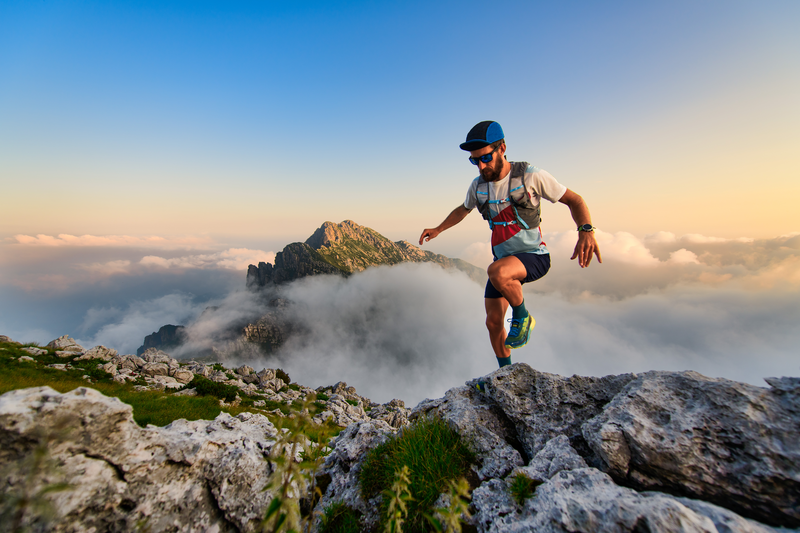
94% of researchers rate our articles as excellent or good
Learn more about the work of our research integrity team to safeguard the quality of each article we publish.
Find out more
REVIEW article
Front. Oncol. , 09 September 2024
Sec. Genitourinary Oncology
Volume 14 - 2024 | https://doi.org/10.3389/fonc.2024.1455428
This article is part of the Research Topic Multidisciplinary Management Of Urological Malignancies in the Era of Precision Medicine: Integration of Advances in Technology and Cancer Care View all 5 articles
The therapeutic landscape of metastatic prostate cancer has undergone a profound revolution in recent years. In addition to the introduction of novel molecules in the clinics, the field has witnessed a tremendous development of functional imaging modalities adding new biological insights which can ultimately inform tailored treatment strategies, including local therapies. The evolution and rise of Stereotactic Body Radiotherapy (SBRT) have been particularly notable in patients with oligometastatic disease, where it has been demonstrated to be a safe and effective treatment strategy yielding favorable results in terms of disease control and improved oncological outcomes. The possibility of debulking all sites of disease, matched with the ambition of potentially extending this treatment paradigm to polymetastatic patients in the not-too-distant future, makes Biology-guided Radiotherapy (BgRT) an attractive paradigm which can be used in conjunction with systemic therapy in the management of patients with metastatic prostate cancer.
Prostate cancer (PCa) is the most common type of cancer in men, with more than 280,000 new cases expected to be diagnosed in the United States in 2023, according to the American Cancer Society (1). While the majority of patients with localized disease have an excellent prognosis and may not even require active treatment, some forms are more aggressive and necessitate prompt intervention to prevent metastasis formation.
Metastatic prostate cancer refers to cancer that has spread from the prostate to other parts of the body, such as bones, lymph nodes, or visceral organs (2). This colonization of neoplastic cells represents a serious and often life-threatening condition, despite the currently wider therapeutic armamentarium that physicians have at their disposal.
The biology of metastatic prostate cancer is complex and multifaceted. It is believed that cancer cells can spread from the prostate via lymphatic system and blood vessels. According to the “Seed and Soil” theory, once these cells reach a favorable area, they interact and cooperate with the host micro-environment to form a new tumor deposit (3). At this level, the cross talk between resident immune cells and the tumor is key in determining further spreading of the disease to other organs and tissues.
Different somatic and germline mutations may lead to androgen receptor overexpression resulting in prostate cells overgrowth and tumorigenesis promotion, even in the absence of testosterone (4). Besides, the loss of suppressor genes such as PTEN and TP53 may also be observed in metastatic prostate cancer, as these genes normally play key roles in regulating cells’ growth and division (5).
Understanding genetic alterations, along with a careful evaluation of the interplay between the tumor and its microenvironment is therefore of paramount importance to elucidate the mechanisms behind metastasis. This knowledge could lead to the development of new and more effective systemic treatments for patients with stage IV prostate cancer, who are generally offered palliative therapy.
Nevertheless, the existence of an intermediate stage of disease has been hypothesized during recent years; the concept of the so-called “oligometastatic state” has emerged to identify those patients presenting with a limited number of metastases (usually up to three or five lesions) and who may be amenable to local ablative therapy (6), such as surgery or Stereotactic Body Radiotherapy (SBRT). The latter represents a radiotherapeutic approach that can accurately target and destroy the metastatic foci with curative doses aiming at potentially prolonging survival, at least in a subset of these patients, and improving their quality of life (7–9).
While there is a growing body of evidence supporting the integration of SBRT in treatment strategies for oligometastatic prostate cancer, the decision to pursue focal ablative treatment in this patient group should be made on a case-by-case basis. Factors to consider include location and size of the metastases, natural history of the disease and the possibility to integrate systemic therapy, together with a thorough evaluation of patients’ overall performance status and life expectancy (10).
Biology-guided radiotherapy (BgRT) represents an innovative approach in radiation oncology that aims to personalize and optimize cancer treatment based on the biology of each patient’s tumor. Radiotherapy traditionally consists of the delivery of a defined dose of radiation to the tumor, taking into account its size and anatomical location. In the setting of metastatic cancer, the logistical limitations of present-day technology have restricted its use to the palliation of symptomatic metastases, in which simple techniques and low doses are generally required. Therefore, there is a wide range of patients with stage IV cancer who are currently not deemed eligible for radiotherapy. One of the key components of BgRT is the use of advanced imaging techniques such as positron emission tomography (PET), which provides detailed information about the tumor’s metabolic activity, oxygen levels, and other biological features, depending on the type of radiotracer used. Moreover, by transforming tumors into their own fiducials after intravenous injection of a radiotracer, BgRT has the potential to simplify the process of radiotherapy delivery to multiple sites of disease throughout the body in the same treatment session and to track tumors in real time. The integration of imaging and biological data in BgRT enables clinicians to create treatment plans that are tailored to each patient’s tumor (11). This approach offers several potential benefits. Mainly, it could allow for more accurate targeting of the tumor, minimizing side effects due to radiation exposure of the surrounding healthy tissues. This precision can lead to a tangible improvement in patients’ quality of life during and after treatment. Nonetheless, the rationale behind BgRT could be crucial in the management of patients affected by metastatic disease and could make us rethink the role of radiotherapy in this clinical scenario. In recent years, several studies demonstrated the safety and efficacy of Stereotactic Body Radiotherapy (SBRT) as a metastasis-directed therapy (MDT) delivered with ablative intent in patients affected by metastatic cancer and more specifically in the oligometastatic state, defined as an intermediate state between a localized tumor and widespread metastatic disease (12, 13). Landmark studies such as the SABR-COMET (7), have shown that adding SBRT to the standard of care improves overall survival (OS) by reducing the total cancer burden, debulking gross lesions sited near sensible structures and ultimately diminishing the number of neoplastic clones that need to be eradicated by systemic treatment. Another piece of evidence on the role of ablative radiotherapy comes from the STOMP trial, the first prospective randomized study assessing the potential of MDT, primarily SBRT, to forestall initiation of Androgen Deprivation Therapy (ADT) in hormone-sensitive oligometastatic prostate cancer patients presenting with three or fewer detectable metastases) (14). Similarly, a single-arm phase II trial of SBRT in oligometastatic disease demonstrated a 2-yr freedom from ADT of 48% (15). Moreover, the STAMPEDE trial showed that treating the primary tumor with radiotherapy improves OS, without detriment in QoL, in men with newly diagnosed low-burden metastatic prostate cancer, indicating that it should be recommended as a standard of care (16). Additional evidence regarding the importance of SBRT in low-burden disease comes from a post-hoc analysis of the KEYNOTE-001 study; in this trial, patients affected by non-small cell lung cancer (NSCLC) with metastatic disease showed improved OS if they received metastasis-directed therapy compared to the ones who didn’t (6 months OS was 73% vs. 45) (17). Based on available data from clinical trials, we currently therefore include SBRT as a valid treatment option which may be integrated with other treatment modalities in oligometastatic cancer patients. More specifically, the use of SBRT is based on the hypothesis that early ablation of metastatic disease may both prevent further seeding and influence metastatic crosstalk. Prostate cancer is a multifocal tumor with high intra-tumoral and inter-tumoral heterogeneity that can harbor or develop aggressive foci with time (18). Individual subclones can seed from the primary or from one metastasis to another in a polyclonal fashion, so that, every metastatic site can be considered a conglomerate of different subclones. In addition, it seems that metastasis-private mutations are associated with drug resistance (19). In this biological scenario, ablation of both the primary and oligometastatic tumor deposits can potentially eliminate sources for additional seeding events and could improve oncologic outcomes in patients with metastatic disease (20, 21). Regarding the tumor microenvironment, it has been hypothesized that SBRT induces an in situ vaccine response triggering the local activation of systemic antitumor immunity and promoting tumor-antigen expression and T-cell re-activation (22, 23). Considering the growing importance of cancer immunotherapy, numerous preclinical studies investigated the combination of a range of radiation regimens and immune checkpoint inhibitors. Lee et al. (24) explored the effect of high-dose single fractions which improves antigens presentation by antigen-presenting cells (APCs) and induces CD8+ T-cell priming. High doses of ionizing radiation induce pro-inflammatory signals via the cGAS-STING-IFN pathway, which could convert immune cells in the tumor microenvironments into phenotypes more amenable to T-cell trafficking but could also induce the release of a diverse array of neoantigens from the different metastatic sites into the blood circle (25–27). This hypothesis was reinforced by Diamond et al. findings which proved that even a lower dose of 8 Gy per fraction could also lead to antitumor T-cell responses in poorly immunogenic tumors, by promoting I IFN production and recruitment of dendritic cells into tumors (28).
Based on all these biological and clinical considerations, it may be plausible to expand this treatment paradigm in the setting of polymetastatic disease as well.
In the polymetastatic state, characterized by a widespread dissemination of metastases, patients are treated mainly with systemic therapies and may eventually become resistant to all different drug regimens. Recent advances in radiation oncology may give us the opportunity to raise the bar and allow the treatment of all sites of disease, overcoming the limit in terms of number of lesions that we imposed ourselves when we introduced the concept of oligometastases.
A crucial issue of metastasis-directed therapy is that the imaging techniques used to detect the lesions should be accurate enough to define the actual metastatic burden. It is presumed that the more precise the disease extent definition is, the greater the percentage of patients receiving the appropriate MDT treatment would be, with expected improved oncological outcomes. In the 2017 Advanced Prostate Cancer Consensus Conference meeting (APCCC), oligometastatic prostate cancer was defined as the presence of three or fewer bone or lymph node metastases according to standard imaging modalities, including bone scintigraphy, contrast-enhanced computed tomography (CT) and morphological Magnetic Resonance Imaging (MRI) (29). However, although recommended by most guidelines, these techniques have poor diagnostic accuracy, underestimating the exact number of metastatic deposits (30). In this setting, functional imaging may represent an added value because it provides information on the biologically active cancer lesions and modern imaging techniques including PET/CT with specific tracers have demonstrated an improved detection rate (DR) for PCa recurrences compared to conventional imaging, especially for PSA levels less than 2 ng/mL (31).
Fluorine-18 (18F) or Carbon-11 (11C) radiolabeled choline was the first radiopharmaceutical agent employed in PCa evaluation. Choline is an essential nutrient involved in the synthesis of phosphatidylcholine, a vital component of the cell membrane. The increase in cell proliferation as well as the activity of the enzyme choline kinase in PCa cells is associated with an increase in choline uptake (32). For several years, it has been recommended by international guidelines as the gold-standard approach for PCa restaging in the presence of biochemical recurrence (BCR) (33). However, the clinical utility of choline-labeled PET/CT remains controversial in patients with early BCR. Treglia et al. in their meta-analysis, found that PSA doubling-time (PSAdt) ≤ 6 months and PSA levels >1 or >2 ng/mL/year proved to be relevant factors in predicting the positive result of radiolabeled choline PET/CT. The detection rate (DR) of radiolabeled choline PET/CT increased to 65% when PSAdt was ≤6 months and to 71% and 77% when PSA levels were >1 or >2 ng/mL/year, respectively (34). These data support the use of choline in this patient population, particularly for cases with PSA values exceeding 1 ng/mL.
The need for more accurate radiotracers led to the development of a new PET radiopharmaceutical and in May 2016, following its FDA approval, [18F]fluciclovine emerged as a useful molecular imaging agent in patients with PCa (35).
[18F]fluciclovine is a leucine analogue absorbed via the L-type amino acid transporter (LAT1) and the sodium-dependent neutral amino acid transporter (ASCT2), which is upregulated in many human cancers, including PCa (36).
When considering the impact of different biochemical parameters on prostate-radiopharmaceuticals DR, the [18F]fluciclovine PET/CT demonstrated superior performance in case of both low (0.5–1 ng/mL) and high PSA levels (>1 ng/mL). Additionally, PSAdt did not have significant impact on the effectiveness of [18F]fluciclovine DR, preserving a good performance also for PSAdt > 12 months (37).
However, [18F]fluorocholine PET/CT demonstrated better performance in the evaluation of the bone region (37).
In the last few years, the introduction of radiopharmaceuticals targeting Prostate-Specific Membrane Antigen (PSMA) has revolutionized diagnostic imaging of PCa (38). Positron-emitting radioisotopes such as Fluorine-18 (18F), Gallium-68 (68Ga), Copper-64 (64Cu), and Zirconium-89 (89Zr) all selectively bind to the extracellular domain of PSMA, which is typically overexpressed in PCa. 18F-based PSMA- targeted PET agents have the advantage of a longer radioisotope half-life (110 minutes), with increased positron yield, shorter positron range and good spatial resolution, favoring centralized radioisotope production and distribution (39, 40). However, there have not been large randomized clinical trials comparing these agents, and to date there is little clinical difference between them (41).
Also, the different radiochemistry may sometimes reflect a distinct physiologic and para-physiologic organ distribution. However, molecule leakage may also lead to non-specific uptake. Therefore, the PSMA ligand imaging analysis reports potential pitfalls (e.g. ganglia, benign bone lesions or non-specific lymph nodes). For example, 18F can leak out of the molecule leading to non-specific bone uptake but it also presents an advantage in distinguishing ureter or bladder radioactivity from local recurrence or locoregional metastases, due to minimally excreted concentration via the urinary tract in the case of 18F-PSMA-1007 (42, 43).
According to the recently published EANM/SNMMI guidelines (44), the radiopharmaceuticals 68 Ga-PSMA-11, 68 Ga-PSMA-I&T, 18F-DCFPyL, 18F-PSMA-1007, and 18F-rhPSMA-7.3 represent the most advanced imaging tools. Among them, 68 Ga-PSMA-11 (ILLUCCIX), 18F-DCFPyL (PYLARIFY/PYLCLARI), and 18F-rhPSMA-7.3 (POSLUMA) are already FDA and EU-approved.
Currently in the clinic, PSMA PET is predominantly indicated in recurrent or persistent prostate cancer, as a re-staging procedure following curative-intent therapy; it can also be employed as a primary staging procedure in high-risk disease and in the setting of castrate-resistant prostate cancer, which appears localized on conventional imaging. The factors associated with a higher DR are PSA, PSAdt, Gleason score and PSMA expression of the primary (45–49).
Concerning lymph nodes detection, PSMA PET has demonstrated a sensitivity and a specificity of 99% and 76%, respectively (50).
The value of the bone detection rate was also analyzed by several authors. Zhou et al. (51) obtained per-patient pooled sensitivity values of PSMA-PET/CT, choline-PET/CT, NaF-PET/CT, MRI, and BS; they were 0.97, 0.87, 0.96, 0.91, and 0.86, respectively, while the pooled specificities were 1.00, 0.99, 0.97, 0.96, and 0.95.
The key focus at present is to establish whether this improved diagnostic performance may guide changes in the therapeutic management of PCa patients. In their meta-analysis of 34 studies including 1057 patients with biochemical relapse-free survival, Pozdnyakov et al. demonstrated that PSMA PET can alter treatment plans in 56.4% of the population. BCR-free survival was 60.2% at median follow up of 20 months (52).
In another multicenter experience, PSMA PET showed a DR of 40.9% for PSA values of 0.2–0.4 ng/ml and 64.2% for values from 0.8 to 1 ng/ml (53).
Overall, these results make PSMA PET a very appealing tool for guiding MDT approach, especially in metachronous oligometastatic disease occurring after radical surgery; this therapeutic approach may not only provide local control of the treated lesions and potentially better oncological outcomes, but it can also ameliorate quality of life by delaying the onset of ADT and its related side effects (54, 55).
Metastatic hormone-sensitive prostate cancer (mHSPC) is an advanced stage of prostate cancer characterized by the presence of distant metastases and sensitivity to hormonal manipulation.
Although Androgen Deprivation Therapy (ADT) has historically represented the gold standard pillar for the treatment of this stage of disease, the therapeutic management of mHSPC has undergone significant changes especially during the last decade, leading to expanded treatment options for these patients and improved oncological outcomes (56). Concomitantly with the development of several new drugs, which have demonstrated the capacity of potentiating standard ADT and overcoming its initial mechanisms of resistance, a particular form of high intensity radiotherapy (SBRT) was shown to improve outcomes in the oligometastatic setting according to the results of specifically designed Phase II trials (14, 21) and one phase III trial (7).
Systemic oncological therapy plays a crucial role in the treatment of mHSPC. For several decades, androgen deprivation therapy (ADT) alone represented the standard approach (57), aiming to suppress the production or block the action of androgens, which fuel the growth of prostate cancer cells.
However, emerging evidence has demonstrated the benefits of combining ADT with other agents, such as chemotherapy and novel hormonal therapies (58). These systemic treatments target various pathways involved in prostate cancer growth and progression, offering the potential for enhanced tumor control and improved patient outcomes.
One of the trials that revolutionized the treatment landscape for mHSPC was the CHAARTED trial. This landmark study compared ADT alone versus ADT in combination with docetaxel, a taxane-based chemotherapy, in patients with newly diagnosed mHSPC (59). The trial demonstrated a significant improvement in overall survival and delayed disease progression in the combination arm, leading to the incorporation of docetaxel into the standard treatment regimen for mHSPC. Subsequent studies, including the STAMPEDE (60) and LATITUDE (61) trials, further supported the use of combination therapies by showing prolonged survival and delayed disease progression with the addition of abiraterone acetate, an androgen biosynthesis inhibitor, to ADT.
Moreover, the understanding of prostate cancer biology has led to the development of novel hormonal therapies targeting the androgen receptor pathway. Drugs such as enzalutamide and apalutamide, which act as androgen receptor antagonists, have demonstrated efficacy in improving survival outcomes and delaying disease progression in mHSPC (62, 63). These agents provide additional options for patients who may not be suitable for or have contraindications to chemotherapy.
Nonetheless, the landmark trial PEACE 1 (64) demonstrated that for selected patients presenting with de novo, high-volume, hormone-sensitive metastatic prostate cancer, triplet therapy with ADT, an androgen-receptor inhibitor, and docetaxel may represent the most efficacious strategy.
The oligometastatic state refers to a stage of prostate cancer characterized by a limited number of metastases, typically confined to a few anatomic sites (6). In recent years, studies have explored the use of localized treatments, including ablative RT, to target and control these limited metastatic lesions (65). The rationale behind this approach is to eradicate or control oligometastatic disease, potentially delaying or preventing the need for systemic therapy, and improving long-term outcomes.
Concerning the role of SBRT, The COMET trial focused on evaluating the benefits of metastasis-directed therapy in a group of oligometastatic patients from different primitive tumors, including prostate cancer patients (7). The trial demonstrated a significant improvement in survival outcomes in patients receiving MDT. These findings have generated interest in the use of ablative RT as a potential therapeutic option for patients with oligometastatic prostate cancer, leading to ongoing research and exploration of the optimal timing, dose, and target selection for RT in this context.
Additionally, multiple retrospective studies and some phase II trials have reported encouraging outcomes with the use of SBRT in oligometastatic prostate cancer (66). These studies have shown that the addition of ablative radiotherapy to the oligometastases led to improved local control, progression free survival and delayed initiation of systemic therapy (14, 15). However, it is important to underline that further investigations in appropriate clinical trials will be crucial for determining optimal patient selection, radiation techniques, target volumes and dose fractionation schemes for these patients.
One of the arms of the STAMPEDE trial investigated the addition of RT to the primary tumor in patients with newly diagnosed mHSPC (16). Low metastatic burden was determined to be predictive of improved overall survival (OS) when radiation therapy (RT) was added to standard of care (SOC) therapy in these patients; of note, this benefit was not demonstrated in patients presenting with high burden metastatic disease. A subsequent subgroup analysis (67) revealed that the association of radiotherapy to the prostate + SOC did improve OS (p-value = 0.003) and failure-free survival (FFS) (treatment interaction p-value 0.001) in patients with only non-regional lymph nodes or < 4 bone metastases regardless of location and no visceral metastases over SOC alone.
The RefleXion® X1 biology-guided radiotherapy system (RefleXion Medical, Inc., Hayward, CA) combines dual imaging technologies (CT and PET) with a 6MV linear accelerator in a ring gantry (68). kVCT imaging is classically used for patient setup, while PET detects outgoing tumor emissions used to direct the therapy beam with sub-second latency. This interplay between the lesion and treatment machine allows the system to accurately guide and conform radiation beamlets to the tumor, taking into account its possible movement during treatment, to finally obtain a tracked dose distribution with the aim of sparing the irradiation of surrounding normal tissues. This has a profound impact on the classic radiotherapy workflow. Emissions from a tumor after radiotracer injection act as a fiducial, raising the confidence in target identification and reducing the need for positional margins to be added to the treated volumes during treatment planning. Besides, predictive motion models and motion management itself may be dramatically simplified and reduced by using a single radiotracer injection to manage motion during treatment to multiple sites of gross disease deposits throughout the body. The RefleXion biology-guided radiotherapy, referred to as SCINTIX® therapy, has been FDA-cleared in 2023 for the treatment of lung and bone tumors (that could be primary or metastatic) using 18F-FDG. We can envision therapeutic avenues of this technology for metastatic prostate cancer patients, as well as the potential of using other radiotracers such as PSMA for SCINTIX therapy. Indeed, a preliminary study (69) reported promising results regarding the feasibility of [18F]-DCFPyL-guided BgRT planning. Twenty patients who were already scheduled for a PSMA diagnostic PET scan underwent a second scan on the RefleXion X1 to evaluate the quality of detection of [18F]-DCFPyL PSMA by the PET subsystem and the feasibility of [18F]-DCFPyL-guided BgRT planning. A PET avid tumor was identified and segmented for planning in 16 patients (4 lymph nodes, 5 bone, 6 prostate gland, and 1 prostate bed). Wong et al. demonstrated that BgRT planning was feasible and met standard of care stereotactic body radiotherapy (SBRT) organ dose constraints in 8 patients (3 prostate gland, 3 bone, 2 lymph nodes) (68).
In this context, Palma et al. recently designed a phase I trial analyzing the safety and tolerability of SBRT for the treatment of polymetastatic disease (70). If confirmed in future research, these results would therefore open new horizons for radiotherapy applications in the clinics, and BgRT may emerge as a valuable tool for the treatment of all metastatic sites with ablative doses in cases of polymetastatic cancer.
Notable technological advancements have enabled a prominent role for medical imaging in cancer treatments, specifically in radiation oncology (71). With the emergence of the field of radiomics, the interest of the scientific community in extracting quantitative image parameters for radiotherapy applications and prognostication is now increasing (72). In prostate cancer, several attempts have been made to find prognostic biomarkers at different time points of the disease, from diagnosis to metastases formation (Table 1). Of note, radiomic features extracted from PET images may provide valuable information for better patient selection and response to therapies. Furthermore, integrating functional imaging in the BgRT workflow may pave the way towards potentially relevant clinical applications in the field of radiotherapy, ultimately leading to a more personalized approach and plan optimization. The Dominant Intraprostatic Lesion (DIL) visible at functional imaging and/or MRI in localized prostate cancer, represents an example of when a biology-guided prescription and delivery of a non-uniform dose to the clinical target volume (CTV) is beneficial; boosting the intraprostatic lesion was shown to improve biochemical disease-free survival in patients with localized prostate cancer without impacting toxicity and quality of life (88). Likewise, the functional information obtained with molecular imaging in metastatic prostate cancer may not only reduce inter-observer variability in target volume delineation and decrease radiation therapy planning time, but it can also guide dose escalation to potential tumor sub volumes with increased radioresistance or higher tumor burden. Gaudreault et al. (89) have explored the possibility of integrating LuPSMA therapy with BgRT in metastatic CRPC patients who have PSMA-negative/FDG-positive PET imaging; more specifically, these patients are not likely to benefit from radionuclide therapy because some disease sites may not represent a target due to inadequate PSMA uptake, but they may be good candidates for FDG PET-guided BgRT.
In conclusion, we believe that BgRT, already a new addition in the armamentarium against lung and bone tumors using 18F-FDG, could represent a potentially valuable add-on in the integrative therapy management of prostate cancer patients using a PSMA radiotracer. This is particularly relevant in the setting of metastatic disease, where we are already exploring offering metastasis directed therapy in a polymetastatic state (70).
Further research is warranted to confirm the safety and the efficacy of this fascinating therapeutic hypothesis.
AL: Writing – original draft, Writing – review & editing. GI: Writing – original draft, Writing – review & editing. BD: Writing – original draft, Writing – review & editing. EF: Writing – original draft, Writing – review & editing. EB: Writing – original draft, Writing – review & editing. FL: Writing – original draft, Writing – review & editing. FC: Writing – original draft, Writing – review & editing. NB: Writing – original draft, Writing – review & editing. SL: Writing – original draft, Writing – review & editing. CO: Writing – original draft, Writing – review & editing. GF: Writing – original draft, Writing – review & editing. EA: Writing – original draft, Writing – review & editing. LL: Writing – original draft, Writing – review & editing. CA: Writing – original draft, Writing – review & editing. DD: Writing – review & editing. KA: Writing – review & editing. SS: Writing – review & editing. CB: Writing – original draft, Writing – review & editing.
The author(s) declare financial support was received for the research, authorship, and/or publication of this article. APC will be paid by RefleXion Medical. The funder was not involved in the study design, collection, analysis, interpretation of data, the writing of this article, or the decision to submit it for publication.
Authors DD and KA were employed by the company RefleXion Medical, Inc.
The remaining authors declare that the research was conducted in the absence of any commercial or financial relationships that could be construed as a potential conflict of interest.
The author(s) declared that they were an editorial board member of Frontiers, at the time of submission. This had no impact on the peer review process and the final decision
All claims expressed in this article are solely those of the authors and do not necessarily represent those of their affiliated organizations, or those of the publisher, the editors and the reviewers. Any product that may be evaluated in this article, or claim that may be made by its manufacturer, is not guaranteed or endorsed by the publisher.
1. Siegel RL, Miller KD, Wagle NS, Jemal A. Cancer statistics, 2023. CA Cancer J Clin. (2023) 73:17–48. doi: 10.3322/caac.21763
2. Gundem G, Van Loo P, Kremeyer B, Alexandrov LB, Tubio JMC, Papaemmanuil E, et al. The evolutionary history of lethal metastatic prostate cancer. Nature. (2015) 520:353–7. doi: 10.1038/nature14347
3. Langley RR, Fidler IJ. The seed and soil hypothesis revisited–the role of tumor-stroma interactions in metastasis to different organs. Int J Cancer. (2011) 128:2527–35. doi: 10.1002/ijc.26031
4. Hoang DT, Iczkowski KA, Kilari D, See W, Nevalainen MT. Androgen receptor-dependent and -independent mechanisms driving prostate cancer progression: Opportunities for therapeutic targeting from multiple angles. Oncotarget. (2017) 8:3724–45. doi: 10.18632/oncotarget.v8i2
5. Al-Toubat M, Serrano S, Elshafei A, Koul K, Feibus AH, Balaji KC. Metastatic prostate cancer is associated with distinct higher frequency of genetic mutations at diagnosis. Urol Oncol. (2023) 41 (11):455.e7–.e15. doi: 10.1016/j.urolonc.2023.09.014
6. Weichselbaum RR, Hellman S. Oligometastases revisited. Nat Rev Clin Oncol. (2011) 8:378–82. doi: 10.1038/nrclinonc.2011.44
7. Harrow S, Palma DA, Olson R, Gaede S, Louie AV, Haasbeek C, et al. Stereotactic radiation for the comprehensive treatment of oligometastases (SABR-COMET): extended long-term outcomes. Int J Radiat Oncol Biol Phys. (2022) 114:611–6. doi: 10.1016/j.ijrobp.2022.05.004
8. Mohan R, Kneebone A, Eade T, Hsiao E, Emmett L, Brown C, et al. Long-term outcomes of SBRT for PSMA PET detected oligometastatic prostate cancer. Radiat Oncol. (2023) 18:127. doi: 10.1186/s13014-023-02302-8
9. Francolini G, Gaetano Allegra A, Detti B, Di Cataldo V, Caini S, Bruni A, et al. Stereotactic body radiation therapy and abiraterone acetate for patients affected by oligometastatic castrate-resistant prostate cancer: A randomized phase II trial (ARTO). J Clin Oncol. (2023) 41(36):5561–8. doi: 10.1200/JCO.23.00985
10. Zilli T, Achard V, Dal Pra A, Schmidt-Hegemann N, Jereczek-Fossa BA, Lancia A, et al. Recommendations for radiation therapy in oligometastatic prostate cancer: An ESTRO-ACROP Delphi consensus. Radiother Oncol. (2022) 176:199–207. doi: 10.1016/j.radonc.2022.10.005
11. Shirvani SM, Huntzinger CJ, Melcher T, Olcott PD, Voronenko Y, Bartlett-Roberto J, et al. Biology-guided radiotherapy: redefining the role of radiotherapy in metastatic cancer. Br J Radiol. (2021) 94:20200873. doi: 10.1259/bjr.20200873
12. Gomez DR, Tang C, Zhang J, Blumenschein GR Jr, Hernandez M, Lee JJ, et al. Local consolidative therapy vs. Maintenance therapy or observation for patients with oligometastatic non-small-cell lung cancer: long-term results of a multi-institutional, phase II, randomized study. J Clin Oncol. (2019) 37:1558–65. doi: 10.1200/JCO.19.00201
13. Iyengar P, Wardak Z, Gerber DE, Tumati V, Ahn C, Hughes RS, et al. Consolidative radiotherapy for limited metastatic non-small-cell lung cancer: A phase 2 randomized clinical trial. JAMA Oncol. (2018) 4:e173501. doi: 10.1001/jamaoncol.2017.3501
14. Ost P, Reynders D, Decaestecker K, Lumen N, De Bruycker A, Lambert B, et al. Surveillance or metastasis-directed therapy for oligometastatic prostate cancer recurrence: A prospective, randomized, multicenter phase II trial. J Clin Oncol. (2018) 36:446–53. doi: 10.1200/JCO.2017.75.4853
15. Siva S, Bressel M, Murphy DG, Shaw M, Chander S, Violet J, et al. Stereotactic abative body radiotherapy (SABR) for oligometastatic prostate cancer: A prospective clinical trial. Eur Urol. (2018) 74:455–62. doi: 10.1016/j.eururo.2018.06.004
16. Parker CC, James ND, Brawley CD, Clarke NW, Hoyle AP, Ali A, et al. Radiotherapy to the primary tumour for newly diagnosed, metastatic prostate cancer (STAMPEDE): a randomised controlled phase 3 trial. Lancet. (2018) 392:2353–66. doi: 10.1016/S0140-6736(18)32486-3
17. Shaverdian N, Lisberg AE, Bornazyan K, Veruttipong D, Goldman JW, Formenti SC, et al. Previous radiotherapy and the clinical activity and toxicity of pembrolizumab in the treatment of non-small-cell lung cancer: a secondary analysis of the KEYNOTE-001 phase 1 trial. Lancet Oncol. (2017) 18:895–903. doi: 10.1016/S1470-2045(17)30380-7
18. Haffner MC, Zwart W, Roudier MP, True LD, Nelson WG, Epstein JI, et al. Genomic and phenotypic heterogeneity in prostate cancer. Nat Rev Urol. (2021) 18:79–92. doi: 10.1038/s41585-020-00400-w
19. Hu Z, Li Z, Ma Z, Curtis C. Multi-cancer analysis of clonality and the timing of systemic spread in paired primary tumors and metastases. Nat Genet. (2020) 52:701–8. doi: 10.1038/s41588-020-0628-z
20. Heidenreich A, Fossati N, Pfister D, Suardi N, Montorsi F, Shariat S, et al. Cytoreductive radical prostatectomy in men with prostate cancer and skeletal metastases. Eur Urol Oncol. (2018) 1:46–53. doi: 10.1016/j.euo.2018.03.002
21. Phillips R, Shi WY, Deek M, Radwan N, Lim SJ, Antonarakis ES, et al. Outcomes of observation vs stereotactic ablative radiation for oligometastatic prostate cancer: the ORIOLE phase 2 randomized clinical trial. JAMA Oncol. (2020) 6:650–9. doi: 10.1001/jamaoncol.2020.0147
22. Formenti SC, Rudqvist NP, Golden E, Cooper B, Wennerberg E, Lhuillier C, et al. Radiotherapy induces responses of lung cancer to CTLA-4 blockade. Nat Med. (2018) 24:1845–51. doi: 10.1038/s41591-018-0232-2
23. Golden EB, Chhabra A, Chachoua A, Adams S, Donach M, Fenton-Kerimian M, et al. Local radiotherapy and granulocyte-macrophage colony-stimulating factor to generate abscopal responses in patients with metastatic solid tumours: a proof-of-principle trial. Lancet Oncol. (2015) 16:795–803. doi: 10.1016/S1470-2045(15)00054-6
24. Lee Y, Auh SL, Wang Y, Burnette B, Wang Y, Meng Y, et al. Therapeutic effects of ablative radiation on local tumor require CD8+ T cells: changing strategies for cancer treatment. Blood. (2009) 114:589–95. doi: 10.1182/blood-2009-02-206870
25. Tang C, Wang X, Soh H, Seyedin S, Cortez MA, Krishnan S, et al. Combining radiation and immunotherapy: a new systemic therapy for solid tumors? Cancer Immunol Res. (2014) 2:831–8. doi: 10.1158/2326-6066.CIR-14-0069
26. Brooks ED, Schoenhals JE, Tang C, Micevic G, Gomez DR, Chang JY, et al. Stereotactic ablative radiation therapy combined with immunotherapy for solid tumors. Cancer J. (2016) 22:257–66. doi: 10.1097/PPO.0000000000000210
27. Formenti SC, Demaria S. Systemic effects of local radiotherapy. Lancet Oncol. (2009) 10:718–26. doi: 10.1016/S1470-2045(09)70082-8
28. Diamond JM, Vanpouille-Box C, Spada S, Rudqvist NP, Chapman JR, Ueberheide BM, et al. Exosomes shuttle TREX1-sensitive IFN-stimulatory dsDNA from irradiated cancer cells to DCs. Cancer Immunol Res. (2018) 6:910–20. doi: 10.1158/2326-6066.CIR-17-0581
29. Gillessen S, Attard G, Beer TM, Beltran H, Bossi A, Bristow R, et al. Management of patients with advanced prostate cancer: the report of the advanced prostate cancer consensus conference APCCC 2017. Eur Urol. (2018) 73:178–211. doi: 10.1016/j.eururo.2017.06.002
30. deSouza NM, Liu Y, Chiti A, Oprea-Lager D, Gebhart G, Van Beers BE, et al. Strategies and technical challenges for imaging oligometastatic disease: Recommendations from the European Organisation for Research and Treatment of Cancer imaging group. Eur J Cancer. (2018) 91:153–63. doi: 10.1016/j.ejca.2017.12.012
31. Fanti S, Minozzi S, Antoch G, Banks I, Briganti A, Carrio I, et al. Consensus on molecular imaging and theranostics in prostate cancer. Lancet Oncol. (2018) 19:e696–708. doi: 10.1016/S1470-2045(18)30604-1
32. Podo F. Tumour phospholipid metabolism. NMR Biomed. (1999) 12:413–39. doi: 10.1002/(ISSN)1099-1492
33. Cornford P, Bellmunt J, Bolla M, Briers E, De Santis M, Gross T, et al. EAU-ESTRO-SIOG guidelines on prostate cancer. Part II: treatment of relapsing, metastatic, and castration-resistant prostate cancer. Eur Urol. (2017) 71:630–42. doi: 10.1016/j.eururo.2016.08.002
34. Treglia G, Ceriani L, Sadeghi R, Giovacchini G, Giovanella L. Relationship between prostate-specific antigen kinetics and detection rate of radiolabelled choline PET/CT in restaging prostate cancer patients: a meta-analysis. Clin Chem Lab Med. (2014) 52:725–33. doi: 10.1515/cclm-2013-0675
35. Schuster DM, Nanni C, Fanti S. PET tracers beyond FDG in prostate cancer. Semin Nucl Med. (2016) 46:507–21. doi: 10.1053/j.semnuclmed.2016.07.005
36. Savir-Baruch B, Zanoni L, Schuster DM. Imaging of prostate cancer using fluciclovine. Urol Clin North Am. (2018) 45:489–502. doi: 10.1016/j.ucl.2018.03.015
37. Ferrari C, Mammucci P, Lavelli V, Pisani AR, Nappi AG, Rubini D, et al. [18F]fluciclovine vs. [18F]fluorocholine positron emission tomography/computed tomography: A head-to-head comparison for early detection of biochemical recurrence in prostate cancer patients. Tomography. (2022) 8:2709–22. doi: 10.3390/tomography8060226
38. Hofman MS, Lawrentschuk N, Francis RJ, Tang C, Vela I, Thomas P, et al. Prostate-specific membrane antigen PET-CT in patients with high-risk prostate cancer before curative-intent surgery or radiotherapy (proPSMA): a prospective,randomised, multicentre study. Lancet. (2020) 395:1208–16. doi: 10.1016/S0140-6736(20)30314-7
39. Mena E, Lindenberg L, Choyke P. The impact of PSMA PET/CT imaging in prostate cancer radiation treatment. Semin Nucl Med. (2022) 52:255–62. doi: 10.1053/j.semnuclmed.2021.12.008
40. Werner RA, Derlin T, Lapa C, Sheikbahaei S, Higuchi T, Giesel FL, et al. 18F-labeled, PSMA-targeted radiotracers: leveraging the advantages of radiofluorination for prostate cancer molecular imaging. Theranostics. (2020) 10:1–16. doi: 10.7150/thno.37894
41. Huang S, Ong S, McKenzie D, Mirabelli A, Chen DC, Chengodu T, et al. Comparison of 18F-based PSMA radiotracers with [68Ga]Ga-PSMA-11 in PET/CT imaging of prostate cancer-a systematic review and meta-analysis. Prostate Cancer Prostatic Dis. (2023). doi: 10.1038/s41391-023-00755-2
42. Rauscher I, Krönke M, König M, Gafita A, Maurer T, Horn T, et al. Matched-pair comparison of 68Ga-PSMA-11 PET/CT and 18F-PSMA-1007 PET/CT: frequency of pitfalls and detection efficacy in biochemical recurrence after radical prostatectomy. J Nucl Med. (2020) 61:51–7. doi: 10.2967/jnumed.119.229187
43. Rahbar K, Afshar-Oromieh A, Seifert R, Wagner S, Schäfers M, Bögemann M, et al. Diagnostic performance of 18F-PSMA-1007 PET/CT in patients with biochemical recurrent prostate cancer. Eur J Nucl Med Mol Imaging. (2018) 45:2055–61. doi: 10.1007/s00259-018-4089-x
44. Fendler WP, Eiber M, Beheshti M, Bomanji J, Calais J, Ceci F, et al. PSMA PET/CT: joint EANM procedure guideline/SNMMI procedure standard for prostate cancer imaging 2.0. Eur J Nucl Med Mol Imaging. (2023) 50:1466–86. doi: 10.1007/s00259-022-06089-w
45. Yilmaz U, Komek H, Can C, Altindag S. The role of (68Ga)PSMA I&T in biochemical recurrence after radical prostatectomy: detection rate and the correlation between the level of PSA, Gleason score, and the SUVmax. Ann Nucl Med. (2019) 33:545–53. doi: 10.1007/s12149-019-01360-x
46. Verburg FA, Pfister D, Heidenreich A, Vogg A, Drude NI, Vöö S, et al. Extent of disease in recurrent prostate cancer determined by [(68)Ga]PSMA-HBED-CC PET/CT in relation to PSA levels, PSA doubling time and Gleason score. Eur J Nucl Med Mol Imaging. (2016) 43:397–403. doi: 10.1007/s00259-015-3240-1
47. Uprimny C, Kroiss AS, Decristoforo C, Fritz J, Warwitz B, Scarpa L, et al. Early dynamic imaging in 68Ga- PSMA-11 PET/CT allows discrimination of urinary bladder activity and prostate cancer lesions. Eur J Nucl Med Mol Imaging. (2017) 44:765–75. doi: 10.1007/s00259-016-3578-z
48. Rüschoff JH, Ferraro DA, Muehlematter UJ, Laudicella R, Hermanns T, Rodewald AK, et al. What's behind 68Ga-PSMA-11 uptake in primary prostate cancer PET? Investigation of histopathological parameters and immunohistochemical PSMA expression patterns. Eur J Nucl Med Mol Imaging. (2021) 48:4042–53. doi: 10.1007/s00259-021-05501-1
49. Ferraro DA, Rüschoff JH, Muehlematter UJ, Kranzbühler B, Müller J, Messerli M, et al. Immunohistochemical PSMA expression patterns of primary prostate cancer tissue are associated with the detection rate of biochemical recurrence with 68Ga-PSMA-11-PET. Theranostics. (2020) 10:6082–94. doi: 10.7150/thno.44584
50. Hope TA, Goodman JZ, Allen IE, Calais J, Fendler WP, Carroll PR. Metaanalysis of 68Ga-PSMA-11 PET accuracy for the detection of prostate cancer validated by histopathology. J Nucl Med. (2019) 60:786–93. doi: 10.2967/jnumed.118.219501
51. Zhou J, Gou Z, Wu R, Yuan Y, Yu G, Zhao Y. Comparison of PSMA-PET/CT, choline-PET/CT, NaF-PET/CT, MRI, and bone scintigraphy in the diagnosis of bone metastases in patients with prostate cancer: a systematic review and meta-analysis. Skeletal Radiol. (2019) 48:1915–24. doi: 10.1007/s00256-019-03230-z
52. Pozdnyakov A, Kulanthaivelu R, Bauman G, Ortega C, Veit-Haibach P, Metser U. The impact of PSMA PET on the treatment and outcomes of men with biochemical recurrence of prostate cancer: a systematic review and meta-analysis. Prostate Cancer Prostatic Dis. (2023) 26:240–8. doi: 10.1038/s41391-022-00544-3
53. Francolini G, Detti B, Bottero M, Zilli T, Lancia A, Bruni A, et al. Detection rate, pattern of relapse and influence on therapeutic decision of PSMA PET/CT in patients affected by biochemical recurrence after radical prostatectomy, a retrospective case series. Clin Transl Oncol. (2021) 23:364–71. doi: 10.1007/s12094-020-02427-2
54. Fodor A, Lancia A, Ceci F, Picchio M, Hoyer M, Jereczek-Fossa BA, et al. Oligorecurrent prostate cancer limited to lymph nodes: getting our ducks in a row: Nodal oligorecurrent prostate cancer. World J Urol. (2019) 37:2607–13. doi: 10.1007/s00345-018-2322-7
55. Ingrosso G, Trippa F, Maranzano E, Carosi A, Ponti E, Arcidiacono F, et al. Stereotactic body radiotherapy in oligometastatic prostate cancer patients with isolated lymph nodes involvement: a two-institution experience. World J Urol. (2017) 35:45–9. doi: 10.1007/s00345-016-1860-0
56. Kostos L, Murphy DG. Treatment options for metastatic hormone-sensitive prostate cancer. Lancet Oncol. (2022) 23:1234–5. doi: 10.1016/S1470-2045(22)00520-4
57. Garnick MB. Hormonal therapy in the management of prostate cancer: from Huggins to the present. Urology. (1997) 49:5–15. doi: 10.1016/s0090-4295(97)00163-5
58. Fallara G, Robesti D, Nocera L, Raggi D, Marandino L, Belladelli F, et al. Chemotherapy and advanced androgen blockage, alone or combined, for metastatic hormone-sensitive prostate cancer a systematic review and meta-analysis. Cancer Treat Rev. (2022) 110:102441. doi: 10.1016/j.ctrv.2022.102441
59. Kyriakopoulos CE, Chen YH, Carducci MA, Liu G, Jarrard DF, Hahn NM, et al. Chemohormonal therapy in metastatic hormone-sensitive prostate cancer: long-term survival analysis of the randomized phase III E3805 CHAARTED trial. J Clin Oncol. (2018) 36:1080–7. doi: 10.1200/JCO.2017.75.3657
60. James ND, de Bono JS, Spears MR, Clarke NW, Mason MD, Dearnaley DP, et al. Abiraterone for prostate cancer not previously treated with hormone therapy. N Engl J Med. (2017) 377:338–51. doi: 10.1056/NEJMoa1702900
61. Fizazi K, Tran N, Fein L, Rodriguez-Antolin A, Alekseev BY, et al. Abiraterone plus prednisone in metastatic, castration-sensitive prostate cancer. N Engl J Med. (2017) 377:352–60. doi: 10.1056/NEJMoa1704174
62. Davis ID, Martin AJ, Stockler MR, Begbie S, Chi KN, Chowdhury S, et al. Enzalutamide with standard first-line therapy in metastatic prostate cancer. N Engl J Med. (2019) 381:121–31. doi: 10.1056/NEJMoa1903835
63. Chi KN, Agarwal N, Bjartell A, Chung BH, Pereira de Santana Gomes AJ, Given R, et al. Apalutamide for metastatic, castration-sensitive prostate cancer. N Engl J Med. (2019) 381:13–24. doi: 10.1056/NEJMoa1903307
64. Fizazi K, Foulon S, Carles J, Roubaud G, McDermott R, Fléchon A, et al. Abiraterone plus prednisone added to androgen deprivation therapy and docetaxel in de novo metastatic castration-sensitive prostate cancer (PEACE-1): a multicentre, open-label, randomised, phase 3 study with a 2 × 2 factorial design. Lancet. (2022) 399:1695–707. doi: 10.1016/S0140-6736(22)00367-1
65. Ponti E, Lancia A, Ost P, Trippa F, Triggiani L, Detti B, et al. Exploring all avenues for radiotherapy in oligorecurrent prostate cancer disease limited to lymph nodes: A systematic review of the role of stereotactic body radiotherapy. Eur Urol Focus. (2017) 3:538–44. doi: 10.1016/j.euf.2017.07.006
66. Lancia A, Zilli T, Achard V, Dirix P, Everaerts W, Gomez-Iturriaga A, et al. Oligometastatic prostate cancer: The game is afoot. Cancer Treat Rev. (2019) 73:84–90. doi: 10.1016/j.ctrv.2019.01.005
67. Ali A, Hoyle A, Haran ÁM, Brawley CD, Cook A, Amos C, et al. Association of bone metastatic burden with survival benefit from prostate radiotherapy in patients with newly diagnosed metastatic prostate cancer: A secondary analysis of a randomized clinical trial. JAMA Oncol. (2021) 7:555–63. doi: 10.1001/jamaoncol.2020.7857
68. Oderinde OM, Shirvani SM, Olcott PD, Kuduvalli G, Mazin S, Larkin D. The technical design and concept of a PET/CT linac for biology-guided radiotherapy. Clin Transl Radiat Oncol. (2021) 29:106–12. doi: 10.1016/j.ctro.2021.04.003
69. Wong J, Liu B, Dandapani S, Li Y, Glaser S, Liu J, et al. A prospective pilot study of the refleXion X1 PET-CT subsystem imaging performance with [18F]-DCFPyL PSMA in patients with prostate cancer. JNM abstract. (2023). https://jnm.snmjournals.org/content/64/supplement_1/P521.abstract.
70. Bauman GS, Corkum MT, Fakir H, Nguyen TK, Palma DA. Ablative radiation therapy to restrain everything safely treatable (ARREST): study protocol for a phase I trial treating polymetastatic cancer with stereotactic radiotherapy. BMC Cancer. (2021) 21:405. doi: 10.1186/s12885-021-08020-2
71. Fass L. Imaging and cancer: a review. Mol Oncol. (2008) 2:115–52. doi: 10.1016/j.molonc.2008.04.001
72. Aerts HJ. The potential of radiomic-based phenotyping in precision medicine: A review. JAMA Oncol. (2016) 2:1636–42. doi: 10.1001/jamaoncol.2016.2631
73. Liu X, Tian J, Wu J, Zhang Y, Wang X, Zhang X, et al. Utility of diffusion weighted imaging-based radiomics nomogram to predict pelvic lymph nodes metastasis in prostate cancer. BMC Med Imaging. (2022) 22:190. doi: 10.1186/s12880-022-00905-3
74. Mattoni S, Farolfi A, Formaggio F, Bruno G, Caroli P, Cerci JJ, et al. PSMA PET for the evaluation of liver metastases in castration-resistant prostate cancer patients: A multicenter retrospective study. Cancers (Basel). (2022) 14:5680. doi: 10.3390/cancers14225680
75. Kairemo K, Kangasmäki A, Kappadath SC, Joensuu T, Macapinlac HA. A retrospective comparative study of sodium fluoride na18F-PET/CT and 68Ga-PSMA-11 PET/CT in the bone metastases of prostate cancer using a volumetric 3-D radiomic analysis. Life (Basel). (2022) 12:1977. doi: 10.3390/life12121977
76. Assadi M, Manafi-Farid R, Jafari E, Keshavarz A, Divband G, Moradi MM, et al. Predictive and prognostic potential of pretreatment 68Ga-PSMA PET tumor heterogeneity index in patients with metastatic castration-resistant prostate cancer treated with 177Lu-PSMA. Front Oncol. (2022) 12:1066926. doi: 10.3389/fonc.2022.1066926
77. Hou Y, Bao J, Song Y, Bao ML, Jiang KW, Zhang J, et al. Integration of clinicopathologic identification and deep transferrable image feature representation improves predictions of lymph node metastasis in prostate cancer. EBioMedicine. (2021) 68:103395. doi: 10.1016/j.ebiom.2021.103395
78. Alongi P, Stefano A, Comelli A, Laudicella R, Scalisi S, Arnone G, et al. Radiomics analysis of 18F-choline PET/CT in the prediction of disease outcome in high-risk prostate cancer: an explorative study on machine learning feature classification in 94 patients. Eur Radiol. (2021) 31:4595–605. doi: 10.1007/s00330-020-07617-8
79. Moazemi S, Khurshid Z, Erle A, Lütje S, Essler M, Schultz T, et al. Machine learning facilitates hotspot classification in PSMA-PET/CT with nuclear medicine specialist accuracy. Diagnostics. (2020) 10:622. doi: 10.3390/diagnostics10090622
80. Zhang W, Mao N, Wang Y, Xie H, Duan S, Zhang X, et al. A radiomics nomogram for predicting bone metastasis in newly diagnosed prostate cancer patients. Eur J Radiol. (2020) 128:109020. doi: 10.1016/j.ejrad.2020.109020
81. Cysouw MCF, Jansen BHE, van de Brug T, Oprea-Lager DE, Pfaehler E, de Vries BM, et al. Machine learning-based analysis of [18F]DCFPyL PET radiomics for risk stratification in primary prostate cancer. Eur J Nucl Med Mol Imaging. (2021) 48:340–9. doi: 10.1007/s00259-020-04971-z
82. Wang Y, Yu B, Zhong F, Guo Q, Li K, Hou Y, et al. MRI-based texture analysis of the primary tumor for pre-treatment prediction of bone metastases in prostate cancer. Magn Reson Imaging. (2019) 60:76–84. doi: 10.1016/j.mri.2019.03.007
83. Lin C, Harmon S, Bradshaw T, Eickhoff J, Perlman S, Liu G, et al. Response-to-repeatability of quantitative imaging features for longitudinal response assessment. Phys Med Biol. (2019) 64:025019. doi: 10.1088/1361-6560/aafa0a
84. Zhao Y, Gafita A, Vollnberg B, Tetteh G, Haupt F, Afshar-Oromieh A, et al. Deep neural network for automatic characterization of lesions on 68Ga-PSMA-11 PET/CT. Eur J Nucl Med Mol Imaging. (2020) 47:603–13. doi: 10.1007/s00259-019-04606-y
85. Reischauer C, Patzwahl R, Koh D-M, Froehlich JM, Gutzeit A. Texture analysis of apparent diffusion coefficient maps for treatment response assessment in prostate cancer bone metastases—A pilot study. Eur J Radiol. (2018) 101:184–90. doi: 10.1016/j.ejrad.2018.02.024
86. Khurshid Z, Ahmadzadehfar H, Gaertner FC, Papp L, Zsóter N, Essler M, et al. Role of textural heterogeneity parameters in patient selection for 177Lu-PSMA therapy. via response prediction Oncotarget. (2018) 9:33312–21. doi: 10.18632/oncotarget.26051
87. Perk T, Bradshaw T, Chen S, Im HJ, Cho S, Perlman S, et al. Automated classification of benign and Malignant lesions in 18 F-naF PET/CT images using machine learning. Phys Med Biol. (2018) 63:225019. doi: 10.1088/1361-6560/aaebd0
88. Kerkmeijer LGW, Groen VH, Pos FJ, et al. Focal boost to the intraprostatic tumor in external beam radiotherapy for patients with localized prostate cancer: results from the FLAME randomized phase III trial. J Clin Oncol. (2021) 39:787–96. doi: 10.1200/JCO.20.02873
Keywords: radiotherapy, prostate cancer, PET/CT, biology-guided radiotherapy, metastases
Citation: Lancia A, Ingrosso G, Detti B, Festa E, Bonzano E, Linguanti F, Camilli F, Bertini N, La Mattina S, Orsatti C, Francolini G, Abenavoli EM, Livi L, Aristei C, de Jong D, Al Feghali KA, Siva S and Becherini C (2024) Biology-guided radiotherapy in metastatic prostate cancer: time to push the envelope? Front. Oncol. 14:1455428. doi: 10.3389/fonc.2024.1455428
Received: 26 June 2024; Accepted: 19 August 2024;
Published: 09 September 2024.
Edited by:
Neal Shore, GenesisCare, United StatesReviewed by:
Borghetti Paolo, Civil Hospital of Brescia, ItalyCopyright © 2024 Lancia, Ingrosso, Detti, Festa, Bonzano, Linguanti, Camilli, Bertini, La Mattina, Orsatti, Francolini, Abenavoli, Livi, Aristei, de Jong, Al Feghali, Siva and Becherini. This is an open-access article distributed under the terms of the Creative Commons Attribution License (CC BY). The use, distribution or reproduction in other forums is permitted, provided the original author(s) and the copyright owner(s) are credited and that the original publication in this journal is cited, in accordance with accepted academic practice. No use, distribution or reproduction is permitted which does not comply with these terms.
*Correspondence: Andrea Lancia, YW5kcmVhLmxhbmNpYS5kckBnbWFpbC5jb20=; Dorine de Jong, ZGRlam9uZzk5MEBnbWFpbC5jb20=
Disclaimer: All claims expressed in this article are solely those of the authors and do not necessarily represent those of their affiliated organizations, or those of the publisher, the editors and the reviewers. Any product that may be evaluated in this article or claim that may be made by its manufacturer is not guaranteed or endorsed by the publisher.
Research integrity at Frontiers
Learn more about the work of our research integrity team to safeguard the quality of each article we publish.