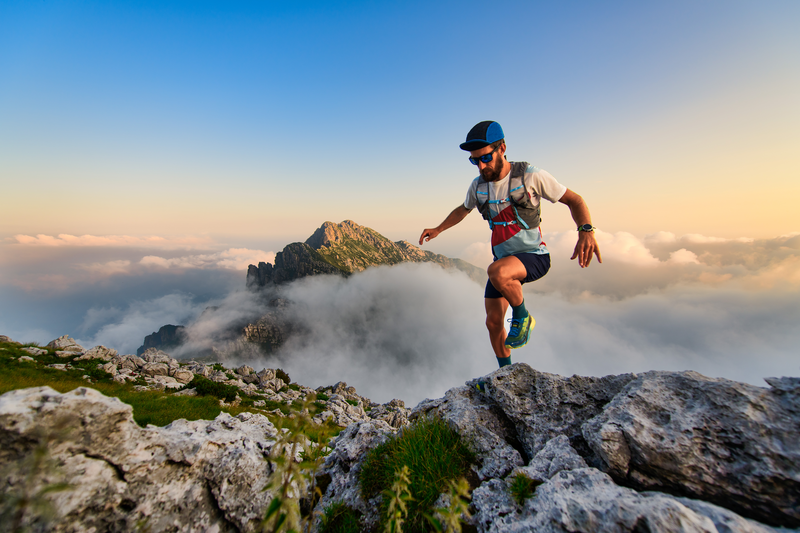
94% of researchers rate our articles as excellent or good
Learn more about the work of our research integrity team to safeguard the quality of each article we publish.
Find out more
ORIGINAL RESEARCH article
Front. Oncol. , 18 October 2024
Sec. Thoracic Oncology
Volume 14 - 2024 | https://doi.org/10.3389/fonc.2024.1462519
Background: Molecular screening using next-generation sequencing (NGS) in the pathologic evaluation of lung cancer is considered the standard in clinical practice; hence, we evaluated the diagnostic yields of various sampling methods for NGS.
Methods: NGS data from patients with lung cancer at the Pusan National University Hospital (Busan, South Korea), admitted October, 2020–April, 2023, was obtained. The sampling methods by which NGS data was obtained were divided into surgical and nonsurgical. Surgical methods included thoracoscopic surgery, surgical biopsy from the metastatic site, and lymph node excisional biopsy, whereas nonsurgical methods included bronchoscopy procedures and medical thoracoscopic biopsy.
Results: In total, we obtained 319 patients’ NGS data:150 (47.0%) and 169 (53.0%) was obtained using surgical and nonsurgical methods, respectively. The overall diagnostic yield of NGS analysis was 97.5% for all samples. There were no significant differences in the success rates of deoxyribonucleic acid sequencing between surgical and nonsurgical sampling methods (98.0% vs. 96.4%, p = 0.313). On the other hand, the success rate of ribonucleic acid (RNA) sequencing was significantly lower in the surgical method group (78.0% vs. 92.3%; p < 0.001). Multivariate analysis showed that surgical sampling significantly correlated with RNA sequencing failure (Odd Ratio 4.128, 95% Confidence Interval 1.681–10.133, p = 0.002).
Conclusions: Small samples obtained using nonsurgical procedures are suitable for NGS analysis in clinical practice. However, surgical sampling showed a relatively lower success rate for RNA sequencing than nonsurgical sampling. This information may help in the development of protocols to reduce RNA degradation during the surgical process.
Next-generation sequencing (NGS), known also as massively parallel or deep sequencing, describes a deoxyribonucleic acid (DNA)/ribonucleic acid (RNA) sequencing technology (1). NGS technologies were introduced between 2004 and 2006, transforming biomedical inquiries and resulting in a dramatic increase in sequencing data outputs (2, 3). This novel technology has revolutionized our understanding of tumor biology and consequently improved survival over the past decade (4, 5). NGS was mainly used for academic purposes at early development, however, facilitated by decreasing costs and overall increased availability, it has recently been widely implemented in the routine diagnostic workflows at many institutions (6).
NGS has proven to be a valuable strategy for improving the diagnosis, prognosis, and treatment decisions in the field of oncology, including lung cancer. NGS provides a comprehensive analysis of gene mutations, rearrangements, and amplifications in the DNA/RNA-based reactions of targeted genes (3, 5). RNA plays an essential role in numerous biological processes and RNA-based biomolecules hold promise for various applications in oncology (7). Detection of gene fusions and differential expression of known disease-causing transcripts by RNA sequencing represents some of the most immediate opportunities (7–9). Therefore, the ESMO guidelines recommend that NGS be performed on either RNA or DNA if it includes level I fusions in the panel (6). Furthermore, NGS offers information on rare mutations and co-occurring alterations previously unidentifiable through conventional testing, potentially uncovering druggable targets or providing insights into resistance mechanisms (3, 5). To date, current international guidelines strongly recommend molecular testing, including epidermal growth factor receptor (EGFR), anaplastic lymphoma kinase (ALK), kirsten rat sarcoma (KRAS), ROS Proto-Oncogene 1 (ROS1), v-Raf murine sarcoma viral oncogene homolog B1 (BRAF), neurotrophic tyrosine kinase receptor 1/2/3 (NTRK1/2/3), mesenchymal epithelial transition (MET) exon 14 skipping, rearranged during transfection proto-oncogene (RET), and Erb-B2 receptor tyrosine kinase 2 (ERBB2) (human epidermal growth factor receptor 2 (HER2)) mutations, with broader molecular profiling to identify rare driver mutations for which effective drugs may already be available (5, 10, 11).
However, the successful application of NGS technology in routine practice faces several challenges. Many technical and cost-associated considerations play a role in the decision-making processes (4). Notably, the most reliable sampling method in clinical practice and the quantity and quality of the obtained tumor tissue samples for NGS has not been widely evaluated.
Here, we report the yield of NGS analysis based on various sampling methods, and the unreliability of surgical specimens for RNA sequencing in lung cancer.
NGS data from patients with lung cancer at the Pusan National University Hospital (a university-affiliated, tertiary referral hospital in Busan, Republic of Korea), during October, 2020– April, 2023, was obtained. Some of the patients in the current study have already been included in our previous study (12). Actionable mutations in the NGS reports were defined as available or potential targets for anticancer treatment, including EGFR, ALK, ROS-1, KRAS (G12C), BRAF (V600E), NTRK, MET, RET, and ERBB2 mutations. Co-mutations were also identified in NGS reports. This study was approved by the Institutional Review Board (IRB) of Pusan National University Hospital (IRB no. 2305-028-127). This study was conducted in accordance with the principles of the Declaration of Helsinki. The requirement for informed consent was waived by the IRB of Pusan National University Hospital because of the retrospective nature of the study and because the analysis used anonymous clinical data.
The sampling methods for the NGS analysis were divided into two groups: surgical and nonsurgical. Surgical methods included thoracoscopic surgery, surgical biopsy from the metastatic site, and lymph node excisional biopsy. Nonsurgical methods included bronchoscopy procedures [transbronchial lung biopsy (TBB)], endobronchial ultrasound-guided transbronchial needle aspiration (EBUS-TBNA)) and medical thoracoscopic biopsy. The decision regarding the tumor sampling modality was based on the anatomical location of the primary lung cancer, involvement of the mediastinal lymph nodes, applicability of the procedure, and performance status of the patient.
Briefly, from our previous study (12), DNA and RNA were extracted from formalin-fixed and paraffin-embedded (FFPE) samples using a total DNA/RNA extraction kit (RecoverALL, Thermo Fisher Scientific, Waltham, MA, USA) following the manufacturer’s protocol. Targeted NGS was performed using the Oncomine Comprehensive Assay Plus (OCA-Plus, Thermo Fischer Scientific, Waltham, MA, USA). OCA-Plus covers 501 cancer-associated genes and allows the detection of single-nucleotide variants, multiple-nucleotide variants, small insertions/deletions, amplifications, and fusions. In addition, the OCA-Plus includes microsatellite instability and tumor mutational burden assays. NGS library preparation for OCA-Plus using the extracted DNA and synthesized cDNA was performed using the Ion AmpliSeq Library Preparation on the IonChef System protocol (Thermo Fisher Scientific, Waltham, MA, USA) following the manufacturer’s instructions. The deamination reaction implemented in OCA-Plus was conducted using Uracil-DNA glycosylase-heat labile (Thermo Fisher Scientific, Waltham, MA, USA) prior to polymerase chain reaction (PCR) amplification. Sequencing was performed on the IonTorrent S5 XL platform (Thermo Fisher Scientific) with an Ion 550 Chip Kit (Thermo Fisher Scientific, Waltham, MA, USA), according to the manufacturer’s instructions. RNA libraries were prepared from 20 ng RNA which were mixed with two primer pools and the AmpliSeq HiFi Master Mix and transferred to a PCR cycler (SimpliAmp™ Thermal Cycler, Life Technologies, Thermo Fisher Scientific, Waltham, MA, USA). After the end of the PCR reaction, RNA primer end sequences were partially digested using FuPa reagent, followed by the ligation of barcoded sequencing adapters (Ion Xpress Barcode Adapters; Life Technologies, Thermo Fisher Scientific, Waltham, MA, USA). The final libraries were purified using Celemics MagBeads (Celemics, Seoul, South Korea) and quantified using quantitative PCR.
Continuous variables were analyzed using Student’s t-test, and dichotomous variables were analyzed using χ2 or Fisher’s exact test. The success rate of each sampling method was analyzed using Fisher’s exact test. All p-values were two-sided, and statistical significance was set at p < 0.05. Multivariate analysis of factors related to RNA sequencing failure was performed using logistic regression. All statistical analyses were performed using the IBM SPSS Statistics Software for Windows (version 25.0; IBM Corp., Armonk, NY, USA).
A total of 319 patients were enrolled in this study. The clinical characteristics of the patients are summarized in Table 1.
The median age was 69 years (range, 39–89 years), and 209 patients (65.5%) were men. Adenocarcinoma was the most common pathological subtype (n = 212, 66.5%), followed by squamous cell carcinoma (SCC) (n = 69, 21.6%). According to the American Joint Committee on Cancer staging system 8th edition, 14.4% (n = 46) of the patients were stage I, 10.3% (n = 33) were stage II, 18.2% (n =58) were stage III, and 57.1% (n = 182) were stage IV. Patients who underwent nonsurgical methods had more non-adenocarcinoma histology (p < 0.001) and advanced stages (p < 0.001). A total of 254 samples (79.6%) were from treatment-naïve patients and 65 samples (20.4%) were from previously treated patients.
Of the 311 patients’ samples available for NGS, 148 (47.5%) harbored actionable mutations. Details of the actionable mutations reported at the time of sample collection are shown in Table 2.
The details of the sampling methods used for the 319 patients are presented in Table 3. Among the specimens, 150 (47.0%) and 169 (53.0%) were obtained using surgical and nonsurgical methods, respectively. Among all specimens, eight samples (2.5%) showed suboptimal quality for NGS analysis, resulting in an overall NGS yield of 97.5% (311/319 patients). Of these eight samples, six were obtained using nonsurgical methods (five from TBB and one from medical thoracoscopic biopsy), and two from surgical lymph node excisional biopsy. Seven of these eight samples provided sufficient materials for histological diagnosis; however, the quantity was insufficient for NGS sequencing. In one case, only necrotic material was obtained, preventing any histological diagnosis from being made.
The median yields of DNA and RNA quantities obtained from each sampling method were significantly higher in the surgical method group than in the nonsurgical method group (for DNA, median 53.6 (range, 3.76–271.00) and 40.2 (range, 3.7–148.0) ng/μL, p < 0.001) and (for RNA, median 120.0 (range, 0–788.0) and 75.8 (range, 0–994.0) ng/μL, p < 0.001). The success rates of NGS were 97.2% for DNA sequencing and 85.6% for RNA sequencing. There were no significant differences in the success rates of DNA sequencing between surgical and nonsurgical sampling methods (98.0% vs. 96.4%, p = 0.313). However, the success rate of RNA sequencing was significantly lower in the surgical method group (78.0% vs. 92.3%; p < 0.001). The NGS success rates for each method are listed in Table 3.
A total of 46 cases (14.4%) showed suboptimal RNA sequencing quality. Thirty-three samples (71.7%) were obtained using surgical methods, and 13 samples (28.9%) were obtained using nonsurgical methods. The clinical characteristics of the patients are shown in Table 4.
There were no statistically significant differences in the clinical characteristics between patients who showed RNA sequencing failure and those who did not. Univariate analysis revealed that advanced stage and surgical sampling methods were significantly correlated with the risk of RNA sequencing failure. Multivariate analysis showed that only surgical sampling methods were significantly correlated with RNA sequencing failure (Odd Ratio (OR) 4.128, 95% Confidence Interval (CI) 1.681–10.133, p = 0.002) (Table 5).
In this study, we report the diagnostic yield of NGS analysis based on various sampling methods and the NGS results in clinical practice. The overall diagnostic yield of NGS analysis was 97.5% for all the sampling methods. The success rates of DNA and RNA sequencing were 97.2% and 85.6%, respectively.
Among the nonsurgical methods, TBB was the most commonly used method for NGS (42.9%). We observed a relatively high success rate for both DNA and RNA sequencing (96.4% and 93.4%, respectively) using TBB. Furuya et al. summarized and reported the feasibility of using TBB for NGS analysis in several previous studies (13). The DNA sequencing success rate was 71.3%–100%, and the RNA sequencing success rate was 64%–84.4% (13–17). The authors explained that the use of fresh frozen tissue was one reason for the relatively high success rate compared with previous studies. Fresh frozen tissue is the preferred sample for analyzing gene mutations because of its superiority in preserving nucleic acid quality (18). However, fresh frozen tissue is often not available in clinical practice, due to a complicated protocol and relatively high cost (18, 19). In this study, we demonstrated a high success rate of NGS analysis using small FFPE samples obtained using TBB. Moreover, EBUS-TBNA showed comparably high NGS success rates (100%), despite the relatively lower extracted yield of nucleic acids. There have been concerns that EBUS-TBNA is limited in terms of the volume that can be used to obtain tumor tissue (20). However, our findings suggest that respiratory physicians could choose among several bronchoscopy procedures for NGS analysis depending on the tumor localization.
Unexpectedly, the success rate of RNA sequencing in surgical specimens was much lower than that in nonsurgical specimens, despite the larger amount of RNA extracted. Multivariate analysis also showed that the surgical method was significantly associated with RNA sequencing failure. In particular, thoracoscopic surgery specimens showed an RNA sequencing success rate of 76.4%. Several hypotheses have been proposed to explain the phenomenon. The first pertains to the tissue fixation time. Traditional methods for storing histological and cytological specimens for future use in molecular assays consist of either snap-freezing with subsequent cryopreservation, or collection in a fixative or preservation solution, most frequently in the form of FFPE, which is the standard preservation procedure for routine clinical diagnostics in pathology laboratories (19). Formalin fixation is commonly used in routine organizational examinations (21–23). Formalin is cost-effective, safe for human exposure, easy to use, and versatile, making it suitable for almost all staining purposes, except for some specific cases (21–23). As tissue permeability is approximately 1 mm/h at room temperature, the fixation duration varies depending on the type of fixative and the tissue size. Generally, small biopsy specimens require several hours to 12 hours, whereas tissues exceeding 5 mm in thickness are fixed for approximately 24 hours (23, 24). Therefore, the amount of RNA degradation may be higher in surgical specimens than in small specimens obtained using various nonsurgical methods because of the longer fixation time of the surgical specimens.
Another factor may be the effects of time delay and surgical antiseptics (24). In contrast to DNA, RNA is extremely unstable and degrades easily. Because most clinical specimens are obtained during surgical operations, doctors have limited time for protective storage of these samples. This leads to substantial degradation of RNA. Wilcox et al. have reported the effects of time delay and surgical antiseptics on RNA isolated from human and rodent peripheral nerves (25). They found that time delays between surgical liberation and cryopreservation significantly decreased RNA concentrations. In addition, the detrimental effect of antiseptic surgical reagents such as chlorhexidine and iodine on RNA yield was confirmed in a rodent model, where the RNA yield was 8.3-fold lower than that in non-exposed samples. Moreover, in this study, the time from the NGS prescription date to the start date in the surgical method group was significantly longer than that in the nonsurgical method group (median 3 days (range 0–34, vs 1 d (range 0–10), p < 0.001). These factors might cause a significantly low success rate in RNA sequencing using surgical methods. Therefore, our results suggest that samples for NGS analysis should be processed separately from the entire surgically harvested specimen. However, further studies are required to confirm this phenomenon.
This study had several limitations. First, this was a single-center study that encompassed challenges in generalization, the risk of selection bias, limited diversity, and site-specific factors, potentially compromising external validity and replicability. However, with a relatively large sample size and our institution’s status as a referral center in the region, we contend that these factors mitigate these limitations to some extent. Second, the exact fixation time for each sample is unclear. Undoubtedly, surgical specimens require more fixation time than small specimens obtained using nonsurgical methods because of their larger volume; however, the exact time for sample fixation has not been recorded. Third, we need to perform additional experimental studies are required to further investigate the effects of time delay and exposure to antiseptic agents.
In conclusion, this study reported the success rates of NGS analysis based on various sampling methods and results. Small FFPE samples obtained using bronchoscopy procedures are suitable for NGS analysis in clinical practice. These findings suggest that bronchoscopy is a reasonable diagnostic tool for NGS, particularly for patients with advanced lung cancer. However, surgical specimens showed a relatively lower success rate for RNA sequencing than nonsurgical specimens. This information may help in the development of protocols to reduce RNA degradation during the surgical process
The datasets used and/or analyzed during the current study are available from the corresponding author upon reasonable request.
The studies involving humans were approved by Institutional Review Board (IRB) of Pusan National University Hospital (IRB no. 2305-028-127). The studies were conducted in accordance with the local legislation and institutional requirements. The human samples used in this study were acquired from a by- product of routine care or industry. Written informed consent for participation was not required from the participants or the participants’ legal guardians/next of kin in accordance with the national legislation and institutional requirements.
JE: Data curation, Formal Analysis, Writing – original draft, Writing – review & editing. SK: Data curation, Formal Analysis, Writing – review & editing. KK: Data curation, Writing – review & editing, Methodology. AK: Data curation, Methodology, Writing – review & editing. HA: Data curation, Writing – review & editing. JM: Data curation, Writing – review & editing. JC: Data curation, Methodology, Writing – review & editing. ML: Supervision, Writing – review & editing. JS: Formal Analysis, Methodology, Writing – review & editing. M-HK: Conceptualization, Data curation, Formal Analysis, Methodology, Supervision, Writing – original draft, Writing – review & editing.
The author(s) declare that no financial support was received for the research, authorship, and/or publication of this article.
We would like to thank Editage (www.editage.co.kr) for English language editing.
Author JS was employed by GC Genome Corporation.
The remaining authors declare that the research was conducted in the absence of any commercial or financial relationships that could be construed as a potential conflict of interest.
All claims expressed in this article are solely those of the authors and do not necessarily represent those of their affiliated organizations, or those of the publisher, the editors and the reviewers. Any product that may be evaluated in this article, or claim that may be made by its manufacturer, is not guaranteed or endorsed by the publisher.
1. Behjati S, Tarpey PS. What is next-generation sequencing? Arch Dis Child Educ Pract Ed. (2013) 98:236–8. doi: 10.1136/archdischild-2013-304340
2. Mardis ER. Next-generation sequencing platforms. Annu Rev Anal Chem (Palo Alto Calif). (2013) 6:287–303. doi: 10.1146/annurev-anchem-062012-092628
3. De Maglio G, Pasello G, Dono M, Fiorentino M, Follador A, Sciortino M, et al. The storm of NGS in NSCLC diagnostic-therapeutic pathway: How to sun the real clinical practice. Crit Rev Oncol Hematol. (2022) 169:103561. doi: 10.1016/j.critrevonc.2021.103561
4. Berger MF, Mardis ER. The emerging clinical relevance of genomics in cancer medicine. Nat Rev Clin Oncol. (2018) 15:353–65. doi: 10.1038/s41571-018-0002-6
5. Mosele F, Remon J, Mateo J, Westphalen CB, Barlesi F, Lolkema MP, et al. Recommendations for the use of next-generation sequencing (NGS) for patients with metastatic cancers: a report from the ESMO Precision Medicine Working Group. Ann Oncol. (2020) 31:1491–505. doi: 10.1016/j.annonc.2020.07.014
6. Schmid S, Jochum W, Padberg B, Demmer I, Mertz KD, Joerger M, et al. How to read a next-generation sequencing report-what oncologists need to know. ESMO Open. (2022) 7:100570. doi: 10.1016/j.esmoop.2022.100570
7. Byron SA, Van Keuren-Jensen KR, Engelthaler DM, Carpten JD, Craig DW. Translating RNA sequencing into clinical diagnostics: opportunities and challenges. Nat Rev Genet. (2016) 17:257–71. doi: 10.1038/nrg.2016.10
8. De Luca C, Pepe F, Iaccarino A, Pisapia P, Righi L, Listì A, et al. RNA-based assay for next-generation sequencing of clinically relevant gene fusions in non-small cell lung cancer. Cancers (Basel). (2021) 13:139. doi: 10.3390/cancers13010139
9. De Luca C, Pepe F, Pisapia P, Iaccarino A, Righi L, Listì A, et al. RNA-based next-generation sequencing in non-small-cell lung cancer in a routine setting: an experience from an Italian referral center. Per Med. (2022) 19:395–401. doi: 10.2217/pme-2022-0020
10. National Comprehensive Cancer Network (NCCN). NCCN clinical practice guidelines in oncology, in: Non-Small Cell Lung Cancer . Fort Washington (PA. Available online at: https://www.nccn.org/professionals/physician_gls/pdf/nscl.pdf (Accessed 26 Oct 2023). Version 3.2023.
11. Singh N, Jaiyesimi IA, Ismaila N, Leighl NB, Mamdani H, Phillips T, et al. Therapy for stage IV non-small-cell lung cancer with driver alterations: ASCO living guideline, version 2023.1. J Clin Oncol. (2023) 41:e42–50. doi: 10.1200/JCO.23.00281
12. Kim MH, Kim SH, Lee G, Mok J, Lee MK, Song JS, et al. Next-generation sequencing using tissue specimen collected with a 1.1 mm-diameter cryoprobe in patients with lung cancer. Respirology. (2024) 29:333–9. doi: 10.1111/resp.14680
13. Furuya N, Matsumoto S, Kakinuma K, Morikawa K, Inoue T, Saji H, et al. Suitability of transbronchial brushing cytology specimens for next-generation sequencing in peripheral lung cancer. Cancer Sci. (2021) 112:380–7. doi: 10.1111/cas.14714
14. Kage H, Kohsaka S, Shinozaki-Ushiku A, Hiraishi Y, Sato J, Nagayama K, et al. Small lung tumor biopsy samples are feasible for high quality targeted next generation sequencing. Cancer Sci. (2019) 110:2652–7. doi: 10.1111/cas.14112
15. Ku BM, Heo MH, Kim JH, Cho BC, Cho EK, Min YJ, et al. Molecular screening of small biopsy samples using next-generation sequencing in Korean patients with advanced non-small cell lung cancer: Korean lung cancer consortium (KLCC-13-01). J Pathol Transl Med. (2018) 52:148–56. doi: 10.4132/jptm.2018.03.12
16. Stoy SP, Segal JP, Mueller J, Furtado LV, Vokes EE, Patel JD, et al. Feasibility of endobronchial ultrasound-guided transbronchial needle aspiration cytology specimens for next generation sequencing in non-small-cell lung cancer. Clin Lung Cancer. (2018) 19:230–8.e2. doi: 10.1016/j.cllc.2017.11.010
17. Turner SR, Buonocore D, Desmeules P, Rekhtman N, Dogan S, Lin O, et al. Feasibility of endobronchial ultrasound transbronchial needle aspiration for massively parallel next-generation sequencing in thoracic cancer patients. Lung Cancer. (2018) 119:85–90. doi: 10.1016/j.lungcan.2018.03.003
18. Gao XH, Li J, Gong HF, Yu GY, Liu P, Hao LQ, et al. Comparison of fresh frozen tissue with formalin-fixed paraffin-embedded tissue for mutation analysis using a multi-gene panel in patients with colorectal cancer. Front Oncol. (2020) 10:310. doi: 10.3389/fonc.2020.00310
19. da Cunha Santos G. FTA cards for preservation of nucleic acids for molecular assays: A review on the use of cytologic/tissue samples. Arch Pathol Lab Med. (2018) 142:308–12. doi: 10.5858/arpa.2017-0303-RA
20. Murakami S, Yokose T, Nemoto D, Suzuki M, Usui R, Nakahara Y, et al. Suitability of bronchoscopic biopsy tissue samples for next-generation sequencing. Diagnostics (Basel). (2021) 11(3):391. doi: 10.3390/diagnostics11030391
21. Warmington AR, Wilkinson JM, Riley CB. Evaluation of ethanol-based fixatives as a substitute for formalin in diagnostic clinical laboratories. J Histotechnology. (2000) 23:299–308. doi: 10.1179/014788800794812878
22. Rahman MA, Sultana N, Ayman U, Bhakta S, Afrose M, Afrin M, et al. Alcoholic fixation over formalin fixation: A new, safer option for morphologic and molecular analysis of tissues. Saudi J Biol Sci. (2022) 29:175–82. doi: 10.1016/j.sjbs.2021.08.075
23. Bhat AH. Fixation and different types of fixatives: Their role and functions: A review. Int J Clin Diagn Pathol. (2021) 4(4):113–9. doi: 10.33545/pathol.2021.v4.i4b.433
24. Han JH. The pathological diagnosis and interpretation of pathological results: emphasis on immunohistochemical staining. Korean J Med. (2017) 92:36–40. doi: 10.3904/kjm.2017.92.1.36
Keywords: next-generation sequencing, RNA, surgical procedures, operative, lung neoplasm
Citation: Eom JS, Kim SH, Kim K, Kim A, Ahn HY, Mok J, Cho JS, Lee MK, Song JS and Kim M-H (2024) The clinical relevance of surgical specimens for RNA sequencing in lung cancer: a cohort study. Front. Oncol. 14:1462519. doi: 10.3389/fonc.2024.1462519
Received: 10 July 2024; Accepted: 02 October 2024;
Published: 18 October 2024.
Edited by:
Gary S. Stein, University of Vermont, United StatesReviewed by:
Pasquale Pisapia, University of Naples Federico II, ItalyCopyright © 2024 Eom, Kim, Kim, Kim, Ahn, Mok, Cho, Lee, Song and Kim. This is an open-access article distributed under the terms of the Creative Commons Attribution License (CC BY). The use, distribution or reproduction in other forums is permitted, provided the original author(s) and the copyright owner(s) are credited and that the original publication in this journal is cited, in accordance with accepted academic practice. No use, distribution or reproduction is permitted which does not comply with these terms.
*Correspondence: Mi-Hyun Kim, bWloeXVua2ltQHB1c2FuLmFjLmty
Disclaimer: All claims expressed in this article are solely those of the authors and do not necessarily represent those of their affiliated organizations, or those of the publisher, the editors and the reviewers. Any product that may be evaluated in this article or claim that may be made by its manufacturer is not guaranteed or endorsed by the publisher.
Research integrity at Frontiers
Learn more about the work of our research integrity team to safeguard the quality of each article we publish.