- 1Section for Cancer Cytogenetics, Institute for Cancer Genetics and Informatics, The Norwegian Radium Hospital, Oslo University Hospital, Oslo, Norway
- 2Department of Pathology, The Norwegian Radium Hospital, Oslo University Hospital, Oslo, Norway
- 3Institute of Clinical Medicine, Faculty of Medicine, University of Oslo, Oslo, Norway
- 4Department of Haematology, Oslo University Hospital, Rikshospitalet, Oslo, Norway
Background/Aim: Isodicentric [idic(X)(q13)] and isochromosome [i(X)(q10)] are infrequent aberrations in neoplastic diseases. The former is mainly reported in elderly women with myelodysplastic syndrome (MDS) and acute myeloid leukemia (AML), whereas the latter is mostly found as a secondary aberration or part of complex karyotypes in various types of neoplasms, including MDS and AML. Here, we present the molecular genetics and clinical features of six patients with myeloid neoplasia and the above-mentioned aberrations.
Patients and Methods: Array comparative genome hybridization (aCGH) and next-generation sequencing (NGS) myeloid panel were used to examine genetic alterations in five bone marrow samples containing neoplastic cells carrying idic(X)(q13) and one sample with i(X)(q10).
Results: The breakpoints of idic(X)(q13) were clustered within a 200 kbp region encompassing FAM236B, DMRTC1B, and DMRTC1. The breakpoint of i(X)(q10) was identified within a 112 kbp region on sub-band p11.22 containing SSX2, SSX2B, and SPANXN5. Pathogenic variants of TET2 were identified in four cases, SF3B1 in three cases, ASXL1 and SRSF2 in two cases each, whereas STAG2, RUNX1, U2AF1, and TP53 pathogenic variants were detected in only single cases.
Conclusions: The breakpoints of idic(X)(q13) are within a 200kbp. i(X)(q10) in our study turned out to be a cryptic idic(X)(p11) aberration, reported for the first time here. TET2, SF3B1, ASXL1, or SRSF2 were highly prevalent in patients with idic(X)(q13)/i(X)(q10) abnormalities and were often associated with a worse prognosis.
Introduction
An isodicentric chromosome with breakage and reunion at band Xq13, idic(X)(q13), and an isochromosome of the long arm of the chromosome X, i(X)(q10), are rare cytogenetic abnormalities in cancer (1, 2). The last update (April 15, 2024) of the “Mitelman Database of Chromosome Aberrations and Gene Fusions in Cancer” (1) contains 47 entries carrying an idic(X)(q13) and 55 entries with i(X)(q10). idic(X)(q13) has been predominantly observed in elderly women diagnosed with either myelodysplastic syndrome (MDS) or acute myeloid leukemia (AML), often representing the sole cytogenetic aberration in most cases (1, 3–8). Conversely, i(X)(q10) was found mostly as a secondary aberration within complex karyotypes across various neoplasms, including MDS and AML (1). Single cases of AML and MDS had i(X)(q10) as the only cytogenetic abnormality (9, 10).
Detailed characterization of the genomic breakpoint in band Xq13 has been reported in only a few cases of MDS/AML (5, 11, 12). MDS/AML patients with idic(X)(q13) were also found to carry additional sub-microscopic genetic aberrations in their bone marrow cells (5, 13). No investigation on possible additional genetic aberrations has been reported in cases with i(X)(q10). The primary consequence of i(X)(q10) is considered to be the loss of Xp and the gain of several genes on Xq. Moreover, additional genetic abnormalities, including the pathogenic variants of Tet methylcytosine dioxygenase 2 (TET2) gene, have been associated as a common secondary event in patients with idic(X)-positive myeloid malignancies (5).
Because of the rarity of myeloid neoplasms carrying idic(X)(q13) or i(X)(q10) and the incomplete understanding of their pathogenetic mechanisms, we here present the molecular cytogenetics and characterization of pathogenic variants in five patients of myeloid neoplasms with idic(X)(q13) and one patient with i(X)(q10).
Materials and methods
Materials
Information about the patients’ sex, age, diagnoses, and outcomes is given in Table 1. All patients were women between 63 and 86 years old. Flow cytometry was performed as part of the diagnostic routine (Table 1). The study was approved by the Regional Committee for Medical and Health Research Ethics (REK, project number 2010/1389; http://helseforskning.etikkom.no). All patient information has been de-identified.
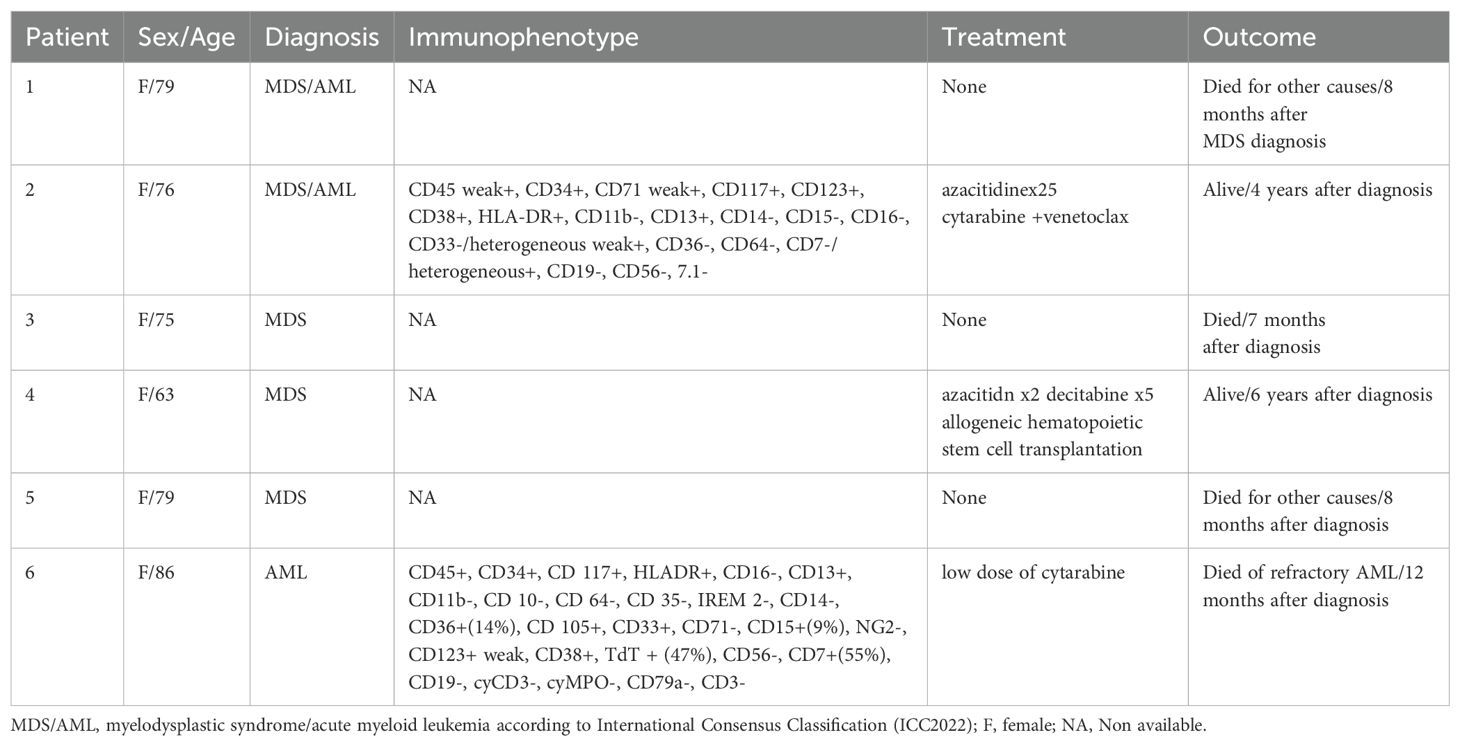
Table 1. Clinicopathological data and hematological findings of six patients with myeloid neoplasms.
Methods
G-banding and karyotyping
Bone marrow cells were short-term cultured and harvested; chromosomal preparations were stained for G-banding analysis and analyzed as previously described (14). The karyotypic description followed the International System of Cytogenomic Nomenclature (15). We received 1 to 5 samples for each patient to monitor the disease.
DNA extraction, array comparative genomic hybridization (aCGH), and detection of gene variants
DNA was extracted from bone marrow cells using the Maxwell 16 Instrument System and Maxwell 16 Cell DNA Purification Kit (Promega, Madison, WI, USA) or by using EZ1 Advanced XL (Qiagen, Hilden, Germany). The concentration was measured with a Quantus fluorometer (Promega) or Qubit fluorometer (Thermo Fischer Scientific, Waltham, MA, USA).
aCGH was performed using CytoSure array products (Oxford Gene Technology, Begbroke, Oxfordshire, UK) following the company’s protocols. The reference DNA was Promega’s human genomic female DNA. The slides (CytoSure Cancer +SNP array) were scanned with the Agilent Sure Scan Dx microarray scanner using Agilent Feature Extraction Software (version 12.1.1.1). Data were analyzed using the CytoSure Interpret analysis software (version 4.9.40). Annotations were based on the human reference sequence GRCh37/hg19.
Gene variants were detected using next-generation sequencing (NGS) panels designed for myeloid neoplasms. For cases 1, 2, and 5, the VariantPlex Myeloid Panel, including 75 genes, was used (Archer DX, Boulder, 2477 55th St #202, United States) and sequenced on the NextSeq 2000 (Illumina, San Diego, CA, United States). For cases 3, 4, and 6, the TruSight Myeloid sequencing panel (Illumina, San Diego, CA, United States), including 54 genes, was used and sequenced on the MiSeq (Illumina, San Diego, CA, United States). Data analyses were performed following the companies’ recommended software using Archer Analysis 6.2.7 or Variant Studio3.0, respectively. Annotations were based on the human reference sequence GRCh37/hg19 and pathogenicity was determined by using relevant databases: Molecular Tumor Board Portal (MTB) Portal (Karolinska Institutet), Human Somatic Mutation Database (HSMD; Qiagen), ClinVar (National Institute of Health), Catalogue of Somatic Mutations in Cancer (COSMIC; Sanger Institute) and the World Health Organization (WHO) classification (16).
Results
The six cases were selected because their bone marrow aberrant karyotypes included an idic(X)(q13) or i(X)(q10) (Table 2, Figure 1). Cases 1 to 5 harbored one or two copies of idic(X)(q13), whereas patient 6 carried i(X)(q10) as the sole aberration (Table 2). Table 2 shows karyotypic data from all samples we received.
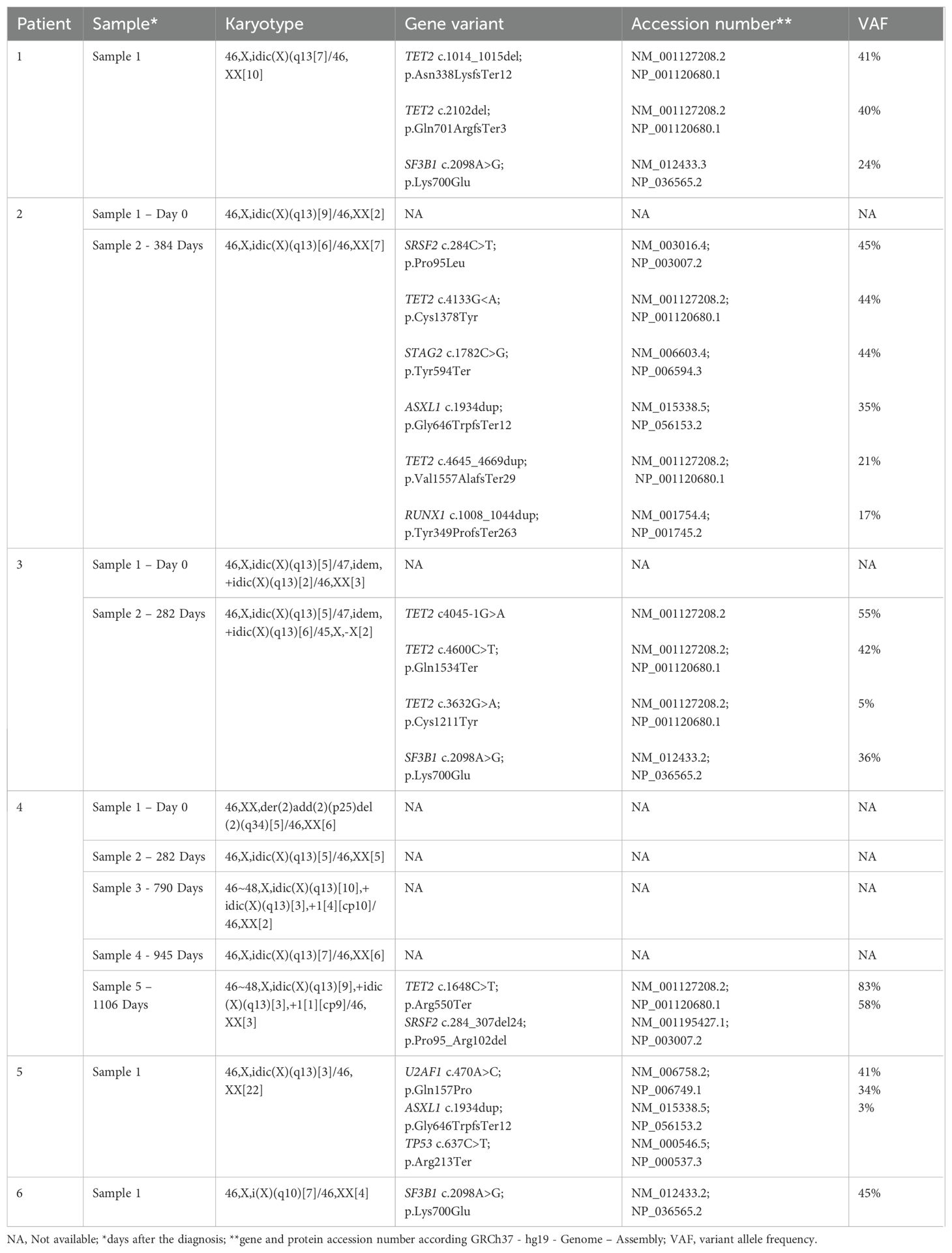
Table 2. Cytogenetic and pathogenic/likely pathogenic variants detected in the six patients with myeloid neoplasms.
aCGH analysis revealed that in cases 1 to 4, the breakpoints of idic(X)(q13) were within a 200 kbp region on chromosomal sub-band Xq13.2, encompassing the genes Family with Sequence Similarity 236 Member B (FAM236B), DMRT Like Family C1B (DMRTC1B), and DMRT Like Family C1 (DMRTC1) (Table 3, Figure 2). However, aCGH did not detect any genomic imbalances in case 5, likely due to the small size of the clone carrying idic(X)(q13). In case 6, with i(X)(q10) as the sole cytogenetic abnormality, aCGH showed that the breakpoint was within a 100 kbp region on chromosome sub-band Xp11.22 (genomic position ChrX:52,725,935-52,838,474). This region includes the genes SSX Family Member 2 (SSX2), SSX Family Member 2B (SSX2B), and SPANX Family Member N5 (SPANXN5) (Table 3, Figure 3).
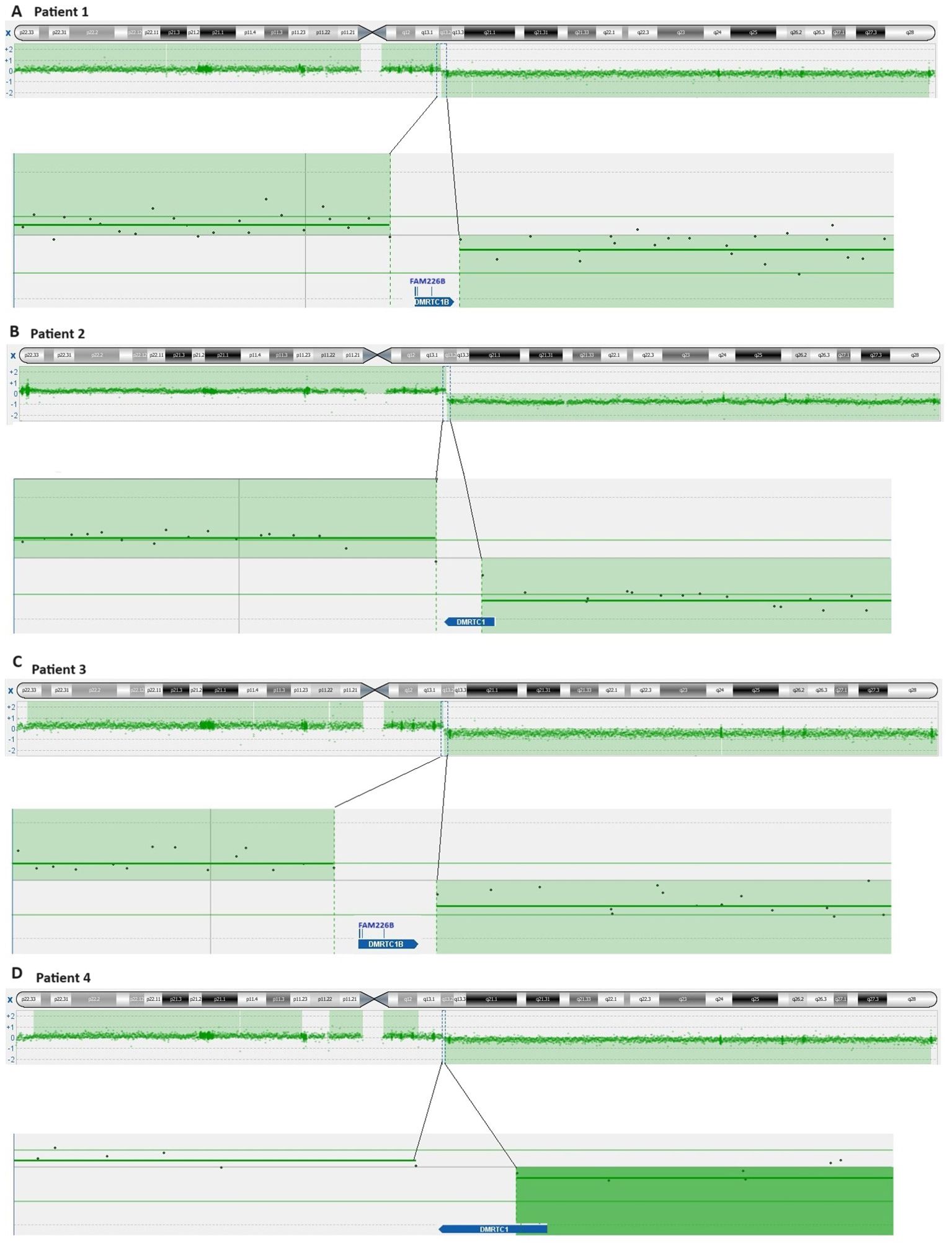
Figure 2. Ideogram of chromosome X and overview of genes clustering the breakpoint idic(X)(q13) according to the imbalances detected by aCGH. (A) Patient 1; (B) Patient 2; (C) Patient 3; (D) Patient 4.
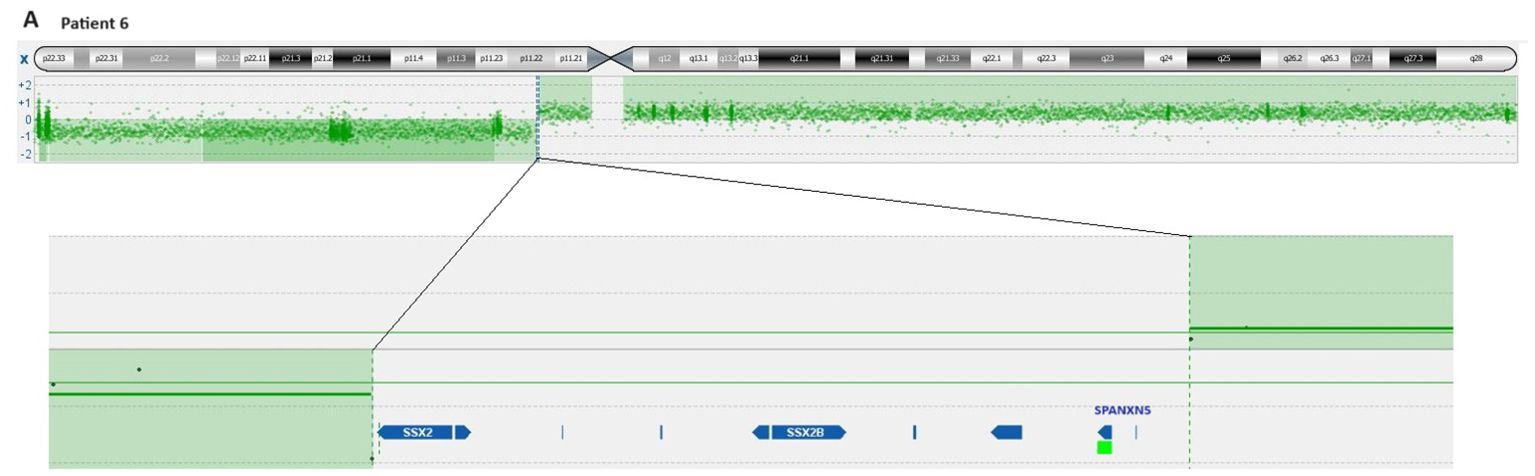
Figure 3. Ideogram of chromosome X according to the imbalances detected by aCGH and overview of genes clustering the cryptic breakpoint idic(X)(p11) in patient 6.
Using a myeloid NGS panel, all six cases were found to harbor genetic variants, classified as pathogenic/likely pathogenic, in more than one gene (Table 2). Specifically, four patients had variants in TET2 (cases 1-4), three had variants in SF3B1 (cases 1, 3, and 6), and two had variants in ASXL1 (cases 2 and 5) or SRSF2 (cases 2 and 4) (Table 2). The variant allele frequency (VAF) indicated that all samples had major and minor neoplastic clones carrying the pathogenic variants (Table 2).
Discussion
In our study, idic(X)(q13) was found as the sole chromosome abnormality in four out of the six patients (patients 1-3 and 5) (Table 2). The initial G-banding analysis of a bone marrow sample drawn from case 4 on day 0 showed a rearrangement involving chromosome 2 as the only clonal change (Table 2). However, upon re-evaluation of the karyotype, one metaphase was found to contain the idic(X)(q13) abnormality. Since this observation was not sufficient to confirm the aberration as clonal, the metaphase was not reported in the initial findings. In sample 5 of case 4, received at 1106 days after the diagnosis, the idic(X)(q13) abnormality was observed alongside an additional chromosome 1. Notably, this was the only instance where idic(X)(q13) was accompanied by other chromosomal aberrations (Table 2).
For patients 1-4, aCGH analysis detected the loss of an 83.6 Mbp region on the q arm of chromosome X, spanning from chromosome sub-band Xq13.2 to band Xq28, and the gain of a 72 Mbp region from Xp22.33 to Xq13.2. Additionally, the breakpoints on Xq13.2 were identified within the X:71,951,773–72,146,832 region. In case 5, aCGH analysis could not detect genomic imbalances, likely due to the presence of a small clone carrying idic(X)(q13) (only three out of the 25 examined metaphases had idic(X)(q13)). We compared our findings with the results published by Paulsson et al. (5), converting the genomic position from the human genome reference NCBI36/hg18 to the human reference sequence GRCh37/hg19. The region in which the breakpoints were found in the present study corresponds to the region referred to as “72.1–72.3 Mb” by Paulsson et al. (5). This region is rich in repetitive DNA sequences and contains two large segmental duplications harboring the genes FAM236B, two copies of FAM236D, and two copies of DMRTC1 (5). Little is known about these genes. However, DMRTC1 appears to encode a protein related to double-sex- and mab-3-related transcription factors (17, 18). It contains a region named “DMRT-like” but lacks the DNA binding domain of DMRT transcription factors (17, 18). The protein is predicted to be nuclear, part of the chromatin, and acts as a transcriptional regulator (19).
For case 6, carrying the abnormality i(X)(q10), aCGH analysis revealed, for the first time, that the breakpoints of the rearranged chromosome X occurred in sub-band Xp11.22, within the genomic region interval ChrX:52,725,935-52,838,474. Consequently, the aberrant chromosome X was not a true isochromosome but rather a cryptic idic(X)(p11). Notably, a similar finding was observed with i (17)(q10) found in many hematologic neoplasms. The apparent i (17)(q10) also had the breakpoints in the proximal part of the short arm, specifically at 17p11, indicating that the i (17)(q10) is actually a cryptic idic (17)(p11) (20).
The approximately 112 Kbp genomic region ChrX:52,725,935-52,838,474 is rich in repetitive DNA sequences, contains many segmental duplications, and harbors the genes SSX2, SSX2B, and SPANXN5. Breakpoints in this region have also been reported in patients with Turner syndrome carrying idic(X)(p11), and they have been linked to repetitive DNA sequences and segmental duplications. The repetitive sequence configurations at the breakpoint intervals suggest non-allelic homologous recombination as a principal mechanism for the formation of idic(X)(p11). Non-allelic homologous recombination may also be the mechanism for the formation of idic(Xq13) in myeloid neoplasms since the breakpoints occur in a region rich in repetitive DNA sequences containing two large segmental duplications (5, 21).
SSX2 gene belongs to a multigene family of cancer-testis antigens and can be found overexpressed in multiple malignancies, e.g., in colorectal carcinoma, prostate cancer, breast cancer, hepatocellular carcinoma, glioma, lymphoma, leukemia, gastric carcinoma, and thyroid carcinoma tissue samples (22). In addition, it has been shown to be elevated in approximately one-third of acute myeloid leukemia (AML) patient samples at presentation, and it has been suggested to be a relevant target antigen for chimeric antigen receptor (CAR) therapy (23). SSX2B, an important paralog of SSX2, may function as a transcription repressor and can, together with SSX2, potentially be useful targets in vaccine-based immunotherapy (23). Moreover, the SPANXN5 gene is expressed in normal testis and some melanoma cell lines, suggesting that it could also be a potential target for cancer immunotherapy (24).
In all patients, concurrent with cytogenetic aberrations, multiple pathogenic or likely pathogenic gene variants were detected using targeted sequencing (NGS-panel) (Table 2). The tumor suppressor gene TET2, located at chromosomal band 4q24, was the most commonly altered gene (four out of six patients). This finding is consistent with two previous studies, where pathogenic variants of TET2 were frequently observed in idic(X)-positive myeloid malignancies, suggesting their involvement as early oncogenic events (5, 13). Pathogenic or likely pathogenic variants of TET2 have been detected in a significant proportion of myeloid malignancies (5, 7, 13); they can occur in MDS (30%) and in AML (10%) (25, 26). Most of these variants lead to truncation of the TET2 protein, resulting in its reduced or lost function. The acquisition of additional pathogenic variants in other genes, such as SF3B1, ASXL1, or SRSF2, can influence the clinical phenotype, leading to modifications in clone morphology, type of lineage production defect, myeloproliferative features, or rates of progression to AML (27). In our study, the MDS cases, which harbored pathogenic or likely pathogenic variants of TET2, also carried pathogenic variants of additional genes, indicating a worse outcome. Song et al. (28) reported that double SF3B1/TET2 pathogenic variants were most common in patients with MDS. Patients with only TET2 variants were more likely to be diagnosed with AML than those with SF3B1 mutations. In conclusion, our data showed that the breakpoints in the idic(X)(q13) are found in a narrow region, 200kbp, of sub-band Xq13 and that the i(X)(q10) is formally an idic(X)(p11). The breakpoint regions were rich in repetitive DNA sequences containing large segmental duplications, indicating non-allelic homologous recombination as a principal mechanism for the formation of idic(X)(q13)/idic(X)(p11). All patients showed additional pathogenic variants in more than one gene. Pathogenic variants of TET2 gene were the most commonly identified, conferring a worse outcome for the patients.
Data availability statement
The original contributions presented in the study are included in the article/supplementary material. Further inquiries can be directed to the corresponding author.
Ethics statement
The studies involving humans were approved by The Regional Committee for Medical and Health Research Ethics (REK, project number 2010/1389; http://helseforskning.etikkom.no). The studies were conducted in accordance with the local legislation and institutional requirements. The participants provided their written informed consent to participate in this study. Written informed consent was obtained from the individual(s) for the publication of any potentially identifiable images or data included in this article.
Author contributions
MB: Conceptualization, Investigation, Methodology, Resources, Software, Validation, Visualization, Writing – original draft, Writing – review & editing. KA: Methodology, Writing – review & editing. GT: Methodology, Software, Writing – review & editing. FM: Data curation, Funding acquisition, Project administration, Writing – review & editing. SS: Data curation, Writing – review & editing. AL: Data curation, Writing – review & editing. MT: Data curation, Writing – review & editing. IP: Conceptualization, Investigation, Resources, Software, Supervision, Validation, Visualization, Writing – review & editing.
Funding
The author(s) declare that financial support was received for the research, authorship, and/or publication of this article. This research was funded by The Norwegian Radium Hospital Foundation (Radiumhospitalets legater).
Acknowledgments
The authors wish to thank the diagnostic group at the Section for Cancer Cytogenetics, Institute for Cancer Genetics and Informatics, Oslo University Hospital, for technical assistance.
Conflict of interest
The authors declare that the research was conducted in the absence of any commercial or financial relationships that could be construed as a potential conflict of interest.
Publisher’s note
All claims expressed in this article are solely those of the authors and do not necessarily represent those of their affiliated organizations, or those of the publisher, the editors and the reviewers. Any product that may be evaluated in this article, or claim that may be made by its manufacturer, is not guaranteed or endorsed by the publisher.
References
1. Mitelman Database of Chromosome Aberrations and Gene Fusions in Cancer (2024) Mitelman F, Johansson B, Mertens F. (Eds.). Available at: https://mitelmandatabase.isb-cgc.org.
2. Heim S, Mitelman F. Cancer cytogenetics: chromosomal and molecular genetic aberrations of tumor cells. (2015) 62–152. doi: 10.1002/9781118795569
3. Dierlamm J, Michaux L, Criel A, Wlodarska I, Zeller W, Louwagie A, et al. Isodicentric (X)(q13) in haematological Malignancies: presentation of five new cases, application of fluorescence in situ hybridization (FISH) and review of the literature. Br J haematology. (1995) 91:885–91. doi: 10.1111/j.1365-2141.1995.tb05405.x
4. Dewald GW, Pierre RV, Phyliky RL. Three patients with structurally abnormal X chromosomes, each with Xq13 breakpoints and a history of idiopathic acquired sideroblastic anemia. Blood. (1982) 59:100–5. doi: 10.1182/blood.V59.1.100.100
5. Paulsson K, Haferlach C, Fonatsch C, Hagemeijer A, Andersen MK, Slovak ML, et al. The idic(X)(q13) in myeloid Malignancies: breakpoint clustering in segmental duplications and association with TET2 mutations. Hum Mol Genet. (2010) 19:1507–14. doi: 10.1093/hmg/ddq024
6. Döhner H, Wei AH, Appelbaum FR, Craddock C, DiNardo CD, Dombret H, et al. Diagnosis and management of AML in adults: 2022 recommendations from an international expert panel on behalf of the ELN. Blood. (2022) 140:1345–77. doi: 10.1182/blood.2022016867
7. WHO Classification of Tumours Editorial Board. Haematolymphoid tumours. In: WHO classification of tumours series, 5th ed, vol. 11. International Agency for Research on Cancer, Lyon, France. (2024) Available at: https://tumourclassification.iarc.who.int/chapters/63.
8. Arber DA, Orazi A, Hasserjian RP, Borowitz MJ, Calvo KR, Kvasnicka H-M, et al. International Consensus Classification of Myeloid Neoplasms and Acute Leukemias: integrating morphologic, clinical, and genomic data. Blood. (2022) 140:1200–28. doi: 10.1182/blood.2022015850
9. Debiec-Rychter M, Kałuzewski B, Bugała I, Zajazkowska D. Isochromosome X in acute myeloblastic leukemia. Cancer Genet cytogenetics. (1985) 15:137–41. doi: 10.1016/0165-4608(85)90141-4
10. Larson DP, Akkari YM, Van Dyke DL, Raca G, Gardner JA, Rehder CW, et al. Conventional cytogenetic analysis of hematologic neoplasms: A 20-year review of proficiency test results from the college of american pathologists/american college of medical genetics and genomics cytogenetics committee. Arch Pathol Lab Med. (2021) 145:176–90. doi: 10.5858/arpa.2020-0089-CP
11. Rack KA, Chelly J, Gibbons RJ, Rider S, Benjamin D, Lafreniére RG, et al. Absence of the XIST gene from late-replicating isodicentric X chromosomes in leukaemia. Hum Mol Genet. (1994) 3:1053–9. doi: 10.1093/hmg/3.7.1053
12. McDonell N, Ramser J, Francis F, Vinet MC, Rider S, Sudbrak R, et al. Characterization of a highly complex region in Xq13 and mapping of three isodicentric breakpoints associated with preleukemia. Genomics. (2000) 64:221–9. doi: 10.1006/geno.2000.6128
13. Penther D, Etancelin P, Lusina D, Bidet A, Quilichini B, Gaillard B, et al. Isolated isochromosomes i(X)(p10) and idic(X)(q13) are associated with myeloid Malignancies and dysplastic features. Am J hematology. (2019) 94:E285–e8. doi: 10.1002/ajh.25601
14. Czepulkowski B, Bhatt B, Rooney D. Basic techniques for the preparation and analysis of. Hum cytogenetics: Malignancy acquired abnormalities. (1992) 2:1.
15. McGowan-Jordan J HR, Moore S. ISCN 2020: An International system for human cytogenomic nomenclature. (2020), 164. doi: 10.1159/isbn.978-3-318-06867-2
16. Busque L Vassiliou GS, Bejar R, et al. Clonal haematopoieses. In: WHO Classification of Tumours Editorial Board. Haematolymphoid tumours, 5th ed, vol. 11. International Agency for Research on Cancer, Lyon (France (2024). Available at: https://tumourclassification.iarc.who.int/chapters/63.
17. Hong CS, Park BY, Saint-Jeannet JP. The function of Dmrt genes in vertebrate development: it is not just about sex. Dev Biol. (2007) 310:1–9. doi: 10.1016/j.ydbio.2007.07.035
18. Zarkower D, Murphy MW. DMRT1: an ancient sexual regulator required for human gonadogenesis. Sexual development: genetics Mol biology evolution endocrinology embryology Pathol sex determination differentiation. (2022) 16:112–25. doi: 10.1159/000518272
19. National library of Medicine - National center for Biotechnology Information . Available online at: https://www.ncbi.nlm.nih.gov/gene/63947. (Accessed April 19, 2024)
20. Fioretos T, Strömbeck B, Sandberg T, Johansson B, Billström R, Borg A, et al. Isochromosome 17q in blast crisis of chronic myeloid leukemia and in other hematologic Malignancies is the result of clustered breakpoints in 17p11 and is not associated with coding TP53 mutations. Blood. (1999) 94:225–32. doi: 10.1182/blood.V94.1.225.413k24_225_232
21. Scott SA, Cohen N, Brandt T, Warburton PE, Edelmann L. Large inverted repeats within Xp11.2 are present at the breakpoints of isodicentric X chromosomes in Turner syndrome. Hum Mol Genet. (2010) 19:3383–93. doi: 10.1093/hmg/ddq250
22. Smith HA, McNeel DG. The SSX family of cancer-testis antigens as target proteins for tumor therapy. Clin Dev Immunol. (2010) 2010:150591. doi: 10.1155/2010/150591
23. Raskin S, Van Pelt S, Toner K, Balakrishnan PB, Dave H, Bollard CM, et al. Novel TCR-like CAR-T cells targeting an HLA∗0201-restricted SSX2 epitope display strong activity against acute myeloid leukemia. Mol Ther Methods Clin Dev. (2021) 23:296–306. doi: 10.1016/j.omtm.2021.09.008
24. Kouprina N, Mullokandov M, Rogozin IB, Collins NK, Solomon G, Otstot J, et al. The SPANX gene family of cancer/testis-specific antigens: rapid evolution and amplification in African great apes and hominids. Proc Natl Acad Sci United States America. (2004) 101:3077–82. doi: 10.1073/pnas.0308532100
25. Delhommeau F, Dupont S, Della Valle V, James C, Trannoy S, Massé A, et al. Mutation in TET2 in myeloid cancers. N Engl J Med. (2009) 360:2289–301. doi: 10.1056/NEJMoa0810069
26. Tyner JW, Tognon CE, Bottomly D, Wilmot B, Kurtz SE, Savage SL, et al. Functional genomic landscape of acute myeloid leukaemia. Nature. (2018) 562:526–31. doi: 10.1038/s41586-018-0623-z
27. Hirsch CM, Nazha A, Kneen K, Abazeed ME, Meggendorfer M, Przychodzen BP, et al. Consequences of mutant TET2 on clonality and subclonal hierarchy. Leukemia. (2018) 32:1751–61. doi: 10.1038/s41375-018-0150-9
Keywords: acute myeloid leukemia, myelodysplastic syndrome, cytogenetics, idic(X)(q13), i(X)(q10), array comparative genomic hybridization, pathogenic variants
Citation: Brunetti M, Andersen K, Trøen G, Micci F, Spetalen S, Lenartova A, Tandsæther MR and Panagopoulos I (2024) Molecular genetic characterization of myeloid neoplasms with idic(X)(q13) and i(X)(q10). Front. Oncol. 14:1428984. doi: 10.3389/fonc.2024.1428984
Received: 07 May 2024; Accepted: 09 September 2024;
Published: 26 September 2024.
Edited by:
Francesco Bertolini, European Institute of Oncology (IEO), ItalyReviewed by:
Dhanlaxmi Shetty, Research and Education in Cancer (ACTREC), IndiaArianna Valeriano, European Institute of Oncology (IEO), Italy
Yi Ning, University of Maryland, United States
Copyright © 2024 Brunetti, Andersen, Trøen, Micci, Spetalen, Lenartova, Tandsæther and Panagopoulos. This is an open-access article distributed under the terms of the Creative Commons Attribution License (CC BY). The use, distribution or reproduction in other forums is permitted, provided the original author(s) and the copyright owner(s) are credited and that the original publication in this journal is cited, in accordance with accepted academic practice. No use, distribution or reproduction is permitted which does not comply with these terms.
*Correspondence: Marta Brunetti, YnJ1bmV0dGkubWFydGE5MEBnbWFpbC5jb20=