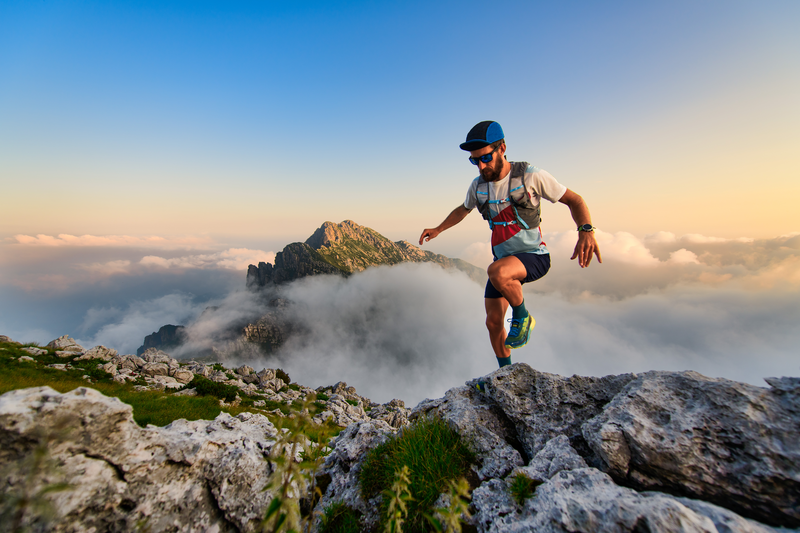
95% of researchers rate our articles as excellent or good
Learn more about the work of our research integrity team to safeguard the quality of each article we publish.
Find out more
ORIGINAL RESEARCH article
Front. Oncol. , 20 January 2025
Sec. Radiation Oncology
Volume 14 - 2024 | https://doi.org/10.3389/fonc.2024.1445558
Background: Radiotherapy can improve the survival rates of patients with glioma; meanwhile, impaired cognitive functions have been brought to the forefront with the offending organ, the radiosensitive hippocampus. This study aimed to assess the feasibility of hippocampus-sparing volumetric-modulated arc therapy (HS VMAT) in patients with World Health Organization (WHO) grade II glioma.
Methods: HS VMAT plans and non-hippocampus-sparing volumetric-modulated arc therapy (NHS VMAT) plans were generated using a computed tomography (CT) dataset of 10 patients who underwent postoperative radiotherapy. The dose volume histogram (DVH), homogeneity index (HI), conformity index (CI), and irradiated dose of the hippocampus and other organs at risk (OARs) were analyzed.
Results: No significant differences were observed in HI and CI between the two plans. Regarding the protection of OARs, HS VMAT plans were equally capable and even lowered the radiation dosages to the brainstem (35.56 vs. 41.74 Gy, p = 0.017) and spinal cord (1.34 vs. 1.43 Gy, p = 0.006). Notably, HS VMAT plans markedly decreased doses to the ipsilateral hippocampus and the contralateral hippocampus, demonstrating its efficacy in hippocampal dose reduction.
Conclusion: The HS VMAT plan can be used to efficiently lower the dosage delivered to the hippocampus and may, to some extent, help lessen the risk of cognitive damage. The encouraging results of our study need to be further validated by clinical trials to confirm the benefits of the HS VMAT plans in preserving cognitive functions in patients with glioma.
At present, gliomas, akin to those of regular glial cells histologically, are still one of the most common original malignant brain tumors (1). According to the Central Brain Tumor Registry of the United States (CBTRUS), there were 106,808 patients diagnosed with glioma in the USA from 2015 to 2019 (2). The World Health Organization (WHO) has classified glioma into four grades based on their pathological appearances, and the concept of low-grade glioma was defined as grade I or II glioma (3, 4). Low-grade gliomas are customarily deemed to be benign, for the symptoms are rare apart from seizures, but there is potential for cancerous growth yet (5, 6). The comprehensive treatment for low-grade glioma consists of surgery, chemotherapy, radiotherapy, and immunotherapy (7). Among these, radiotherapy (RT) is one of the clinically routine therapies for glioma, including conformal RT, intensity-modulated RT, stereotactic radiosurgery (SRS), et al. (8). Several studies have confirmed that RT can improve the survival rates of patients with glioma (9, 10). However, there are also side effects caused by RT, among which impaired cognitive functions have been brought to the forefront. Long-term follow-up research showed that receiving RT could result in impairments in attentional and cognitive functions in patients with low-grade glioma (11). High radiation dosages could result in defects in language fluency, executive capability, and speed in processing information (12). Moreover, an intervention review conducted by Theresa et al. drew a similar conclusion that patients with glioma who undergo RT may be more likely to experience cognitive impairments (13). In a prospective study that evaluated the hippocampal radiation dosages with cognitive functions’ changes after whole brain radiotherapy (WBRT) (14), the hippocampus was regarded as an essential construction for normal cognition in humans, which could be easily injured during RT and takes responsibility for cognitive impairments in these patients. Due to a more favorable prognosis and extended survival compared with patients with high-grade glioma, individuals diagnosed with low-grade glioma face an elevated risk of cognitive impairments. Reducing the radiation dose to the hippocampus helps protect patients’ cognitive functions (12), but the hippocampus is not usually considered an organ at risk (OAR) in clinical practice. A retrospective review conducted by Pinkham MB et al. revealed that hippocampal-sparing intensity-modulated radiotherapy (IMRT) can limit hippocampal Dmean to less than 30 Gy on at least one side of the hippocampus while maintaining the doses necessary for treating WHO grade II and III glioma (15).
In this study, hippocampal-sparing was defined as maintaining an average dose to the ipsilateral hippocampus adjacent to the tumor at no more than 30 Gy. Concurrently, the dose administered to the entirety of the contralateral hippocampus was restricted to not exceed 9 Gy, with the maximum dose constrained to remain below 16 Gy. Several techniques have been investigated in hippocampus-sparing VMAT for whole brain irradiation. Head-tilting technique adjusts the tilt angle of head by using a baseplate, leading to the removal of the lens from the beam pathway (16, 17). This method has demonstrated efficacy in hippocampal protection by significantly lowering the radiation dose to the hippocampus, which in turn reduces the potential for cognitive impairment. Split-arc partial-field VMAT (sapf-VMAT) techniques can also further reduce the dose to the hippocampus (18). The expansive irradiation field required for the whole brain target volume requires a broader jaw opening. This sapf-VMAT technique reduces the field size and solves the island blocking problem, which exists when ≥ 2 areas of whole brain target volume share the same multi-leaf collimator (MLC) leaf pair, and therefore it can shield the hippocampus more effectively. However, for gliomas, the target area is relatively small, the irradiation field only requires relatively small jaw opening. Hybrid sapf-VMAT with an improved beam arrangement ensures adequate dose delivery to the tumor target area while minimizing exposure to the hippocampus and other critical organs, providing a more beneficial treatment approach for patients (19).
The IMRT technique is routinely used in the treatment of glioma. However, a growing number of studies have found that volume-modulated arc therapy (VMAT) further improves dose conformity, reduces the radiation dose to surrounding normal tissues, and shortens treatment time compared with IMRT (20, 21). Beyond our expectation, there is no further research exploring whether we can minimize the radiation dose to the hippocampus of patients with WHO grade II glioma for the purpose of preserving their cognitive functions while using VMAT as the treatment.
Regarding the above-mentioned issue, this study aimed to assess the feasibility of hippocampus-sparing volumetric modulated arc therapy (HS VMAT) in patients diagnosed with WHO grade II glioma pathologically.
The CT datasets of ten patients with WHO grade II glioma diagnosed at the Cancer Hospital of Shantou University Medical College between 2014 and 2023 were included in this dosimetric planning analysis. Table 1 lists the characteristics of these cases, including gender, histological types of glioma, location, clinical target volume (CTV), ipsilateral hippocampus volume, and contralateral hippocampus volume. In all cases, the tumors were localized in either the left or right hemisphere. Therefore, a clear region of the ipsilateral and contralateral hippocampus could be delineated.
All patients were positioned in the supine position, while their heads were immobilized using a specialized thermoplastic cast. CT scans (with a slice thickness of 3 mm) were conducted from the uppermost region of the cranium’s scalp to the first cervical vertebrae (Philips Brilliance CT Big Bore Oncology Configuration, Cleveland, OH) using intravenous contrast injection. The aforementioned CT images were subsequently transferred to the Eclipse version 15.6 treatment planning system (Varian Medical System, Inc., Palo Alto, CA, USA) to enable target and organ-at-risk delineation as well as treatment planning.
All target volumes and organs at risk (OARs) were delineated by our radiation oncologists according to the 2022 NCCN Guidelines® in low-grade glioma. Targets and the OARs were localized on the basis of the CT images and modified according to the pre-treatment MRI images in fusion. Tumor volumes are defined using pre- and postoperative MRI imaging, usually T2 fluid-attenuated inversion recovery (FLAIR) and T1 post-contrast sequences, to define gross tumor volume (GTV). The CTV encompassed the surgical cavity, any residual regions exhibiting gadolinium enhancement as observed on T1-weighted images, and areas of hyperintensity identified on T2-weighted images, with an additional 1.5 cm margin to account for potential microscopic invasion. The CTV was delineated to exclude natural barriers, such as bone and the falx cerebri. The planning target volume (PTV) was defined by expanding the CTV by 0.3 cm in all directions. The hippocampal structure was delineated in accordance with the RTOG 0933 hippocampal atlas (22). The hippocampal structure was delineated in accordance with the RTOG 0933 hippocampal atlas. The hippocampal avoidance region was created by extending the hippocampal contour with a volumetric margin of 5 mm. In this study, the OARs were contoured following the 2022 NCCN Guidelines®, encompassing the eyeballs, lenses, optic nerves, optic chiasm, pituitary gland, and spinal cord. Notably, the hippocampus was classified as an OAR exclusively in the HS VMAT plans. When the PTV overlaps with critical structures like the brainstem and spinal cord, dose limitation to these OARs is prioritized. If the overlap involves less critical OARs like lenses, eyeballs, and the hippocampus, ensuring adequate PTV coverage takes precedence.
The VMAT technique using 6-MV photons from a TrueBeam (Varian Medical System, Inc., Pao Alto, CA) linear accelerator was employed on these patients. The VMAT plans using coplanar dual half arcs or dual full arcs with collimator 330°/30° were generated in Eclipse version 15.6 (Varian Medical Systems, Palo Alto, CA, USA) treatment planning systems. The median value of field size in X direction was 11.05 (range from 9.1 to 14.9) cm. Dose-limiting ring structures were generated to form the dose gradients surrounding the PTV. The Photon Optimizer (PO, version 15.6.06) algorithm was used for plan optimization. To avoid bias, the optimization objectives for both techniques were kept the same for each patient except that the priorities of the hippocampus were set as 0 for non-hippocampus-sparing (NHS) VMAT. Details of which can be found in the Supplementary Material. The Anisotropic Analytical Algorithm (AAA, version 15.6.06) was applied for final dose calculations, with a grid size of 2.5 mm. Each plan was normalized such that the prescribed dose covered 95% of PTV.
In the VMAT plans in this study, the prescribed dose for these patients was a total of 54 Gy administered in 30 fractions.
The aim of the treatment was to ensure that the PTV received a minimum of 95% and a maximum of 110% of the prescribed dose while minimizing the radiation exposure to the rest of the brain. The attainment of HS VMAT plans was determined by ensuring that the mean dose to the ipsilateral hippocampus did not exceed 30 Gy (23). Simultaneously, the dosage constraints for the contralateral hippocampus within this investigation mirrored those delineated in the RTOG 0933 study (22). Specifically, the dosage to 100% of the contralateral hippocampus was not permitted to surpass 9 Gy, and the maximum hippocampal dosage was capped at 16 Gy. Any dosage to 100% of the contralateral hippocampus that exceeded 10 Gy, or a maximum hippocampal dosage surpassing 17 Gy, was deemed an intolerable deviation, necessitating the revision of the treatment plan. Adequate coverage of the PTV was never compromised to achieve sufficient sparing of the hippocampal structures. Dose constraints to other OARs (such as the optic chiasm and brainstem) were prescribed as clinically indicated and prioritized over hippocampal constraints.
The evaluation of the treatment plans was conducted by considering the following parameters: dose volume histogram (DVH), homogeneity index (HI), conformity index (CI), PTVminus volume (defined as the planning target volume excluding the overlap with the brain stem PRV), PTVminus D98 (the dose covering 98% of the PTVminus volume), PTVminus D50 (the dose covering 50% of the PTVminus volume), PTVminus D2 (the dose covering 2% of the PTVminus volume), and PTVminus V54 (the volume covered by the prescription dose, excluding the overlap with the brain stem PRV). Given the brain stem’s vital role, we added a 0.3-0.5 cm buffer around it, termed the brain stem PRV.
The following formulas were used for the calculation of the HI and the CI:
In this study, D2, D50, and D98 denote the doses encompassing 2%, 50%, and 98% of the target volume, respectively. The prescribed dose across all treatment plans is 54 Gy. For the PTV, D2 and D98 were selected as representative of the approximate maximal and minimal doses, respectively, to assess regions of higher and lower dose concentrations, commonly referred to as hot and cold spots. The target volume (TV) covered by the reference isodose is denoted as TVRI, while VRI represents the volume of the reference isodose (24). Ideally, the homogeneity index (HI) should approach 0, signifying improved dose homogeneity with lower values, whereas the conformity index (CI) should ideally be 1, indicating enhanced conformality with higher values.
The parameters utilized to assess the radiation dosage in the ipsilateral, contralateral, and bilateral hippocampus encompassed Dmax, Dmean, Dmin, D100, and D40. The planning constraints for the OARs are specified as follows: Dmax should be less than 60 Gy for the brainstem PRV, less than 54 Gy for the brainstem, less than 8 Gy for the lens, and less than 45 Gy for the spinal cord; Dmean should be less than 50 Gy for the pituitary gland, and Dmax should be less than 50 Gy for the eyeballs, optic nerves, and optic chiasm.
In this context, Dx denotes the dose level that is achieved or surpassed in x% the specified volume. Meanwhile, V100% refers to the volume that receives a minimum of 100% of the prescribed dose. Additionally, Dmax signifies the maximum dose administered, whereas Dmean indicates the average dose delivered.
All of the HS VMAT plans and NHS VMAT plans were clinically acceptable. The dose distributions for the NHS VMAT plans and HS VMAT plans in one patient are shown in Figure 1. Table 2 displays the simulated radiation dose for the PTVminus in both plans. The CI and HI analyses were analyzed utilizing the paired-sample t-test. The HI (0.057 ± 0.014 vs. 0.046 ± 0.009, p=0.011*) and CI (0.91 ± 0.02 vs. 0.92 ± 0.02, p=0.071) of HS VMAT plans had no significant differences compared with NHS VMAT plans. The DVH of the PTV54 in one glioma case for NHS VMAT plans and HS VMAT plans is shown in Figure 2. The above results indicated that the HS VMAT plans were not inferior to the traditional NHS VMAT plans in terms of dose distribution.
Figure 1. View of dose distribution of the hippocampus contoured on CT-MRI fusion for one glioma case. (A) Hippocampal contour in transverse plane. (B) Hippocampal contour in sagittal plane. (C) HS VMAT plans. (D) NHS VMAT plans.
Figure 2. Dose-volume histograms (DVHs) comparison of PTV54 in one glioma case for NHS VMAT plans and HS VMAT plans.
The dosimetric outcomes for the various OARs in each treatment plan are presented in Table 3. In comparison to the standard VMAT plans, the HS VMAT plans demonstrated a statistically significant reduction in radiation doses to the brainstem (35.56 ± 16.67 vs. 41.74 ± 16.18, p=0.017) and spinal cord (1.34 ± 0.77 vs. 1.43 ± 0.80, p=0.006), without a significant increase in radiation exposure to other OARs, including the pituitary gland, eyeball, lens, and optic nerve. Despite notable differences between the two plans concerning the OARs for the right eyeball, left lens, and right optic nerve, the dosage constraints for these OARs complied with established guidelines. Figure 3 illustrates the DVH of the OARs in a glioma case for both NHS VMAT and HS VMAT plans.
Figure 3. Dose-volume histograms (DVHs) comparison of OARs in one glioma case for NHS VMAT plans and HS VMAT plans: (A) The dose-volume histogram for brainstem and brainstem PRV, (B) The dose-volume histogram for pituitary and spinal cord, (C) The dose-volume histograms for eyeballs, (D) The dose-volume histogram for lens, (E) The dose-volume histogram for optic nerves and optic chiasm.
The radiation dosages of the hippocampus were much lower in HS VMAT plans when compared with NHS VMAT plans. The accumulated dose to the ipsilateral, contralateral, and bilateral hippocampus under two different VMAT plans was analyzed. Details were reported in Table 4. For the ipsilateral hippocampus, HS VMAT plans reduced radiation doses to 7.80 ± 4.20 Gy (Dmin), 18.60 ± 8.52 Gy (Dmean), 34.28 ± 12.79 Gy (Dmax), 7.89 ± 4.08 Gy (D100), and 19.98 ± 9.63 Gy (D40) from higher doses seen in NHS VMAT plans. For the contralateral hippocampus, HS VMAT plans achieved reductions to 3.38 ± 1.12 Gy (Dmin), 4.86 ± 0.91 Gy (Dmean), 8.41 ± 1.38 Gy (Dmax), 3.42 ± 1.05 Gy (D100), and 4.89 ± 0.94 Gy (D40). Moreover, for the bilateral hippocampus, HS VMAT plans decreased radiation doses to 3.38 ± 1.12 Gy (Dmin), 11.52 ± 4.74 Gy (Dmean), 34.28 ± 12.79 Gy (Dmax), 3.42 ± 1.05 Gy (D100), and 12.54 ± 6.40 Gy (D40) compared to the higher doses in NHS VMAT plans. The DVH of the hippocampus in one glioma case for NHS VMAT plans and HS VMAT plans is shown in Figure 4.
Figure 4. Dose-volume histograms (DVHs) comparison of hippocampus in one glioma case for NHS VMAT plans and HS VMAT plans.
While radiotherapy has been established as a standard treatment for glioma, and patients with low-grade glioma have experienced improved long-term survival outcomes, it is imperative to minimize adverse effects, such as cognitive decline. This approach aims to enhance the quality of life for these patients.
This study focused exclusively on patients diagnosed with WHO grade II glioma, given that these lower-grade gliomas exhibit less aggressive and malignant characteristics compared to higher-grade gliomas. In contrast, patients with WHO grade III or IV glioma generally experience a limited survival period of only a few years post whole-brain radiotherapy (25–27). This duration may be inadequate for the emergence of significant cognitive deficits attributable to hippocampal damage, as such cognitive impairments typically develop over an extended period (28). In contrast, patients diagnosed with WHO grade II glioma may survive for several decades, during which hippocampal damage can result in considerable cognitive deterioration, imposing a substantial burden on both families and society. Since individuals with WHO grade I glioma typically do not require postoperative radiotherapy, they were excluded from this study (29).
The definite mechanisms of the decline in cognitive functions after radiotherapy remain unclear. Currently, there is a prevailing belief that radiation-induced damage to hippocampal neuron stem cells and alterations in the microenvironment may hold a significant influence. The dentate gyrus and subgranular zone of the hippocampi are the major regions where human neuron stem cells are, except for the subventricular zone (30). Physically, neuron stem cells undergo differentiation into granule cells in neurogenesis, resulting in the migration of these cells from the subgranular zone to the granular layer. These newly formed cells exhibit greater excitability compared to mature cells, enabling them to contribute to message integration, learning, memory, mood regulation, and other complex cognitive functions in humans (31). While undergoing RT, progenitor cells with greater potential for division and differentiation are more susceptible to influence, resulting in cognitive function impairments (30). Meanwhile, RT can induce the destabilization of the vascular endothelium, causing vascular rarefaction. Because of hippocampi’s dependence on blood flow integrity, decreased vascular density leads to incomplete differentiation of neuron stem cells, which could be reversed by systemic hypoxia (32). There are plenty of inflammatory cytokines accumulated in the hippocampi microenvironment after RT, including TNF-α, IL-1β, IL-6, NF-κb, prostaglandin E2, and so on, causing vascular injury and suppressing neurogenesis (33). In addition, inflammatory responses induced by RT may play irreplaceable roles in cognitive dysfunction. A novel finding revealed that radiation-induced NACHT, LRR, and PYD domain-containing protein 3 inflammasomes generate IL-1β, IL-18, and caspase-3 downstream, therefore causing hippocampal neuron pyroptosis. The above inflammatory signaling pathways could be inhibited by alternate-day fasting (34).
In this dosimetric feasibility study, ten patients with WHO grade II glioma were included. All of them had already been treated with standardized VMAT without hippocampus sparing. Therefore, bilateral hippocampi were defined as OARs, and we chose to generate inverse-planning VMAT with Eclipse version 15.6 retrospectively, owing to the restrictive dosage constraints in OARs. Evaluation of target dosage distribution in two radiotherapy plan indicators showed that there were no significant differences in HI and CI between the HS VMAT and the NHS VMAT plans. What excited us the most was the significant reduction in radiation dosages in the hippocampi area with the HS VMAT plans, including Dmax, Dmean, Dmin, D100, and D40. Gondi et al.’s research found that irradiating over 40% of the bilateral hippocampus with doses above 7.3 Gy led to long-term memory recall issues (35). However, in our study, it was not feasible to restrict the irradiation dose to less than 7.3 Gy for 40% of the bilateral hippocampus in all patients, due to the proximity of some tumors to the hippocampus and the presence of large tumor foci. Nonetheless, the HS VMAT plans effectively reduced the bilateral hippocampal D40 compared to the NHS VMAT plans. Furthermore, the HS VMAT plans significantly decreased radiation exposure to the brainstem and spinal cord, without substantially increasing the radiation dose to other OARs, such as the pituitary gland, eyeball, lens, and optic nerve, in comparison to the NHS VMAT plans. As for the brain stem, Dmax was 35.56 ± 16.67 Gy when using the HS VMAT plan, in contrast to 41.74 ± 16.18 in the NHS plan, with a P-value equal to 0.017. Additionally, Dmax in the spinal cord could be decreased to 1.35 ± 0.77 Gy in the HS VMAT plan, with a P-value of 0.006. This study revealed that the HS VMAT plan exhibited certain advantages over the NHS VMAT plan in radiotherapy for WHO grade II glioma.
The negative effects of irradiation on the hippocampi, or irradiation-tolerance, can be influenced by patient age, tumor location, radiation dose per fraction, interval between fractions, and specific radiotherapy techniques. When gliomas are near the hippocampi, the PTV may encroach on the protected area, potentially reducing the effectiveness of HS VMAT plans. It’s disappointing that there’s no agreed-upon dose limit for hippocampal radiation. A study by Tsai et al. found that administering over 7.45 Gy and 5.83 Gy to 50-100% of both hippocampi significantly affected verbal memory four months post-WBRT.[11]. According to a previous phase II study conducted by Gondi V et al. (22), the maximum dose to the hippocampus should be restricted to less than 16 Gy and the D100 to less than 9 Gy in patients with metastatic brain tumors receiving WBRT. However, the prescribed dose for a glioma radiotherapy plan is 54 Gy. Contemporary radiation treatments, including IMRT and VMAT, have considerable hurdles in reducing the maximum dosage to the tumor’s ipsilateral hippocampus to less than 16 Gy while keeping the D100 under 9 Gy. These limitations are mostly due to the tumor’s anatomical position, form, and size, as well as the dose restrictions imposed by the closeness of nearby normal tissues. Moreover, it has been shown that unilateral hippocampal damage has a lower risk of significant cognitive impairment compared to bilateral hippocampal damage (36). Meanwhile, Goda et al. showed that an average dose of less than 30 Gy to one side of the hippocampus reduced the risk of long-term neurocognitive decline in patients (23). Therefore, we believe that limiting the average dose to the ipsilateral hippocampus to less than 30 Gy is a reasonable choice to strike a balance between protection and treatment. Simultaneously, the dosage to 100% of the contralateral hippocampus was not permitted to surpass 9 Gy, and the maximum hippocampal dosage was capped at 16 Gy.
The controversy and improvement of radiation technology have never stopped in the past decades. In a dosimetric study containing 20 patients with high-grade glioma, Canyilmaz E et al. reported a significantly greater reduction in hippocampus doses in IMRT when compared with VMAT (21). Recent research suggests that VMAT provides improved PTV coverage and better preservation of OARs, excluding the hippocampi, in glioma radiation than IMRT (37). In line with these findings, our study shows that VMAT can successfully minimize the irradiation dosage to the hippocampus, obtaining levels lower than those reported in prior studies.
There was a non-negligible limitation in this dosimetric feasibility study, which needs to be taken into account. This study evaluates the differential impact of two different radiotherapy plans on hippocampal radiation dose in patients with WHO grade II glioma. Nevertheless, damage to hippocampal function is caused by a combination of factors, not just the high radiotherapy dose to which one is exposed. Furthermore, the appropriate dose constraints that ensure hippocampal protection without compromising treatment effectiveness remain to be delineated. These pivotal considerations mandate further exploration through clinical research. In addition, the sample size of our patients was not sufficient to generalize our findings to the general patient population, despite the impressive advantages shown by our simulation of HS VMAT plans. The promising outcomes from our study are needed for further verification through clinical trials in order to determine the potential benefits of preserving cognitive functions in glioma patients undergoing HS VMAT.
The use of the HS VMAT plan is a feasible approach for the radiotherapy plan of WHO grade II glioma, and it can successfully reduce the dose delivered to the hippocampus without appreciably deteriorating the HI, CI, or the dosage delivered to the OARs. As such, it might, to some extent, help reduce the risk of cognitive impairment. The encouraging results of our study need to be further validated by clinical trials to confirm the benefits of HS VMAT plans in preserving cognitive functions in patients with glioma.
The raw data supporting the conclusions of this article will be made available by the authors, without undue reservation.
The studies involving humans were approved by Ethical Commission of the Cancer Hospital of Shantou University Medical College. The studies were conducted in accordance with the local legislation and institutional requirements. Written informed consent for participation was not required from the participants or the participants’ legal guardians/next of kin in accordance with the national legislation and institutional requirements.
RX: Data curation, Project administration, Validation, Writing – original draft, Writing – review & editing. HH: Validation, Writing – original draft. QC: Project administration, Supervision, Writing – review & editing. JL: Funding acquisition, Supervision, Writing – review & editing. TC: Project administration, Supervision, Writing – review & editing. KX: Project administration, Validation, Writing – review & editing. JC: Project administration, Supervision, Writing – review & editing. CC: Data curation, Methodology, Project administration, Supervision, Writing – review & editing.
The author(s) declare financial support was received for the research, authorship, and/or publication of this article. This work was sponsored by Medical Scientific Research Foundation of Guangdong Province (A2022218) and Science and Technology Innovation Strategy Special Foundation (Vertical Collaborative Management Direction) Project of Guangdong Province (Shan Fu Ke (2018) No. 157).
Authors thank Home for Researchers editorial team (www.home-for-researchers.com) for improving the English language in this manuscript.
The authors declare that the research was conducted in the absence of any commercial or financial relationships that could be construed as a potential conflict of interest.
All claims expressed in this article are solely those of the authors and do not necessarily represent those of their affiliated organizations, or those of the publisher, the editors and the reviewers. Any product that may be evaluated in this article, or claim that may be made by its manufacturer, is not guaranteed or endorsed by the publisher.
The Supplementary Material for this article can be found online at: https://www.frontiersin.org/articles/10.3389/fonc.2024.1445558/full#supplementary-material
Supplementary Figure 1 | Optimization objectives of major structures for NHS-VMAT.
Supplementary Figure 2 | Optimization objectives of major structures for HS-VMAT.
1. Miller KD, Ostrom QT, Kruchko C, Patil N, Tihan T, Cioffi G, et al. Brain and other central nervous system tumor statistics, 2021. CA Cancer J Clin. (2021) 71:381–406. doi: 10.3322/caac.21693
2. Ostrom QT, Price M, Neff C, Cioffi G, Waite KA, Kruchko C, et al. CBTRUS statistical report: primary brain and other central nervous system tumors diagnosed in the United States in 2015-2019. Neuro Oncol. (2022) 24:v1–v95. doi: 10.1093/neuonc/noac202
3. Louis DN, Ohgaki H, Wiestler OD, Cavenee WK, Burger PC, Jouvet A, et al. The 2007 WHO classification of tumours of the central nervous system. Acta Neuropathol. (2007) 114:97–109. doi: 10.1007/s00401-007-0243-4
4. Louis DN, Perry A, Wesseling P, Brat DJ, Cree IA, Figarella-Branger D, et al. The 2021 WHO classification of tumors of the central nervous system: a summary. Neuro Oncol. (2021) 23:1231–51. doi: 10.1093/neuonc/noab106
5. Pallud J, Blonski M, Mandonnet E, Audureau E, Fontaine D, Sanai N, et al. Velocity of tumor spontaneous expansion predicts long-term outcomes for diffuse low-grade gliomas. Neuro Oncol. (2013) 15:595–606. doi: 10.1093/neuonc/nos331
6. Slegers RJ, Blumcke I. Low-grade developmental and epilepsy associated brain tumors: a critical update 2020. Acta Neuropathol Commun. (2020) 8:27. doi: 10.1186/s40478-020-00904-x
7. Youssef G, Miller JJ. Lower grade gliomas. Curr Neurol Neurosci Rep. (2020) 20:21. doi: 10.1007/s11910-020-01040-8
8. Wu W, Klockow JL, Zhang M, Lafortune F, Chang E, Jin L, et al. Glioblastoma multiforme (GBM): An overview of current therapies and mechanisms of resistance. Pharmacol Res. (2021) 171:105780. doi: 10.1016/j.phrs.2021.105780
9. Bitterman DS, MacDonald SM, Yock TI, Tarbell NJ, Wright KD, Chi SN, et al. Revisiting the role of radiation therapy for pediatric low-grade glioma. JCO. (2019) 37:3335–9. doi: 10.1200/JCO.19.01270
10. Lu VM, Welby JP, Laack NN, Mahajan A, Daniels DJ. Pseudoprogression after radiation therapies for low grade glioma in children and adults: A systematic review and meta-analysis. Radiother Oncol. (2020) 142:36–42. doi: 10.1016/j.radonc.2019.07.013
11. Douw L, Klein M, Fagel SS, van den Heuvel J, Taphoorn MJ, Aaronson NK, et al. Cognitive and radiological effects of radiotherapy in patients with low-grade glioma: long-term follow-up. Lancet Neurol. (2009) 8:810–8. doi: 10.1016/S1474-4422(09)70204-2
12. Haldbo-Classen L, Amidi A, Lukacova S, Wu LM, von Oettingen G, Lassen-Ramshad Y, et al. Cognitive impairment following radiation to hippocampus and other brain structures in adults with primary brain tumours. Radiother Oncol. (2020) 148:1–7. doi: 10.1016/j.radonc.2020.03.023
13. Lawrie TA, Gillespie D, Dowswell T, Evans J, Erridge S, Vale L, et al. Long-term neurocognitive and other side effects of radiotherapy, with or without chemotherapy, for glioma. Cochrane Database Syst Rev. (2019) 8:CD013047. doi: 10.1002/14651858.CD013047.pub2
14. Tsai P-F, Yang C-C, Chuang C-C, Huang T-Y, Wu Y-M, Pai P-C, et al. Hippocampal dosimetry correlates with the change in neurocognitive function after hippocampal sparing during whole brain radiotherapy: a prospective study. Radiat Oncol. (2015) 10:253. doi: 10.1186/s13014-015-0562-x
15. Pinkham MB, Bertrand KC, Olson S, Zarate D, Oram J, Pullar A, et al. Hippocampal-sparing radiotherapy: The new standard of care for World Health Organization grade II and III gliomas? J Clin Neurosci. (2014) 21:86–90. doi: 10.1016/j.jocn.2013.04.005
16. Oh SA, Yea JW, Park JW, Park J. Use of a head-tilting baseplate during volumetric-modulated arc therapy (VMAT) to better protect organs at risk in hippocampal sparing whole brain radiotherapy (HS-WBRT). PloS One. (2020) 15:e0232430. doi: 10.1371/journal.pone.0232430
17. Yuan C, Xu S, Li Y, Quan E, Chen D, Liang J, et al. Assessment of different head tilt angles in volumetric modulated arc therapy for hippocampus-avoidance whole-brain radiotherapy. Front Oncol. (2024) 14:1415471. doi: 10.3389/fonc.2024.1415471
18. Yuen AHL, Wu PM, Li AKL, Mak PCY. Volumetric modulated arc therapy (VMAT) for hippocampal-avoidance whole brain radiation therapy: planning comparison with Dual-arc and Split-arc partial-field techniques. Radiat Oncol. (2020) 15:42. doi: 10.1186/s13014-020-01488-5
19. Yuen AHL, Wu PM, Li AKL, Mak PCY. Hybrid split-arc partial-field volumetric modulated arc therapy: an improved beam arrangement for linear accelerator-based hippocampal-avoidance whole brain radiation therapy. Rep Pract Oncol Radiother. (2022) 27:352–9. doi: 10.5603/RPOR.a2022.0022
20. Canyilmaz E, Uslu GDH, Colak F, Hazeral B, Haciislamoglu E, Zengin AY, et al. Comparison of dose distributions hippocampus in high grade gliomas irradiation with linac-based imrt and volumetric arc therapy: a dosimetric study. SpringerPlus. (2015) 4:114. doi: 10.1186/s40064-015-0894-x
21. Davidson MTM, Masucci GL, Follwell M, Blake SJ, Xu W, Moseley DJ, et al. Single arc volumetric modulated arc therapy for complex brain gliomas: is there an advantage as compared to intensity modulated radiotherapy or by adding a partial arc? Technol Cancer Res Treat. (2012) 11:211–20. doi: 10.7785/tcrt.2012.500289
22. Gondi V, Pugh SL, Tome WA, Caine C, Corn B, Kanner A, et al. Preservation of memory with conformal avoidance of the hippocampal neural stem-cell compartment during whole-brain radiotherapy for brain metastases (RTOG 0933): a phase II multi-institutional trial. J Clin Oncol. (2014) 32:3810–6. doi: 10.1200/JCO.2014.57.2909
23. Goda JS, Dutta D, Krishna U, Goswami S, Kothavade V, Kannan S, et al. Hippocampal radiotherapy dose constraints for predicting long-term neurocognitive outcomes: mature data from a prospective trial in young patients with brain tumors. Neuro-Oncology. (2020) 22:1677–85. doi: 10.1093/neuonc/noaa076
24. Feuvret L, Noël G, Mazeron J-J, Bey P. Conformity index: A review. Int J Radiat OncologyBiologyPhysics. (2006) 64:333–42. doi: 10.1016/j.ijrobp.2005.09.028
26. Curran WJ, Scott CB, Horton J, Nelson JS, Weinstein AS, Fischbach AJ, et al. Recursive partitioning analysis of prognostic factors in three radiation therapy oncology group Malignant glioma trials. JNCI J Natl Cancer Instit. (1993) 85:704–10. doi: 10.1093/jnci/85.9.704
27. Buckner JC. Factors influencing survival in high-grade gliomas. Semin Oncol. (2003) 30:10–4. doi: 10.1053/j.seminoncol.2003.11.031
28. Bitterlich C, Vordermark D. Analysis of health-related quality of life in patients with brain tumors prior and subsequent to radiotherapy. Oncol Lett. (2017) 14:1841–6. doi: 10.3892/ol.2017.6310
29. Jung T-Y, Jung S, Moon J-H, Kim I-Y, Moon K-S, Jang W-Y. Early prognostic factors related to progression and Malignant transformation of low-grade gliomas. Clin Neurol Neurosurg. (2011) 113:752–7. doi: 10.1016/j.clineuro.2011.08.002
30. Hellström NAK, Björk-Eriksson T, Blomgren K, Kuhn HG. Differential recovery of neural stem cells in the subventricular zone and dentate gyrus after ionizing radiation. Stem Cells. (2009) 27:634–41. doi: 10.1634/stemcells.2008-0732
31. Anacker C, Hen R. Adult hippocampal neurogenesis and cognitive flexibility - linking memory and mood. Nat Rev Neurosci. (2017) 18:335–46. doi: 10.1038/nrn.2017.45
32. Warrington JP, Csiszar A, Mitschelen M, Lee YW, Sonntag WE. Whole brain radiation-induced impairments in learning and memory are time-sensitive and reversible by systemic hypoxia. PloS One. (2012) 7:e30444. doi: 10.1371/journal.pone.0030444
33. Makale MT, McDonald CR, Hattangadi-Gluth JA, Kesari S. Mechanisms of radiotherapy-associated cognitive disability in patients with brain tumours. Nat Rev Neurol. (2017) 13:52–64. doi: 10.1038/nrneurol.2016.185
34. Wu Q, Yu M, Wang Z, Ai X, Liu Z, Zeng J, et al. Alternate-day fasting for the protection of cognitive impairment in c57BL/6J mice following whole-brain radiotherapy. Neurochem Int. (2023) 162:105463. doi: 10.1016/j.neuint.2022.105463
35. Gondi V, Hermann BP, Mehta MP, Tomé WA. Hippocampal dosimetry predicts neurocognitive function impairment after fractionated stereotactic radiotherapy for benign or low-grade adult brain tumors. Int J Radiat OncologyBiologyPhysics. (2012) 83:e487–93. doi: 10.1016/j.ijrobp.2011.10.021
36. Yoo S-A, Rosenbaum RS, Tsotsos JK, Fallah M, Hoffman KL. Long-term memory and hippocampal function support predictive gaze control during goal-directed search. J Vision. (2020) 20:10. doi: 10.1167/jov.20.5.10
37. Shaffer R, Nichol AM, Vollans E, Fong M, Nakano S, Moiseenko V, et al. A comparison of volumetric modulated arc therapy and conventional intensity-modulated radiotherapy for frontal and temporal high-grade gliomas. Int J Radiat Oncol Biol Phys. (2010) 76:1177–84. doi: 10.1016/j.ijrobp.2009.03.013
Keywords: hippocampus-sparing, volumetric-modulated arc therapy, glioma, dosimetric feasibility, World Health Organization grade II
Citation: Xie R, Huang H, Cai Q, Lu J, Chen T, Xie K, Chen J and Chen C (2025) Hippocampus-sparing volume-modulated arc therapy in patients with World Health Organization grade II glioma: a feasibility study. Front. Oncol. 14:1445558. doi: 10.3389/fonc.2024.1445558
Received: 07 June 2024; Accepted: 31 December 2024;
Published: 20 January 2025.
Edited by:
Valerio Nardone, University of Campania Luigi Vanvitelli, ItalyReviewed by:
Adams Hei Long Yuen, Tung Wah College, Hong Kong SAR, ChinaCopyright © 2025 Xie, Huang, Cai, Lu, Chen, Xie, Chen and Chen. This is an open-access article distributed under the terms of the Creative Commons Attribution License (CC BY). The use, distribution or reproduction in other forums is permitted, provided the original author(s) and the copyright owner(s) are credited and that the original publication in this journal is cited, in accordance with accepted academic practice. No use, distribution or reproduction is permitted which does not comply with these terms.
*Correspondence: Chuangzhen Chen, Y3pjaGVuMkBzdHUuZWR1LmNu
Disclaimer: All claims expressed in this article are solely those of the authors and do not necessarily represent those of their affiliated organizations, or those of the publisher, the editors and the reviewers. Any product that may be evaluated in this article or claim that may be made by its manufacturer is not guaranteed or endorsed by the publisher.
Research integrity at Frontiers
Learn more about the work of our research integrity team to safeguard the quality of each article we publish.