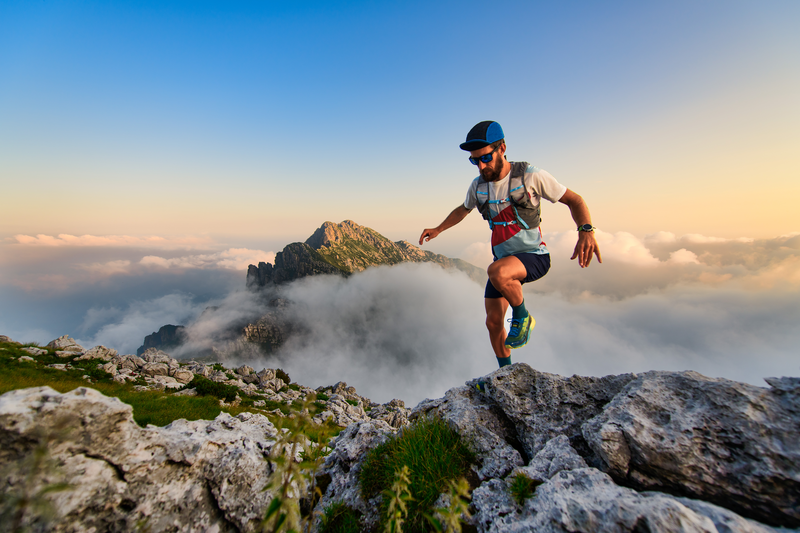
94% of researchers rate our articles as excellent or good
Learn more about the work of our research integrity team to safeguard the quality of each article we publish.
Find out more
REVIEW article
Front. Oncol. , 07 August 2024
Sec. Cancer Molecular Targets and Therapeutics
Volume 14 - 2024 | https://doi.org/10.3389/fonc.2024.1421902
This article is part of the Research Topic Unraveling the Role of Receptor Signaling in Tumorigenesis and Cancer Therapy View all 4 articles
Integrin β4 (ITGB4) is a transmembrane protein that functions as a mechanosensor, mediating the bidirectional exchange of information between the intracellular and extracellular matrices. ITGB4 plays a critical role in cell adhesion, migration, and signaling. Numerous studies have implicated ITGB4 as a key facilitator of tumor migration and invasion. This review provides a foundational description of the mechanisms by which ITGB4 regulates tumor migration and invasion through pathways involving focal adhesion kinase (FAK), protein kinase B (AKT), and matrix metalloproteinases (MMPs). These mechanisms encompass epithelial-mesenchymal transition (EMT), phosphorylation, and methylation of associated molecules. Additionally, this review explores the role of ITGB4 in the migration and invasion of prevalent clinical tumors, including those of the digestive system, breast, and prostate.
Cancer poses a significant social, public health, and economic burden. Data from the International Agency for Research on Cancer (IARC) indicate that roughly one in five individuals, both men and women, will develop cancer during their lifetime. Furthermore, approximately one in nine men and one in two women succumb to the disease (1). Metastasis, the spread of cancer cells from the primary tumor to distant organs, is the leading cause of death from cancer. Unlike primary tumors, metastasis represents a systemic disease affecting the entire body (2). Therefore, elucidating the mechanisms underlying tumor metastasis and identifying non-specific targets within the metastatic cascade are crucial for advancing cancer therapy.
Integrins are heterodimeric transmembrane receptors composed of non-covalently associated α and β subunits. The human genome encodes 18 α and 8 β subunits, which can combine to form a repertoire of 24 distinct integrin receptors. Each integrin receptor possesses unique binding specificities and exhibits a distinct tissue distribution (3). These receptors span the plasma membrane and serve as platforms for the assembly of signaling complexes that physically connect the extracellular matrix to the intracellular cytoskeleton. Notably, integrins exhibit a remarkable capacity for bidirectional signaling across the plasma membrane, mediating both “inside-out” (4) and “outside-in” signaling (5). This bidirectional communication enables human cells to not only respond to changes in their surrounding extracellular environment but also actively influence it (6). It is now understood that integrins primarily mediate cell-matrix interactions and play crucial roles in various cellular processes, including adhesion, proliferation, differentiation, migration, and invasion (7).
Integrin β4 (ITGB4, also known as CD104), a member of the tryptophan-aspartic acid (WD)-40 repeat family, is a tumor-associated antigen (TAA) exhibiting high expression levels in diverse malignant tumors. ITGB4 promotes tumor progression by amplifying distinct signaling pathways (8) and facilitating tumor cell migration and invasion (9, 10). Transcriptome analysis from The Cancer Genome Atlas (TCGA) pinpointed ITGB4 as a target gene in colorectal cancer, suggesting a critical role in disease development (11). Likewise, in squamous cell carcinoma of the lung, elevated ITGB4 expression is associated with tumorigenesis and progression via modulation of various signaling pathways (12). ITGB4 is widely recognized as a molecule with both prognostic and predictive value in cancer patients (13). Notably, ITGB4 exhibits high expression levels in oral squamous cell carcinoma, gliomas, and pancreatic cancer (14–17). In colon cancer patients, high ITGB4 expression correlates with poor overall survival. Additionally, ITGB4 serves as a pivotal gene for constructing a predictive risk model for the clinical prognosis of lung adenocarcinoma (18–20). Furthermore, ITGB4 possesses diagnostic value for lung adenocarcinoma, demonstrating a significant correlation with overall survival (21).
ITGB4 has been implicated in promoting the invasive potential of various squamous cell carcinomas, breast cancers, and gastric cancers by activating MAPK and NF-κB signaling pathways (22–25). Mechanistically, ITGB4 engagement with extracellular ligands, such as human leukocyte antigen-1 (HLA-1), triggers the phosphorylation of Src kinase. This phosphorylation event subsequently leads to the activation of focal adhesion kinase (FAK) and downstream PI3K/AKT and Erk signaling pathways, ultimately promoting tumor cell migration (26). Furthermore, epidermal growth factor receptor (EGFR)/Src signaling can mediate the tyrosine phosphorylation of ITGB4, facilitating the recruitment of FAK to ITGB4. FAK activation then stimulates the Akt signaling pathway, thereby promoting tumor cell invasion (27–29). Collectively, these findings highlight the strong correlation between ITGB4 expression and tumor cell migration and invasion.
In contrast to other integrin β subunits, ITGB4 exclusively partners with the α6 subunit to form the α6β4 integrin, a cell adhesion molecule. Notably, within various cancers, α6β4 integrin undergoes release from hemidesmosomes. In this unbound state, α6β4 cooperates with growth factor receptors such as EGFR, ErbB-2, and c-Met. This cooperation leads to the amplification of downstream signaling pathways, including PI3K, AKT, MAPK, and Rho family GTPases. These activated pathways ultimately contribute to tumor migration and invasion (30). This study focused on the interplay between ITGB4 and other messenger RNAs (mRNAs) and molecules during its expression. We aimed to elucidate how ITGB4 interacts with these factors to influence tumor migration and invasion. By deciphering these mechanisms, we sought to provide valuable insights for further research exploring the impact of ITGB4 on cancer metastasis.
Focal adhesion kinase is a central cytoplasmic protein tyrosine kinase. Functioning as a signaling hub, FAK integrates signals from various pathways and plays a critical role in promoting tumor cell invasion and migration through both kinase-dependent and -independent mechanisms. ITGB4 acts as an upstream regulator of FAK, influencing its expression and phosphorylation. For example, in ovarian cancer cells, Spectrin beta non-erythrocytic 2 (SPTBN2) may promote tumor migration and invasion by inhibiting the expression of focal adhesion-related proteins and downstream signaling pathways via ITGB4. Notably, overexpression of ITGB4 reversed the decrease in phosphorylated FAK (p-FAK) induced by SPTBN2 knockdown, while the combination of SPTBN2 knockdown and ITGB4 overexpression had no effect on total FAK protein expression (31). Furthermore, ITGB4 appears to influence FAK phosphorylation in various cancers. Spindle pole component 25 homologue (SPC25) preferentially affects genes associated with ECM-integrin interactions. Upregulation of ITGB4 partially reverses the decrease in hepatocellular carcinoma cell invasion and migration caused by SPC25 silencing. Interestingly, both SPC25 and ITGB4 knockdown result in reduced phosphorylation of FAK, Phosphoinositide 3-kinase (PI3K), and AKT, all of which play crucial roles in these processes. In lung cancer, KCNF1, a regulator of epithelial-mesenchymal transition (EMT) and ECM-integrin interactions, positively regulates signaling downstream of ITGB4. Notably, the expression level of ITGB4 affects the phosphorylation of both FAK and AKT (32, 33).
The ITGB4/FAK signaling pathway is critical in regulating a diverse array of downstream molecules and signaling cascades associated with cancer cell metastasis. FAK activation, characterized by phosphorylation, is triggered upon cell adhesion to the extracellular matrix. This activation, in turn, initiates downstream signaling pathways intricately linked to cancer cell metastasis. Notably, the ITGB4/FAK pathway not only influences AKT phosphorylation but also modulates the activity of the FAK/AKT and PI3K/AKT signaling pathways. AKT, a central node within the PI3K/AKT pathway, exerts a profound influence on tumor cell proliferation, metastasis, invasion, and ultimately, patient prognosis (34).
ITGB4 functions as an upstream regulator of FAK, exerting a significant impact on AKT phosphorylation in non-small cell lung cancer (NSCLC) and hepatocellular carcinoma (32, 33). Studies further suggest that ITGB4 can activate the Akt signaling pathway by triggering FAK. These downstream effects of ITGB4 appear to be mediated by factors such as ZNF306 (35).
The interplay between ITGB4 and FAK can culminate in the inactivation of Akt and p38MAPK signaling pathways. Ziyuglycoside II, the primary compound isolated from Sanguisorba officinalis L., has been shown to inhibit the migration and invasion of triple-negative breast cancer (TNBC) cells. This inhibitory effect was mediated through Src/EGFR-dependent inactivation of the ITGB4/FAK signaling pathway, consequently leading to the inactivation of AKT and p38MAPK signaling pathways (36). EGFR has been found to interact with ITGB4 and influence anoikis through the ITGB4/FAK axis. By modulating the AKT pathway, this interaction plays a role in regulating cancer metastasis (28, 37, 38). Moreover, binding of PD-L1 to ITGB4 activates the AKT/GSK3β signaling pathway, thereby impacting the expression of the transcription inhibitor SNAIL and ultimately suppressing anti-tumor immunity (39).
EMT is a well-characterized cellular process during which epithelial cells undergo a phenotypic transformation, losing their epithelial characteristics and acquiring mesenchymal cell properties. This transition alters the adhesion molecules expressed on the cell surface, enabling cells to adopt migratory and invasive behaviors. EMT is demonstrably linked to tumor cell migration. A hallmark of EMT is the downregulation of the epithelial marker E-cadherin and the concomitant upregulation of the mesenchymal marker vimentin (40, 41). Notably, overexpression of ITGB4 has been shown to induce EMT by upregulating the transcription factor Slug. This upregulation leads to a loss of E-cadherin expression and the acquisition of a mesenchymal phenotype characterized by enhanced migratory and invasive capabilities (42). Conversely, the knockdown of ITGB4 in clear cell renal cell carcinoma (ccRCC) cells results in decreased expression of N-cadherin, vimentin, and the EMT-related transcription factor ZEB1 while simultaneously increasing E-cadherin expression (43).
Reciprocally, AKT can also function as an upstream regulator of ITGB4 expression, as evidenced by studies on NSCLC cells harboring pp53-R273H mutations. In these cells, AKT activation leads to a downregulation of ITGB4, thereby impacting their migratory and invasive phenotypes (44). This intricate interplay between ITGB4 and AKT highlights their critical roles in tumor cell migration and invasion. This interplay is further influenced by epidermal growth factor (EGF), various transcription factors, and drug monomers.
Matrix metalloproteinases (MMPs) are a family of proteases that play a critical role in shaping the extracellular matrix (ECM). Their expression is tightly regulated at the transcriptional level, and they are implicated in various cellular processes, including cell migration, invasion, angiogenesis, apoptosis, and inflammation (45). MMPs play a pivotal role in tumor progression, particularly in tumor migration and invasion, by facilitating the breakdown of histological barriers that normally restrict tumor cell movement. Studies have shown that nerve growth factor (NGF) promotes corneal epithelial migration by inducing the expression of MMP-9 (46). Furthermore, elevated expression of both MMP15 and ITGB4 has been observed in colorectal cancer patients following radical surgery. This finding suggests that ITGB4 may be involved in tumor progression by regulating MMPs (47). Supporting this notion, studies in ovarian cancer cells have demonstrated a positive correlation between the expression levels of ITGB4 and MMP2, MMP7, and MMP9 (31).
The mechanisms by which ITGB4 interacts with FAK, AKT and MMP to promote tumor migration and invasion are described in Table 1
The influence of ITGB4 on tumor migration and invasion extends beyond canonical signaling pathways. For instance, ITGB4 can interact with enzymes like 12-lipoxygenase (12-LOX), impacting tumor metastasis. Stimulation of ITGB4 triggers the recruitment of 12-LOX to the cell membrane, leading to its activation and subsequent production of 12(S)-HETE. This metabolite, in turn, regulates both angiogenesis and cell migration (49).
The multifaceted role of ITGB4 in tumor migration and invasion is further highlighted by its diverse effects across different cancer types. In esophageal cancer, the splice variant ITGB4E exhibits an inhibitory effect on esophageal squamous cell migration, while other variants appear to promote cell migration (50). Similarly, in gastric cancer, MPS-1 plays a regulatory role in invasion and migration by influencing ITGB4 expression (51). In colorectal cancer (CRC), Transcobalamin 1 (TCN1) disrupts the cytoskeletal network through modulation of ITGB4 signaling. Notably, TCN1 knockdown exhibits a synergistic effect with ITGB4-mediated inactivation of Ki-67 and PCNA, further promoting CRC progression (52). Another study identified the transcriptional inhibitor CBX8 as a repressor of the ITGB4 promoter. Knockdown of CBX8 leads to derepression and increased ITGB4 protein expression. This, in turn, reduces active RhoA, leading to actin rearrangements and enhanced CRC metastasis (53). Additionally, the microRNA miR-21 has been shown to regulate the colorectal cancer invasion-metastasis cascade by targeting ITGB4 (54). The influence of ITGB4 extends to hepatocellular carcinoma as well. In this context, ITGB4 overexpression downregulates the epithelial markers E-cadherin and N-cadherin, while simultaneously upregulating vimentin, p-AKT, Slug, Sox2, and Nanog in Bel-7402 or SMMC-77721 cells. These changes collectively induce epithelial-to-mesenchymal transition (EMT), promoting hepatocellular carcinoma invasion (48). Furthermore, ITGB4 functions as a laminin receptor, regulating bile duct cancer cell migration (55). In pancreatic ductal adenocarcinoma, ITGB4 promotes vimentin expression, induces EMT, and regulates migration and invasion (56).
Netrin-1, a guidance cue molecule, exhibits anti-tumorigenic properties in pancreatic ductal adenocarcinoma. Overexpression of netrin-1 has been shown to impede the growth of pancreatic ductal adenocarcinoma cells by downregulating ITGB4 expression. Mechanistically, netrin-1 signaling through the UNC5B/FAK axis stimulates nitric oxide production. This, in turn, promotes the PP2A-mediated inhibition of the MEK/ERK pathway, ultimately leading to a reduction in the recruitment of phosphorylated c-Jun to ITGB4 promoters (57). The MEK/ERK pathway is a well-established signaling cascade implicated in various cellular processes, including gene expression, cell proliferation, and behaviors that influence tumor progression (58, 59). Activation of MEK rapidly triggers the phosphorylation of downstream ERK 1/2. These phosphorylated ERKs then activate transcription factors, kinases, and other signaling molecules, ultimately influencing tumor migration and invasion. Interestingly, studies have shown that phosphorylated ITGB4 at the Y1510 site can regulate the MEK1-ERK1/2 signaling cascade. Therefore, modulation of ITGB4 expression or its phosphorylation at Y1510 represents a potential novel therapeutic approach for pancreatic cancer (60). Furthermore, the MEK/ERK pathway can also regulate integrin α6β4 expression. KRAS mutations, frequently observed in pancreatic cancer, have been shown to regulate the expression of integrin α6β4 through the MEK/ERK pathway, thereby altering the migratory and invasive potential of tumor cells (61). Recepteur d’origine nantais (RON) receptor tyrosine kinases have been implicated in promoting the aggressive behavior of pancreatic cancer cells. These kinases disrupt the interaction between plectin and ITGB4, thereby stimulating cell migration. This disruption of the plectin-ITGB4 interaction is dependent on PI3K activity and RON signaling (62).
In summary, the multifaceted influence of ITGB4 on a diverse array of downstream signaling molecules underscores its critical role in promoting tumor migration and invasion across a spectrum of cancers.
The role of ITGB4 in digestive system tumor migration and invasion are described in Table 2.
Caveolin-1 (P132L), a frequently occurring mutation in breast cancer, has been shown to selectively enhance the expression of ITGB4. ITGB4 is a well-characterized signaling molecule linked to tumor cell migration and invasion (63). Notably, overexpression of ITGB4 in triple-negative breast cancer cells has been implicated in the transfer of ITGB4 protein to cancer-associated fibroblasts (CAFs) via exosomes. This transfer, in turn, induces BNIP3L-dependent mitochondrial dysfunction and lactate production in CAFs. Co-culture assays revealed that the level of ITGB4 expression directly correlated with enhanced breast cancer cell proliferation, EMT, and invasion. Conversely, the knockdown of ITGB4 or inhibition of exosome production in MDA-MB-231 cells, or blockade of c-Jun or AMPK phosphorylation in CAFs, significantly suppressed ITGB4-mediated mitochondrial autophagy and glycolysis in CAFs (9). Similar to ITGB4, Rac1 functions as a key regulator of the cytoskeleton. Both proteins represent promising therapeutic targets for disrupting the ability of cancer cells to reattach and establish themselves in new locations (reconnection ability or RA). Intriguingly, sustained Rac1 activity can prevent the lysosomal degradation of β4 integrins, highlighting a potential mechanism for its role in cancer progression (64).
SPARC acts as a downstream effector molecule that amplifies ITGB4-mediated invasion in breast cancer. However, the miR-29a microRNA directly targets SPARC, inhibiting this invasive process. Interestingly, breast cancer cells with low ITGB4 expression exhibit a concomitant decrease in miR-29a levels (65). TMEM268 is a transmembrane protein that interacts directly with the β4 subunit of integrin. Knockdown of TMEM268 promotes the ubiquitin-mediated degradation of ITGB4, ultimately leading to cytoskeletal remodeling (36).
The role of ITGB4 in breast cancer migration and invasion are described in Table 3.
ITGB4 has emerged as a critical driver of metastatic tumor cell migration and invasion in prostate cancer, as evidenced by studies using DU145 cells (68). ZEB1, a well-characterized regulator of EMT, interacts with the promoters of laminin α2 (LAMC2) and ITGB4, thereby influencing their expression levels (69). Interestingly, ITGB4 promoter methylation levels exhibit variation across prostate cancer cell lines representing distinct disease stages, including local tumors, lymph node metastases, and bone metastases (70). Estrogen receptor-regulated microRNA-182-5p (miR-182-5p) has been shown to promote proliferation, invasion, migration, and inhibit apoptosis in prostate cancer cells. This oncogenic effect is mediated through ARRDC3/ITGB4 signaling (71). Parathyroid hormone-related peptide (PTHrP) also contributes to prostate cancer growth and metastasis by regulating ITGB4 levels through transcriptional and post-translational pathways, including association with the NF-κB signaling pathway (72).
Proteomic analysis of exosomes isolated from body fluids has revealed upregulation of ITGB4. This finding suggests that ITGB4 may serve as a potential biomarker for prostate cancer progression and taxane resistance. Furthermore, the knockout of ITGB4 in PC-3R cells significantly weakened their migratory and invasive capabilities, further highlighting the crucial role of ITGB4 in these processes (73).
The role of ITGB4 in prostate cancer migration and invasion are described in Table 4.
Quantitative real-time PCR analysis has identified a high ITGB4/JUP ratio as a significant factor promoting distant metastasis in oral cancer (74). This finding suggests that the relative expression level of ITGB4 compared to JUP may be a valuable prognostic indicator. Vimentin, an intermediate filament protein, has been shown to play a novel role in regulating cell motility by destabilizing adhesions mediated by β4 integrin. Interestingly, the knockdown of vimentin in oral cancer cells enhances the mechanical binding function of ITGB4 (75). This finding suggests a potential therapeutic strategy targeting the interaction between vimentin and ITGB4 to regulate cell motility and metastasis. Micropeptides that inhibit the actin cytoskeleton (MIACs) have been demonstrated to regulate interactions between the cytoskeletal protein SEPT2 and ITGB4. By suppressing the actin cytoskeleton, MIACs ultimately inhibit tumor growth and metastasis in head and neck squamous cell carcinoma (76).
In nasopharyngeal carcinoma, latent membrane protein 2A (LMP2A) competes with tyrosine kinase (ySyk) to bind ITGB4, promoting cell migration and invasion (77). Conversely, inhibition of LMP2A expression or miR-182-5p reduction leads to decreased migration and invasion, highlighting the potential regulatory role of these factors. Moreover, a meta-analysis of ccRCC identified ITGB4 as a target gene regulated by microRNA-204 (miR-204), further emphasizing the importance of ITGB4 in the context of cancer progression (78).
ITGB4 emerges as a key player with diverse regulatory mechanisms across different cancers. In brain cancer, mutations in the isocitrate dehydrogenase 1 (IDH1) gene, particularly IDH1R132H/WT, promote cell migration and inhibit proliferation by upregulating ITGB4, suggesting altered molecular targets and pathways (79). Ovarian cancer highlights another regulatory mechanism, where mutant p53 promotes peritoneal metastasis by upregulating ITGB4 and activating the AKT pathway to enhance adhesion between cancer cells and mesothelial cells (42). Hypochlorous acid (HOCl) affects the oxidative modification of glucose-regulated protein 78 (GRP78) and GRP78 ATPase activity to regulate autophagy. ZBM-H acts as a probe for HOCl and can bind directly to GRP78 in the presence or absence of ATP. The interaction between GRP78 and annexin A7 (ANXA7) was facilitated after the use of ZBM-H, which was accompanied by an increased phosphorylation of ITGB4, which in turn regulated lung cancer cell behavior (80). In NSCLC, ZBM-H activates GRP78 ATPase, reduces ITGB4 protein levels, inhibits A549 cell migration, and suppresses EMT processes (81).
The role of ITGB4 in other tumors migration and invasion are described in Table 5
ITGB4 exhibits high expression levels in numerous tumors, making it a potential tumor marker. Furthermore, methylation and genetic alterations within the ITGB4 gene hold significant promise for tumor diagnosis and treatment development (19, 82). Notably, the function of ITGB4 varies across different tumor types. In hepatocellular carcinoma and prostate cancer, ITGB4 expression is associated with tumor-associated fibroblasts. Conversely, in breast and lung cancers, ITGB4 correlates with the level of immune cell infiltration, suggesting potential roles in NA metabolism and protein processing within its functional mechanism (83). In gliomas, ITGB4 demonstrates high expression and serves as a reliable prognostic indicator for low-grade tumors, as confirmed by both bioinformatic analysis and tissue sample comparison (84). Integrins play a critical role in regulating various hallmarks of cancer, including tumor metastasis, immune evasion, and metabolic reprogramming. This has led to the exploration of integrin-targeted immunotherapy and other integrin inhibitors in preclinical and clinical studies (85). Interestingly, recent research suggests that ITGB4 may serve as a targeted immune site for CSCs (86). Furthermore, studies have revealed a novel signaling pathway in TNBC. TNFAIP2 promotes the proliferation and migration of TNBC cells by activating RAC1. ITGB4, through TNFAIP2 and IQGAP1, further activates RAC1, conferring resistance to DNA damage-induced cell death in TNBC. This ITGB4/TNFAIP2/IQGAP1/RAC1 signaling axis presents a potential therapeutic target to overcome DNA damage resistance in TNBC (87).
While research has confirmed the differential expression of upstream and downstream molecular pathways associated with ITGB4, a significant knowledge gap remains regarding its role in tumor biology, particularly its function as a cell adhesion molecule. Further studies are warranted to elucidate the precise molecular mechanisms by which ITGB4, a critical molecule in tumor progression and a potential target for therapeutic intervention, contributes to tumor development and metastasis.
GH: Conceptualization, Writing – original draft, Writing – review & editing. MZ: Conceptualization, Writing – original draft, Writing – review & editing. DL: Conceptualization, Writing – original draft, Writing – review & editing. JL: Data curation, Formal analysis, Writing – original draft. QT: Data curation, Formal analysis, Writing – original draft. CX: Data curation, Formal analysis, Writing – original draft. FL: Supervision, Writing – original draft, Writing – review & editing. RC: Supervision, Writing – original draft, Writing – review & editing.
The author(s) declare financial support was received for the research, authorship, and/or publication of this article. This work was supported by Natural Science Foundation of China, Grant No. 82374585.
We would like to thank everyone who contributed to this article. We also would like to acknowledge the reviewers for their helpful comments and insights in this paper.
The authors declare that the research was conducted in the absence of any commercial or financial relationships that could be construed as a potential conflict of interest.
All claims expressed in this article are solely those of the authors and do not necessarily represent those of their affiliated organizations, or those of the publisher, the editors and the reviewers. Any product that may be evaluated in this article, or claim that may be made by its manufacturer, is not guaranteed or endorsed by the publisher.
1. Bray F, Laversanne M, Sung H, Ferlay J, Siegel RL, Soerjomataram I, et al. Global cancer statistics 2022: GLOBOCAN estimates of incidence and mortality worldwide for 36 cancers in 185 countries. CA Cancer J Clin. (2024) 74:229–63. doi: 10.3322/caac.21834
2. Ganesh K, Massagué J. Targeting metastatic cancer. Nat Med. (2021) 27:34–44. doi: 10.1038/s41591-020-01195-4
3. Hynes RO. Integrins: bidirectional, allosteric signaling machines. Cell. (2002) 110:673–87. doi: 10.1016/S0092-8674(02)00971-6
4. Faull RJ, Ginsberg MH. Inside-out signaling through integrins. J Am Soc Nephrol. (1996) 7:1091–7. doi: 10.1681/ASN.V781091
5. Zhu J, Carman CV, Kim M, Shimaoka M, Springer TA, Luo BH. Requirement of alpha and beta subunit transmembrane helix separation for integrin outside-in signaling. Blood. (2007) 110:2475–83. doi: 10.1182/blood-2007-03-080077
6. Slack RJ, Macdonald SJF, Roper JA, Jenkins RG, Hatley RJD. Emerging therapeutic opportunities for integrin inhibitors. Nat Rev Drug Discovery. (2022) 21:60–78. doi: 10.1038/s41573-021-00284-4
7. Anderson LR, Owens TW, Naylor MJ. Structural and mechanical functions of integrins. Biophys Rev. (2014) 6:203–13. doi: 10.1007/s12551-013-0124-0
8. Ali SR, Jordan M, Nagarajan P, Amit M. Nerve density and neuronal biomarkers in cancer. Cancers (Basel). (2022) 14. doi: 10.3390/cancers14194817
9. Sung JS, Kang CW, Kang S, Jang Y, Chae YC, Kim BG, et al. ITGB4-mediated metabolic reprogramming of cancer-associated fibroblasts. Oncogene. (2020) 39:664–76. doi: 10.1038/s41388-019-1014-0
10. Qi L, Knifley T, Chen M, O'Connor KL. Integrin α6β4 requires plectin and vimentin for adhesion complex distribution and invasive growth. J Cell Sci. (2022) 135. doi: 10.1242/jcs.258471
11. Chen B, Ma Y, Bi J, Wang W, He A, Su G, et al. Regulation network of colorectal-cancer-specific enhancers in the progression of colorectal cancer. Int J Mol Sci. (2021) 22. doi: 10.3390/ijms22158337
12. Zhong F, Lu HP, Chen G, Dang YW, Li GS, Chen XY, et al. The clinical significance and potential molecular mechanism of integrin subunit beta 4 in laryngeal squamous cell carcinoma. Pathol Res Pract. (2020) 216:152785. doi: 10.1016/j.prp.2019.152785
13. Zhang M, Liu Y, Kong D. Erratum to "Identifying biomolecules and constructing a prognostic risk prediction model for recurrence in osteosarcoma. J Bone Oncol. (2021) 29:100371. doi: 10.1016/j.jbo.2021.100371
14. Chen X, Yuan Q, Liu J, Xia S, Shi X, Su Y, et al. Comprehensive characterization of extracellular matrix-related genes in PAAD identified a novel prognostic panel related to clinical outcomes and immune microenvironment: A silico analysis with in vivo and vitro validation. Front Immunol. (2022) 13:985911. doi: 10.3389/fimmu.2022.985911
15. Ma SR, Liu JF, Jia R, Deng WW, Jia J. Identification of a favorable prognostic subgroup in oral squamous cell carcinoma: Characterization of ITGB4/PD-L1(high) with CD8/PD-1(high). Biomolecules. (2023) 13. doi: 10.3390/biom13061014
16. Ma B, Zhang L, Zou Y, He R, Wu Q, Han C, et al. Reciprocal regulation of integrin β4 and KLF4 promotes gliomagenesis through maintaining cancer stem cell traits. J Exp Clin Cancer Res. (2019) 38:23. doi: 10.1186/s13046-019-1034-1
17. Lévy P, Vidaud D, Leroy K, Laurendeau I, Wechsler J, Bolasco G, et al. Molecular profiling of Malignant peripheral nerve sheath tumors associated with neurofibromatosis type 1, based on large-scale real-time RT-PCR. Mol Cancer. (2004) 3:20. doi: 10.1186/1476-4598-3-20
18. Guo W, Zhao G, Liu S, Deng T, Zhang G, Zhang B. Development of the prognostic value in lung adenocarcinoma based on anoikis-related genes and initial experimental validation. J Gene Med. (2023) 25:e3534. doi: 10.1002/jgm.3534
19. Huang W, Fan L, Tang Y, Chi Y, Li J. A pan-cancer analysis of the oncogenic role of integrin beta4 (ITGB4) in human tumors. Int J Gen Med. (2021) 14:9629–45. doi: 10.2147/IJGM.S341076
20. Li M, Jiang X, Wang G, Zhai C, Liu Y, Li H, et al. ITGB4 is a novel prognostic factor in colon cancer. J Cancer. (2019) 10:5223–33. doi: 10.7150/jca.29269
21. Guo Q, Liu XL, Liu HS, Luo XY, Yuan Y, Ji YM, et al. The risk model based on the three oxidative stress-related genes evaluates the prognosis of LAC patients. Oxid Med Cell Longev. (2022) 2022:4022896. doi: 10.1155/2022/4022896
22. Shaw LM, Rabinovitz I, Wang HH, Toker A, Mercurio AM. Activation of phosphoinositide 3-OH kinase by the alpha6beta4 integrin promotes carcinoma invasion. Cell. (1997) 91:949–60. doi: 10.1016/S0092-8674(00)80486-9
23. Lipscomb EA, Mercurio AM. Mobilization and activation of a signaling competent alpha6beta4integrin underlies its contribution to carcinoma progression. Cancer Metastasis Rev. (2005) 24:413–23. doi: 10.1007/s10555-005-5133-4
24. Nikolopoulos SN, Blaikie P, Yoshioka T, Guo W, Puri C, Tacchetti C, et al. Targeted deletion of the integrin beta4 signaling domain suppresses laminin-5-dependent nuclear entry of mitogen-activated protein kinases and NF-kappaB, causing defects in epidermal growth and migration. Mol Cell Biol. (2005) 25:6090–102. doi: 10.1128/MCB.25.14.6090-6102.2005
25. Yoon SO, Shin S, Lipscomb EA. A novel mechanism for integrin-mediated ras activation in breast carcinoma cells: the alpha6beta4 integrin regulates ErbB2 translation and transactivates epidermal growth factor receptor/ErbB2 signaling. Cancer Res. (2006) 66:2732–9. doi: 10.1158/0008-5472.CAN-05-2941
26. Zhang X, Rozengurt E, Reed EF. HLA class I molecules partner with integrin β4 to stimulate endothelial cell proliferation and migration. Sci Signal. (2010) 3:ra85. doi: 10.1126/scisignal.2001158
27. Margadant C, Frijns E, Wilhelmsen K, Sonnenberg A. Regulation of hemidesmosome disassembly by growth factor receptors. Curr Opin Cell Biol. (2008) 20:589–96. doi: 10.1016/j.ceb.2008.05.001
28. Leng C, Zhang ZG, Chen WX, Luo HP, Song J, Dong W, et al. An integrin beta4-EGFR unit promotes hepatocellular carcinoma lung metastases by enhancing anchorage independence through activation of FAK-AKT pathway. Cancer Lett. (2016) 376:188–96. doi: 10.1016/j.canlet.2016.03.023
29. Tai YL, Chu PY, Lai IR, Wang MY, Tseng HY, Guan JL, et al. An EGFR/Src-dependent β4 integrin/FAK complex contributes to Malignancy of breast cancer. Sci Rep. (2015) 5:16408. doi: 10.1038/srep16408
30. Stewart RL, O'Connor KL. Clinical significance of the integrin α6β4 in human Malignancies. Lab Invest. (2015) 95:976–86. doi: 10.1038/labinvest.2015.82
31. Yang L, Gu Y. SPTBN2 regulates endometroid ovarian cancer cell proliferation, invasion and migration via ITGB4−mediated focal adhesion and ECM receptor signalling pathway. Exp Ther Med. (2023) 25:277. doi: 10.3892/etm
32. Shi WK, Shang QL, Zhao YF. SPC25 promotes hepatocellular carcinoma metastasis via activating the FAK/PI3K/AKT signaling pathway through ITGB4. Oncol Rep. (2022) 47. doi: 10.3892/or
33. Chen CY, Wu PY, Van Scoyk M, Simko SA, Chou CF, Winn RA. KCNF1 promotes lung cancer by modulating ITGB4 expression. Cancer Gene Ther. (2023) 30:414–23. doi: 10.1038/s41417-022-00560-4
34. Song M, Bode AM, Dong Z, Lee MH. AKT as a therapeutic target for cancer. Cancer Res. (2019) 79:1019–31. doi: 10.1158/0008-5472.CAN-18-2738
35. Li J, Hao N, Han J, Zhang M, Li X, Yang N. ZKSCAN3 drives tumor metastasis via integrin β4/FAK/AKT mediated epithelial-mesenchymal transition in hepatocellular carcinoma. Cancer Cell Int. (2020) 20:216. doi: 10.1186/s12935-020-01307-7
36. Wang K, Zou P, Zhu X, Zhang T. Ziyuglycoside II suppresses the aggressive phenotype of triple negative breast cancer cells through regulating Src/EGFR-dependent ITGB4/FAK signaling. Toxicol In Vitro. (2019) 61:104653. doi: 10.1016/j.tiv.2019.104653
37. Schinke H, Shi E, Lin Z, Quadt T, Kranz G, Zhou J, et al. A transcriptomic map of EGFR-induced epithelial-to-mesenchymal transition identifies prognostic and therapeutic targets for head and neck cancer. Mol Cancer. (2022) 21:178. doi: 10.1186/s12943-022-01646-1
38. Zhu JF, Liu Y, Huang H, Shan L, Han ZG, Liu JY, et al. MicroRNA-133b/EGFR axis regulates esophageal squamous cell carcinoma metastases by suppressing anoikis resistance and anchorage-independent growth. Cancer Cell Int. (2018) 18:193. doi: 10.1186/s12935-018-0684-y
39. Wang S, Li J, Xie J, Liu F, Duan Y, Wu Y, et al. Programmed death ligand 1 promotes lymph node metastasis and glucose metabolism in cervical cancer by activating integrin β4/SNAI1/SIRT3 signaling pathway. Oncogene. (2018) 37:4164–80. doi: 10.1038/s41388-018-0252-x
40. Nieto MA, Huang RY, Jackson RA, Thiery JP. EMT: 2016. Cell. (2016) 166:21–45. doi: 10.1016/j.cell.2016.06.028
41. Puisieux A, Brabletz T, Caramel J. Oncogenic roles of EMT-inducing transcription factors. Nat Cell Biol. (2014) 16:488–94. doi: 10.1038/ncb2976
42. Lee JG, Ahn JH, Jin Kim T, Ho Lee J, Choi JH. Mutant p53 promotes ovarian cancer cell adhesion to mesothelial cells via integrin β4 and Akt signals. Sci Rep. (2015) 5:12642. doi: 10.1038/srep12642
43. Liu Z, Sun T, Piao C, Zhang Z, Kong C. METTL14-mediated N(6)-methyladenosine modification of ITGB4 mRNA inhibits metastasis of clear cell renal cell carcinoma. Cell Commun Signal. (2022) 20:36. doi: 10.1186/s12964-022-00831-5
44. Lv T, Lv H, Fei J, Xie Y, Lian D, Hu J, et al. p53-R273H promotes cancer cell migration via upregulation of neuraminidase-1. J Cancer. (2020) 11:6874–82. doi: 10.7150/jca.44718
45. de Almeida LGN, Thode H, Eslambolchi Y, Chopra S, Young D, Gill S, et al. Matrix metalloproteinases: From molecular mechanisms to physiology, pathophysiology, and pharmacology. Pharmacol Rev. (2022) 74:712–68. doi: 10.1124/pharmrev.121.000349
46. Blanco-Mezquita T, Martinez-Garcia C, Proença R, Zieske JD, Bonini S, Lambiase A, et al. Nerve growth factor promotes corneal epithelial migration by enhancing expression of matrix metalloprotease-9. Invest Ophthalmol Vis Sci. (2013) 54:3880–90. doi: 10.1167/iovs.12-10816
47. Ortega P, Moran A, Fernandez-Marcelo T, De Juan C, Frias C, Lopez-Asenjo JA, et al. MMP-7 and SGCE as distinctive molecular factors in sporadic colorectal cancers from the mutator phenotype pathway. Int J Oncol. (2010) 36:1209–15. doi: 10.3892/ijo_00000604
48. Li XL, Liu L, Li DD, He YP, Guo LH, Sun LP, et al. Integrin β4 promotes cell invasion and epithelial-mesenchymal transition through the modulation of Slug expression in hepatocellular carcinoma. Sci Rep. (2017) 7:40464. doi: 10.1038/srep40464
49. Liu SY, Ge D, Chen LN, Zhao J, Su L, Zhang SL, et al. A small molecule induces integrin β4 nuclear translocation and apoptosis selectively in cancer cells with high expression of integrin β4. Oncotarget. (2016) 7:16282–96. doi: 10.18632/oncotarget.v7i13
50. Yang ZY, Jiang H, Qu Y, Wei M, Yan M, Zhu ZG, et al. Metallopanstimulin-1 regulates invasion and migration of gastric cancer cells partially through integrin β4. Carcinogenesis. (2013) 34:2851–60. doi: 10.1093/carcin/bgt226
51. Kelly GT, Faraj R, Dai Z, Cress AE, Wang T. A mutation found in esophageal cancer alters integrin beta4 mRNA splicing. Biochem Biophys Res Commun. (2020) 529:726–32. doi: 10.1016/j.bbrc.2020.06.078
52. Zhu X, Jiang X, Zhang Q, Huang H, Shi X, Hou D, et al. TCN1 deficiency inhibits the Malignancy of colorectal cancer cells by regulating the ITGB4 pathway. Gut Liver. (2023) 17:412–29. doi: 10.5009/gnl210494
53. Tang J, Wang G, Zhang M, Li FY, Sang Y, Wang B, et al. Paradoxical role of CBX8 in proliferation and metastasis of colorectal cancer. Oncotarget. (2014) 5:10778–90. doi: 10.18632/oncotarget.v5i21
54. Ferraro A, Kontos CK, Boni T, Bantounas I, Siakouli D, Kosmidou V, et al. Epigenetic regulation of miR-21 in colorectal cancer: ITGB4 as a novel miR-21 target and a three-gene network (miR-21-ITGΒ4-PDCD4) as predictor of metastatic tumor potential. Epigenetics. (2014) 9:129–41. doi: 10.4161/epi.26842
55. Islam K, Thummarati P, Kaewkong P, Sripa B, Suthiphongchai T. Role of laminin and cognate receptors in cholangiocarcinoma cell migration. Cell Adh Migr. (2021) 15:152–65. doi: 10.1080/19336918.2021.1924422
56. Masugi Y, Yamazaki K, Emoto K, Effendi K, Tsujikawa H, Kitago M, et al. Upregulation of integrin β4 promotes epithelial-mesenchymal transition and is a novel prognostic marker in pancreatic ductal adenocarcinoma. Lab Invest. (2015) 95:308–19. doi: 10.1038/labinvest.2014.166
57. An XZ, Zhao ZG, Luo YX, Zhang R, Tang XQ, Hao D, et al. Netrin-1 suppresses the MEK/ERK pathway and ITGB4 in pancreatic cancer. Oncotarget. (2016) 7:24719–33. doi: 10.18632/oncotarget.v7i17
58. Wang C, Li Z, Shao F, Yang X, Feng X, Shi S, et al. High expression of Collagen Triple Helix Repeat Containing 1 (CTHRC1) facilitates progression of oesophageal squamous cell carcinoma through MAPK/MEK/ERK/FRA-1 activation. J Exp Clin Cancer Res. (2017) 36:84. doi: 10.1186/s13046-017-0555-8
59. Iezzi A, Caiola E, Scagliotti A, Broggini M. Generation and characterization of MEK and ERK inhibitors- resistant non-small-cells-lung-cancer (NSCLC) cells. BMC Cancer. (2018) 18:1028. doi: 10.1186/s12885-018-4949-6
60. Meng X, Liu P, Wu Y, Liu X, Huang Y, Yu B, et al. Integrin beta 4 (ITGB4) and its tyrosine-1510 phosphorylation promote pancreatic tumorigenesis and regulate the MEK1-ERK1/2 signaling pathway. Bosn J Basic Med Sci. (2020) 20:106–16. doi: 10.17305/bjbms.2019.4255
61. Choi SH, Kim JK, Chen CT, Wu C, Marco MR, Barriga FM, et al. KRAS mutants upregulate integrin β4 to promote invasion and metastasis in colorectal cancer. Mol Cancer Res. (2022) 20:1305–19. doi: 10.1158/1541-7786.MCR-21-0994
62. Yu PT, Babicky M, Jaquish D, French R, Marayuma K, Mose E, et al. The RON-receptor regulates pancreatic cancer cell migration through phosphorylation-dependent breakdown of the hemidesmosome. Int J Cancer. (2012) 131:1744–54. doi: 10.1002/ijc.27447
63. Bonuccelli G, Casimiro MC, Sotgia F, Wang C, Liu M, Katiyar S, et al. Caveolin-1 (P132L), a common breast cancer mutation, confers mammary cell invasiveness and defines a novel stem cell/metastasis-associated gene signature. Am J Pathol. (2009) 174:1650–62. doi: 10.2353/ajpath.2009.080648
64. Mori K, Higurashi M, Ishikawa F, Shibanuma M. Rac1-mediated sustained β4 integrin level develops reattachment ability of breast cancer cells after anchorage loss. Cancer Sci. (2021) 112:3205–17. doi: 10.1111/cas.14985
65. Gerson KD, Shearstone JR, Maddula V, Seligmann BE, Mercurio AM. Integrin β4 regulates SPARC protein to promote invasion. J Biol Chem. (2012) 287:9835–44. doi: 10.1074/jbc.M111.317727
66. Mori K, Higurashi M, Ishikawa F, Shibanuma M. Rac1-mediated sustained beta4 integrin level develops reattachment ability of breast cancer cells after anchorage loss. Cancer Sci. (2021) 112:3205–17. doi: 10.1111/cas.14985
67. Gerson KD, Shearstone JR, Maddula V, Seligmann BE, Mercurio AM. Integrin beta4 regulates SPARC protein to promote invasion. J Biol Chem. (2012) 287:9835–44. doi: 10.1074/jbc.M111.317727
68. Banyard J, Chung I, Migliozzi M, Phan DT, Wilson AM, Zetter BR, et al. Identification of genes regulating migration and invasion using a new model of metastatic prostate cancer. BMC Cancer. (2014) 14:387. doi: 10.1186/1471-2407-14-387
69. Drake JM, Barnes JM, Madsen JM, Domann FE, Stipp CS, Henry MD. ZEB1 coordinately regulates laminin-332 and {beta}4 integrin expression altering the invasive phenotype of prostate cancer cells. J Biol Chem. (2010) 285:33940–8. doi: 10.1074/jbc.M110.136044
70. Wilkinson EJ, Woodworth AM, Parker M, Phillips JL, Malley RC, Dickinson JL, et al. Epigenetic regulation of the ITGB4 gene in prostate cancer. Exp Cell Res. (2020) 392:112055. doi: 10.1016/j.yexcr.2020.112055
71. Yao J, Xu C, Fang Z, Li Y, Liu H, Wang Y, et al. Androgen receptor regulated microRNA miR-182-5p promotes prostate cancer progression by targeting the ARRDC3/ITGB4 pathway. Biochem Biophys Res Commun. (2016) 474:213–9. doi: 10.1016/j.bbrc.2016.04.107
72. Bhatia V, Mula RV, Falzon M. Parathyroid hormone-related protein regulates integrin α6 and β4 levels via transcriptional and post-translational pathways. Exp Cell Res. (2013) 319:1419–30. doi: 10.1016/j.yexcr.2013.03.003
73. Kawakami K, Fujita Y, Kato T, Mizutani K, Kameyama K, Tsumoto H, et al. Integrin β4 and vinculin contained in exosomes are potential markers for progression of prostate cancer associated with taxane-resistance. Int J Oncol. (2015) 47:384–90. doi: 10.3892/ijo.2015.3011
74. Nagata M, Noman AA, Suzuki K, Kurita H, Ohnishi M, Ohyama T, et al. ITGA3 and ITGB4 expression biomarkers estimate the risks of locoregional and hematogenous dissemination of oral squamous cell carcinoma. BMC Cancer. (2013) 13:410. doi: 10.1186/1471-2407-13-410
75. Dmello C, Sawant S, Alam H, Gangadaran P, Tiwari R, Dongre H, et al. Vimentin-mediated regulation of cell motility through modulation of beta4 integrin protein levels in oral tumor derived cells. Int J Biochem Cell Biol. (2016) 70:161–72. doi: 10.1016/j.biocel.2015.11.015
76. Li M, Li X, Zhang Y, Wu H, Zhou H, Ding X, et al. Micropeptide MIAC inhibits HNSCC progression by interacting with aquaporin 2. J Am Chem Soc. (2020) 142:6708–16. doi: 10.1021/jacs.0c00706
77. Zhou X, Matskova L, Rathje LS, Xiao X, Gish G, Werner M, et al. SYK interaction with ITGβ4 suppressed by Epstein-Barr virus LMP2A modulates migration and invasion of nasopharyngeal carcinoma cells. Oncogene. (2015) 34:4491–9. doi: 10.1038/onc.2014.380
78. Han Y, Wang L, Wang Y. Integrated analysis of three publicly available gene expression profiles identified genes and pathways associated with clear cell renal cell carcinoma. Med Sci Monit. (2020) 26:e919965. doi: 10.12659/MSM.919965
79. Wei S, Wang J, Oyinlade O, Ma D, Wang S, Kratz L, et al. Heterozygous IDH1(R132H/WT) created by "single base editing" inhibits human astroglial cell growth by downregulating YAP. Oncogene. (2018) 37:5160–74. doi: 10.1038/s41388-018-0334-9
80. Ning J, Wang X, Li N, Cui X, Li N, Zhao B, et al. ZBM-H-induced activation of GRP78 ATPase promotes apoptosis via annexin A7 in A549 lung cancer cells. J Cell Biochem. (2022) 123:798–806. doi: 10.1002/jcb.30224
81. Ning J, Cui X, Li N, Li N, Zhao B, Miao J, et al. Activation of GRP78 ATPase suppresses A549 lung cancer cell migration by promoting ITGB4 degradation. Cell Adh Migr. (2022) 16:107–14. doi: 10.1080/19336918.2022.2130415
82. Yang H, Xu Z, Peng Y, Wang J, Xiang Y. Integrin β4 as a potential diagnostic and therapeutic tumor marker. Biomolecules. (2021) 11. doi: 10.3390/biom11081197
83. Carlow TJ. Medical treatment of nystagmus and ocular motor disorders. Int Ophthalmol Clin. (1986) 26:251–64. doi: 10.1097/00004397-198602640-00022
84. Chen P, Ma T, Yan T, Song Z, Liu C, Pan C, et al. ITGB4 upregulation is associated with progression of lower grade glioma. Sci Rep. (2024) 14:421. doi: 10.1038/s41598-023-49801-y
85. Liu F, Wu Q, Dong Z, Liu K. Integrins in cancer: Emerging mechanisms and therapeutic opportunities. Pharmacol Ther. (2023) 247:108458. doi: 10.1016/j.pharmthera.2023.108458
86. Ruan S, Lin M, Zhu Y, Lum L, Thakur A, Jin R, et al. Integrin β4-targeted cancer immunotherapies inhibit tumor growth and decrease metastasis. Cancer Res. (2020) 80:771–83. doi: 10.1158/0008-5472.CAN-19-1145
Keywords: integrin β4 (ITGb4), tumor metastasis, cell adhesion, epithelialmesenchymal transition (EMT), cancer therapy
Citation: Huang G, Zhou M, Lu D, Li J, Tang Q, Xiong C, Liang F and Chen R (2024) The mechanism of ITGB4 in tumor migration and invasion. Front. Oncol. 14:1421902. doi: 10.3389/fonc.2024.1421902
Received: 23 April 2024; Accepted: 24 July 2024;
Published: 07 August 2024.
Edited by:
Shashi Anand, Mitchell Cancer Institute, United StatesReviewed by:
Kunj Bihari Gupta, Augusta University, United StatesCopyright © 2024 Huang, Zhou, Lu, Li, Tang, Xiong, Liang and Chen. This is an open-access article distributed under the terms of the Creative Commons Attribution License (CC BY). The use, distribution or reproduction in other forums is permitted, provided the original author(s) and the copyright owner(s) are credited and that the original publication in this journal is cited, in accordance with accepted academic practice. No use, distribution or reproduction is permitted which does not comply with these terms.
*Correspondence: Rui Chen, MjAwMXhoMDY2OUBodXN0LmVkdS5jbg==; Fengxia Liang, ZnhsaWFuZzVAaG90bWFpbC5jb20=
†These authors have contributed equally to this work
Disclaimer: All claims expressed in this article are solely those of the authors and do not necessarily represent those of their affiliated organizations, or those of the publisher, the editors and the reviewers. Any product that may be evaluated in this article or claim that may be made by its manufacturer is not guaranteed or endorsed by the publisher.
Research integrity at Frontiers
Learn more about the work of our research integrity team to safeguard the quality of each article we publish.