- 1Pharmacy Department, General Hospital of Ningxia Medical University, Yinchuan, Ningxia Hui Autonomous Region, China
- 2College of Pharmacy, Ningxia Medical University, Yinchuan, Ningxia Hui Autonomous Region, China
- 3Key Laboratory of Ningxia Minority Medicine Modernization Ministry of Education, Ningxia Medical University, Yinchuan, Ningxia Hui Autonomous Region, China
- 4Department of Chinese Medical Gastrointestinal, China-Japan Friendship Hospital, Beijing, China
Lonicerae Japonicae Flos (LJF, called Jinyinhua in China), comes from the dried flower buds or flowers to be opened of Lonicera japonica Thunb. in the Lonicera family. It has a long history of medicinal use and has a wide range of application prospects. As modern research advances, an increasing number of scientific experiments have demonstrated the anticancer potential of LJF. However, there is a notable absence of systematic reports detailing the anti-tumor effects of LJF. This review integrates the principles of Traditional Chinese Medicine (TCM) with contemporary pharmacological techniques, drawing upon literature from authoritative databases such as PubMed, CNKI, and WanFang to conduct a comprehensive study of LJF. Notably, a total of 507 compounds have been isolated and characterized from the plant to date, which include volatile oils, organic acids, flavonoids, iridoids, triterpenes and triterpenoid saponins. Pharmacological studies have demonstrated that LJF extract, along with components such as chlorogenic acid, luteolin, rutin, luteoloside, hyperoside and isochlorogenic acid, exhibits potential anticancer activities. Consequently, we have conducted a comprehensive review and summary of the mechanisms of action and clinical applications of these components. Furthermore, we have detailed the pharmacokinetics, quality control, and toxicity of LJF, while also discussing its prospective applications in the fields of biomedicine and preventive healthcare. It is hoped that these studies will provide valuable reference for the clinical research, development, and application of LJF.
1 Introduction
Cancer, a significant global public health challenge, continues to impose a substantial burden on human lives. According to the International Agency for Research on Cancer (IARC), the number of new cases worldwide in 2020 has risen to 19.3 million, with an estimated 10 million deaths resulting from it (1). Among these, breast, lung, colorectal, prostate, and stomach cancers are responsible for the highest number of cancer-related deaths. Cancer has emerged as the primary cause of mortality, significantly hindering the improvement of life expectancy. Despite the availability of diverse treatment options such as surgery, radiotherapy, chemotherapy, immunotherapy, and targeted therapy, the efficacy of these treatments remains inadequate, with challenges like toxic side effects, drug resistance, tumor recurrence, and metastasis (2). Thus, there is an urgent necessity to explore and develop novel therapeutic approaches and medications to more effectively combat cancer and safeguard human health.
In recent years, numerous complementary and alternative therapies for cancer treatment have emerged. Among these, Traditional Chinese Medicine (TCM) has gained prominence as a significant option due to its low toxicity, safety, and high efficacy (3, 4). As a core component of TCM, Chinese herbs with medicinal and edible value (5, 6), offer advantages such as multi-component, multi-target, and multi-pathway anti-tumor effects. Furthermore, they can integrate medicinal properties with nutritional benefits, thereby providing a unique approach to food therapy that aims to restore the balance of Yin and Yang in the body and enhance overall resistance. Currently, the extracts and active monomer components from various medicinal and edible Chinese herbs have demonstrated anticancer effects. Their integration with surgical resection, chemotherapy, radiotherapy, and targeted therapy not only enhances the efficacy of drug therapy, but also effectively mitigates multi-drug resistance and reduces adverse reactions, thereby significantly improving the quality of life for patients (7). Overall, the role of medicinal and edible herbs in cancer treatment is becoming increasingly significant, offering patients more treatment options and hope.
Lonicerae Japonicae Flos (LJF) is a renowned Chinese herbal medicine, first documented in Shen Nong’s Herbal Classic (Shennong Bencao Jing), where it is classified as a top-quality herb. LJF has a sweet and cold taste and is known for its functions in clearing heat and detoxification, as well as antibacterial and anti-inflammatory. Clinically, it is frequently used to treat conditions such as carbuncles, pustules, throat pain, erysipelas, heat-induced blood dysentery, colds caused by wind heat, and fevers. Due to its therapeutic properties, it has earned the reputation of being a Chinese medicine antibiotic (8, 9).
To date, 507 compounds have been isolated and identified from LJF, including volatile oils, organic acids, flavonoids, iridoids, triterpenes and triterpenoid saponins. Modern pharmacological studies have demonstrated that LJF exhibits a range of effects, including antibacterial, anti-inflammatory, antiviral, liver-protective, intestinal-regulating, anti-depressive, antioxidant, anti-allergic, hypolipidemic, hypoglycemic, and immune-regulatory properties (10–12). Notably, LJF has also shown significant anti-tumor activity. Numerous studies indicate that LJF extract and its active components, such as chlorogenic acid, luteolin, rutin, luteoloside, hyperoside and isochlorogenic acid, exhibit inhibitory effects on various cancers, including liver (13), pancreatic (14), and lung cancers (15). Its mechanism of action mainly includes inhibiting cell proliferation, inducing cell apoptosis, blocking cell cycle, inhibiting cell metastasis, regulating inflammation and immune function, and activating related signaling pathways. In clinical practice, LJF is frequently utilized as an adjunct in cancer treatment to mitigate the side effects of therapies and enhance therapeutic efficacy. Furthermore, in the biomedical field, LJF is also employed in the production of nanoparticles and in the research and development of photosensitizers, thereby expanding its potential applications in cancer treatment.
While previous reviews have highlighted the anti-tumor properties of LJF, a comprehensive systematic review examining the specific anti-tumor effects of LJF extract and its active components has yet to be conducted. Therefore, this review systematically summarizes the mechanisms of action and clinical applications of LJF extract and its active components across various cancers, drawing upon the principles of TCM and modern pharmacological techniques. Additionally, the review analyzes pharmacokinetics, toxicology, and quality control, identifying the limitations associated with the clinical application of LJF. Furthermore, this paper discusses the potential value of LJF in the biomedical field and in preventive health care, aiming to promote its broader application and development in anti-tumor therapies.
2 Anti-cancer theory of TCM
TCM has a long-standing history in cancer treatment, with its fundamental therapeutic principle centered on enhancing the body’s resistance and eliminating pathogenic factors (16). In the following sections, I will elaborate on LJF’s TCM anti-cancer theory from these two perspectives and explore its compatibility with contemporary medical theories.
2.1 Strengthening body resistance
According to the Huangdi Neijing (The Yellow Emperor’s Inner Classic), TCM posits that the development of cancer is closely associated with a deficiency of healthy qi and the invasion of pathogenic factors within the human body. The intrusion of pathogenic factors disrupts the delicate balance of Yin and Yang, leading to dysfunction in the zang-fu organs and impairing the flow of Qi-Blood-Body fluid, which can result in various pathological changes throughout the body or in specific localized areas. Therefore, the principle of TCM (17, 18) in the treatment of cancer focuses on strengthening the body’s foundational health, promoting blood circulation, and removing blood stasis. This approach aims to achieve a balance between Yin and Yang, enhance the body’s resistance, and suppress tumor growth.
Modern medicine posits that immunity plays a crucial role in enhancing the body’s resistance and maintaining its relative stability. The development of cancer is closely associated with a diminished function of the human immune system (19). Consequently, the deficiency of healthy qi in TCM is theoretically regarded as analogous to low immunity in modern medicine. The TCM concept of strengthening healthy qi to eliminate pathogenic factors is highly related to the strengthening of immunity emphasized by modern medicine. Recent pharmacological studies have demonstrated (20) that LJF can modulate the body's immune function through various targets and pathways, thereby enhancing the ability to resist cancer invasion. Therefore, the principles of strengthening health qi and eliminating pathogenic factors, which are emphasized in TCM, can serve as a guiding theory for the anticancer effects of LJF.
2.2 Clearing heat and detoxification, eliminating pathogenic factors
According to the theory of TCM, cancer is considered a disease resulting from the accumulation of heat toxins and the stasis of qi and blood within the body. Similar to inflammatory factors, pathogenic heat and toxins are implicated in the etiology of cancer and can facilitate its progression. As a traditional Chinese herbal medicine, LJF exhibits numerous pharmacological effects, including antioxidant, anti-inflammatory, antibacterial, and antiviral properties, which are linked to its heat-clearing and detoxifying capabilities (21, 22).
In clinical practice, patients with middle to advanced stages of cancer frequently experience symptoms such as localized lumps and burning pain. Consequently, a primary treatment approach involves the use of heat-clearing and detoxifying medications (23–25). By effectively eliminating heat and toxins from the body, these treatments can significantly alleviate the pain experienced by cancer patients. This approach not only aligns with the theoretical principles of TCM but also supports its practical application in clinical settings.
3 Network pharmacological analysis
To explore the link between LJF and cancer, we conducted an exhaustive search of the active ingredients and their associated targets with the help of Traditional Chinese Medicine Systems Pharmacology Database and Analysis Platform (TCMSP) (https://old.tcmsp-e.com/tcmsp.php). The active ingredients were required to satisfy two key conditions: oral bioavailability (OB) ≥ 30% and drug likeness (DL) ≥ 0.18. Then we used the Uniprot database (https://www.uniprot.org/) to standardize the target names. Finally, the drug-component-target network diagram was constructed by Cytoscape 3.9.0 software, and Kyoto Encyclopedia of Genes and Genomes (KEGG) enrichment analysis was performed using the DAVID database (https://david.ncifcrf.gov/). The top 30 key signaling pathways were screened based on p-values and histograms were plotted with the help of the bioinformatics online platform. The smaller the p-value, the more significant the enrichment of the pathway.
As shown in Figure 1, after screening, we obtained 23 active ingredients from LJF, involving 199 targets. After KEGG analysis, we successfully enriched 176 signaling pathways, among which 7 of the top 30 signaling pathways were closely related to tumors.
4 Active ingredients
In recent years, comprehensive studies on the active components of LJF have elucidated its unique pharmacological effects and potential applications. Phytochemical analyses have identified various bioactive compounds in LJF, including volatile oils, organic acids, flavonoids, iridoids, triterpenes and triterpenoid saponins (26, 27). These active ingredients form a robust basis for the medicinal effects attributed to LJF. The specific chemical structure formula is shown in Figure 2.
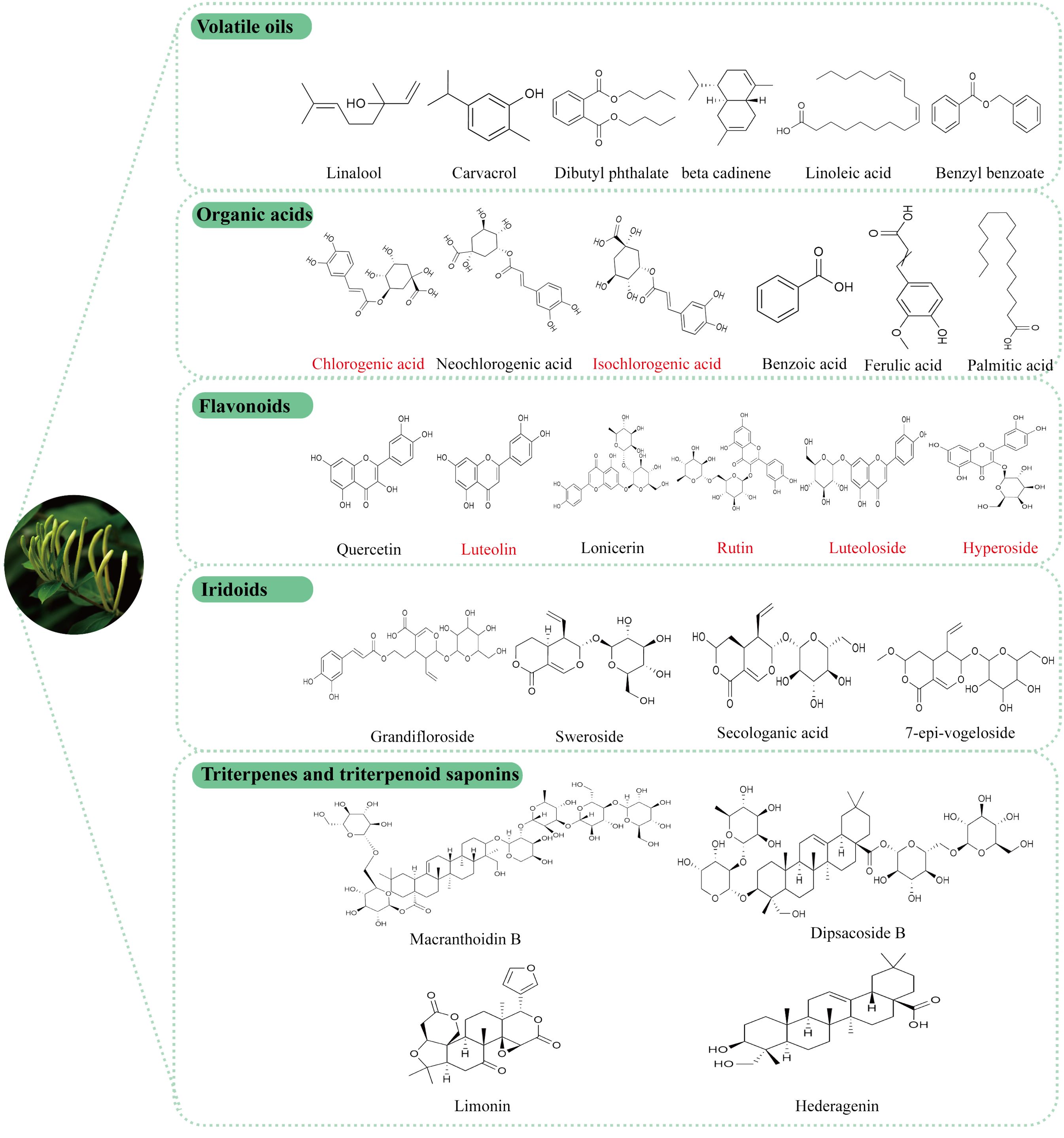
Figure 2. Structural formula of the active components of LJF, the red label is the main representative component of LJF’s anti-tumor effect.
4.1 Volatile oils
LJF, known for its distinct aromatic scent, contains a variety of volatile oil components, including acids, aldehydes, alcohols, ketones, and their esters. These components may vary depending on geographical location. Through detailed identification (28, 29), it has been found that in the Ningxia Province region of China, the three main volatile oil components in LJF are linalool (13.59%), carvacrol (7.67%), and dibutyl phthalate (7.54%), all of which have demonstrated anticancer properties (30–32). In Hunan Province (33), the primary components of LJF consist of n-hexadecanoic acid, linoleic acid, and α-curcumene. Linoleic acid, an essential nutrient, not only enhances anti-tumor immunity (34), but also plays a role in regulating glucose homeostasis.
4.2 Organic acids
The common organic acids, including chlorogenic acid, neochlorogenic acid, and isochlorogenic acid, have been identified to possess various pharmacological activities such as anti-inflammatory (35), anti-tumor (36), antibacterial (37), antioxidant (38), hypoglycemic (39), neuroprotective (40). Additionally, a novel organic acid compound, (2S,7R,2′R)-2-(3-hydroxy-5-methyl-4-oxo-3,4-dihydro-2H-pyran-6-yl)-3-((2-hydroxypropanoyl) oxy) propanoic acid, has recently been discovered to exhibit hepatoprotective effects (41).
4.3 Flavonoids
Flavonoids are compounds found in various herbal plants and have been shown to exhibit a range of biological activities that offer protective effects on different organs (42). Quercetin, luteolin, luteoloside, hyperoside, lonicerin, and rutin, which are extracted from LJF, are examples of flavonoids with proven antibacterial (43), anti-inflammatory (44), antioxidant (45), immune-regulating (46) and anticancer activities (47). The concentration of flavonoids in LJF is closely linked to its growth stage (48), with studies indicating that the content initially increases, then decreases, peaking at the white alabastrum stage (49). Furthermore, research has demonstrated that luteoloside specifically possesses antidepressant properties (50).
4.4 Iridoids
Iridoids are the most abundant compounds in LJF, with secoiridoid glycosides making up more than half of them. These include secologanic acid, sweroside, and loniaceticiridoside. Sweroside has been found to partially reduce hepatic steatosis by activating AMPK/mTOR-mediated autophagy in mice (51), as well as protect against LPS-induced ALI by suppressing inflammation (52). Recent studies have shown that secoiridoid glucosides and their derivatives extracted from LJF buds, such as secoxyloganin and dimethylsecol-ologanoside, have inhibitory effects against influenza A (53). Additionally, two new iridoids were isolated from LJF leaves by Yu J et al. (41), namely (1R,5R,9S)-5-epi-sweroside and rel-(1R,5R,9R)-1-O-β-D-glucopyranosyl-9-vinyl-2-oxabicyclo[4.3.0]non-3-en-11-one. (1R,5R,9S)-5-epi-sweroside showed weaker antioxidant activity than quercetin and a slightly lower hepatoprotective effect than magnesium isoglycyrrhizinate.
4.5 Triterpenes and triterpenoid saponins
More than 35 triterpenoids and triterpenoid saponins have been identified in LJF (54). Ursolic acid has been found to enhance the antitumor effects of sorafenib in human cancers through mechanisms related to Mcl-1-dependent apoptosis and SLC7A11-related iron apoptosis (55). In vitro studies have shown that hederagenin can inhibit ovarian cancer cell proliferation by modulating mitochondrial translocation and apoptosis (56). Daucosterol has been reported to induce autophagy-dependent apoptosis in prostate cancer cells via activation of the c-Jun N-terminal kinase (JNK) signaling pathway (57). Additionally, loniceroside A, loniceroside B, loniceroside C, loniceroside D, and loniceroside E (58, 59), all classified as saponins, with loniceroside C demonstrating significant anti-inflammatory activity in a croton oil-induced ear edema assay in mice (60).
5 Anti-tumor mechanism
Over the past few decades, researchers have concentrated on investigating the effects of LJF and its active compounds on various cancers. The comprehensive study of LJF extract and its active components is crucial for elucidating the anti-tumor mechanisms associated with this plant. As research has progressed, the anti-tumor mechanisms of LJF have gradually been unveiled. Numerous studies indicate that LJF extract, along with its active ingredients such as chlorogenic acid, luteolin, rutin, luteoloside, hyperoside and isochlorogenic acid, possesses the capability to combat various malignant tumors (Table 1). The mechanisms of action include inhibiting cancer cell proliferation, blocking the cell cycle, inducing apoptosis, and inhibiting cell migration. Additionally, LJF also has anti-inflammatory, regulating immune function, autophagy, and activating related signaling pathways. The specific action pathways and molecular mechanisms are illustrated in Figures 3 and 4.
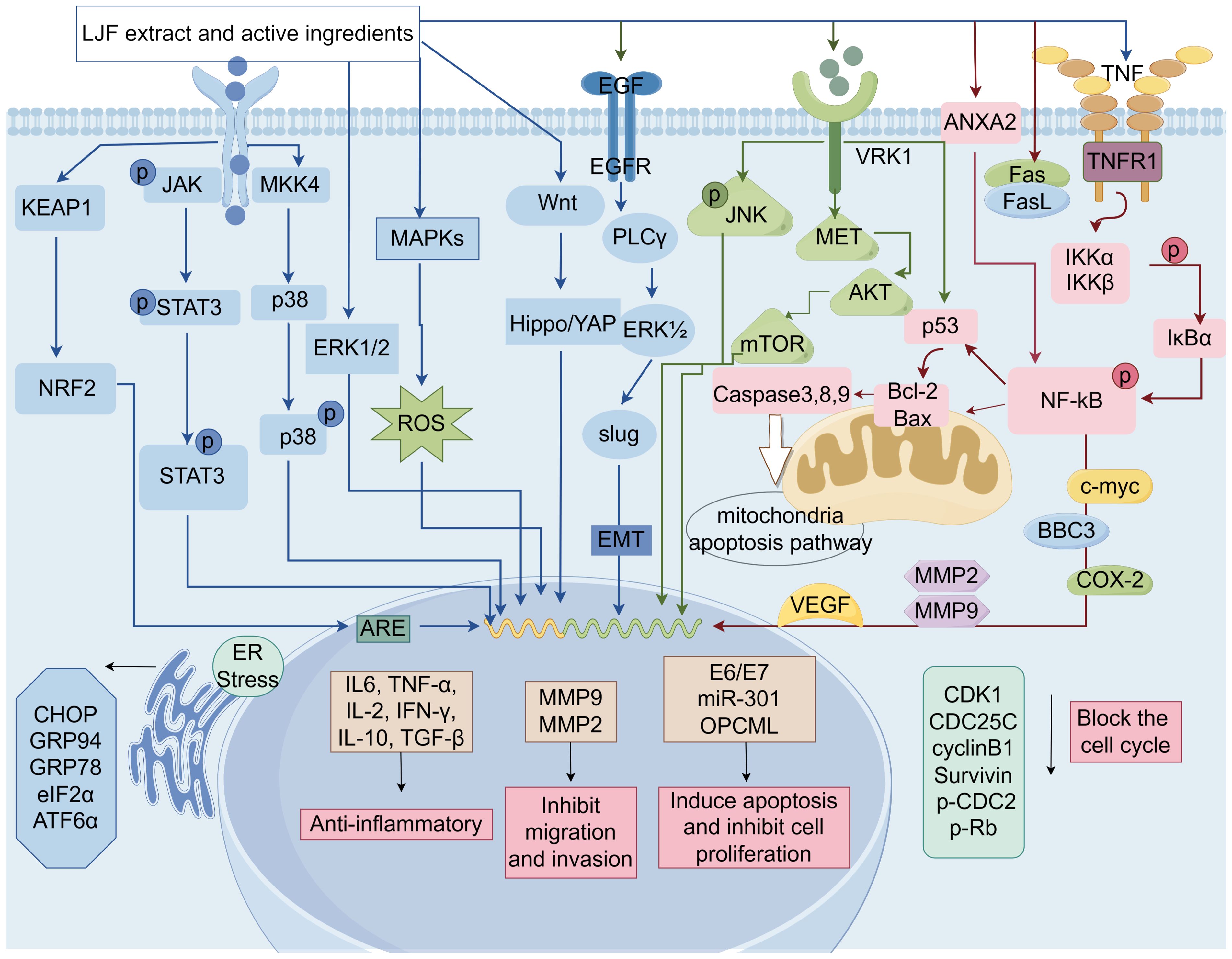
Figure 4. The molecular mechanisms of LJF and its active compounds for cancer treatment (Created via Figdraw).
5.1 Inhibition of cancer cell proliferation
Cancer arises from the abnormal proliferation and differentiation of normal cells, influenced by both internal and external factors. This uncontrolled proliferation is a defining characteristic of tumor cells and is intrinsically linked to the cancer development process. Consequently, an essential aspect of cancer treatment is the effective inhibition of the excessive growth and proliferation of tumor cells (92, 93).
Several studies have shown that LJF extract and its active ingredients have the ability to exert anti-cell proliferation in specific cancers. Chlorogenic acid (80) regulates cell proliferation, apoptosis and tumor-microenvironment by down-regulating the expression of RTK ligands and inflammatory factors, and has shown outstanding therapeutic effect on breast cancer. In addition (94), chlorogenic acid binds to annexin A2, resulting in decreased expression of downstream NF-κB anti-apoptotic gene, thereby inhibiting the growth of human lung cancer A549 cells. In addition, chlorogenic acid (95) has the function of activating ERK1/2. This mechanism plays a significant inhibitory role in the proliferation of osteosarcoma U2OS cells.
Han et al. demonstrated that luteolin inhibits the proliferation and induces apoptosis of prostate cancer (PCa) cells by triggering DEDD2 expression and down-regulating miR-301. As a prognostic marker, miR-301 may represent a novel approach for PCa treatment (96). Furthermore, luteolin (97) has been shown to reduce the expression level of VRK1, activate the p53 signaling pathway, induce G2/M cell apoptosis and cell cycle arrest, and effectively inhibit the proliferation of high-grade serous ovarian cancer (HGSOC) cells. More importantly, luteolin (98) was found to enhance the expression of the OPCML gene and promote its demethylation process, which contributes to the inhibition of proliferation in breast cancer MDA-MB-231 cells. The OPCML gene has been recognized by the scientific community as a crucial tumor suppressor gene, essential for maintaining normal cell function, differentiation, and development. Its abnormal expression can lead to uncontrolled cell growth.
Luteolin has been shown to possess significant pharmacological effects in the diagnosis and treatment of nasopharyngeal carcinoma. It can precisely regulate both exogenous and endogenous apoptotic pathways, as well as AKT signal transduction pathways, thereby effectively inhibiting the proliferation and inducing apoptosis in nasopharyngeal carcinoma cell lines, such as NPC-039 and NPC-BM (75). Furthermore, this compound also can reduce mitochondrial membrane potential and diminish the production of reactive oxygen species, subsequently activating caspase-3 and caspase-8 (83). This activation alters the nuclear morphology of cells and causes DNA damage. Collectively, these effects demonstrate a pronounced inhibitory impact of luteolin on HeLa cell proliferation in cervical cancer and initiate the apoptotic process.
The exosomal miR2911 derived from LJF (84) has been reported to exhibit significant antitumor effects. Its mechanism of action involves targeting the E6/E7 genes of HPV16/18, thereby effectively inhibiting the proliferation of cervical cancer cells. Concurrently, experimental results demonstrated that at a concentration of 1 mg/mL, the polysaccharide extract of LJF (14) significantly inhibited the growth of BxPC-3 and PANC-1 pancreatic cancer cells, with inhibition rates reaching 66.7% and 52.1%, respectively. These findings not only provide robust evidence for the anti-proliferative properties of LJF extract but also suggest new directions and ideas for the development of novel therapeutic strategies.
5.2 Induction of cell cycle arrest
The cell cycle is the process through which cells progress from the completion of one division to the end of the next, and it is divided into two main phases: interphase and division. The progression of the cell cycle is primarily regulated by cyclin-dependent kinases (CDKs), cyclins, and endogenous CDK inhibitors (CKIs), which interact to influence cell proliferation, growth, and repair. Regulation of the cell cycle is a complex and delicate process. any abnormalities can lead to uncontrolled cell proliferation and the development of cancer (99, 100). Consequently, adjusting cell cycle distribution and inducing cell cycle arrest are considered effective strategies for cancer treatment.
A study indicated that LJF polyphenol extract (64) induced apoptosis in hepatocellular carcinoma cells by decreasing the expression of CDK1, CDC25C, and Cyclin B1, thereby obstructing the cell cycle at the G2/M phase. When compared to control data (12.55 ± 3.14%), treatment with 600 and 800 µg/ml of polyphenol extract significantly increased the percentage of cells in the G2/M phase to 25.37 ± 1.67% and 27.13 ± 2.12%, respectively.
An experimental study demonstrated that rutin mediates cell death in human cervical cancer Caski cells by down-regulating the expression levels of CDK4, Cyclin D1, Notch-1, and Hes-1, effectively blocking the cell cycle at the G0/G1 phase (101). Furthermore, Huang et al. found (102) that luteolin inhibited the cell cycle in the S phase and induced apoptosis in breast cancer MDA-MB-231 cells by down-regulating the expression of hTERT, Cyclin D1, and Survivin, while simultaneously increasing the expression level of p21. The proposed mechanism of action may involve the inhibition of the NF-κB-c-Myc axis, leading to reduced hTERT expression. Additionally, luteolin has the capacity to modulate mitochondrial function, thereby facilitating apoptotic cell death. Cai et al. reported (103) that luteolin treatment of lung cancer A549 cells resulted in a significant increase in the proportion of G2 phase cells and a marked decrease in the expression of G2 phase cell cycle-related proteins (cyclin A, p-CDC2, and p-Rb), ultimately inducing apoptotic cell death.
Moreover, Lei et al. found (77) that cynaroside significantly inhibited colorectal cancer cell proliferation and colony formation in vitro by down-regulating CDC25A, which induced G1 cell cycle arrest. The inhibitory effect of the active ingredient luteoloside on the growth of liver cancer cells is mainly due to G2/M phase arrest and reactive oxygen species (ROS) production. In addition, luteoloside also increased the phosphorylation of JNK (65).
5.3 Induction of tumor cell apoptosis
Apoptosis (104) is a mode of programmed death that is genetically regulated and can be involved in a variety of pathological processes. During cell death, significant changes in cell morphology occur. These changes include reduction in cell size, fragmentation of the nucleus, and the appearance of eosinophilic vesicles in the cytoplasm. These features can be used to identify the type of apoptosis (105, 106). Apoptosis has long been recognized as an important mechanism for preventing the emergence of tumors. Therefore, killing cancer cells through the apoptotic pathway has been the mainstay of clinical cancer therapy.
In recent years, numerous studies have focused on inducing apoptosis by targeting apoptosis-related genes in the pursuit of improved cancer treatments. The anti-apoptotic protein Bcl-2 and the pro-apoptotic protein Bax are pivotal regulatory genes in the apoptosis process, as they regulate the release of substances such as cytochrome C through the mitochondrial pathway, thereby mediating cell survival or death. Consequently, effectively targeting the pro-apoptotic effects of Bcl-2 family members may represent a promising strategy for cancer therapy.
Qi et al. (107) found that LJF polysaccharide extract promoted apoptosis in triple-negative breast cancer cells by regulating the Bcl-2/Bax ratio. Hyperoside can inhibit the proliferation and promote apoptosis of human pancreatic cancer cell lines PANC-1 and BxPC-3 by up-regulating the Bax/Bcl-2 and Bcl-xl ratios, and down-regulating NF-κB as well as survivin, c-Myc, cyclin D1, and COX-2 (108). In particular, NF-κB plays an important role in regulating the level or localization of Bcl-2 family proteins in cells. This was confirmed in the study of chlorogenic acid against liver cancer in vitro. The specific mechanism involves chlorogenic acid (109) activating the mitochondrial apoptotic pathway in Hep-G2 and Ha-7 cells by inhibiting the non-classical NF-κB signaling pathway and up-regulating BBC3, a member of the BH3-only pro-apoptotic subclass. The BH3 domain is regarded as an essential structural component in the apoptosis process, playing a significant role in pro-apoptotic activity.
All apoptotic signaling pathways have been reported to be activated by cysteinyl aspartate-specific proteinase (110). These enzymes function by specifically targeting cysteine residues in proteins and cleaving peptide bonds following aspartic acid residues. Consequently, the activation of caspase to induce apoptosis has emerged as a significant strategy in tumor therapy. Through a literature search, we identified various active ingredients in LJF that possess the potential to activate caspase. hyperoside and rutin (111) have been shown to induce apoptosis in HT-29 human colon cancer cells via the activation of cleaved caspase-3, caspase-8, and caspase-9. This phenomenon is mediated through the mitochondrial intrinsic apoptosis pathway, offering novel insights for cancer therapy. Additionally, an in vivo study revealed (81) that treatment of 4T1 breast cancer mice with chlorogenic acid significantly increased caspase-3 gene expression, highlighting a key mechanism underlying the pro-apoptotic effect of chlorogenic acid.
The aforementioned studies have demonstrated that LJF extract and its active ingredients possess the potential to activate the mitochondrial intrinsic apoptotic pathway, exhibiting significant therapeutic promise in cancer treatment. Notably, apoptosis occurs through two primary signaling pathways, the extrinsic/death receptor pathway and the intrinsic/mitochondrial pathway. It has been reported that hyperoside (112) effectively induces apoptosis in human thyroid squamous cell carcinoma SW579 by upregulating the expression of Fas and FasL mRNA while downregulating the expression of survivin. This outcome is partly attributed to the activation of the extrinsic death receptor pathway following the binding of Fas and FasL.
Recent studies have emphasized that oxidative stress-mediated apoptosis represents a promising therapeutic strategy for targeting cancer cells (113). Elevated levels of ROS play a significant role in the induction of apoptosis. In colon cancer investigations, luteolin (114) has been shown to induce apoptotic cell death by enhancing the antioxidant activity of human colon cancer HT-29 cells. The specific mechanisms involved include ROS scavenging and the activation of the MAPK signaling pathway. Meanwhile, Japoflavone D (63), extracted from LJF buds, effectively mitigates cellular damage caused by excessive ROS through the activation of the KEAP1/NRF2/ARE signaling axis, thereby regulating apoptosis. Furthermore, numerous studies have demonstrated that ROS levels correlate with endoplasmic reticulum stress. Notably, Wang et al. showed that (115) luteolin induced lethal stress and ultimately apoptosis in glioblastoma cells by increasing ROS levels within the endoplasmic reticulum.
The endoplasmic reticulum is a crucial organelle within the cell that facilitates the proper folding of newly synthesized proteins through processes such as methylation, hydroxylation, lipidation, and the formation of disulfide bonds (116). However, when a significant accumulation of unfolded or misfolded proteins occurs in the lumen of the endoplasmic reticulum, a cellular stress response known as endoplasmic reticulum stress (ERS) is activated. In response to this stress, the cell initiates the unfolded protein response (UPR). Under conditions of normal homeostasis, the UPR serves as an adaptive response program. However, when the level of stress surpasses the adaptive capacity of the UPR, apoptosis is triggered. Consequently, the precise regulation of ERS is of paramount importance, as it directly influences cell survival or death.
In a recent study, we demonstrated that luteolin (117) derived from LJF induce the upregulation of the UPR pathway through ERS sensors, thereby contributing to the regulation of the apoptotic pathway in adrenal medullary pheochromocytoma PC12 cells. Furthermore, luteolin (118) plays a role in regulating the expression of ERS-associated proteins, including CHOP, GRP94, and GRP78, as well as the cleavage of ATF6α and the phosphorylation of eIF2α. These regulatory processes ultimately trigger ERS, leading to the induction of apoptosis.
5.4 Inhibition of cell metastasis
Cancer metastasis, defined as the dissemination of cancer cells from the primary tumor to adjacent tissues and distant organs, is a critical factor in cancer progression and patient mortality (119). Research has identified several mechanisms that significantly contribute to tumor cell migration and invasion, including epithelial-mesenchymal transition (EMT), tumor angiogenesis, the initiation of an inflammatory tumor microenvironment, and apoptosis (120–122). Consequently, inhibiting these pathways may effectively impede the metastasis of cancer cells and slow tumor progression.
Metastasis is a complex process that involves several stages, including invasiveness, introgression, extravasation, and growth in distant organs. Tumor invasion through the extracellular matrix (ECM) is recognized as a critical stage in this progression. Several matrix metalloproteinases, such as MMP-2 and MMP-9, are believed to play direct roles in the migration, invasion, and metastasis of tumor cells, and they are associated with various prognostic factors. Among the active monomer components of LJF, luteolin and luteoloside have demonstrated anti-metastatic effects. Luteolin (123) acts as an anti-metastatic agent by inhibiting the production of MMP-9 and MMP-2, while luteoloside (90) regulates the expression of MMP-2 in human oral squamous cell carcinoma, including FaDu, HSC-3, and CA9-22, and inhibits cell migration and invasion. Additionally, In addition, the ethanol extract mixture of LJF (50μg/mL) also inhibited the migration and invasion of melanoma cells (124). It was found to significantly reduce melanoma cell viability by increasing miR-let-7a/f levels and decreasing the expression of CCR7, MMP-2, MMP-9, p-p38, and p-JNK proteins in melanomainvaded lung tissues.
It is becoming increasingly evident that (120) EMT-a process wherein epithelial cells lose their morphology and function, gradually transforming into mesenchymal-like cells-is associated with tumor recurrence and metastasis. During this transformation, epithelial cells acquire characteristics typical of mesenchymal cells, leading to enhanced motility and migration. Numerous regulatory factors influence EMT, including E-cadherin, transforming growth factor β, the Wnt signaling pathway, the circular RNAs, and various transcription factors (125). Notably, the activation of E-cadherin serves as a marker for the initiation of EMT. In a scientific study, treatment of the triple-negative breast cancer cell line MDA-MB-231 with isochlorogenic acid C (126), sourced from LJF, resulted in a decrease in the expression of mesenchymal markers slug and vimentin, alongside an increase in the expression of the epithelial marker E-cadherin, compared to the control group, indicating a reversal of EMT. Furthermore, isochlorogenic acid C was found to decrease the expression of MMP-9, a key driver involved in ECM degradation.
Tumor angiogenesis plays a crucial role in the metastasis of tumor cells to distant organs. Vascular endothelial growth factor A (VEGFA) serves as a key regulator, stimulating the formation and expansion of tumor blood vessels to supply essential nutrients and oxygen, thereby promoting rapid tumor growth and metastasis. A recent study has demonstrated that luteolin (127), the active compounds found in LJF, exhibit anti-angiogenic properties. Specifically, it can inhibit angiogenesis in gastric cancer by suppressing the secretion of VEGF, which depends on the expression of Notch1, ultimately leading to a reduction in the migration and proliferation of gastric cancer cells. Conversely, rutin (128), another active ingredient in LJF, appears to activate the angiogenic pathway that facilitates the spread of breast tumors to adjacent organs. This occurs because rutin enhances the expression of the pro-angiogenic marker VEGFA while diminishing the expression of the anti-angiogenic marker Thrombospondin 1 in the MDA-MB-231 cell line.
5.5 Anti-inflammatory and immunomodulatory effects
There exists a significant connection between the occurrence and development of malignant tumors and the body’s immune defense mechanisms. As tumor cells proliferate, spread, and metastasize, the body’s immune function tends to decline (129). Relevant reports indicate that chronic inflammation plays a crucial role in all stages of tumor development, as well as in the treatment process. It serves not only as a factor that induces tumorigenesis, growth, deterioration, and metastasis but is also closely related to the anti-tumor immune response (130). Therefore, regulating the immune response and the expression of inflammatory factors is essential for delaying tumor progression and improving both the survival rate and quality of life for patients.
LJF extract has been found to mitigate TNF-α or IL-6-induced inflammatory responses in hepatocellular carcinoma and macrophage cell lines by inhibiting NF-κB/IL-6/STAT3 signaling, thereby reversing immune suppression (131). NF-κB and STAT3 are crucial transcription factors linking cancer and inflammation (132). The ethanol extract of LJF demonstrates anti-melanoma effects by targeting STAT3 signaling and reshaping the immune microenvironment (20).
Anti-microbiome therapy utilizing LJF bud extract notably reduced polyp burden in ApcMin/+ mice and alleviated intestinal inflammation by shifting macrophages from an M1 to M2 phenotype (133). In cyclophosphamide (CTX)-induced immunosupressed mouse models, LJF polysaccharides significantly enhanced organ index, splenic lymphocyte proliferation, macrophage phagocytosis, and NK cell activity, while restoring serum cytokine levels of IL-2, TNF-α, and IFN-γ, confirming its anti-inflammatory and immunomodulatory properties (134). Moreover, LJF-derived miRNAs have shown anti-tumor immune effects by targeting TGF-β1 to enhance T lymphocyte infiltration in tumor environments (135).
5.6 Regulation of autophagy
Autophagy is an evolutionarily conserved, lysosome-mediated biodegradation process that is essential for regulating cell growth and maintaining internal homeostasis (136). Under conditions of cellular stress, autophagy acts prior to apoptosis to preserve cellular equilibrium. Consequently, autophagy is often regarded as a cellular strategy and mechanism for survival in stressful environments (137). It has been reported that autophagic cell death is closely related to many human diseases, especially plays a key role in the initiation and development of cancer (138). In the early stages of tumorigenesis, autophagy functions as a survival pathway and quality control mechanism, inhibiting early tumor development by enhancing antitumor activity. However, once the tumor progresses to advanced stages and encounters environmental stress, autophagy can promote tumor progression by stimulating growth. Therefore, the regulation of autophagy presents a potential intervention strategy for cancer therapy.
In lung cancer studies, luteolin (139) significantly regulated the expression of autophagy-associated proteins, including the accumulation of the microtubule-associated protein light chain-3 (LC3) II, the increase of LC3 puncta, and the enhancement of autophagic flux, which collectively induced autophagy to promote cell death. In contrast, luteolin (140) induced autophagy in human hepatocellular carcinoma Hep3B cells by up-regulating the level of LC3-II protein and down-regulating the level of p62 protein, thereby promoting cancer cell survival.
5.7 Activation of related signaling pathways
The occurrence and development of malignant tumors are closely associated with the activation of various signaling pathways. As a traditional Chinese herb, LJF extract and its active components have demonstrated a certain anti-tumor effect in related studies, which is attributed to the regulation of multiple signaling pathways.
In recent years, NF-κB and STAT3 have garnered significant attention in various cancers. Numerous studies have demonstrated that NF-κB is extensively involved in a range of physiological and pathological processes within the body, including inflammatory responses, cell survival, proliferation, differentiation, and tumorigenesis (141, 142). Concurrently, STAT3 plays a crucial role in regulating cell growth and apoptosis by modulating the expression of multiple genes in response to cellular stimuli (132). Research conducted by Ju et al. indicated that (143) luteolin can effectively inhibit NF-κB activity, thereby enhancing the pro-apoptotic effects of JNK on TNF-induced lung cancer cells. Additionally, kaempherol, an active component of LJF, in conjunction with luteolin, was found to inhibit the binding of STAT3 to the claudin-2 promoter region, resulting in decreased expression and proliferation of claudin-2 in A549 cells (144). These findings offer new insights and directions for further exploration in cancer therapy.
The Hippo/YAP pathway is a highly conserved cellular signaling pathway that plays a crucial role in regulating organ size and tumorigenesis (145). In parallel, the Wnt/β-catenin pathway serves as a fundamental molecular mechanism in embryonic development and tissue homeostasis, with its aberrant activation being a significant contributor to the onset and progression of various cancers (146). In our scientific study, we discovered that the mechanism by which LJF exerts its effects against hepatocellular carcinoma (HCC) may be linked to these two pathways (61). Our research data indicated that 3,4-di-O-cafeoylquinic acid, a novel compound isolated from LJF buds, inhibits the proliferation and migration of HCC cells through the suppression of the Hippo/YAP pathway. Additionally, 3,4-di-O-cafeoylquinic acid appears to induce cell cycle arrest in HCC cells by inhibiting the Wnt/β-catenin pathway. These findings suggest that both the Hippo/YAP and Wnt/β-catenin pathways are likely involved in the inhibition of HCC cells induced by 3,4-di-O-cafeoylquinic acid, indicating its potential as a promising therapeutic agent for HCC.
JNK is a significant member of the MAPK family in mammalian cells and has been demonstrated to activate a diverse array of substrates in response to various stimuli, including the regulation of apoptosis, proliferation, tumorigenesis, and inflammation (147). In a screening of the anti-liver cancer active ingredients of LJF extract (protocatechuic acid, chlorogenic acid and luteolin), the researchers found that (62) only protocatechuic acid could activate the JNK and p38 subgroups. Additionally, both the aqueous extract of LJF and protocatechuic acid were found to induce HepG2 liver cancer cell death in a JNK-dependent manner. Another study of hepatocellular carcinoma cells indicated that (148) luteolin, the active ingredient of LJF, enhanced TRAIL-induced apoptosis, potentially mediated by JNK-mediated DR5 expression and autophagy.
Src homology-2 domain-containing protein tyrosine phosphatase-2 (SHP2) is a non-receptor protein tyrosine phosphatase that serves dual roles as both an oncogenic factor and a tumor suppressor in various diseases, making it a promising therapeutic target for cancer treatment. Previous studies have demonstrated that (149) the SHP2/Ras/MAPK signaling pathway is involved in mediating a range of cellular functions, including cancer cell proliferation, apoptosis, and invasion. Notably, total saponins derived from LJF have been reported to inhibit the proliferation, invasion, and apoptosis of thyroid cancer TPC-1 cells by suppressing the activation of the SHP2/Ras/MAPK signaling pathway (87).
6 Pharmacokinetics
The determination of the pharmacokinetics of Chinese medicines is of critical importance for optimizing drug delivery and enhancing bioavailability. However, the diversity and complexity of the constituents in Chinese medicines, coupled with the low content of active ingredients in crude extracts and their unique pharmacokinetic properties, present significant challenges for research in this area. Currently, pharmacokinetic studies on LJF remain limited, primarily concentrating on the exploration of active compounds isolated from this plant.
Flavonoids are significant components of LJF. Chen et al. provided data (150) for the investigation of their pharmacokinetics. The flavonoid extracts included rutin, luteolin-7-O-β-D-glucoside (LEG), quercetin-3-O-β-D-glucoside (QEG), and lonicerin. Analysis of the blood plasma concentration-versus-time curve revealed that these components exhibited a similar trend in rats, characterized by rapid absorption and slow elimination. Based on the plasma clearance (CL) data, rutin and LEG demonstrated superior performance compared to QEG. And from the apparent volume of distribution (Vd) data, these components are widely distributed in rats, and their Vd values far exceed the actual blood volume of rats. This method is suitable for the pharmacokinetic study of multi-components of Chinese medicine.
Accumulated studies have demonstrated that chlorogenic acid is not only the principal active ingredient of LJF but also serves as a significant marker for evaluating its quality. Among the investigations into LJF extracts, the pharmacokinetic studies of chlorogenic acid are particularly extensive. Zhou et al. (151) examine meticulously the pharmacokinetics and tissue distribution of chlorogenic acid in rats following oral administration. The results indicated that chlorogenic acid was rapidly absorbed and eliminated in the rats, exhibiting an elimination half-life (T1/2) of approximately 0.8 h. Furthermore, chlorogenic acid was predominantly distributed in the liver, followed by the kidneys, lungs, heart, and spleen in descending order. A separate study by Zhou et al. (152) revealed that the metabolic processes of chlorogenic acid in rats, after intravenous, intramuscular, and gavage administration of LJF extract, conformed to a two-compartmental model. The T1/2 were (0.44 ± 0.08) h, (0.50 ± 0.12) h, and (0.38 ± 0.11) h, respectively. The absolute bioavailability of gavage and intramuscular injection were 37.39% and 94.50%, respectively. These findings provide a scientific basis for further studies on the routes of administration and dosage forms of LJF extract.
Luteoloside serves as a crucial marker for assessing the quality of LJF. Pharmacokinetic studies utilizing scientific bioanalytical methods are significantly important for the further development of luteoloside. Qiu et al. (153) discussed in detail the pharmacokinetics of experimental dogs after intravenous injection of 20mg/kg luteoloside. The results indicated that the T1/2 of luteoloside was approximately 1.21 ± 0.14 h. The mean area under the plasma concentration-time curve from time zero to the last measurable plasma concentration point (AUClast) and the mean area under the plasma concentration-time curve from time zero to infinity (AUCInf) were 785 ± 54.6 and 788 ± 54.1 h ng/mL, respectively. Additionally, CL, mean residence time (MRT), and volume of distribution at steady state (Vss) were 425 ± 30.4 mL/min/kg, 0.62 ± 0.03 h, and 15.8 ± 0.39 L/kg, respectively.
By comparing pharmacokinetic data following a single oral and intravenous dose of isochlorogenic acid C (IAC), Huang et al. (154) found that IAC is rapidly absorbed after oral administration, reaching its maximum concentration (Cmax) at approximately 1 hour. The study also demonstrated that IAC exhibits poor bioavailability in rats, with Cmax and the area under the curve (AUC0-∞) positively correlated with dose. Additionally, Zhan et al. analyzed (155) the metabolite composition in rats after oral administration of 4,5-dicaffeoylquinic acid. Their analysis revealed 15 metabolites in plasma and 16 metabolites in urine, encompassing various reaction types such as methylation, hydration, dehydrogenation, reduction, glucuronidation, and sulfate esterification. Furthermore, Luo et al. (156) quantitatively monitored sweroside in the plasma, urine, feces, and bile of rats using the high performance liquid chromatography coupled to ultraviolet detection (HPLC-UV) method. The results indicated that the bioavailability of sweroside was extremely low at 0.31%, which may be attributed to its primary excretion via feces.
Pharmacokinetics focuses on the exploration of the absorption, distribution, metabolism, and excretion processes of drugs within living organisms. Conducting relevant pharmacokinetic studies on LJF extracts and their isolated compounds can elucidate the active ingredients present in LJF and provide valuable reference information for its development and application. However, current studies on the pharmacokinetics of LJF exhibit certain limitations. Most research has been conducted using normal animal models, with relatively few studies addressing pathological conditions. In the future, it is of great clinical significance to study the pharmacokinetics of LJF in pathological models.
7 Quality control
LJF is a kind of medicinal and edible plant. The flower is white at first, and then becomes yellow, so it is also called double flower or two flower. This color change represents not only a distinctive biological trait of LJF but also serves as an external indicator of its quality and efficacy, which are closely tied to its economic value. In traditional botanical research (157), the color of LJF is considered a critical criterion for assessing its quality. The initial green-white samples were of the best quality, during which the contents of chlorogenic acid and cynaroside were particularly abundant. Furthermore, the quality of LJF is influenced by various factors, including processing methods, water conditions, pruning techniques, and pesticide residues (158, 159).
According to the Chinese Pharmacopoeia (2015 edition), luteoloside and chlorogenic acid were designated as the quality markers for LJF. Over time, the Chinese Pharmacopoeia (2020 edition) has made more rigorous and detailed provisions on the quality standards of LJF. In addition to retaining the original markers, luteoloside and chlorogenic acid, the pharmacopoeia also incorporated isochlorogenic acid A and isochlorogenic acid C as key indicators for quality control. Furthermore, it clearly states the minimum content standards for various components, including chlorogenic acid, to ensure the stability and controllability of LJF’s efficacy.
Stability and controllable quality are essential prerequisites for the advancement of modern Chinese medicine. The foundation of quality control lies in the thorough analysis of its active ingredients. LJF exhibits a broad spectrum of pharmacological effects due to its intricate chemical composition. Consequently, relying solely on a few iconic components for quality evaluation is insufficient. Drawing on the theory of quality markers (Q-marker) in Chinese medicine, Yuan and his colleagues (160) conducted a comprehensive analysis and prediction of LJF’s quality based on the efficacy, measurability, and specificity of its chemical constituents. They proposed that chlorogenic acid, isochlorogenic acid A, isochlorogenic acid B, isochlorogenic acid C, luteoloside, rutin, sweroside, and secoxyloganin could serve as candidate quality markers for LJF. This study offers a more holistic perspective for the quality assessment of LJF, which holds significant implications for the quality control of Chinese medicines, including LJF.
In recent years, researchers have also worked to establish other quality evaluation methods for LJF. Zhang et al. (161, 162) successfully prepared specific monoclonal antibodies against chlorogenic acid and lignoceroside, named mAb2E2 and mAb3A4, respectively. Based on these monoclonal antibodies, they skillfully developed an indirect competitive enzyme-linked immunosorbent assay (icELISA). This method is not only simple and rapid, but also the results are in high agreement with the HPLC validation results. What’s more, with its excellent performance, icELISA proved to have great potential for detecting chlorogenic acid and lignoceroside content in different LJF herbal samples, providing a new means for quality control of LJF.
In addition, zhang et al. (163) developed an innovative colloidal gold-based lateral flow dipstick immunoassay that enables rapid determination of chlorogenic acid and luteoloside content in LJF, achieving results in just 10 minutes. By visually assessing the color of the test line, one can ascertain whether the sample concentration exceeds the detection limit of the test strip, thereby confirming compliance with quality standards. The convenience and high efficiency of this method provide robust technical support for the rapid detection and quality control of Chinese herbal medicines.
8 Toxicity
The current understanding of LJF toxicity is still in its initial stages, with only a limited number of studies conducted on animal models. In an assessment of the acute and subacute toxicity of the ethanol extract of LJF, a single oral dose of 5,000 mg/kg did not result in mortality or any significant visceral pathological changes in rats. Furthermore, continuous administration of a lower dose of 1,000 mg/kg/day over a period of 14 days led to a notable increase in testicular weight in male mice. Additionally, hematological analyses indicated that the extract does not exhibit toxic effects (164).
An in-depth study on the acute toxicity of LJF was conducted by Chi et al. (165) using both intravenous and intraperitoneal administration methods. The experimental results indicated that the median lethal dose (LD50) values ranged from 74.3 g/kg to 84.7 g/kg in mice and rats. Given that the recommended clinical dosage of LJF is 20 g/60 kg, our comprehensive evaluation suggests that its use is relatively safe. Additionally, the acute toxicity of tetraploid LJF was thoroughly assessed by Hu and his colleagues, who (166) compared the LD50 values of aqueous extracts from tetraploid and diploid LJF in mice. The results revealed negligible differences, with LD50 values reaching approximately 412 and 400 times the safe dosage for humans (based on a body mass of 60 kg), respectively. This finding implies that the use of aqueous extracts of tetraploid LJF is relatively safe within a specific dose range. Furthermore, Zhang et al. (167) reported that LJF tablets did not demonstrate significant toxicity in rats at conventional doses following a 30-day feeding experiment.
A study conducted by Huang et al. (168) demonstrated that LJF exhibits an in vitro hemolytic effect, particularly when administered as an injection, which poses a potential risk of hemolysis. This effect is attributed to the total saponin content present in LJF. Hemolysis was observed to commence when the mass concentration of total saponins reached 0.6 g/L, with a significant increase in the rate of hemolysis corresponding to higher concentrations. At a mass concentration of 1.2 g/L, the hemolysis rate reached 55.3%. However, in practical applications, Chinese medicine injections typically do not attain such elevated drug concentrations. Consequently, we conclude that it is safe to utilize LJF, either orally or via injection, at conventional dosages.
9 Clinical research
9.1 Clinical study of LJF extract
Research into the anti-tumor properties of traditional Chinese medicine (TCM) has increasingly focused on LJF as an adjuvant in cancer treatment. Its anti-cancer potential has been validated through both cellular and animal studies, and is progressively being substantiated in clinical settings. Previous clinical trials indicate that the combination of LJF with radiotherapy, chemotherapy and targeted therapies is effective in treating non-small cell lung cancer, liver cancer, nasopharyngeal cancer, and esophageal cancer, etc. This combination therapy not only significantly enhances patient tolerance to radiotherapy and chemotherapy but also effectively mitigates adverse reactions associated with various cancer treatment drugs, including acne-like rashes, hand and foot skin reactions, and oral mucositis.
In the clinical practice of Four Flavor-LJF decoction (169) combined with chemotherapy drugs (Carboplatin and Etoposide) in the treatment of non-small cell lung cancer, the use of LJF not only reduces the frequency of nausea and vomiting, but also inhibits adverse reactions such as neutropenia and thrombocytopenia. It also reduced the incidence of alopecia, neurotoxicity, and muscle and joint pain. Compared with chemotherapy alone, Four Flavor-LJF decoction combined with chemotherapy has better tolerance and safety in the treatment of central NSCLC.
According to the clinical study conducted by Sun et al. (170), the use of LJF in combination therapy for patients undergoing radiotherapy for nasopharyngeal carcinoma significantly reduces the incidence of mild mucosal reactions and effectively alleviates oral and nasal mucosal reactions, thereby improving patient symptoms. In the context of LJF in combination therapy for esophageal cancer radiotherapy (171), the concurrent use of LJF decoction and aluminum magnesium suspension not only significantly enhances treatment efficacy but also shortens the duration of esophagitis. Furthermore, LJF demonstrates immunomodulatory effects in the treatment of radiation-induced esophagitis. In the study by Song et al. (172), the incidence of esophagitis and the levels of inflammatory factors in the treatment group were significantly lower than those in the control group, while the levels of CD3+ T cells, CD4+ T cells, and the CD4+/CD8+ ratio were significantly increased. These findings collectively underscore the positive impact of LJF in the context of cancer radiotherapy.
Most patients treated with epidermal growth factor receptor inhibitors (EGFRIs) experience skin toxic effects, such as acne or papulopustular rash, that seriously affect patients’ quality of life and may lead to treatment interruption. However, a prospective, randomized and controlled study has shown that LJF therapy can effectively reduce acne-like rash caused by EGFRIs. In this study, different treated patients were randomly divided into three groups. The data showed that the incidence of acneiform rash in group A patients treated with LJF prophylacticly was 56.5%, much lower than that in group B (68.1%) and group C (71.7%). Not only that, LJF treatment has a significant effect on reducing the severity of skin toxicity, controlling the time of rash occurrence, reducing the degradation rate and improving the progress (173). This series of research results show that the LJF in the treatment of skin toxicity caused by EGFRIs has significant preventive treatment effect.
As a multi-kinase inhibitor, sorafenib has been shown to prolong the survival of patients and effectively inhibit tumor progression. However, it is often accompanied by a series of adverse reactions during the treatment, one of which is notable is hand-foot skin reaction (HFSR). Patients may have symptoms such as dry skin, rash, itching, hair drying, desquamation, hair loss, and skin induration. These symptoms bring great distress to patients. However, it is encouraging that LJF combined with sorafenib (174, 175) can significantly reduce the incidence of HFSR and improve the tolerance of patients. More importantly, the attenuated efficacy was more significant when LJF and Shengjigao were combined with sorafenib (176).
In the context of clinical research on LJF, we conducted a comprehensive search of authoritative databases. Recognizing the importance of clinical trial registration, we specifically reviewed the Chinese Clinical Trial Registry (https://www.chictr.org.cn/showproj.html?proj=219472) and the International Clinical Trial Register Platform (https://trialsearch.who.int/). Through precise retrieval using the keywords “LJF and cancer,” we identified a notable single-arm clinical study titled “A single-arm clinical study of LJF oral liquid in the treatment of non-small cell lung cancer EGFR-TKIs-associated oral mucositis” with the patent number ChiCTR2400080982. This study examined the potential of combining LJF with EGFR TKIs in the treatment of non-small cell lung cancer, particularly focusing on LJF as an auxiliary agent to mitigate adverse effects. The findings indicate that the use of LJF in treating oral mucositis resulting from EGFR TKIs in non-small cell lung cancer patients may lead to reduced side effects and improved efficacy. Given LJF’s low toxicity, broad availability, and cost-effectiveness, we propose its consideration as a preferable adjunctive therapy in cancer treatment.
9.2 Combined anti-tumor efficacy of LJF active components
In recent years, research on Chinese medicine has deepened, leading to widespread recognition of the natural effects of its extracts and active ingredients in the field of anti-tumor therapy. Currently, the combination of conventional therapeutic drugs with natural compounds has emerged as a prominent strategy in cancer treatment. This approach leverages the unique complementary advantages of both modalities, allowing for a reduction in the dosage of chemotherapy drugs while simultaneously minimizing toxicity and side effects. Furthermore, it effectively delays the onset of drug resistance and significantly enhances therapeutic outcomes. Various anticancer active ingredients found in LJF, including chlorogenic acid, luteolin, luteoloside, isochlorogenic acid, rutin, and hyperoside, have been reported to be combined with other chemical therapies and are widely utilized in cancer treatment.
9.2.1 Synergistic effects
In order to improve the effect of cancer treatment, we have successfully combined the active ingredients of LJF with a variety of chemotherapeutic drugs to form an innovative combination therapy. This treatment involves multiple targets and multiple signal transduction pathways, which can effectively enhance the anti-cancer efficacy of chemotherapy drugs.
Oxaliplatin, as a highly effective cytotoxic drug, is often used as the preferred drug for postoperative treatment of colorectal carcinoma. When we combine luteolin with oxaliplatin (177), they can significantly block the cycle progression of cancer cells, and then induce more apoptosis in the periphery of tumor clusters and tumor cell clusters, thus effectively inhibiting tumor growth. In addition, the combination of luteolin and cisplatin (178) not only significantly inhibited cell migration and invasion, but also promoted early apoptosis of cancer cells by down-regulating Bcl-2, and enhanced the anti-proliferative effect of cisplatin on ovarian cancer CAOV3/DDP cells. Further mechanism studies have shown that luteolin can synergistically enhance the anti-tumor effect of 5-fluorouracil (179) on HepG2 and Bel7402 ovarian cancer cells by inducing apoptosis and regulating metabolism. It is worth mentioning that the combination of chlorogenic acid and 5-fluorouracil (180) produced more prominent ROS production and more obvious ERK1/2 inactivation than single treatment, which further mediated the enhancement of 5-fluorouracil-induced inhibition of liver cancer cell proliferation and significantly improved the therapeutic effect of 5-fluorouracil.
In addition to the effective combination with conventional single chemotherapeutic drugs, the anticancer components contained in LJF also show the potential to be combined with new targeted drugs to improve the therapeutic effect. Take lapatinib as an example, which is a tyrosine kinase inhibitor. When lapatinib is used in combination with luteolin (181), they can significantly inhibit the proliferation of breast cancer cells, thereby enhancing the therapeutic effect of lapatinib on human breast cancer. This combination therapy not only increased the sensitivity of SKBR-3, BT-474 and ZR-75-1 cells to treatment, but also up-regulated the gene expression levels of FOXO3 a and NQO1. Therefore, the combination of promising chemotherapeutic drugs with less toxic natural compounds has shown good therapeutic effects and provided new possibilities and directions for cancer treatment.
9.2.2 Sensitization effects
The active ingredient luteolin in LJF can inhibit autophagy and reduce the expression of LC3-II, thereby inhibiting cell viability. At the same time, it can also enhance the sensitivity to cisplatin by inhibiting the expression of RARP1 in epithelial ovarian cancer cells (182).
Studies have shown (183) that the production of cisplatin resistance is closely related to the induced expression of progesterone receptor membrane component (PGRMC1) in ovarian cancer cells. Hyperoside activates AKT signal transduction and Bcl-2 family expression by relying on the autophagy of PGRMC1, induces increased apoptosis, and makes cancer cells more sensitive to cisplatin treatment.
Among the anticancer drugs, Paclitaxel is a commonly used drug for the treatment of breast cancer, and its efficacy is significant. However, high-dose paclitaxel has problems such as relapse resistance and adverse reactions, which has become a major challenge in the treatment of advanced breast cancer. Recently, the study of Sun et al.provided a new possibility for the application of hyperoside in the field of anticancer-as a sensitizer (184). It is speculated that hyperoside may enhance the sensitivity of breast cancer cells to paclitaxel by blocking the pro-inflammatory and pro-survival mechanisms caused by TLR4 activation.
In addition, the active ingredients of LJF can not only enhance the effect of chemotherapy drugs, but also have a significant radiosensitization effect. When rutin is combined with radiation therapy (185), this combination therapy can significantly increase the number of apoptotic colon cancer cells, resulting in more significant cell death. Compared with other treatment groups, the combination therapy also led to changes in mitochondrial membrane potential, increased DNA damage, increased levels of lipid peroxide markers, and decreased antioxidant status. This indicated that rutin played a significant radiosensitizing effect in HT-29 colon cancer cells.
9.2.3 Attenuation effect
Doxorubicin is one of the most effective chemotherapeutic drugs for the treatment of solid tumors. However, its dose-related potential cardiotoxicity may trigger heart failure in patients. Therefore, it is particularly important to develop a drug that has a cardioprotective effect during doxorubicin treatment and enhances its efficacy in cancer cells. Recent studies have shown that (186) when luteolin is combined with doxorubicin, it can significantly inhibit cell proliferation and metastasis, and effectively induce apoptosis. This not only prevents doxorubicin-induced cardiotoxicity, but also enhances its effect against breast cancer.
Oxaliplatin has shown significant efficacy in the treatment of gastric cancer. However, its long-term use can cause side effects such as nephrotoxicity, ototoxicity, neurotoxicity and bone marrow suppression, and may even cause discomfort such as nausea and vomiting. In response to this problem, Li et al. (187) proposed a method for combining rutin with oxaliplatin. This method not only successfully reduced the dose of oxaliplatin, but also significantly reduced its side effects. Compared with oxaliplatin or rutin alone, this combination therapy is more prominent in anti-tumor effect.
Ovarian injury and infertility are common side effects of chemotherapy in female patients with cancer. In the combined treatment of cyclophosphamide and hyperoside (188), the latter shows a unique protective effect. It increases mitochondrial membrane potential by blocking HIF-1α/BNIP3-mediated autophagy activation, thereby increasing follicular reserve and saving fertility in cyclophosphamide-treated mice. This property of hyperoside makes it show great potential in the field of ovarian protection and is expected to help maintain the fertility rate of women receiving chemotherapy.
9.2.4 Anti-drug resistance
Chemotherapy resistance has become a major obstacle to the cure of ovarian cancer. Among them, cisplatin drug resistance is particularly prominent in the treatment of ovarian cancer and has become one of the main challenges. Luteolin, as a natural compound, effectively inhibits the migration and invasion of CAOV3/DDP cells by promoting the process of apoptosis, thereby enhancing the anti-proliferative effect of cisplatin on drug-resistant ovarian cancer cells (178). In addition, luteolin also makes drug-resistant human breast cancer cells sensitive to tamoxifen by inhibiting the expression of cyclin E2 (189).
The long-term drug resistance of tumor cells is an important reason for the recurrence and metastasis of cancer patients. Therefore, it is particularly important to develop alternative therapies against drug resistance. The active ingredient luteolin in LJF may be a new strategy to overcome the resistance of breast cancer patients to tamoxifen. Luteolin has been shown to inhibit the activation of PI3K/AKT/mTOR signaling pathway, effectively induce tumor cell apoptosis, reduce mitochondrial membrane potential, and arrest the cell cycle in the G2/M phase, thereby inhibiting the proliferation of tamoxifen-resistant estrogen receptor -positive breast cancer cells (190).
10 Biomedical application
10.1 Metal nanoparticles
In recent years, metal nanoparticles (MNPs) have gained significant attention in the biomedical field due to their small size, high area-volume ratio, and excellent reactive activation. Their unique physicochemical properties have made them a focal point of research (191). Currently, it is widely accepted that MNPs synthesized using green plant extracts exhibit superior biocompatibility, medicinal properties, cost-effectiveness, and environmental sustainability, while also demonstrating low toxicity and remarkable anti-cancer effects (192, 193).
LJF, a well-known Chinese herbal plant, is abundant in phenolic acids and flavonoid derivatives, which are associated with effective anti-cancer activities against various cancer cells. Additionally, LJF possesses a strong reducing capability, making it an ideal green material for synthesizing metal nanoparticles. Analysis of existing data indicates that metal nanoparticles prepared with LJF extract as a reducing agent and stabilizer not only offer innovative therapeutic approaches in cancer therapy but also hold significant potential for applications in biomedicine.
Patil et al. (194) found that the majority of gold nanoparticles (AuNPs) synthesized using LJF extract were spherical in shape, with particle sizes ranging from 10 to 40 nm. These nanoparticles exhibited significant cytotoxicity against cervical cancer HeLa cells, effectively inducing apoptosis, which resulted in the death of HeLa cells and inhibited their proliferation. Additionally, Rajivgandhi et al. (195) demonstrated that silver nanoparticles (Ag NPs) synthesized by LJF possess antiproliferative properties against human lung cancer A549 cells. The mechanism of action primarily involves the triggering of ROS production, which subsequently leads to increased apoptotic cell death.
Another study demonstrated that (196) LJF-silver nanoparticles (LJF-Ag NPs), synthesized using LJF as a reducing agent, exhibited a spherical structure similar to that of biological membrane. Supported by comprehensive experimental data, the LJF-Ag NPs significantly enhanced the antioxidant properties, antimicrobial properties, and anticancer activities of LJF extracts. In MTT assays, the synthesized LJF-Ag NPs displayed superior biocompatibility and anticancer effects compared to LJF in cervical cancer HeLa cells, hepatocellular carcinoma HepG2 cells, and breast cancer MDA-MB-231 cells. The nanonization of LJF further amplifies its anticancer activity and presents broader opportunities for clinical trials in the field of anticancer applications. The anti-tumor effect of LJF-metal nanoparticles is worthy of further exploration and research.
10.2 Photodynamic therapy
Photodynamic therapy (PDT) has emerged as a cutting-edge technology in cancer treatment, characterized by its minimally invasive nature, portability, high efficiency, low toxicity, and strong targeting capabilities. It is widely utilized in the treatment of various cancers. This therapy employs a specific wavelength of visible light to activate tumor-targeted photosensitizers, which in turn produce ROS that effectively induce apoptosis in tumor cells, thereby achieving therapeutic objectives (197). As the core component of PDT, advancements in the research of photosensitizers are crucial to enhancing the effectiveness of this treatment. While current photosensitizers have demonstrated significant efficacy in clinical practice, they also present certain limitations, including a degree of phototoxicity and restricted availability. Recent studies indicate that natural product photosensitizers offer promising alternatives due to their lower toxicity and potential therapeutic benefits, highlighting their great application potential and future prospects (198).
LJF, recognized as an edible traditional medicine, exhibits notable anti-inflammatory and anti-tumor properties. Prior research has demonstrated that LJF possesses photosensitive characteristics. When exposed to high-pressure xenon lamp irradiation, it generates photodynamic effects that enhance its anti-tumor efficacy. The application of LJF as a natural photosensitizer in photodynamic therapy for Ehrlich′s ascites carcinoma EAC cells results in a significant therapeutic effect (199).
In recent years, several studies have demonstrated that LJF holds significant potential as a photosensitizer in the treatment of lung cancer, effectively enhancing the therapeutic effects of PDT. An incidental study revealed (74) that LJF exhibited notable photocytotoxicity in human lung squamous carcinoma CH27 cells. The underlying molecular mechanism is closely associated with caspase-3-induced apoptosis. As research progressed, Liao et al. (72) confirmed that mitochondrial dysfunction and ERS play crucial roles in the photoactivated LJF-induced apoptosis in CH27 cells, as evidenced by proteomics studies. The involvement of ROS in the apoptosis of CH27 cells induced by photoactivated LJF was established. Additionally, the study indicated that the ethyl acetate fraction of the LJF extract may be a key compound responsible for its photosensitive activity.
LJF is a valuable resource cultivated globally. It is both low cost and safe, demonstrating potential in the treatment of various cancer types. Notably, when combined with PDT, LJF acts as a natural photosensitizer, resulting in a more pronounced therapeutic effect on lung cancer. In conclusion, LJF-mediated PDT is anticipated to emerge as a novel therapeutic approach, offering a new strategy for the prevention and treatment of multiple cancers.
10.3 Novel drug delivery systems
The anticancer active ingredients of LJF are facing a series of challenges, including poor permeability, non-targeting and low bioavailability. These defects seriously limit their clinical application. In recent years, with the continuous progress and integration in the field of biomedicine, various advanced nanocarrier systems have emerged. These nanocarriers, including nanoparticles, micelles, liposomes and nanoemulsions, have received extensive attention at home and abroad due to their good biocompatibility, long half-life, permeability, strong targeting and high bioavailability (200–202). In particular, self-microemulsifying drug delivery systems (SMEDDS) and HER2 nanospheres drug delivery systems have made significant progress in improving the delivery and efficacy of LJF active ingredients.
SMEDDS is an attractive carrier system (203), which has excellent performance in improving drug solubility and oral absorption and utilization. For example, Chen et al. (204) successfully delivered chlorogenic acid using a self-microemulsifying drug delivery system. The results showed that chlorogenic acid could be completely released from SMEDDS within 480 minutes. Moreover, the oral bioavailability of chlorogenic acid was significantly increased (249.4% relative to the chlorogenic acid suspension) after oral administration of SMEDDS in mice. More interestingly, SMEDDS significantly changed the tissue distribution of chlorogenic acid, showing better targeting to the kidney, and its relative intake efficiency reached 2.79 (2.79 of the relative intake efficiency). This may be mainly attributed to the fact that SMEDDS increased the absorption of chlorogenic acid and changed its distribution from liver to kidney, thereby slowing down the metabolism of chlorogenic acid and improving its oral bioavailability. Therefore, SMEDDS is considered to be a promising carrier for oral administration of chlorogenic acid.
In addition, biological nanomaterials are gradually being widely studied and applied in the diagnosis and treatment of tumors due to their good physical and chemical properties. Xiao et al. (205) designed a Her-2 antibody-modified nanosphere drug delivery system. This nanospheres can overcome the non-targeted defects of ordinary liposomes, significantly improve the uptake efficiency of luteolin, and play an anti-breast cancer role by significantly inhibiting the proliferation and migration of breast cancer cells and up-regulating the expression of FOXO1. HER2 nanospheres can produce substantial killing effect on tumor cells by enhancing targeting and specificity, which is a very promising drug carrier.
At the same time, chlorogenic acid encapsulated SMEDDS (CHA-SME) can effectively deliver chlorogenic acid to mesenteric lymph nodes for immunotherapy of glioblastoma (206). As an effective targeting carrier, CHA-SME can activate anti-tumor immune response, thereby enhancing the immunotherapy effect of CHA. It may provide new strategies and directions for immunotherapy.
11 Homology of medicine and food: prevention and health values
11.1 The preventive effect of LJF
In recent years, TCM has garnered significant attention for its distinctive alternative therapies. LJF, as a plant with important medicinal value, not only exhibits anti-tumor potential but also serves multiple adjuvant therapeutic roles in cancer treatment. These roles include preventing postoperative recurrence, alleviating the side effects of radiotherapy and chemotherapy, and enhancing overall treatment efficacy. Moreover, LJF addresses issues such as loss of appetite, insufficient nutritional intake, and decreased immunity associated with tumors. Its unique nutritional properties can provide essential nutrients to help regulate the spleen and stomach, while simultaneously promoting weight gain and improving bodily resistance. Given these benefits, we leverage the LJF medicine and food homology to integrate it into daily porridge or stew, so as to make a variety of therapeutic decoctions (Table 2). This approach not only enhances the flavor of porridge or soup but also maximizes the medicinal benefits of LJF, making it both nutritious and effective in preventing and treating diseases, promoting health, and extending life.
11.2 Modern product development
In recent years, there have been a large number of common food development with LJF as the main component, such as drinks, powder, tea, paste, etc., as shown in Table 3.
11.3 Health food
LJF has a long history of use in healthcare. Historical records, such as the Ben Cao Gang Mu, say that long-term use of LJF can lighten the body and prolong life. A search of the special food information query platform (http://ypzsx.gsxt.gov.cn/specialfood/#/food) of China’s State Administration for Market Regulation reveals that there are currently 80 health products on the market that feature LJF as a primary ingredient, including 75 varieties of “National Food Health Note” and 5 varieties of “National Food Health Word”.Such as Pipa Jinyinhua lozenges (G20120480), Jinyinhua Danshen pearl capsule (G20140309), Fuling Huangqi Jinyinhua capsule (G20190507), Jinyinhua Xiangyuan capsule (G20200152). These LJF-based products are known to not only aid in clearing and nourish throat, eliminating acne, but also enhancing immunity and safeguarding against liver damage.
12 Summary and outlook
Cancer is a worldwide disease and all mankind is actively searching for effective alternative treatments. TCM has a rich history of experience in the prevention and treatment of cancer (211–213). LJF, as a commonly used Chinese herbal medicine, has the effects of clearing heat and detoxifying, anti-bacterial and anti-inflammatory. A number of pharmacological studies have shown that LJF extract and its active ingredients (such as chlorogenic acid, luteoloside, rutin, luteolin, hyperoside, isochlorogenic acid) have significant anti-tumor effects. In clinical practice, LJF is often used as an adjuvant drug for cancer treatment. Combined with the results of TCM theory and modern pharmacological research, we speculate that LJF may be a potential alternative method for cancer treatment. Therefore, we have conducted in-depth research on the anti-tumor effect of LJF to highlight its practical application value in clinical practice.
By collating the literature, this article summarizes the active ingredients, anti-cancer mechanisms, pharmacokinetics, quality control, toxicity, clinical research, biomedical applications, and preventive health care functions of LJF. Compared with the previous review, our review more comprehensively expounds the anti-tumor effect of LJF and its clinical application, and provides supplements and updates for related research. Although the research of LJF has achieved rich results, there are still some problems to be solved that we need to explore and overcome.
So far, we have isolated and identified 507 compounds from LJF by plant extraction technology. Including volatile oil, organic acids, flavonoids, iridoids, triterpenoids and triterpenoid saponins. These compounds have shown significant effects in antibacterial, anti-inflammatory, antiviral, antioxidant, hypolipidemic, hypoglycemic, anti-cancer and immune regulation. However, there are still undiscovered trace components in LJF (54). These trace components may have an important impact on the efficacy, safety and clinical application of LJF. Therefore, the isolation and identification of LJF still need further study.
In the process of summarizing the anti-tumor mechanism of LJF, we found that the research on the anti-tumor effect of LJF extract is not enough. Existing anti-tumor evaluations mainly focus on limited active ingredients, and most studies use in vivo, in vitro, or a combination of both, and the clinical trials involved are very limited. In order to more accurately evaluate the efficacy and reliability of LJF in the field of anti-tumor, we need to carry out more clinical trials to broaden its application potential in clinical treatment.
Through in-depth analysis of pharmacokinetics, we found that LJF extract and its active ingredients showed rapid absorption, slow elimination, wide distribution, and low bioavailability in the human body, which limited its clinical application to a certain extent. Although some nanocarriers have shown positive effects in solving the pharmacodynamics, solubility, targeting and bioavailability of LJF active compounds. However, the number of related studies is limited and there is a lack of sufficient clinical data support. At present, the research on the pharmacokinetics of LJF is mainly carried out in normal animals, and the research on pathological conditions is still insufficient. Considering the complexity of the chemical composition of LJF, its pharmacokinetic process is easily affected by individual differences, drug interactions, disease status and other factors, which makes the research results uncertain. If the pharmacokinetic study of LJF in disease models can be carried out in the future, it will have far-reaching and important clinical significance.
In addition, we must also note that LJF contains a wide variety of chemical components, and its efficacy will be affected by many factors such as origin and processing. Therefore, it is of great significance to construct a set of standardized quality control methods to promote the development of the pharmacodynamics of LJF. The current quality evaluation and control methods are mainly limited to a few compounds such as chlorogenic acid and luteoloside, which cannot fully represent the quality of LJF. Combined with the Q-marker theory of Chinese medicine, we should include more active ingredients into the category of quality markers. In the future, it is suggested that the combination of chemical quality marker evaluation and bioassay should be used to evaluate the quality of LJF more accurately, which can not only provide reference for the quality standard of LJF, but also help us to establish a more comprehensive quality control system, and provide guarantee for the efficacy and safety of clinical medication.
In terms of safety evaluation, there is a lack of sufficient studies regarding the acute toxicity of LJF. However, the 2010 edition of the Chinese Pharmacopoeia recommends a daily dosage of 6 to 15 grams for humans. In practical applications, whether oral or injectable, the drug concentration of LJF usually does not reach such a high level. Therefore, it is safe to use LJF at a conventional dose.
LJF has been widely used in the field of biomedicine due to its strong reducing ability and unique photocytotoxicity. However, the research on the combination of LJF and modern biotechnology is still in its infancy, and its wide application still faces many challenges and problems. Although LJF nanoparticles have many potential application values, their specific utility and safety still need further research and evaluation.
In general, LJF, as a Chinese herbal medicine with rich pharmacological activity, has broad application prospects in cancer treatment. Should future research address the aforementioned related challenges, it will provide more support and guarantee for its clinical application.
Author contributions
PM: Writing – original draft. LY: Writing – review & editing. SJ: Software, Writing – review & editing. ZiZ: Conceptualization, Writing – review & editing. DX: Resources, Writing – review & editing. SH: Investigation, Writing – review & editing. FM: Methodology, Writing – review & editing. ZhZ: Supervision, Writing – review & editing. YN: Supervision, Writing – review & editing.
Funding
The author(s) declare financial support was received for the research, authorship, and/or publication of this article. The project was supported by Ningxia Key Research and Development Program (project no. 2023BEG02015) and National Natural Science Foundation of China (project no. 82260879).
Acknowledgments
The authors acknowledge any support given which is not covered by the author contribution or funding sections.
Conflict of interest
The authors declare that the research was conducted in the absence of any commercial or financial relationships that could be construed as a potential conflict of interest.
Publisher’s note
All claims expressed in this article are solely those of the authors and do not necessarily represent those of their affiliated organizations, or those of the publisher, the editors and the reviewers. Any product that may be evaluated in this article, or claim that may be made by its manufacturer, is not guaranteed or endorsed by the publisher.
Glossary
OB: Oral Availability
DL: Drug-like properties
KEGG: Kyoto Encyclopedia of Traditional Chinese Medicine
TCM: Traditional Chinese Medicine
CHM: Chinese herbal medicine
IL-1β: Interleukin-1β
IL-6: Interleukin-6
VEGF: Vascular endothlial growth factor
EGF: Epidermal Growth Factor
IL-10: Interleukin-10
TGF-β: Transforming growth factor-β1
Mcl-1: Myeloid cell leukemia-1
JNK: C-Jun N-terminal kinase
ANXA2: Annexin A2
NF-κB: Nuclear transcription factor-κB
PI3K: Phosphatidylinositol 3 kinase
MAPKs: Mitogen-activated protein kinase
KEAP1: Recombinant Kelch Like ECH Associated Protein 1
NRF2: Nuclear Factor erythroid 2-Related Factor 2
ARE: Antioxidant response element
Bcl-2: B-cell lymphoma-2
Bax: Bcl-2-associated X
P53: Tumor Suppressor Protein
EMT: Epithelial-mesenchymal transition
EGFR: Epidermal growth factor receptor
PLCγ: Phospholipase Cγ
ERK1/2: Extracellular signal-regulated kinase 1/2
MMP-9: Matrix metalloproteinase-9
CCR7: Recombinant Chemokine C-C-Motif Receptor 7
MMP-2: Matrix metalloproteinase-2
TNF-α: Tumor Necrosis Factor Alpha
STAT3: Signal transducer and activator of transcription 3
IFN-γ: Interferon-γ
IL-2: Interleukin-2
YAP: , Yes-associated protein
CTGF: Connective tissue growth factor
mTOR: Mechanistic Target Of Rapamycin
AKT: Protein kinase B
NLRP3: NOD-like receptor thermal protein domain associated protein 3
SOX2: SRY-box containing gene 2
XIAP: X-linked inhibitor-of-apoptosis protein
Ras: Rat sarcoma
AgNPs: Silver nanoparticles
PDT: Photodynamic therapy
PCa: Prostate cancer
VRK1: Vaccinia related kinase 1
OPCML: Opioid Binding Protein/Cell Adhesion Molecule Like
CDKs: Cyclin-dependent kinases
CKIs: CDK inhibitors
CDC25C: Cell Division Cycle 25C
Notch-1: Notch (Drosophila) homolog 1
Hes-1: Hes family bHLH transcription factor 1
COX-2: Cyclooxygenase-2
FasL: Fas ligand
CHOP: C/EBP homologous protein
GRP94: Glucose-Regulated Protein 94
GRP78: Glucose-Regulated Protein 78
DR5: Death receptor 5
FOXO3: Forkhead box O3
NQO1: Human NAD (P)H quinone oxidoreductase 1
References
1. Sung H, Ferlay J, Siegel RL, Laversanne M, Soerjomataram I, Jemal A, et al. Global cancer statistics 2020: GLOBOCAN estimates of incidence and mortality worldwide for 36 cancers in 185 countries. CA Cancer J Clin. (2021) 71:209–49. doi: 10.3322/caac.21660
2. Kaur R, Bhardwaj A, Gupta S. Cancer treatment therapies: traditional to modern approaches to combat cancers. Mol Biol Rep. (2023) 50:9663–76. doi: 10.1007/s11033-023-08809-3
3. Xiang Y, Guo Z, Zhu P, Chen J, Huang Y. Traditional Chinese medicine as a cancer treatment: Modern perspectives of ancient but advanced science. Cancer Med. (2019) 8:1958–75. doi: 10.1002/cam4.2108
4. Liu Y, Yang S, Wang K, Lu J, Bao X, Wang R, et al. Cellular senescence and cancer: Focusing on traditional Chinese medicine and natural products. Cell Prolif. (2020) 53:e12894. doi: 10.1111/cpr.12894
5. Wang BL, Ge ZK, Qiu JR, Luan SQ, Hao XC, Zhao YH. Sedum aizoon L.: a review of its history, traditional uses, nutritional value, botany, phytochemistry, pharmacology, toxicology, and quality control. Front Pharmacol. (2024) 15:1349032. doi: 10.3389/fphar.2024.1349032
6. Zhao L, Xu C, Zhou W, Li Y, Xie Y, Hu H, et al. Polygonati Rhizoma with the homology of medicine and food: A review of ethnopharmacology, botany, phytochemistry, pharmacology and applications. J Ethnopharmacol. (2023) 309:116296. doi: 10.1016/j.jep.2023.116296
7. Wang Y, Zhang Q, Chen Y, Liang CL, Liu H, Qiu F, et al. Antitumor effects of immunity-enhancing traditional Chinese medicine. BioMed Pharmacother. (2020) 121:109570. doi: 10.1016/j.biopha.2019.109570
8. Shang X, Pan H, Li M, Miao X, Ding H. Lonicera japonica Thunb.: ethnopharmacology, phytochemistry and pharmacology of an important traditional Chinese medicine. J Ethnopharmacol. (2011) 138:1–21. doi: 10.1016/j.jep.2011.08.016
9. Li W, Zhang L, He P, Li H, Pan X, Zhang W, et al. Traditional uses, botany, phytochemistry, and pharmacology of Lonicerae japonicae flos and Lonicerae flos: A systematic comparative review. J Ethnopharmacol. (2024) 322:117278. doi: 10.1016/j.jep.2023.117278
10. Mu W, Hu N, Zhang LH, Jiang W, Yan T, Zhang T, et al. Lonicerae japonicae flos ameliorates radiotherapy-induced mesenteric artery endothelial dysfunction through GTPCH1/BH(4)/eNOS pathway. Phytomedicine. (2022) 102:154146. doi: 10.1016/j.phymed.2022.154146
11. Yang R, Fang L, Li J, Zhang YQ. A new anti-inflammatory lignan from Lonicerae Japonicae flos. Nat Prod Res. (2021) 35:587–92. doi: 10.1080/14786419.2019.1587430
12. Zhou W, Yin A, Shan J, Wang S, Cai B, Di L. Study on the rationality for antiviral activity of flos lonicerae japonicae-fructus forsythiae herb chito-oligosaccharide via integral pharmacokinetics. Molecules. (2017) 22:654. doi: 10.3390/molecules22040654
13. Ge L, Xiao L, Wan H, Li J, Lv K, Peng S, et al. Chemical constituents from Lonicera japonica flower buds and their anti-hepatoma and anti-HBV activities. Bioorg Chem. (2019) 92:103198. doi: 10.1016/j.bioorg.2019.103198
14. Lin L, Wang P, Du Z, Wang W, Cong Q, Zheng C, et al. Structural elucidation of a pectin from flowers of Lonicera japonica and its antipancreatic cancer activity. Int J Biol Macromol. (2016) 88:130–7. doi: 10.1016/j.ijbiomac.2016.03.025
15. Park KI, Park H, Nagappan A, Hong GE, Yumnam S, Lee HJ, et al. Polyphenolic compounds from Korean Lonicera japonica Thunb. induces apoptosis via AKT and caspase cascade activation in A549 cells. Oncol Lett. (2017) 13:2521–30. doi: 10.3892/ol.2017.5771
16. Xu HB, Chen XZ, Wang X, Pan J, Yi-Zhuo Z, Zhou CH. Xihuang pill in the treatment of cancer: TCM theories, pharmacological activities, chemical compounds and clinical applications. J Ethnopharmacol. (2023) 316:116699. doi: 10.1016/j.jep.2023.116699
17. Li S, Zhang B. Traditional Chinese medicine network pharmacology: theory, methodology and application. Chin J Nat Med. (2013) 11:110–20. doi: 10.3724/SP.J.1009.2013.00110
18. Wang S, Long S, Wu W. Application of traditional chinese medicines as personalized therapy in human cancers. Am J Chin Med. (2018) 46:953–70. doi: 10.1142/S0192415X18500507
19. Ikeda H, Togashi Y. Aging, cancer, and antitumor immunity. Int J Clin Oncol. (2022) 27:316–22. doi: 10.1007/s10147-021-01913-z
20. Liu YX, Bai JX, Li T, Fu XQ, Guo H, Zhu PL, et al. A TCM formula comprising Sophorae Flos and Lonicerae Japonicae Flos alters compositions of immune cells and molecules of the STAT3 pathway in melanoma microenvironment. Pharmacol Res. (2019) 142:115–26. doi: 10.1016/j.phrs.2019.02.020
21. Zhang Y, Liang Y, He C. Anticancer activities and mechanisms of heat-clearing and detoxicating traditional Chinese herbal medicine. Chin Med. (2017) 12:20. doi: 10.1186/s13020-017-0140-2
22. Wang Q, Su CP, Zhang HM, Ren YL, Wang W, Guo SZ. Anti-inflammatory mechanism of heat-clearing and detoxifying Chinese herbs. Zhongguo Zhong Yao Za Zhi. (2018) 43:3787–94. doi: 10.1016/j.jacc.2018.08.101
23. Fan M, Zhang X, Song H, Zhang Y. Dandelion (Taraxacum genus): A review of chemical constituents and pharmacological effects. Molecules. (2023) 28:5022. doi: 10.3390/molecules28135022
24. Wang Z, Xia Q, Liu X, Liu W, Huang W, Mei X, et al. Phytochemistry, pharmacology, quality control and future research of Forsythia suspensa (Thunb. ) Vahl: A review. J Ethnopharmacol. (2018) 210:318–39. doi: 10.1016/j.jep.2017.08.040
25. Lu D, Yang Y, Du Y, Zhang L, Yang Y, Tibenda JJ, et al. The potential of glycyrrhiza from "Medicine food homology" in the fight against digestive system tumors. Molecules. (2023) 28:7719. doi: 10.3390/molecules28237719
26. Wang L, Jiang Q, Hu J, Zhang Y, Li J. Research Progress on Chemical Constituents of Lonicerae japonicae flos. BioMed Res Int. (2016) 2016:8968940. doi: 10.1155/2016/8968940
27. Yang QR, Zhao YY, Hao JB, Li WD. Research progress on chemical constituents and their differences between Lonicerae Japonicae Flos and Lonicerae Flos. Zhongguo Zhong Yao Za Zhi. (2016) 41:1204–11. doi: 10.4268/cjcmm20160708
28. Yang X, Liu Y, Hou A, Yang Y, Tian X, He L. Systematic review for geo-authentic Lonicerae Japonicae Flos. Front Med. (2017) 11:203–13. doi: 10.1007/s11684-017-0504-0
29. Yan-gui Z. Analysis of volatile components of honeysuckle in ningxia by GC-MS. J Zhengzhou Univ. (2006) 01:95–7. Available online at: https://www.semanticscholar.org/paper/Analysis-of-Volatile-Components-of-Honeysuckle-in-Yan-gui/133aa7dbf0c086de48d4e18d455717aee461b617#citing-papers.
30. Ahmad A, Tiwari RK, Saeed M, Al-Amrah H, Han I, Choi EH, et al. Carvacrol instigates intrinsic and extrinsic apoptosis with abrogation of cell cycle progression in cervical cancer cells: Inhibition of Hedgehog/GLI signaling cascade. Front Chem. (2022) 10:1064191. doi: 10.3389/fchem.2022.1064191
31. Zhao Y, Cheng X, Wang G, Liao Y, Qing C. Linalool inhibits 22Rv1 prostate cancer cell proliferation and induces apoptosis. Oncol Lett. (2020) 20:289. doi: 10.3892/ol
32. Bu H, Tang S, Liu G, Miao C, Zhou X, Yang H, et al. In silico, in vitro and in vivo studies: Dibutyl phthalate promotes prostate cancer cell proliferation by activating Forkhead Box M1 and remission after Natura-α pretreatment. Toxicology. (2023) 488:153465. doi: 10.1016/j.tox.2023.153465
33. Liu YP, Wang Y, Jia Z, Zhang W, Zhang C. Difference in volatile components of Lonicerae Japonicae Flos of different origins: based on GC-MS and multivariate statistical analysis. Zhongguo Zhong Yao Za Zhi. (2022) 47:5508–19. doi: 10.19540/j.cnki.cjcmm.20220712.102
34. Nava Lauson CB, Tiberti S, Corsetto PA, Conte F, Tyagi P, Machwirth M, et al. Linoleic acid potentiates CD8(+) T cell metabolic fitness and antitumor immunity. Cell Metab. (2023) 35:633–50.e9. doi: 10.1016/j.cmet.2023.02.013
35. Yu MH, Hung TW, Wang CC, Wu SW, Yang TW, Yang CY, et al. Neochlorogenic acid attenuates hepatic lipid accumulation and inflammation via regulating miR-34a in vitro. Int J Mol Sci. (2021) 22:13163. doi: 10.3390/ijms222313163
36. Che J, Zhao T, Liu W, Chen S, Yang G, Li X, et al. Neochlorogenic acid enhances the antitumor effects of pingyangmycin via regulating TOP2A. Mol Med Rep. (2021) 23:158. doi: 10.3892/mmr.2020.11797
37. Chen K, Peng C, Chi F, Yu C, Yang Q, Li Z. Antibacterial and antibiofilm activities of chlorogenic acid against yersinia enterocolitica. Front Microbiol. (2022) 13:885092. doi: 10.3389/fmicb.2022.885092
38. Chaowuttikul C, Palanuvej C, Ruangrungsi N. Pharmacognostic Specification, Chlorogenic Acid Content, and In vitro Antioxidant Activities of Lonicera japonica Flowering Bud. Pharmacognosy Res. (2017) 9:128–32. doi: 10.4103/pr.pr11716
39. Wang HN, Shen Z, Liu Q, Hou XY, Cao Y, Liu DH, et al. Isochlorogenic acid (ICGA): natural medicine with potentials in pharmaceutical developments. Chin J Nat Med. (2020) 18:860–71. doi: 10.1016/S1875-5364(20)60029-2
40. Naveed M, Hejazi V, Abbas M, Kamboh AA, Khan GJ, Shumzaid M, et al. Chlorogenic acid (CGA): A pharmacological review and call for further research. BioMed Pharmacother. (2018) 97:67–74. doi: 10.1016/j.biopha.2017.10.064
41. Yu J, Wang K, Zhao H, Chen L, Wang X. Bioactive constituents from the leaves of Lonicera japonica. Fitoterapia. (2022) 162:105277. doi: 10.1016/j.fitote.2022.105277
42. Chen S, Wang X, Cheng Y, Gao H, Chen X. A review of classification, biosynthesis, biological activities and potential applications of flavonoids. Molecules. (2023) 28:4982. doi: 10.3390/molecules28134982
43. Xie Y, Yang W, Tang F, Chen X, Ren L. Antibacterial activities of flavonoids: structure-activity relationship and mechanism. Curr Med Chem. (2015) 22:132–49. doi: 10.2174/0929867321666140916113443
44. Han MH, Lee WS, Nagappan A, Hong SH, Jung JH, Park C, et al. Flavonoids isolated from flowers of lonicera japonica thunb. Inhibit inflammatory responses in BV2 microglial cells by suppressing TNF-α and IL-β Through PI3K/akt/NF-kb signaling pathways. Phytother Res. (2016) 30:1824–32. doi: 10.1002/ptr.5688
45. Wang Y, Liu XJ, Chen JB, Cao JP, Li X, Sun CD. Citrus flavonoids and their antioxidant evaluation. Crit Rev Food Sci Nutr. (2022) 62:3833–54. doi: 10.1080/10408398.2020.1870035
46. Masad RJ, Haneefa SM, Mohamed YA, Al-Sbiei A, Bashir G, Fernandez-Cabezudo MJ, et al. The immunomodulatory effects of honey and associated flavonoids in cancer. Nutrients. (2021) 13:1269. doi: 10.3390/nu13041269
47. Park MY, Kim Y, Ha SE, Kim HH, Bhosale PB, Abusaliya A, et al. Function and application of flavonoids in the breast cancer. Int J Mol Sci. (2022) 23:7732. doi: 10.3390/ijms23147732
48. Zhang YC, Deng J, Lin XL, Li YM, Sheng HX, Xia BH, et al. Use of ATR-FTIR spectroscopy and chemometrics for the variation of active components in different harvesting periods of lonicera japonica. Int J Anal Chem. (2022) 2022:8850914. doi: 10.1155/2022/8850914
49. Kong DX, Li YQ, Bai M, He HJ, Liang GX, Wu H. Correlation between the dynamic accumulation of the main effective components and their associated regulatory enzyme activities at different growth stages in Lonicera japonica Thunb. Ind Crops Products. (2017) 96:16–22. doi: 10.1016/j.indcrop.2016.11.024
50. Zhou Y, Huang Y, Ye W, Chen Z, Yuan Z. Cynaroside improved depressive-like behavior in CUMS mice by suppressing microglial inflammation and ferroptosis. BioMed Pharmacother. (2024) 173:116425. doi: 10.1016/j.biopha.2024.116425
51. Ding Y, Chen Y, Hu K, Yang Q, Li Y, Huang M. Sweroside alleviates hepatic steatosis in part by activating AMPK/mTOR-mediated autophagy in mice. J Cell Biochem. (2023) 124:1012–22. doi: 10.1002/jcb.30428
52. Wang J, Cai X, Ma R, Lei D, Pan X, Wang F. Anti-inflammatory effects of sweroside on LPS-induced ALI in mice via activating SIRT1. Inflammation. (2021) 44:1961–8. doi: 10.1007/s10753-021-01473-4
53. Kashiwada Y, Omichi Y, Kurimoto S, Shibata H, Miyake Y, Kirimoto T, et al. Conjugates of a secoiridoid glucoside with a phenolic glucoside from the flower buds of Lonicera japonica Thunb. Phytochemistry. (2013) 96:423–9. doi: 10.1016/j.phytochem.2013.09.021
54. Chen ZH, Zou QF, Jiang LJ, Liu CJ, Li JJ, Shi W, et al. The comparative analysis of Lonicerae Japonicae Flos and Lonicerae Flos: A systematical review. J Ethnopharmacol. (2024) 323:117697. doi: 10.1016/j.jep.2023.117697
55. Li H, Yu Y, Liu Y, Luo Z, Law BYK, Zheng Y, et al. Ursolic acid enhances the antitumor effects of sorafenib associated with Mcl-1-related apoptosis and SLC7A11-dependent ferroptosis in human cancer. Pharmacol Res. (2022) 182:106306. doi: 10.1016/j.phrs.2022.106306
56. Su F, Sui X, Xu J, Liu Q, Li J, Liu W, et al. Hederagenin suppresses ovarian cancer via targeting mitochondrial fission through dynamin-related protein 1. Eur J Pharmacol. (2024) 963:176188. doi: 10.1016/j.ejphar.2023.176188
57. Gao P, Huang X, Liao T, Li G, Yu X, You Y, et al. Daucosterol induces autophagic-dependent apoptosis in prostate cancer via JNK activation. Biosci Trends. (2019) 13:160–7. doi: 10.5582/bst.2018.01293
58. Son KH, Jung KY, Chang HW, Kim HP, Kang SS. Triterpenoid saponins from the aerial parts of Lonicera japonica. Phytochemistry. (1994) 35:1005–8. doi: 10.1016/S0031-9422(00)90656-3
59. Lin LM, Zhang XG, Zhu JJ, Gao HM, Wang ZM, Wang WH. Two new triterpenoid saponins from the flowers and buds of Lonicera japonica. J Asian Nat Prod Res. (2008) 10:925–9. doi: 10.1080/10286020802217366
60. Kwak WJ, Han CK, Chang HW, Kim HP, Kang SS, Son KH. Loniceroside C, an antiinflammatory saponin from Lonicera japonica. Chem Pharm Bull (Tokyo). (2003) 51:333–5. doi: 10.1248/cpb.51.333
61. Shen W-Z, Wei X, Li Y, Liu C, Ge L, Yao J, et al. A novel caffeoylquinic acid from lonicera japonica exerts cytotoxic activity by blocking the YAP-CTGF signaling pathway in hepatocellular carcinoma. Rev Bras Farmacognosia. (2023) 33:736 – 46. doi: 10.1007/s43450-023-00397-4
62. Yip EC, Chan AS, Pang H, Tam YK, Wong YH. Protocatechuic acid induces cell death in HepG2 hepatocellular carcinoma cells through a c-Jun N-terminal kinase-dependent mechanism. Cell Biol Toxicol. (2006) 22:293–302. doi: 10.1007/s10565-006-0082-4
63. Wan H, Ge L, Li J, Zhang K, Wu W, Peng S, et al. Effects of a novel biflavonoid of Lonicera japonica flower buds on modulating apoptosis under different oxidative conditions in hepatoma cells. Phytomedicine. (2019) 57:282–91. doi: 10.1016/j.phymed.2018.12.044
64. Park HS, Park KI, Lee DH, Kang SR, Nagappan A, Kim JA, et al. Polyphenolic extract isolated from Korean Lonicera japonica Thunb. induce G2/M cell cycle arrest and apoptosis in HepG2 cells: involvements of PI3K/Akt and MAPKs. Food Chem Toxicol. (2012) 50:2407–16. doi: 10.1016/j.fct.2012.04.034
65. Hwang YJ, Lee EJ, Kim HR, Hwang KA. Molecular mechanisms of luteolin-7-O-glucoside-induced growth inhibition on human liver cancer cells: G2/M cell cycle arrest and caspase-independent apoptotic signaling pathways. BMB Rep. (2013) 46:611–6. doi: 10.5483/BMBRep.2013.46.12.133
66. Fan SH, Wang YY, Lu J, Zheng YL, Wu DM, Li MQ, et al. Luteoloside suppresses proliferation and metastasis of hepatocellular carcinoma cells by inhibition of NLRP3 inflammasome. PloS One. (2014) 9:e89961. doi: 10.1371/journal.pone.0089961
67. Lee MA, Kim WK, Park HJ, Kang SS, Lee SK. Anti-proliferative activity of hydnocarpin, a natural lignan, is associated with the suppression of Wnt/β-catenin signaling pathway in colon cancer cells. Bioorg Med Chem Lett. (2013) 23:5511–4. doi: 10.1016/j.bmcl.2013.08.065
68. Hou N, Liu N, Han J, Yan Y, Li J. Chlorogenic acid induces reactive oxygen species generation and inhibits the viability of human colon cancer cells. Anticancer Drugs. (2017) 28:59–65. doi: 10.1097/CAD.0000000000000430
69. Sadeghi Ekbatan S, Li XQ, Ghorbani M, Azadi B, Kubow S. Chlorogenic acid and its microbial metabolites exert anti-proliferative effects, S-phase cell-cycle arrest and apoptosis in human colon cancer caco-2 cells. Int J Mol Sci. (2018) 19:723. doi: 10.3390/ijms19030723
70. Shimizu M, Yoshimi N, Yamada Y, Matsunaga K, Kawabata K, Hara A, et al. Suppressive effects of chlorogenic acid on N-methyl-N-nitrosourea-induced glandular stomach carcinogenesis in male F344 rats. J Toxicol Sci. (1999) 24:433–9. doi: 10.2131/jts.24.5_433
71. Ji J, Wang Z, Sun W, Li Z, Cai H, Zhao E, et al. Effects of cynaroside on cell proliferation, apoptosis, migration and invasion though the MET/AKT/mTOR axis in gastric cancer. Int J Mol Sci. (2021) 22:12125. doi: 10.3390/ijms222212125
72. Liao JC, Chang WT, Lan YH, Hour MJ, Lee HZ. Application of proteomics to identify the target molecules involved in Lonicera japonica-induced photokilling in human lung cancer CH27 cells. BMC Complement Altern Med. (2013) 13:244. doi: 10.1186/1472-6882-13-244
73. Zhou M, Shen S, Zhao X, Gong X. Luteoloside induces G(0)/G(1) arrest and pro-death autophagy through the ROS-mediated AKT/mTOR/p70S6K signalling pathway in human non-small cell lung cancer cell lines. Biochem Biophys Res Commun. (2017) 494:263–9. doi: 10.1016/j.bbrc.2017.10.042
74. Leung HW, Hour MJ, Chang WT, Wu YC, Lai MY, Wang MY, et al. P38-associated pathway involvement in apoptosis induced by photodynamic therapy with Lonicera japonica in human lung squamous carcinoma CH27 cells. Food Chem Toxicol. (2008) 46:3389–400. doi: 10.1016/j.fct.2008.08.022
75. Ho HY, Chen PJ, Lo YS, Lin CC, Chuang YC, Hsieh MJ, et al. Luteolin-7-O-glucoside inhibits cell proliferation and modulates apoptosis through the AKT signaling pathway in human nasopharyngeal carcinoma. Environ Toxicol. (2021) 36:2013–24. doi: 10.1002/tox.23319
76. Wang X, Liu J, Xie Z, Rao J, Xu G, Huang K, et al. Chlorogenic acid inhibits proliferation and induces apoptosis in A498 human kidney cancer cells via inactivating PI3K/Akt/mTOR signalling pathway. J Pharm Pharmacol. (2019) 71:1100–9. doi: 10.1111/jphp.13095
77. Lei S, Cao W, Zeng Z, Wang L, Lan J, Chen T. Cynaroside induces G1 cell cycle arrest by downregulating cell division cycle 25A in colorectal cancer. Molecules. (2024) 29:1508. doi: 10.3390/molecules29071508
78. Chen YK, Ngoc NTM, Chang HW, Su YF, Chen CH, Goan YG, et al. Chlorogenic acid inhibition of esophageal squamous cell carcinoma metastasis via EGFR/p-akt/snail signaling pathways. Anticancer Res. (2022) 42:3389–402. doi: 10.21873/anticanres.15826
79. Zhan Y, Li R, Feng C, Li X, Huang S, Wang L, et al. Chlorogenic acid inhibits esophageal squamous cell carcinoma growth in vitro and in vivo by downregulating the expression of BMI1 and SOX2. BioMed Pharmacother. (2020) 121:109602. doi: 10.1016/j.biopha.2019.109602
80. Li Y, Li X, Cuiping C, Pu R, Weihua Y. Study on the anticancer effect of an astragaloside- and chlorogenic acid-containing herbal medicine (RLT-03) in breast cancer. Evid Based Complement Alternat Med. (2020) 2020:1515081. doi: 10.1155/2020/1515081
81. Changizi Z, Moslehi A, Rohani AH, Eidi A. Chlorogenic acid induces 4T1 breast cancer tumor's apoptosis via p53, Bax, Bcl-2, and caspase-3 signaling pathways in BALB/c mice. J Biochem Mol Toxicol. (2021) 35:e22642. doi: 10.1002/jbt.22642
82. Li W, Ping Z, Xuemei G, Hongjuan M, Yi H, Xiaoli L, et al. Chlorogenic acid regulates the proliferation and migration of high-grade serous ovarian cancer cells through modulating the miR199a5p/DDR1 axis. Acta Biochim Pol. (2022) 69:855–64. doi: 10.18388/abp.2020_6381
83. Shao J, Wang C, Li L, Liang H, Dai J, Ling X, et al. Luteoloside inhibits proliferation and promotes intrinsic and extrinsic pathway-mediated apoptosis involving MAPK and mTOR signaling pathways in human cervical cancer cells. Int J Mol Sci. (2018) 19:1664. doi: 10.3390/ijms19061664
84. Chi Y, Shi L, Lu S, Cui H, Zha W, Shan L, et al. Inhibitory effect of Lonicera japonica-derived exosomal miR2911 on human papilloma virus. J Ethnopharmacol. (2024) 318::116969. doi: 10.1016/j.jep.2023.116969
85. Han XL, Li JD, Wang WL, Yang C, Li ZY. Sweroside eradicated leukemia cells and attenuated pathogenic processes in mice by inducing apoptosis. BioMed Pharmacother. (2017) 95:477–86. doi: 10.1016/j.biopha.2017.08.007
86. Park C, Lee WS, Han MH, Song KS, Hong SH, Nagappan A, et al. Lonicera japonica Thunb. Induces caspase-dependent apoptosis through death receptors and suppression of AKT in U937 human leukemic cells. Phytother Res. (2018) 32:504–13. doi: 10.1002/ptr.5996
87. Liang JY, Liu K. Total saponins of Honeysuckle on proliferation, invasion of thyroid cancer TPC-1 cells,apoptosis, and SHP2/Ras/MAPK pathway. J Sichuan Traditional Chin Med. (2023) 41:88–92. Available online at: https://kns.cnki.net/kcms2/article/abstract?v=01ddXewXOSACEdvPUtO2O--rTHk8-IUHdVYt7pSbGwECrny_eYJ36S_RWhTiExqHQOQb-4AWffeQUhfdgeUP3Q58jwzVxYET-XlmCwMzf9ua5B80RuulqlD4i-9nju3HFGp0JvvNusvw-7J2ISajn_yjBFP60MP8CkQaHr69_DqdsbwrRHrTvkpxcrsr_JdI&uniplatform=NZKPT&language=CHS.
88. Shan HZ, Dong S, Li XR, Dong YX, Liu XY, He Y, et al. Lonicera japonica extract enhances the tumor killing activity of anti-CD19 CAR-T cells. Cent South Pharmacy. (2022) 20:1545–9. doi: 10.7539/j.issn.1672-2981.2022.07.012
89. Liu YG, Liu YH, Jiang HQ. Inhibitory effect and mechanism of polysaccharide from lonicera japonica on mice bearing S180 sarcoma. J Chin Oncol. (2012) 18:584–7. doi: 10.11735/j.issn.1671-170X.2012.8.B007
90. Velmurugan BK, Lin JT, Mahalakshmi B, Chuang YC, Lin CC, Lo YS, et al. Luteolin-7-O-glucoside inhibits oral cancer cell migration and invasion by regulating matrix metalloproteinase-2 expression and extracellular signal-regulated kinase pathway. Biomolecules. (2020) 10:502. doi: 10.3390/biom10040502
91. He Y, Luo M, Lei S, Zeng Z, Chen T, Wu Y, et al. Luteoloside induces G0/G1 phase arrest of neuroblastoma cells by targeting p38 MAPK. Molecules. (2023) 28:1748. doi: 10.3390/molecules28041748
92. Pérez-Benavente B, Nasresfahani AF, Farràs R. Ubiquitin-regulated cell proliferation and cancer. Adv Exp Med Biol. (2020) 1233:3–28. doi: 10.1007/978-3-030-38266-7_1
93. Du H, Yang X, Fan J, Du X. Claudin 6: Therapeutic prospects for tumours, and mechanisms of expression and regulation (Review). Mol Med Rep. (2021) 24:677. doi: 10.3892/mmr
94. Wang L, Du H, Chen P. Chlorogenic acid inhibits the proliferation of human lung cancer A549 cell lines by targeting annexin A2 in vitro and in vivo. BioMed Pharmacother. (2020) 131:110673. doi: 10.1016/j.biopha.2020.110673
95. Sapio L, Salzillo A, Illiano M, Ragone A, Spina A, Chiosi E, et al. Chlorogenic acid activates ERK1/2 and inhibits proliferation of osteosarcoma cells. J Cell Physiol. (2020) 235:3741–52. doi: 10.1002/jcp.29269
96. Han K, Meng W, Zhang JJ, Zhou Y, Wang YL, Su Y, et al. Luteolin inhibited proliferation and induced apoptosis of prostate cancer cells through miR-301. Onco Targets Ther. (2016) 9:3085–94. doi: 10.2147/OTT
97. Chang X, Tamauchi S, Yoshida K, Yoshihara M, Yokoi A, Shimizu Y, et al. Downregulating vaccinia-related kinase 1 by luteolin suppresses ovarian cancer cell proliferation by activating the p53 signaling pathway. Gynecol Oncol. (2023) 173:31–40. doi: 10.1016/j.ygyno.2023.04.003
98. Dong X, Zheng T, Zhang Z, Bai X, Li H, Zhang J. [Luteolin reverses OPCML methylation to inhibit proliferation of breast cancer MDA-MB-231 cells]. Nan Fang Yi Ke Da Xue Xue Bao. (2020) 40:550–5. doi: 10.12122/j.issn.1673-4254.2020.04.16
99. Evan GI, Vousden KH. Proliferation, cell cycle and apoptosis in cancer. Nature. (2001) 411:342–8. doi: 10.1038/35077213
100. Jamasbi E, Hamelian M, Hossain MA, Varmira K. The cell cycle, cancer development and therapy. Mol Biol Rep. (2022) 49:10875–83. doi: 10.1007/s11033-022-07788-1
101. Khan F, Pandey P, Jha NK, Khalid M, Ojha S. Rutin mediated apoptotic cell death in caski cervical cancer cells via notch-1 and hes-1 downregulation. Life (Basel). (2021) 11:761. doi: 10.3390/life11080761
102. Huang L, Jin K, Lan H. Luteolin inhibits cell cycle progression and induces apoptosis of breast cancer cells through downregulation of human telomerase reverse transcriptase. Oncol Lett. (2019) 17:3842–50. doi: 10.3892/ol
103. Cai X, Ye T, Liu C, Lu W, Lu M, Zhang J, et al. Luteolin induced G2 phase cell cycle arrest and apoptosis on non-small cell lung cancer cells. Toxicol In Vitro. (2011) 25:1385–91. doi: 10.1016/j.tiv.2011.05.009
104. Wong RS. Apoptosis in cancer: from pathogenesis to treatment. J Exp Clin Cancer Res. (2011) 30:87. doi: 10.1186/1756-9966-30-87
105. Kulbay M, Paimboeuf A, Ozdemir D, Bernier J. Review of cancer cell resistance mechanisms to apoptosis and actual targeted therapies. J Cell Biochem. (2022) 123:1736–61. doi: 10.1002/jcb.30173
106. Kashyap D, Garg VK, Goel N. Intrinsic and extrinsic pathways of apoptosis: Role in cancer development and prognosis. Adv Protein Chem Struct Biol. (2021) 125:73–120. doi: 10.1016/bs.apcsb.2021.01.003
107. Qi BJ, Liu JL, Liu HJ, Liu TT, Ren Y, Zhu LL, et al. Effect of honeysuckle polysaccharides on the biological behavior of triple-negative breast cancer cells. J HeBei United University(Health Sci. (2024) 26:123–8,37. doi: 10.19539/j.cnki.2095-2694.2024.02.007
108. Li Y, Wang Y, Li L, Kong R, Pan S, Ji L, et al. Hyperoside induces apoptosis and inhibits growth in pancreatic cancer via Bcl-2 family and NF-κB signaling pathway both in vitro and in vivo. Tumour Biol. (2016) 37:7345–55. doi: 10.1007/s13277-015-4552-2
109. Jiang Y, Nan H, Shi N, Hao W, Dong J, Chen H. Chlorogenic acid inhibits proliferation in human hepatoma cells by suppressing noncanonical NF-κB signaling pathway and triggering mitochondrial apoptosis. Mol Biol Rep. (2021) 48:2351–64. doi: 10.1007/s11033-021-06267-3
110. McIlwain DR, Berger T, Mak TW. Caspase functions in cell death and disease. Cold Spring Harb Perspect Biol. (2015) 7:a026716. doi: 10.1101/cshperspect.a026716
111. Guon TE, Chung HS. Hyperoside and rutin of Nelumbo nucifera induce mitochondrial apoptosis through a caspase-dependent mechanism in HT-29 human colon cancer cells. Oncol Lett. (2016) 11:2463–70. doi: 10.3892/ol.2016.4247
112. Liu Z, Liu G, Liu X, Li S. The effects of hyperoside on apoptosis and the expression of Fas/FasL and survivin in SW579 human thyroid squamous cell carcinoma cell line. Oncol Lett. (2017) 14:2310–4. doi: 10.3892/ol.2017.6453
113. Gao L, Loveless J, Shay C, Teng Y. Targeting ROS-mediated crosstalk between autophagy and apoptosis in cancer. Adv Exp Med Biol. (2020) 1260:1–12. doi: 10.1007/978-3-030-42667-5_1
114. Kang KA, Piao MJ, Ryu YS, Hyun YJ, Park JE, Shilnikova K, et al. Luteolin induces apoptotic cell death via antioxidant activity in human colon cancer cells. Int J Oncol. (2017) 51:1169–78. doi: 10.3892/ijo.2017.4091
115. Wang Q, Wang H, Jia Y, Pan H, Ding H. Luteolin induces apoptosis by ROS/ER stress and mitochondrial dysfunction in gliomablastoma. Cancer Chemother Pharmacol. (2017) 79:1031–41. doi: 10.1007/s00280-017-3299-4
116. Mohi-Ud-Din R, Mir RH, Wani TU, Alsharif KF, Alam W, Albrakati A, et al. The regulation of endoplasmic reticulum stress in cancer: special focuses on luteolin patents. Molecules. (2022) 27:2471. doi: 10.3390/molecules27082471
117. Kwon K, Kwon YS, Kim SW, Yu K, Lee KH, Kwon OY. Luteolin-induced apoptosis through activation of endoplasmic reticulum stress sensors in pheochromocytoma cells. Mol Med Rep. (2017) 16:380–6. doi: 10.3892/mmr.2017.6582
118. Choi AY, Choi JH, Yoon H, Hwang KY, Noh MH, Choe W, et al. Luteolin induces apoptosis through endoplasmic reticulum stress and mitochondrial dysfunction in Neuro-2a mouse neuroblastoma cells. Eur J Pharmacol. (2011) 668:115–26. doi: 10.1016/j.ejphar.2011.06.047
119. Valastyan S, Weinberg RA. Tumor metastasis: molecular insights and evolving paradigms. Cell. (2011) 147:275–92. doi: 10.1016/j.cell.2011.09.024
120. Gundamaraju R, Lu W, Paul MK, Jha NK, Gupta PK, Ojha S, et al. Autophagy and EMT in cancer and metastasis: Who controls whom? Biochim Biophys Acta Mol Basis Dis. (2022) 1868:166431. doi: 10.1016/j.bbadis.2022.166431
121. Fu LQ, Du WL, Cai MH, Yao JY, Zhao YY, Mou XZ. The roles of tumor-associated macrophages in tumor angiogenesis and metastasis. Cell Immunol. (2020) 353:104119. doi: 10.1016/j.cellimm.2020.104119
122. Greten FR, Grivennikov SI. Inflammation and cancer: triggers, mechanisms, and consequences. Immunity. (2019) 51:27–41. doi: 10.1016/j.immuni.2019.06.025
123. Pandurangan AK, Dharmalingam P, Sadagopan SK, Ganapasam S. Luteolin inhibits matrix metalloproteinase 9 and 2 in azoxymethane-induced colon carcinogenesis. Hum Exp Toxicol. (2014) 33:1176–85. doi: 10.1177/0960327114522502
124. Li T, Fu X, Tse AK, Guo H, Lee KW, Liu B, et al. Inhibiting STAT3 signaling is involved in the anti-melanoma effects of a herbal formula comprising Sophorae Flos and Lonicerae Japonicae Flos. Sci Rep. (2017) 7:3097. doi: 10.1038/s41598-017-03351-2
125. Ashrafizadeh M, Dai J, Torabian P, Nabavi N, Aref AR, Aljabali AAA, et al. Circular RNAs in EMT-driven metastasis regulation: modulation of cancer cell plasticity, tumorigenesis and therapy resistance. Cell Mol Life Sci. (2024) 81:214. doi: 10.1007/s00018-024-05236-w
126. Yu JK, Yue CH, Pan YR, Chiu YW, Liu JY, Lin KI, et al. Isochlorogenic Acid C Reverses Epithelial-Mesenchymal Transition via Down-regulation of EGFR Pathway in MDA-MB-231 cells. Anticancer Res. (2018) 38:2127–35. doi: 10.21873/anticanres.12453
127. Zang M, Hu L, Zhang B, Zhu Z, Li J, Zhu Z, et al. Luteolin suppresses angiogenesis and vasculogenic mimicry formation through inhibiting Notch1-VEGF signaling in gastric cancer. Biochem Biophys Res Commun. (2017) 490:913–9. doi: 10.1016/j.bbrc.2017.06.140
128. Hajimehdipoor H, Tahmasvand Z, Nejad FG, Maresca M, Rajabi S. Rutin promotes proliferation and orchestrates epithelial-mesenchymal transition and angiogenesis in MCF-7 and MDA-MB-231 breast cancer cells. Nutrients. (2023) 15:2884. doi: 10.3390/nu15132884
129. Elinav E, Nowarski R, Thaiss CA, Hu B, Jin C, Flavell RA. Inflammation-induced cancer: crosstalk between tumours, immune cells and microorganisms. Nat Rev Cancer. (2013) 13:759–71. doi: 10.1038/nrc3611
130. Kay J, Thadhani E, Samson L, Engelward B. Inflammation-induced DNA damage, mutations and cancer. DNA Repair (Amst). (2019) 83:102673. doi: 10.1016/j.dnarep.2019.102673
131. Lu X, Wo G, Li B, Xu C, Wu J, Jiang C, et al. The anti-inflammatory NHE-06 restores antitumor immunity by targeting NF-κB/IL-6/STAT3 signaling in hepatocellular carcinoma. BioMed Pharmacother. (2018) 102:420–7. doi: 10.1016/j.biopha.2018.03.099
132. Fan Y, Mao R, Yang J. NF-κB and STAT3 signaling pathways collaboratively link inflammation to cancer. Protein Cell. (2013) 4:176–85. doi: 10.1007/s13238-013-2084-3
133. Xia W, Khan I, Li XA, Huang G, Yu Z, Leong WK, et al. Adaptogenic flower buds exert cancer preventive effects by enhancing the SCFA-producers, strengthening the epithelial tight junction complex and immune responses. Pharmacol Res. (2020) 159:104809. doi: 10.1016/j.phrs.2020.104809
134. Zhou X, Dong Q, Kan X, Peng L, Xu X, Fang Y, et al. Immunomodulatory activity of a novel polysaccharide from Lonicera japonica in immunosuppressed mice induced by cyclophosphamide. PloS One. (2018) 13:e0204152. doi: 10.1371/journal.pone.0204152
135. Liu C, Xu M, Yan L, Wang Y, Zhou Z, Wang S. Honeysuckle-derived microRNA2911 inhibits tumor growth by targeting TGF-β1. Chin Med. (2022) 16:49. doi: 10.1186/s13020-021-00453-y
136. Sorice M. Crosstalk of autophagy and apoptosis. Cells. (2022) 11:1479. doi: 10.3390/cells11091479
137. Newton K, Strasser A, Kayagaki N, Dixit VM. Cell death. Cell. (2024) 187:235–56. doi: 10.1016/j.cell.2023.11.044
138. Li X, He S, Ma B. Autophagy and autophagy-related proteins in cancer. Mol Cancer. (2020) 19:12. doi: 10.1186/s12943-020-1138-4
139. Park SH, Park HS, Lee JH, Chi GY, Kim GY, Moon SK, et al. Induction of endoplasmic reticulum stress-mediated apoptosis and non-canonical autophagy by luteolin in NCI-H460 lung carcinoma cells. Food Chem Toxicol. (2013) 56:100–9. doi: 10.1016/j.fct.2013.02.022
140. Lee Y, Kwon YH. Regulation of apoptosis and autophagy by luteolin in human hepatocellular cancer Hep3B cells. Biochem Biophys Res Commun. (2019) 517:617–22. doi: 10.1016/j.bbrc.2019.07.073
141. Yu H, Lin L, Zhang Z, Zhang H, Hu H. Targeting NF-κB pathway for the therapy of diseases: mechanism and clinical study. Signal Transduct Target Ther. (2020) 5:209. doi: 10.1038/s41392-020-00312-6
142. Hoesel B, Schmid JA. The complexity of NF-κB signaling in inflammation and cancer. Mol Cancer. (2013) 12:86. doi: 10.1186/1476-4598-12-86
143. Ju W, Wang X, Shi H, Chen W, Belinsky SA, Lin Y. A critical role of luteolin-induced reactive oxygen species in blockage of tumor necrosis factor-activated nuclear factor-kappaB pathway and sensitization of apoptosis in lung cancer cells. Mol Pharmacol. (2007) 71:1381–8. doi: 10.1124/mol.106.032185
144. Sonoki H, Tanimae A, Endo S, Matsunaga T, Furuta T, Ichihara K, et al. Kaempherol and luteolin decrease claudin-2 expression mediated by inhibition of STAT3 in lung adenocarcinoma A549 cells. Nutrients. (2017) 9:597. doi: 10.3390/nu9060597
145. Cunningham R, Hansen CG. The Hippo pathway in cancer: YAP/TAZ and TEAD as therapeutic targets in cancer. Clin Sci (Lond). (2022) 136:197–222. doi: 10.1042/CS20201474
146. Zhang Y, Wang X. Targeting the Wnt/β-catenin signaling pathway in cancer. J Hematol Oncol. (2020) 13:165. doi: 10.1186/s13045-020-00990-3
147. Wagner EF, Nebreda AR. Signal integration by JNK and p38 MAPK pathways in cancer development. Nat Rev Cancer. (2009) 9:537–49. doi: 10.1038/nrc2694
148. Nazim UM, Park SY. Luteolin sensitizes human liver cancer cells to TRAIL−induced apoptosis via autophagy and JNK−mediated death receptor 5 upregulation. Int J Oncol. (2019) 54:665–72. doi: 10.3892/ijo.2018.4633
149. Liotti F, Kumar N, Prevete N, Marotta M, Sorriento D, Ieranò C, et al. PD-1 blockade delays tumor growth by inhibiting an intrinsic SHP2/Ras/MAPK signalling in thyroid cancer cells. J Exp Clin Cancer Res. (2021) 40:22. doi: 10.1186/s13046-020-01818-1
150. Chen ZB, Yao F, Lei XE, Zhang SB. Pharmacokinetics of flavonoid fraction of flos Lonicerae japonicae in rat by RRLC-MSn method. Chin J Hosp Pharmacy. (2010) 30:743–6. Available online at: https://kns.cnki.net/kcms2/article/abstract?v=01ddXewXOSAvIzQSRq3DEJaItiG8CeaIcbIeTLKq86JtUMbpyOOqT-9Opn_ar20N12VwRxy6PTDVUE5LKEZcLJ5AIXMr8nnApuf2iPxGy74buvdjbAH982W1LhyzdKkgN4oY6D456D_EA667KdiwUFgYn_MQVbDjdVUndlOXcM_DAcXunRcwQI1J9l-d4_1p&uniplatform=NZKPT&language=CHS.
151. Zhou Y, Zhou T, Pei Q, Liu S, Yuan H. Pharmacokinetics and tissue distribution study of chlorogenic Acid from lonicerae japonicae flos following oral administrations in rats. Evid Based Complement Alternat Med. (2014) 2014:979414. doi: 10.1155/2014/979414
152. Zhou YL, Zeng R, Pei Q, Liu SK. Pharmacokinetics and bioavailability of chlorogenic acid extracted from Jinyinhua in rats. Chin J Hosp Pharmacy. (2016) 36:164–7. doi: 10.13286/j.cnki.Chinhosppharmacyj.2016.03.02
153. Qiu F, Li Z, He L, Wang D. HPLC-ESI-MS/MS analysis and pharmacokinetics of luteoloside, a potential anticarcinogenic component isolated from Lonicera japonica, in beagle dogs. BioMed Chromatogr. (2013) 27:311–7. doi: 10.1002/bmc.2793
154. Huang LH, Xiong XH, Zhong YM, Cen MF, Cheng XG, Wang GX, et al. Pharmacokinetics of isochlorgenic acid C in rats by HPLC-MS: Absolute bioavailability and dose proportionality. J Ethnopharmacol. (2016) 185:105–9. doi: 10.1016/j.jep.2016.03.019
155. Zhan MR, Jia YQ, Chen L, Wang XL, Wang Y. [Analysis of metabolites of 4,5-dicaffeoylquinic acid in rat plasma and urine based on LC-MS]. Zhongguo Zhong Yao Za Zhi. (2020) 45:391–7. doi: 10.19540/j.cnki.cjcmm.20190516.501
156. Luo YD, Chen J, Cao J, Wen XD, Li P. Determination of sweroside in rat plasma and bile for oral bioavailability and hepatobiliary excretion. Chem Pharm Bull (Tokyo). (2009) 57:79–83. doi: 10.1248/cpb.57.79
157. Liu Z, Cheng Y, Chao Z. A comprehensive quality analysis of different colors of medicinal and edible honeysuckle. Foods. (2023) 12:3126. doi: 10.3390/foods12163126
158. Xu YC, Zhang JB, Jiang QA, Zhou LY, Miao HB. [Effects of water stress on the growth of Lonicera japonica and quality of honeysuckle]. Zhong Yao Cai. (2006) 29:420–3. doi: 10.13863/j.issn1001-4454.2006.05.002
159. Xiao O, Li M, Chen D, Chen J, Simal-Gandara J, Dai X, et al. The dissipation, processing factors, metabolites, and risk assessment of pesticides in honeysuckle from field to table. J Hazard Mater. (2022) 431:128519. doi: 10.1016/j.jhazmat.2022.128519
160. Yuan Y, Zhang LL, Liu JT, Zhang HB, Xu J, Zhang TJ. [Analysis and prediction of quality markers of Lonicerae Japonicae Flos]. Zhongguo Zhong Yao Za Zhi. (2022) 47:1730–8. doi: 10.19540/j.cnki.cjcmm.20211224.201
161. Zhang B, Nan TG, Zhan ZL, Kang LP, Yang J, Lai CJ, et al. A monoclonal antibody-based enzyme-linked immunosorbent assay for the determination of chlorogenic acid in honeysuckle. J Pharm BioMed Anal. (2018) 148:1–5. doi: 10.1016/j.jpba.2017.09.023
162. Zhang B, Nan T, Zhan Z, Kang L, Yang J, Yuan Y, et al. Development of a monoclonal antibody-based enzyme-linked immunosorbent assay for luteoloside detection in Flos Lonicerae Japonicae. Anal Bioanal Chem. (2016) 408:6053–61. doi: 10.1007/s00216-016-9396-0
163. Zhang B, Nan TG, Xin J, Zhan ZL, Kang LP, Yuan Y, et al. Development of a colloidal gold-based lateral flow dipstick immunoassay for rapid detection of chlorogenic acid and luteoloside in Flos Lonicerae Japonicae. J Pharm BioMed Anal. (2019) 170:83–8. doi: 10.1016/j.jpba.2019.03.035
164. Thanabhorn S, Jaijoy K, Thamaree S, Ingkaninan K, Panthong A. Acute and subacute toxicity study of the ethanol extract from Lonicera japonica Thunb. J Ethnopharmacol. (2006) 107:370–3. doi: 10.1016/j.jep.2006.03.023
165. Chi R. Acute toxicity of honeysuckle for injection. Med Diet Health. (2020) 18:33–4. Available online at: https://kns.cnki.net/kcms2/article/abstract?v=01ddXewXOSBy1DXo9uf9Sime799GtTQzu6QFnbxZ_Qjbs7hS0Kr6eTMDcCAbXe-HevNRLt43G54Hs5nOb3PP0Sr7cFJxuWWTazydS6m3fJNH6iULPiyMhMCtUHdVdM8fPNPlGbF64N1dnJ0tf2kkw3IdR0r2tJGixv4cyypGa7tieLE2H6taHUkUYC_DpAC5&uniplatform=NZKPT&language=CHS.
166. Hu X, Li WD, Zhang SF, Li O, Hao J, Mo C. Experimental study on anti-inflammatory effects and acute toxicity of aqueous extract from tetraploid Lonicerae Flos. Chinese Traditional and Herbal Drugs. (2015) 46:1649–52.
167. Zhang DP, Zhang YX. Study on toxicity test of honeysuckle lozenges. Strait Pharm J. (2006) 18:55–7. doi: 10.3969/j.issn.1006-3765.2006.04.028
168. Huang N, Gao HM, Chen LM, Zhang Y, Zhu JJ, Liu XQ, et al. Hemolytic effects of lonicerae japonicae flos and lonicerae flos in vitro. Chin J Exp Traditional Med Formulae. (2017) 23:6–12. doi: 10.13422/j.cnki.syfjx.2017120006
169. Wang YL, Li HY, Gao L. Clinical observation of modified Four Flavor—-Honeysuckle decoction combined with chemotherapy on central non—small cell lung cancer. Hebei J Traditional Chin Med. (2016) 38:541–4. doi: 10.3969/j.issn.1002-2619.2016.04.018
170. Sun L, Fang W, Hong Y, Jiang H. Observation on the effect of self-made honeysuckle solution washing nasal cavity after radiotherapy for nasopharyngeal carcinoma. Chin J Rural Med Pharmacy. (2023) 30:1–2. doi: 10.19542/j.cnki.1006-5180.007455
171. Lu HM. To observe the curative effect of Lonicerae japonicum decoction combined with aluminum and magnesium suspension in the treatment of esophagitis caused by radiotherapy of esophageal cancer. J Pract Traditional Chin Med. (2023) 39:1357–8. Available online at: https://kns.cnki.net/kcms2/article/abstract?v=01ddXewXOSBe0K-oGBBBmgG-mCmAOoebIyoiZlbuSrb88u7qsnUrbuSMI5C7hOmqitkmYsFTI-1tG8lR4Gjk9KhRkbBetEXdzuiYfZiz7ZI3Jpl0if9GaAyg02Rjmp1SwJMZ6UlOO_TWkVw4V6dAYI_74k0UHyJO2UF0sguA3LzWbY4nr96ODp3ipnY4jUed&uniplatform=NZKPT&language=CHS.
172. Song C, Du X, Yang Q, Zhang Z, Wan L, Yan J, et al. Effect of decoction of honeysuckle combined with dexamethasone on radiation esophagitis. J Modern Oncol. (2019) 27:220–3. doi: 10.3969/j.issn.1672-4992.2019.02.010
173. Du YL, Luo DG, Tan ZB, Hei JL. Clinical study on the prevention and treatment of Honeysuckle in skin toxicity induced by Cetuximab. China Modern Med. (2019) 26:214–7,31. doi: 10.3969/j.issn.1674-4721.2019.09.066
174. Zhang H, Xu L, Yang J, Li Q, Li Y. Oral Lonicerae Flos soaking solution for sorafenib treatment of primary. Modern J Integrated Traditional Chin Western Med. (2016) 25:3682–4. doi: 10.3969/j.issn.1008-8849.2016.33.011
175. Zhang X, Zhan Y. Honeysuckle prevention sola fini liver cancer patients with the investigation of the foot and skin reactions. J Nurses Training. (2013) 28:736–7. doi: 10.3969/j.issn.1002-6975.2013.08.029
176. Liu X, Yu J, Gao RZ, Gao W. Honeysuckle combined with Shengjigao to prevent advanced liver cancer patients taking. Nurs Pract Res. (2016) 13:149–51. doi: 10.3969/j.issn.1672-9676.2016.13.067
177. Jang CH, Moon N, Lee J, Kwon MJ, Oh J, Kim JS. Luteolin synergistically enhances antitumor activity of oxaliplatin in colorectal carcinoma via AMPK inhibition. Antioxidants (Basel). (2022) 11:626. doi: 10.3390/antiox11040626
178. Wang H, Luo Y, Qiao T, Wu Z, Huang Z. Luteolin sensitizes the antitumor effect of cisplatin in drug-resistant ovarian cancer via induction of apoptosis and inhibition of cell migration and invasion. J Ovarian Res. (2018) 11:93. doi: 10.1186/s13048-018-0468-y
179. Xu H, Yang T, Liu X, Tian Y, Chen X, Yuan R, et al. Luteolin synergizes the antitumor effects of 5-fluorouracil against human hepatocellular carcinoma cells through apoptosis induction and metabolism. Life Sci. (2016) 144:138–47. doi: 10.1016/j.lfs.2015.12.002
180. Yan Y, Li J, Han J, Hou N, Song Y, Dong L. Chlorogenic acid enhances the effects of 5-fluorouracil in human hepatocellular carcinoma cells through the inhibition of extracellular signal-regulated kinases. Anticancer Drugs. (2015) 26:540–6. doi: 10.1097/CAD.0000000000000218
181. Zhang L, Liu Q, Huang L, Yang F, Liu A, Zhang J. Combination of lapatinib and luteolin enhances the therapeutic efficacy of lapatinib on human breast cancer through the FOXO3a/NQO1 pathway. Biochem Biophys Res Commun. (2020) 531:364–71. doi: 10.1016/j.bbrc.2020.07.049
182. Liu Q, Zhu D, Hao B, Zhang Z, Tian Y. Luteolin promotes the sensitivity of cisplatin in ovarian cancer by decreasing PRPA1-medicated autophagy. Cell Mol Biol (Noisy-le-grand). (2018) 64:17–22. doi: 10.14715/cmb/2018.64.6.4
183. Zhu X, Ji M, Han Y, Guo Y, Zhu W, Gao F, et al. PGRMC1-dependent autophagy by hyperoside induces apoptosis and sensitizes ovarian cancer cells to cisplatin treatment. Int J Oncol. (2017) 50:835–46. doi: 10.3892/ijo.2017.3873
184. Sun T, Liu Y, Li M, Yu H, Piao H. Administration with hyperoside sensitizes breast cancer cells to paclitaxel by blocking the TLR4 signaling. Mol Cell Probes. (2020) 53:101602. doi: 10.1016/j.mcp.2020.101602
185. Vijay M, Sivagami G, Thayalan K, Nalini N. Radiosensitizing potential of rutin against human colon adenocarcinoma HT-29 cells. Bratisl Lek Listy. (2016) 117:171–8. doi: 10.4149/BLL_2016_033
186. Shi Y, Li F, Shen M, Sun C, Hao W, Wu C, et al. Luteolin prevents cardiac dysfunction and improves the chemotherapeutic efficacy of doxorubicin in breast cancer. Front Cardiovasc Med. (2021) 8:750186. doi: 10.3389/fcvm.2021.750186
187. Li Q, Ren L, Zhang Y, Gu Z, Tan Q, Zhang T, et al. P38 signal transduction pathway has more cofactors on apoptosis of SGC-7901 gastric cancer cells induced by combination of rutin and oxaliplatin. BioMed Res Int. (2019) 2019:6407210. doi: 10.1155/2019/6407210
188. Zhu F, Gao J, Zeng F, Lai Y, Ruan X, Deng G. Hyperoside protects against cyclophosphamide induced ovarian damage and reduced fertility by suppressing HIF-1α/BNIP3-mediated autophagy. BioMed Pharmacother. (2022) 156:113743. doi: 10.1016/j.biopha.2022.113743
189. Tu SH, Ho CT, Liu MF, Huang CS, Chang HW, Chang CH, et al. Luteolin sensitises drug-resistant human breast cancer cells to tamoxifen via the inhibition of cyclin E2 expression. Food Chem. (2013) 141:1553–61. doi: 10.1016/j.foodchem.2013.04.077
190. Wu HT, Liu YE, Hsu KW, Wang YF, Chan YC, Chen Y, et al. MLL3 induced by luteolin causes apoptosis in tamoxifen-resistant breast cancer cells through H3K4 monomethylation and suppression of the PI3K/AKT/mTOR pathway. Am J Chin Med. (2020) 48:1221–41. doi: 10.1142/S0192415X20500603
191. Ullah A, Lim SI. Plant extract-based synthesis of metallic nanomaterials, their applications, and safety concerns. Biotechnol Bioeng. (2022) 119:2273–304. doi: 10.1002/bit.28148
192. Qais FA, Shafiq A, Ahmad I, Husain FM, Khan RA, Hassan I. Green synthesis of silver nanoparticles using Carum copticum: Assessment of its quorum sensing and biofilm inhibitory potential against gram negative bacterial pathogens. Microb Pathog. (2020) 144:104172. doi: 10.1016/j.micpath.2020.104172
193. Mbatha LS, Akinyelu J, Chukwuma CI, Mokoena MP, Kudanga T. Current trends and prospects for application of green synthesized metal nanoparticles in cancer and COVID-19 therapies. Viruses. (2023) 15:741. doi: 10.3390/v15030741
194. Patil MP, Bayaraa E, Subedi P, Piad LLA, Tarte NH, Kim G-D. Biogenic synthesis, characterization of gold nanoparticles using Lonicera japonica and their anticancer activity on HeLa cells. J Drug Delivery Sci Technology. (2019) 51:83–90. doi: 10.1016/j.jddst.2019.02.021
195. Rajivgandhi GN, Chackaravarthi G, Ramachandran G, Manoharan N, Ragunathan R, Siddiqi MZ, et al. Synthesis of silver nanoparticle (Ag NPs) using phytochemical rich medicinal plant Lonicera japonica for improve the cytotoxicity effect in cancer cells. J King Saud Univ - Science. (2022) 34:101798. doi: 10.1016/j.jksus.2021.101798
196. Zhang Y, Cui L, Lu Y, He J, Hussain H, Xie L, et al. Characterization of silver nanoparticles synthesized by leaves of lonicera japonica thunb. Int J Nanomedicine. (2022) 17:1647–57. doi: 10.2147/IJN.S356919
197. Zhou X, Ying X, Wu L, Liu L, Wang Y, He Y, et al. Research progress of natural product photosensitizers in photodynamic therapy. Planta Med. (2024) 90:368–79. doi: 10.1055/a-2257-9194
198. Xiao Q, Wu J, Pang X, Jiang Y, Wang P, Leung AW, et al. Discovery and development of natural products and their derivatives as photosensitizers for photodynamic therapy. Curr Med Chem. (2018) 25:839–60. doi: 10.2174/0929867324666170823143137
199. Yao CS. Photosensitizing effect of two extracts from caulis lonicerae:a preliminary study. Chin J Laser Med Surg. (2006) 06:361–4. doi: 10.3969/j.issn.1003-9430.2006.06.005
200. Batool S, Sohail S, Ud Din F, Alamri AH, Alqahtani AS, Alshahrani MA, et al. A detailed insight of the tumor targeting using nanocarrier drug delivery system. Drug Deliv. (2023) 30:2183815. doi: 10.1080/10717544.2023.2183815
201. Dai J, Ashrafizadeh M, Aref AR, Sethi G, Ertas YN. Peptide-functionalized, -assembled and -loaded nanoparticles in cancer therapy. Drug Discovery Today. (2024) 29:103981. doi: 10.1016/j.drudis.2024.103981
202. Ulldemolins A, Seras-Franzoso J, Andrade F, Rafael D, Abasolo I, Gener P, et al. Perspectives of nano-carrier drug delivery systems to overcome cancer drug resistance in the clinics. Cancer Drug Resist. (2021) 4:44–68. doi: 10.20517/cdr
203. Dokania S, Joshi AK. Self-microemulsifying drug delivery system (SMEDDS)–challenges and road ahead. Drug Deliv. (2015) 22:675–90. doi: 10.3109/10717544.2014.896058
204. Chen L, Liu CS, Chen QZ, Wang S, Xiong YA, Jing J, et al. Characterization, pharmacokinetics and tissue distribution of chlorogenic acid-loaded self-microemulsifying drug delivery system. Eur J Pharm Sci. (2017) 100:102–8. doi: 10.1016/j.ejps.2017.01.011
205. Xiao C, Wang X, Shen J, Xia Y, Qiu S, Liang X, et al. Luteolin-loading her-2 nanospheres enhances targeting and therapeutic effects of breast cancer. J BioMed Nanotechnol. (2021) 17:1545–53. doi: 10.1166/jbn.2021.3137
206. Ye J, Gao Y, Ji M, Yang Y, Wang Z, Wang B, et al. Oral SMEDDS promotes lymphatic transport and mesenteric lymph nodes target of chlorogenic acid for effective T-cell antitumor immunity. J Immunother Cancer. (2021) 9:e002753. doi: 10.1136/jitc-2021-002753
207. Ou YJ. The therapeutic diet of honeysuckle. J Beneficial Readings Drug Inf Med Advices. (2017) 4:27. doi: 10.3969/j.issn.1007-2950.2017.04.024
208. Liu QH. A kind of palace warming soup. China Patent No 201710944637.X. Beijing: China National Intellectural Property Administration. (2018).
209. Bai SJ. Four kinds of medicinal food tea in summer. Nongcun Xinjishu. (2013) 000:44–5. doi: 10.3969/j.issn.1002-3542.2013.08.041
210. Lin FS. Winter cold tea remedy. Oriental Medicated Diet. (2006) 2):1. doi: CNKI:SUN:YSSL.0.2006-02-021
211. Wang S, Long S, Deng Z, Wu W. Positive role of chinese herbal medicine in cancer immune regulation. Am J Chin Med. (2020) 48:1577–92. doi: 10.1142/S0192415X20500780
212. Lin H, Liu J, Zhang Y. Developments in cancer prevention and treatment using traditional Chinese medicine. Front Med. (2011) 5:127–33. doi: 10.1007/s11684-011-0137-7
Keywords: Lonicerae Japonicae Flos, cancer, active ingredients, antitumor mechanisms, pharmacokinetics, quality control, clinical applications, medicine food homology
Citation: Ma P, Yuan L, Jia S, Zhou Z, Xu D, Huang S, Meng F, Zhang Z and Nan Y (2024) Lonicerae Japonicae Flos with the homology of medicine and food: a review of active ingredients, anticancer mechanisms, pharmacokinetics, quality control, toxicity and applications. Front. Oncol. 14:1446328. doi: 10.3389/fonc.2024.1446328
Received: 09 June 2024; Accepted: 12 August 2024;
Published: 09 September 2024.
Edited by:
Xuelin Zhou, Capital Medical University, ChinaReviewed by:
Leena Dhoble, University of Florida, United StatesJin Zhang, University of Mississippi Medical Center, United States
Xiaodong Zou, The Chinese University of Hong Kong, Shenzhen, China
Xianhao Zhou, University of Rhode Island, United States
Copyright © 2024 Ma, Yuan, Jia, Zhou, Xu, Huang, Meng, Zhang and Nan. This is an open-access article distributed under the terms of the Creative Commons Attribution License (CC BY). The use, distribution or reproduction in other forums is permitted, provided the original author(s) and the copyright owner(s) are credited and that the original publication in this journal is cited, in accordance with accepted academic practice. No use, distribution or reproduction is permitted which does not comply with these terms.
*Correspondence: Yi Nan, 20080011@nxmu.edu.cn; Zhe Zhang, Zhangzhe@zryhyy.com.cn