, Johnson-Pais T, et al. Metabolic biosynthesis pathways identified from fecal microbiome associated with prostate cancer. Eur Urol. (2018) 74:575–82. doi: 10.1016/j.eururo.2018.06.033
21. Steinberg MW, Cheung TC, Ware CF. The signaling networks of the herpesvirus entry mediator (TNFRSF14) in immune regulation. Immunol Rev. (2011) 244:169–87. doi: 10.1111/j.1600-065X.2011.01064.x
22. Feng D, Zhu W, Shi X, Wei W, Han P, Wei Q, et al. Leucine zipper protein 2 serves as a prognostic biomarker for prostate cancer correlating with immune infiltration and epigenetic regulation. Heliyon. (2022) 8:e10750. doi: 10.1016/j.heliyon.2022.e10750
23. Demerle C, Gorvel L, Mello M, Pastor S, Degos C, Zarubica A, et al. Anti-HVEM mAb therapy improves antitumoral immunity both in vitro and in vivo, in a novel transgenic mouse model expressing human HVEM and BTLA molecules challenged with HVEM expressing tumors. J Immunother Cancer. (2023) 11:e006348. doi: 10.1136/jitc-2022-006348
24. Qi Z, Xu Z, Zhang L, Zou Y, Li J, Yan W, et al. Overcoming resistance to immune checkpoint therapy in PTEN-null prostate cancer by intermittent anti-PI3Kalpha/beta/delta treatment. Nat Commun. (2022) 13:182. doi: 10.1038/s41467-021-27833-0
25. Shin NR, Whon TW, Bae JW. Proteobacteria: microbial signature of dysbiosis in gut microbiota. Trends Biotechnol. (2015) 33:496–503. doi: 10.1016/j.tibtech.2015.06.011
26. Zhong W, Wu K, Long Z, Zhou X, Zhong C, Wang S, et al. Gut dysbiosis promotes prostate cancer progression and docetaxel resistance via activating NF-kappaB-IL6-STAT3 axis. Microbiome. (2022) 10:94. doi: 10.1186/s40168-022-01289-w
27. Farwell WR, D’Avolio LW, Scranton RE, Lawler EV, Gaziano JM. Statins and prostate cancer diagnosis and grade in a veterans population. J Natl Cancer Inst. (2011) 103:885–92. doi: 10.1093/jnci/djr108
Keywords: prostate cancer, GWAS, gut microbiota, single nucleotide polymorphism, cytokine
Citation: Wang Z, Chen H, Liu Y, Zou L, Zhang Z, Yin Z, Mao S, Guo C, Yang B, Wu P and Yao X (2025) Gut microbiota, metabolites, and cytokines in relation to the risk of prostate cancer in the Asian population. Front. Oncol. 14:1466190. doi: 10.3389/fonc.2024.1466190
Received: 17 July 2024; Accepted: 27 December 2024;
Published: 15 January 2025; Corrected: 01 July 2025.
Edited by:
Trygve Tollefsbol, University of Alabama at Birmingham, United StatesReviewed by:
Songhe Guo, Sun Yat-sen University Cancer Center (SYSUCC), ChinaLi Zhang, Brown University, United States
Copyright © 2025 Wang, Chen, Liu, Zou, Zhang, Yin, Mao, Guo, Yang, Wu and Yao. This is an open-access article distributed under the terms of the Creative Commons Attribution License (CC BY). The use, distribution or reproduction in other forums is permitted, provided the original author(s) and the copyright owner(s) are credited and that the original publication in this journal is cited, in accordance with accepted academic practice. No use, distribution or reproduction is permitted which does not comply with these terms.
*Correspondence: Xudong Yao, eWFveHVkb25nMTk2N0AxNjMuY29t; Pengfei Wu, Z3VhbmdtaW5nd3BmQDE2My5jb20=
†These authors have contributed equally to this work