- 1Medical Systems Biology Group, Lübeck Institute of Experimental Dermatology, University of Lübeck, Lübeck, Germany
- 2University Cancer Center Schleswig-Holstein, University Hospital of Schleswig- Holstein, Lübeck, Germany
- 3Department of Hematology and Oncology, University Hospital of Schleswig-Holstein, Lübeck, Germany
- 4Institut for Pathology, University Hospital of Schleswig-Holstein, Lübeck, Germany
- 5Department of Pathology, Christian-Albrechts-University, Kiel, Germany
- 6Institut for Haematopathology, Christian-Albrechts-University, Kiel, Germany
- 7Department of Radiology, University Hospital of Schleswig-Holstein, Lübeck, Germany
- 8Department of Internal Medicine III, Ameos Krankenhausgesellschaft Ostholstein, Eutin, Germany
Tenosynovial giant cell tumor (TGCT) is a rare type of tumor that originates from the synovium of joints and tendon sheaths. It is characterized by recurring genetic abnormalities, often involving the CSF1 gene. Common symptoms include pain and swelling, which are not specific to TGCT, so MRI and a pathological biopsy are needed for an accurate diagnosis. We report the case of a 45-year-old man who experienced painful swelling in his right hip for six months. Initially, this was diagnosed as Erdheim-Chester disease. However, whole exome sequencing (WES) and RNA-Sequencing revealed a CSF1::GAPDHP64 fusion, leading to a revised diagnosis of TGCT. The patient was treated with pegylated interferon and imatinib, which resulted in stable disease after three months. Single-cell transcriptome analysis identified seven distinct cell clusters, revealing that neoplastic cells expressing CSF1 attract macrophages. Analysis of ligand-receptor interactions showed significant communication between neoplastic cells and macrophages mediated by CSF1 and CSF1R. Our findings emphasize the importance of comprehensive molecular analysis in diagnosing and treating rare malignancies like TGCT.
Introduction
Tenosynovial giant cell tumor (TGCT), previously called pigmented villonodular tenosynovitis (PVNS) or giant cell tumor of the tendon sheath, is a rare mesenchymal neoplasm arising from the synovium of joints and tendon sheaths. It is molecularly characterized by recurrent genomic aberrations often involving the colony-stimulating factor 1 gene (CSF1) (1, 2). According to the 2020 WHO Classification of Soft Tissue and Bone Tumors, TGCT is a type of neoplasm that is locally aggressive (3). The symptoms of TGCT, including pain, tenderness, swelling, or limitation of motion, are not specific. Therefore, it is necessary to perform magnetic resonance imaging (MRI) and pathological biopsy to make an accurate diagnosis (4). Localized TGCT is a focal mass that typically surrounds or abuts the tendon, while the diffuse type often causes joint effusion or synovial fluid (5, 6). Sometimes, TGCT can resemble other soft tissue tumors on MRI, making diagnosis challenging (7).
Materials and methods
A bone marrow biopsy was obtained and immediately stored in a preservation buffer, Singleron, Germany. The cell suspension was then shipped overnight at 4°C to Singleron Labs in Cologne, Germany. Cell viability was assessed at Singleron Labs, and the sample with viability exceeding 80% were subsequently processed into single-cell suspensions using the sCelLiVE Tissue Dissociation Solution (#1190062, Singleron). Library preparation was carried out using the GEXSCOPE Single Cell RNA Library Kit on a microwell chip (SCOPE-chip) with barcoded beads. The finished library was paired-end sequenced on an Illumina NovaSeq.
Single-cell RNA-seq analysis pipeline
Raw gene expression matrices were generated by a custom pipeline combining ‘kallisto’ (v.0.46.1) and ‘bustools’ (v.0.46.1) using GRCh38 as human reference The output-filtered gene expression matrices were analyzed in ‘R’ (v.4.2.1), where empty droplets and doublets were removed for each sample using the packages ‘DropletUtils’ (v.1.8.0) and ‘doubletFinder’, and further analysis was performed using the ‘Seurat’ (v.4.3 (8)) package. Low-quality cells were excluded; cells with fewer than 200 features or less than 10% mitochondrial RNA were removed. Cell clusters were manually annotated using established marker genes from cited literature, complemented by automated approaches, including scType (9), based on the Human Cell Atlas. To identify and visualize the cell cross-talk among cells or between clusters, the R package ‘CellChat’ (v.1) was used according to the developer’s vignette [ https://github.com/sqjin/CellChat]. To identify molecular pathways that are differentially regulated between moderate and severe PCS patients, the PROGENy database was used (10). The PROGENy database, incorporating empirical activity weights from perturbation experiments for perturbation response genes, was used to estimate the activity of 14 signaling pathways. The pathway activity is statistically tested between moderate and severe PCS groups by incorporating a linear model (10).
Bulk RNA-sequencing
For RNA-sequencing, STAR ALIGNER (version 2.7.2b) were used. Additionally, fusion genes from RNA-seq data were identified by the application of STAR-FUSION (version 1.9.0) running in de novo reconstruction mode. The hg19 genome served as the reference genome. The validation of resulting fusion genes was performed by applying FUSIONCATCHER and FUSIONINSPECTOR.
Case presentation
A 45-year-old male patient presented with a six-month history of progressively painful swelling in his right hip joint. Histological evaluation of a biopsy prompted a preliminary diagnosis of Erdheim-Chester disease. However, the clinical and radiological appearance were atypical, see Figure 1. The femoral head MRI scans (Figures 1A, B) reveal bone and soft tissue infiltration by the tumor from various perspectives. Additionally, Figures 1C, D present the tumor tissue, characterized by an abundance of foamy macrophages and multinucleated giant cells, as seen from the hematoxylin and eosin stainings (C) and immunostaining for CD 68 (D).
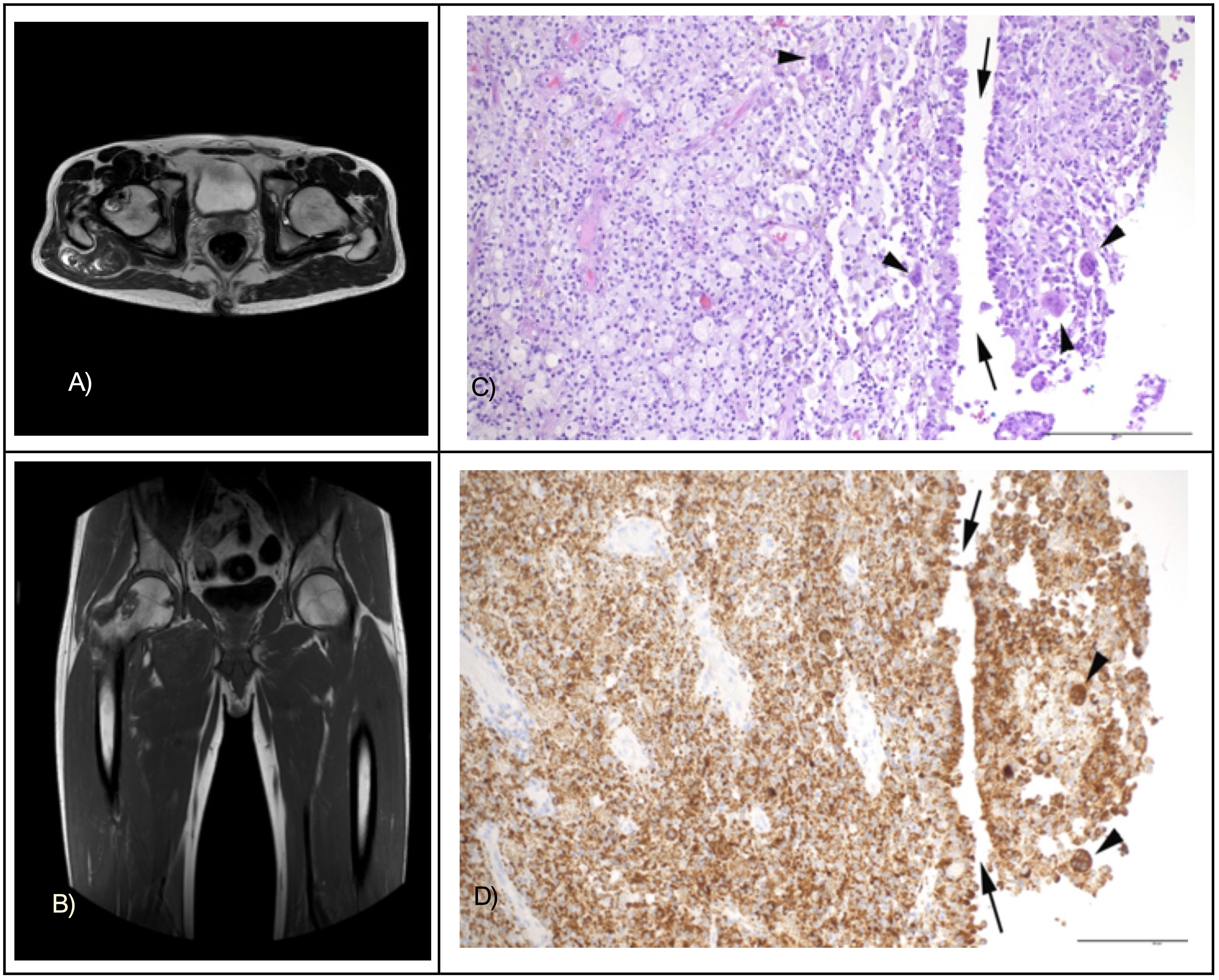
Figure 1. MRT images of the affected femoral head in the transverse plane (A) and frontal plane (B). Tumor tissue with a predominance of infiltrating foamy macrophages, cleft-like spaces (arrows), and multinucleated giant cells (arrowheads) in hematoxylin and eosin stainings (C) and immunostaining for CD68 (D). Original magnifications 200x, bar indicates 200 µm.
Due to the unclear diagnosis, the patient underwent whole exome sequencing (WES) of the primary tumor at the University-Cancer-Center-Schleswig-Holstein (UCCSH), using blood as a germline control. The results were presented at the local molecular tumor board (MTB). Variant calling excluded driver mutations in KRAS, NRAS, or BRAF, which would have been characteristic of Erdheim-Chester disease (11). Instead, WES identified 13 somatic mutations, two of which are known to be associated with cancer: a stopgain mutation (of unclear significance) in MAP2K2 p.Q218X and a recurrent nonsynonymous variant of uncertain significance in v p.C404Y (12). Further analysis of the mutational landscape found a low tumor mutational burden (0.85 mutations/MB) and microsatellite stability, with a notable deficiency in homologous recombination, scoring 66 (cut-off ≥ 42). Additionally, we assessed the BRCAness score based on the SBS6 tumor signature to predict responsiveness to PARP inhibitors but obtained a BRCAness score of 0.
Bulk RNA sequencing (RNA-seq) of the tumor tissue identified a CSF1::GAPDHP64 (1:109925202:: 1:116720422) fusion, a genomic aberration frequently found in TGCT. Based on the above evidence, the patient was re-diagnosed with TGCT driven by a CSFR1 fusion protein with characteristics of a histiocytic disorder. A surgical removal was not possible without large-scale mutilation. As diagnostic outcome from WES and RNA-seq analysis the following treatment options were proposed in decreasing preference: (i) Initiating interferon therapy (ii) administering pexidartinib, a tyrosine kinase inhibitor targeting the CSF1R pathway (iii) implementing therapy targeting MEK2 inhibition (iv) utilizing a tyrosine kinase inhibitor like imatinib (v) considering PARP inhibition supported by the pathological HRD score.
Based on the MTB recommendations, the patient was first treated with pegylated interferon. Six months into the interferon therapy, a restaging revealed a minimal reduction of the gluteal tumor mass and an increase in cystic tumor components. The next therapeutic option under consideration was the utilization of pexidartinib for the CSFR1 pathway. However, pexidartinib is not yet approved and therefore unavailable in the EU. Instead, therapy proceeded using imatinib, resulting in stable disease after 3 months.
Given the low incidence of TGCT, an understanding of tumor heterogeneity and its interaction with the microenvironment remains elusive. We, therefore, performed a single-cell transcriptome analysis of a tumor biopsy from the bone marrow. A total of 8,100 cells were obtained from one patient diagnosed with TGCT. Dimension reduction by Uniform Manifold Approximation And Projection (UMAP) of the gene expression matrix identified seven unique cell clusters (Figure 2A), which were identified as macrophages, T cells, proliferating cells, endothelial cells, myeloid dendritic cells, neoplastic cells, and giant cells. The marker genes of each cluster were: CD86, CD163 for macrophages; GFPT2, PDPN for neoplastic cells; CD3E, CD3D, TRBC2 for T cells; PECAM1, CD34 for endothelial cells, MMP-9 for giant cells (Figure 2B). The expression patterns of all seven clusters were analyzed to elucidate the location and expression of CSF1 and CSF1R. Figure 2B shows that CSF1 is exclusively expressed in the neoplastic cell type; and CSF1R is mainly found in macrophages, dendritic cells, T cells, and giant cells. These findings imply potential communication pathways linking neoplastic cell, dendritic cells, and T cells with macrophages. Previously, van IJzendoorn et al., 2022, identified a subset of tumor cells bearing a CSF1 translocation, exhibiting heightened CSF1 levels (13). High expression of CSF1 in this patient is restricted to neoplastic cells. Neoplastic cells highly expressing CSF1 attract and induce the proliferation of monocytes that express the receptor for CSF1 (CSF1R) on their surface (14). In our study CSF1R is highly expressed in the giant cells, and macrophages, but is absent from the neoplastic cells and other cell populations. Our study also observed that a major component of cells in TGCT consists of bystander macrophages responding to CSF1, Figure 2B.
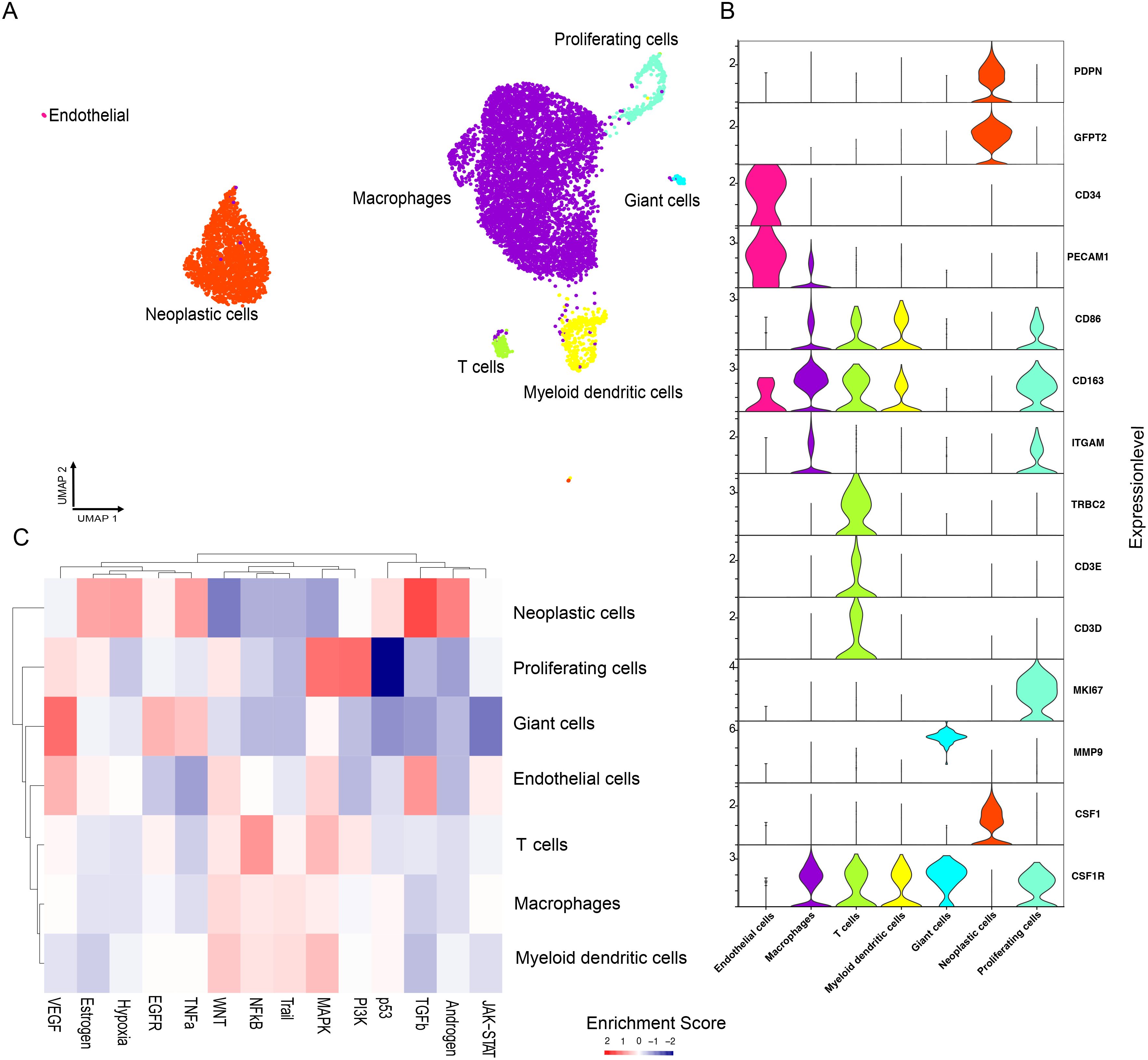
Figure 2. scRNA-seq analysis of cellular composition of bone marrow from Tenosynovial Giant Cell Tumor patient. Single-cell suspension from tenosynovial giant cell tumor patient analyzed by scRNA-seq. (A) UMAP plot of seven distinct cell clusters. (B) Violin plots of marker gene expression for the seven distinct clusters. (C) Relative pathway enrichment. Red indicates positive, whilst blue indicates negative enrichment.
The neoplastic cells have a significant impact on their surrounding environment by attracting a high number of macrophages. This is referred to as the “landscaping effect”. It is worth noting that CSF1R, which plays a crucial role in the growth of cells, is predominantly present in macrophages, dendritic cells, and T cells. Ligand-receptor interactions were investigated within neoplastic cells and other cell types using CellPhoneDB (15), revealing notably robust interactions between neoplastic cells and macrophages, while also revealing significant, albeit less pronounced interactions with giant cells, T cells, and myeloid dendritic cells mediated by CSF1 and CSF1R (Supplementary Figure S1). Our findings align with prior research, indicating that there are no direct CSF1 interactions with neoplastic cells, implying the lack of stimulation of neoplastic cells in TGCT through secreted CSF1 via an autocrine loop.
The putative upstream signaling pathways per cell type were assessed using PROGENy, which correlates known gene response patterns from upstream pathway activities with the observed gene expression. We observed a positive enrichment of pathways mainly in proliferating and platelet cells, comprising inflammatory signaling pathways (TGF-β), PI3K pathway, and MAP kinase signaling. Conversely, cell proliferation, linked to p53 signaling, exhibited negative enrichment, Figure 2C.
In summary, we report on a patient initially diagnosed with Erdheim-Chester disease. RNA-seq identified a CSF1 fusion, characteristic of TGCTs, leading to the correct diagnosis of the patient’s tumor. The TGCT literature predominantly consists of genetic studies, clinical case reports, and treatment strategies, yet precise analysis of its cellular heterogeneity remains challenging. To address this, our study conducted a comprehensive single-cell transcriptome analysis on a TGCT patient. The scRNA-seq analysis unveiled the complex cellular landscape and intratumoral diversity. Examination of all seven clusters indicated a predominant expression of CSF1 in neoplastic cells. Previously, Van IJzendoorn et al. (2022) identified tumor cells with heightened CSF1 levels due to a CSF1 translocation, attracting and stimulating monocytes expressing CSF1R (13). In our study, CSF1R was highly expressed in giant cells and macrophages, but absent in neoplastic cells. Bystander macrophages responding to CSF1 constitute a significant portion of cells in TGCTs, termed the “landscaping effect”. Ligand-receptor interactions investigated via CellPhoneDB showed robust interactions between neoplastic cells and macrophages, implying no direct CSF1 stimulation of neoplastic cells which is in line with previous findings. However, in atypical TGCTs, fusions were identified that are not associated with CSF1, indicating the presence of alternative pathogenic mechanisms that do not involve CSF1 (16).
In conclusion, extensive analysis by the molecular tumor board enabled here the final diagnosis and discovers new potential targets for this patient and MTB could be an avenue to diagnose and guide therapy for rare malignant diseases.
Data availability statement
The original contributions presented in the study are included in the article/Supplementary Material. Further inquiries can be directed to the corresponding author.
Ethics statement
The studies involving humans were approved by Ethics Committee, Universität zu Lübeck, Ratzeburger Allee 160, Building 21, 23562 Lübeck. The studies were conducted in accordance with the local legislation and institutional requirements. The participants provided their written informed consent to participate in this study. Written informed consent was obtained from the individual(s) for the publication of any potentially identifiable images or data included in this article.
Author contributions
AFä: Writing – original draft, Writing – review & editing. ZG: Writing – original draft, Writing – review & editing. YM: Writing – review & editing. FO: Writing – review & editing. HS: Validation, Writing – review & editing. SF: Writing – review & editing. NR: Writing – review & editing. AK: Writing – review & editing. NG: Writing – review & editing. MK: Writing – review & editing. NB: Writing – review & editing. JK: Writing – review & editing. V-WS: Writing – review & editing. CR: Writing – review & editing. BK: Writing – review & editing. WK: Writing – review & editing. AFr: Writing – review & editing. SM: Writing – review & editing. GH: Writing – review & editing. HB: Writing – review & editing. CK: Writing – review & editing.
Funding
The author(s) declare financial support was received for the research, authorship, and/or publication of this article. This work was supported by the Deutsche Forschungsgemeinschaft, the University of Lübeck and the UCCSH. AF and HB acknowledge support by the SFB 1526. HB acknowledge support by the BMBF project OUTLIVE-CRC (FKZ 01KD2103A).
Conflict of interest
The authors declare that the research was conducted in the absence of any commercial or financial relationships that could be construed as a potential conflict of interest.
The author(s) declared that they were an editorial board member of Frontiers, at the time of submission. This had no impact on the peer review process and the final decision.
Publisher’s note
All claims expressed in this article are solely those of the authors and do not necessarily represent those of their affiliated organizations, or those of the publisher, the editors and the reviewers. Any product that may be evaluated in this article, or claim that may be made by its manufacturer, is not guaranteed or endorsed by the publisher.
Supplementary material
The Supplementary Material for this article can be found online at: https://www.frontiersin.org/articles/10.3389/fonc.2024.1445427/full#supplementary-material
Supplementary Figure 1 | Ligand–receptor interactions for the neoplastic cells in TGCT. CellphoneDB identified interactions involving CSF1, between the neoplastic cell clusters and other cell clusters.
References
1. Mastboom MJL, Verspoor FGM, Verschoor AJ, Uittenbogaard D, Nemeth B, Mastboom WJB, et al. Higher incidence rates than previously known in tenosynovial giant cell tumors. Acta Orthopaedica. (2017) 88:688–94. doi: 10.1080/17453674.2017.1361126
2. Cupp JS, Miller MA, Montgomery KD, Nielsen TO, O’Connell JX, Huntsman D, et al. Translocation and expression of CSF1 in pigmented villonodular synovitis, tenosynovial giant cell tumor, rheumatoid arthritis and other reactive synovitides. Am J Surg Pathol. (2007) 31:970–6. doi: 10.1097/PAS.0b013e31802b86f8
3. Stacchiotti S, Dürr HR, Schaefer IM, Woertler K, Haas R, Trama A, et al. Best clinical management of tenosynovial giant cell tumour (TGCT): A consensus paper from the community of experts. Cancer Treat Rev. (2023) 112:102491. doi: 10.1016/j.ctrv.2022.102491
4. Verspoor FG, van der Geest IC, Vegt E, Veth RP, van der Graaf WT, Schreuder HW. Pigmented villonodular synovitis: current concepts about diagnosis and management. Future Oncol (London England). (2013) 9:1515–31. doi: 10.2217/fon.13.124
5. Wan JM, Magarelli N, Peh WC, Guglielmi G, Shek TW. Imaging of giant cell tumour of the tendon sheath. La Radiol Med. (2010) 115:141–51. doi: 10.1007/s11547-010-0515-2
6. Plotkin B, Sampath SC, Sampath SC, Motamedi K. MR imaging and US of the wrist tendons. Radiographics. (2016) 36:1688–700. doi: 10.1148/rg.2016160014
7. Lantos JE, Hameed M, Healey JH, Hwang S. Giant cell tumor of the tendon sheath mimicking a primary intramedullary metatarsal tumor. Skeletal Radiol. (2013) 42:589–93. doi: 10.1007/s00256-012-1533-z
8. Hao Y, Hao S, Andersen-Nissen E, Mauck WM 3rd, Zheng S, Butler A, et al. Integrated analysis of multimodal single-cell data. Cell. (2021) 184:3573–3587.e29. doi: 10.1016/j.cell.2021.04.048
9. Ianevski A, Giri AK, Aittokallio T. Fully-automated and ultra-fast cell-type identification using specific marker combinations from single-cell transcriptomic data. Nat Commun. (2022) 13:1246. doi: 10.1038/s41467-022-28803-w
10. Schubert M, Klinger B, Klünemann M, Sieber A, Uhlitz F, Saue S, et al. Perturbation-response genes reveal signaling footprints in cancer gene expression. Nat Commun. (2018) 9:20. doi: 10.1038/s41467-017-02391-6
11. Emile JF, Diamond EL, Hélias-Rodzewicz Z, Cohen-Aubart F, Charlotte F, Hyman DM, et al. Recurrent RAS and PIK3CA mutations in Erdheim-Chester disease. Blood. (2014) 124:3016–9. doi: 10.1182/blood-2014-04-570937
12. Tsuda Y, Hirata M, Katayama K, Motoi T, Matsubara D, Oda Y, et al. Massively parallel sequencing of tenosynovial giant cell tumors reveals novel CSF1 fusion transcripts and novel somatic CBL mutations. Int J Cancer. (2019) 145:3276–84. doi: 10.1002/ijc.32421
13. van IJzendoorn DGP, Matusiak M, Charville GW, Spierenburg G, Varma S, Colburg DRC, et al. Interactions in CSF1-driven tenosynovial giant cell tumors. Clin Cancer Res. (2022) 28:4934–46. doi: 10.1158/1078-0432.CCR-22-1898
14. Pierce JH, Di Marco E, Cox GW, Lombardi D, Ruggiero M, Varesio L, et al. Macrophage colony-stimulating factor (CSF-1) induces proliferation, chemotaxis, and reversible monocytic differentiation in myeloid progenitor cells transfected with the human c-fms/CSF-1 receptor cDNA. Proc Natl Acad Sci USA. (1990) 87:5613–7. doi: 10.1073/pnas.87.15.5613
15. Jin S, Guerrero-Juarez CF, Zhang L, Chang I, Ramos R, Kuan CH, et al. Inference and analysis of cell-cell communication using CellChat. Nat Commun. (2021) 12:1088. doi: 10.1038/s41467-021-21246-9
Keywords: single cell sequencing (scRNA-seq), RNA sequencing (RNA-seq), CSF1 fusion transcript, tenosynovial giant cell tumor (TGCT), molecular tumor board (MTB)
Citation: Fähnrich A, Gasimova Z, Maluje Y, Ott F, Sievert H, Fliedner S, Reimer N, Künstner A, Gebauer N, Kebenko M, von Bubnoff N, Kirfel J, Sailer V-W, Röcken C, Konukiewitz B, Klapper W, Frydrychowicz A, Mogadas S, Huebner G, Busch H and Khandanpour C (2024) Case report: Tenosynovial giant cell tumor. Front. Oncol. 14:1445427. doi: 10.3389/fonc.2024.1445427
Received: 07 June 2024; Accepted: 06 September 2024;
Published: 26 September 2024.
Edited by:
Eleanor Chen, University of Washington, United StatesReviewed by:
Paari Murugan, University of Minnesota Twin Cities, United StatesJosephine Dermawan, Cleveland Clinic, United States
Copyright © 2024 Fähnrich, Gasimova, Maluje, Ott, Sievert, Fliedner, Reimer, Künstner, Gebauer, Kebenko, von Bubnoff, Kirfel, Sailer, Röcken, Konukiewitz, Klapper, Frydrychowicz, Mogadas, Huebner, Busch and Khandanpour. This is an open-access article distributed under the terms of the Creative Commons Attribution License (CC BY). The use, distribution or reproduction in other forums is permitted, provided the original author(s) and the copyright owner(s) are credited and that the original publication in this journal is cited, in accordance with accepted academic practice. No use, distribution or reproduction is permitted which does not comply with these terms.
*Correspondence: Anke Fähnrich, YW5rZS5mYWVobnJpY2hAdWtzaC5kZQ==
†These authors share first authorship
‡These authors share last authorship