- 1School & Hospital of Stomatology, Wenzhou Medical University, Wenzhou, Zhejiang, China
- 2Department of Clinical Laboratory, The First Affiliated Hospital of Wenzhou Medical University, Wenzhou, Zhejiang, China
- 3Department of Hematology, Wenzhou Key Laboratory of Hematology, The First Affiliated Hospital of Wenzhou Medical University, Wenzhou, Zhejiang, China
- 4Department of Clinical Laboratory, Peking University Cancer Hospital and Institute, Beijing, China
Elevated plasma fibrinogen (Fg) levels consistently correlate with an unfavorable prognosis in various tumor patient cohorts. Within the tumor microenvironment, aberrant deposition and expression of Fg have been consistently observed, interacting with multiple cellular receptors and thereby accentuating its role as a regulator of inflammatory processes. Specifically, Fg serves to stimulate and recruit immune cells and pro-inflammatory cytokines, thereby contributing to the promotion of tumor progression. Additionally, Fg and its fragments exhibit dichotomous effects on tumor angiogenesis. Notably, Fg also facilitates tumor migration through both platelet-dependent and platelet-independent mechanisms. Recent studies have illuminated several tumor-related signaling pathways influenced by Fg. This review provides a comprehensive summary of the intricate involvement of Fg in tumor biology, elucidating its multifaceted role and the underlying mechanisms.
1 Introduction
Fibrinogen (Fg), a 340 kDa dimeric glycoprotein, is synthesized by hepatocytes and composed of three polypeptide chains: fibrinogen Aα (FGA, 52.0 kDa), Bβ (FGB, 52.0 kDa), and γ (FGG, 46.5 kDa (1). Each Fg molecule comprises two outer D domains connected by a central E domain, with the E-region encompassing the N-terminus of Aα, Bβ, and γ chains, while the D-region consists of the C-terminus of the Bβ and γ chains (2).
Traditionally recognized as a coagulation factor, Fg undergoes transformation into a fibrin polymer during the clotting cascade. In its role as an acute-phase reaction protein, Fg actively engages in inflammatory processes and interacts with the activation and migration of leukocytes (3). Noteworthy, previous research has consistently reported elevated plasma Fg levels across various tumor types, underscoring its significant involvement in tumorigenesis (4).
Moreover, Fg deposition has been observed in the tumor extracellular matrix (5) and the walls of angiogenic blood vessels (6), implying associations between Fg and tumor angiogenesis and metastasis. These interactions are mediated by binding sites on Fg, which engage with diverse molecules, including various plasma proteins and cellular receptors (7). Furthermore, multiple pathways have been identified linking Fg to tumor progression (8). This comprehensive review provides an in-depth overview of the pivotal role played by Fg in tumor biology, emphasizing its crucial contributions to angiogenesis, metastasis, and inflammation within the tumor microenvironment.
2 The role and mechanism of Fg in tumors
2.1 Plasma Fg levels in tumor patient cohorts
Elevated plasma fibrinogen(Fg) levels consistently manifest in diverse tumor patient cohorts (9). In lung, rectal, and stomach cancers, heightened Fg levels correlate with poorer survival (10–12) are recognized as an independent prognostic marker (4). In lymphoma and leukemia, multivariate analysis underscores plasma Fg as a biomarker and a robust predictor.
Table 1 furnishes a comprehensive overview delineating the multifaceted roles and associations of pre-treatment plasma Fg levels within diverse medical contexts. The presented findings underscore the versatile nature of Fg, positioning it as both a prognostic marker and a predictor across varying conditions, including considerations of tumor-node-metastasis (TNM) stage and responses to surgery or chemoradiotherapy.
Notably, although the prognosis of Fg has been demonstrated in most tumors, the prognosis of Fg in certain tumors remains controversial. In patients with relapsed and/or metastatic head and neck squamous cell carcinoma (R/M HNSCC), Fg levels may serve as a predictive indicator for survival (51). However, insights from Nenclares’ research on oral squamous cell carcinoma (OSCC) patients suggest that Fg levels lack predictive value in this context (52). Additionally, it is noteworthy that decreased plasma Fg levels are indicative of poor prognosis in angiosarcoma of the head and neck (ASHN) (53) and acute promyelocytic leukemia (APL) (54), as reported in the literature.
Elevated Fg levels in patients undergoing treatment for various tumors often correlate with poor prognosis, offering clinicians valuable insights for treatment planning and patient management. In surgical settings, heightened preoperative Fg levels typically signal a bleak prognosis post-surgery, warranting vigilant monitoring. Regarding chemotherapy, patients with hepatocellular carcinoma and elevated preoperative plasma Fg levels tend to exhibit poor responses to transarterial chemoembolization (TACE) (55). Similarly, in rectal cancer patients undergoing chemoradiotherapy (CRT) with radiotherapy, those with lower pre-treatment Fg levels (≤270 mg/mL) are more than twice as likely to achieve complete remission compared to counterparts with higher pre-treatment Fg levels (>270 mg/mL) (11). Moreover, in esophageal squamous cell carcinoma (ESCC), patients with elevated Fg levels demonstrate reduced responsiveness to navumab (20). These findings underscore the importance of tailoring chemotherapy regimens to individual cancer patients with elevated Fg levels to optimize treatment efficacy. Therefore, prioritizing the assessment of elevated Fg levels before treatment is imperative, enabling clinicians to customize treatment strategies and accurately evaluate post-treatment prognosis for cancer patients.
2.2 Fg in tumor extracellular matrix
Research has unveiled the presence of Fg in the tumor stroma, predominantly composed of connective tissues, inflammatory cells, and newly formed blood vessels (56). Elevated Fg expression has been detected in various original tumor biopsy samples, encompassing patients with breast cancer (57), uterine cervix carcinoma (58) and HCC (5), among others. Mechanistic insights into Fg expression in the tumor mesenchyme vary across different tumor settings. In cases of central nervous system lymphoma, disruption of the blood-brain barrier contributes to enhanced Fg deposition within the tumor stroma (59). In breast cancer, the binding interactions between Fg β15-42 and VE-cadherin improve endothelial barrier permeability (60). Increased vascular permeability within tumors results in the leakage of Fg from plasma into the tumor stroma (61). Notably, Fg deposition in acute promyelocytic leukemia is mediated by Fg binding to CD44 on APL blasts and NB4 cells (62).
Furthermore, tumor cells, including lung adenocarcinoma A549 cells (63), hepatocellular carcinoma HepG2 cells (64), human breast carcinoma MCF-7 cells (57) and uterine cervix carcinoma ME-180 cell (58), have been identified as capable of synthesizing and secreting Fg. This synthesis is attributed to elevated transcription of fibrinogen Aα (FGA), fibrinogen Bβ (FGB), and fibrinogen γ (FGG) genes.
Table 2 provides a comprehensive summary of diverse observations related to Fg expression across different pathological contexts.
2.3 Pro-inflammatory role of Fg in tumors
Inflammation serves as a driving force across all stages of carcinogenesis, fostering cancer growth. The creation of an inflammatory tumor microenvironment (TME) results from intricately coordinated interactions involving cancer cells, stromal cells, and inflammatory cells (76). Additionally, tumor cells actively contribute to crucial stages of leukocyte recruitment and trafficking (77). These processes encompass chemokine-induced migration, coupled with the activation of leukocyte integrins and selectin adhesion molecules, facilitating the interaction of tumor cells with vascular endothelium and subsequent extravasation.
Vascular and leukocyte responses play pivotal roles in the pathogenesis of inflammation. Elevated vascular permeability is among the initial responses to inflammation. Fibrinogen (Fg) plays a role in facilitating the subsequent transendothelial migration of leukocytes by binding to VE-cadherin in vascular endothelial cells (60). Additionally, Fg activates AKT signaling and induces microfilament depolymerization, thereby enhancing endothelial barrier permeability (78).
Recent evidence has highlighted the association between circulating Fg and DNA methylation in peripheral blood leukocytes (79), underscoring Fg’s role in leukocyte migration and recruitment. Acting as a bridging molecule, Fg links leukocyte integrin αMβ2 (Mac-1) to intercellular adhesion molecule-1 (ICAM-1) on endothelial cells (ECs) (80), facilitating leukocyte adhesion and transvascular migration across the vascular endothelium. Moreover, at sites of inflammation, Fg interacts with immune cells, hastening their recruitment to participate in the inflammatory cascade (81). (Figure 1) A reciprocal relationship exists between leukocytes and Fg, wherein during inflammation, reactive oxygen species (ROS) from neutrophils and monocytes, along with nitric oxide from lymphocytes and monocytes, cleave Fg (82). Subsequently, protease-cleaved Fg binds to macrophage toll-like receptors, amplifying the inflammatory response through an allergic cascade (83). This establishes a positive feedback loop between Fg and immune cells, intensifying the inflammatory milieu.
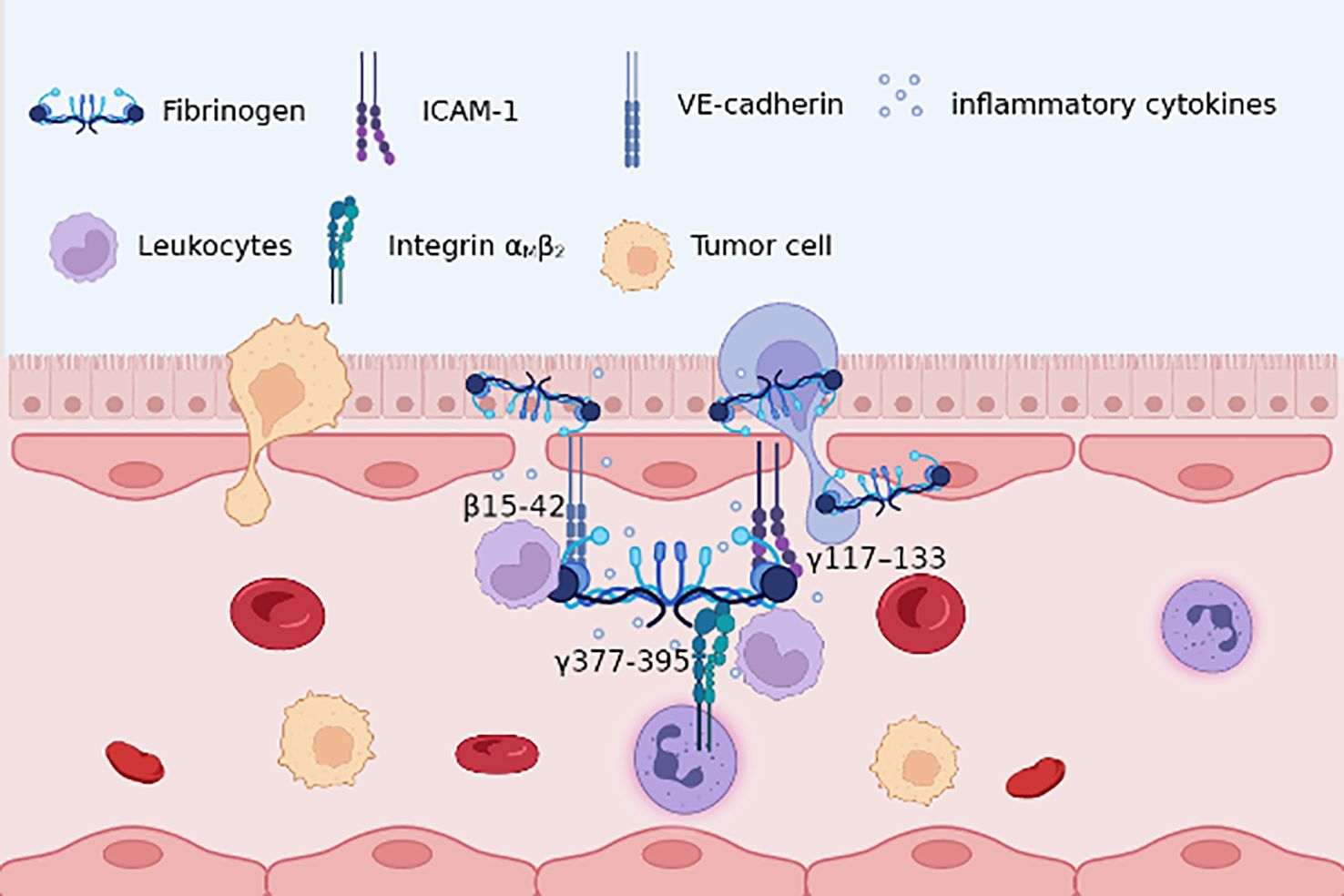
Figure 1 Fg β15-42, γ117–133 with endothelial cells VE-cadherin, ICAM-1, respectively, improves endothelial barrier permeability; γ377-395 with leukocytes integrin αMβ2 promotes adhesion and migration. Besides, Fg may lead to the recruitment of pro-inflammatory cytokines, thus providing an inflammatory role in TME.
Moreover, inflammatory mediators play crucial regulatory roles in both vascular and leukocyte responses. Fg has been implicated in promoting inflammatory responses by enhancing the secretion of inflammatory factors, either independently or in response to various environmental cues.
Studies have demonstrated that Fg upregulates the secretion of several cytokines, including IL-6, IL-17, IL-23, TNF-α, macrophage inflammatory protein-1 α (MIP-1α), MIP-1β, MIP-2, and monocyte chemotactic protein-1 (MCP-1) (84–86). The molecular mechanisms underlying Fg-mediated promotion of inflammatory factors have also been elucidated. Fg participates in the inflammatory process by activating the NF-κB pathway and inducing the expression of interleukin-6 (IL-6), interleukin-8, monocyte chemotactic protein-1 (MCP-1), and C-C chemokine ligand-2 (CCL2) (87, 88).
Furthermore, Fg has been found to stimulate cytokine secretion under various conditions. Specific chemical modifications, such as D-ibose glycosylation, can enhance Fg’s involvement in specific inflammatory responses, resulting in increased expression of inflammation-associated cytokine genes, including TNF-α, IL-6, IL-1β, and IFN-γ (89). Additionally, specific enzymatic structures, such as atypical cross-linking mediated by the glutamine transferase transglutaminase-2 (TG2), enhance the secretion of pro-inflammatory cytokines (e.g. TNF-α) in myeloid macrophages, while simultaneously reducing the expression of IL-10 and inhibiting the phosphorylation of STAT3 (90).
In the specific context of a tumor, the role of Fg and immune cells undergo alterations. Typically, during inflammation, Fg activates macrophages, polarizing them into pro-inflammatory M1 macrophages via integrin α1β3. This activation leads to the expression of M1 macrophage-specific markers such as inducible nitric oxide synthase (iNOS) and pro-inflammatory cytokines (IL-1β, IL-6, TNF-α) (91). However, within the tumor microenvironment, Fg becomes associated with increased infiltration of tumor-associated macrophages (TAMs) exhibiting an M2 phenotype. These M2 TAMs promote tumor growth, induce angiogenesis, and suppress immune responses (20).
Importantly, within the specific context of a tumor, the interplay between Fg and immune cells may contribute to tumor development. Distinct interactions occur between Fg γ390-396A and leukocyte integrin αMβ2 (Mac-1) (92), typically mediated by Fg binding to CD11b (93). These interactions lead to the activation of downstream proteins, including focal adhesion kinase (FAK) phosphorylation and mitogen-activated protein kinase (MAPK) activation. Consequently, this triggers various processes such as leukocyte degranulation, recruitment (94), phagocytosis, and induction of inflammatory responses (95). Specifically, within the tumor microenvironment, Fg γ390-396A’s interaction with leukocyte integrin αMβ2 not only enhances the secretion of pro-inflammatory cytokines like IL-6, IL-1β, IFN-γ, and TNF-α but also promotes tumor cell proliferation (96). This dual role underscores Fg’s contribution to inflammation and tumor development within tumors.
2.4 Fg and angiogenesis
The relationship between Fibrinogen (Fg) and angiogenesis remains a subject of ongoing debate, with recent research shedding light on its dual role—both pro- and anti-angiogenic—in specific tissue contexts, particularly within the spectrum of various diseases.
The role of Fg in angiogenesis varies depending on the environmental context. In skin wound models, a distinctive pattern emerges as Fg infiltrates the lesion during the inflammatory phase, coinciding with a decline in vascular integrity. Notably, this Fg deposition correlates with heightened re-epithelialization and accelerated angiogenesis, emphasizing its context-dependent pro-angiogenic effects (97). Contrasting observations arise in a colon cancer MC38 model, where mice deficient in Fg exhibit lower vascular density compared to control mice. This effect is found to be time-dependent (98), further illustrating the intricate dynamics involved.
Fg demonstrates a pro-angiogenic effect under certain conditions. Recent insights highlight the direct interaction of circulating Fg with various growth factors, encompassing members of the vascular endothelial growth factor (VEGF) family and fibroblast growth factor (FGF). This interaction stimulates endothelial cell proliferation, fostering angiogenesis, and fueling tumor cell growth (99, 100). Mechanistically, tumor-derived Fg amplifies the impact of FGF-2 on endothelial proliferation through coordinated effects involving integrin αvβ3 and FGFR1 (FGF receptor 1) (101).
Moreover, Fg can promote angiogenesis through alternative mechanisms. Fg collaborates with immune cells to orchestrate a dual-promotional role in immune cell recruitment and angiogenesis (102). This multifaceted engagement includes the inhibition of tumor angiogenesis inhibitors, such as Endostatin, by Fg, thereby promoting angiogenic aggregation (103).
Additionally, specific fragments of Fg have been implicated in promoting angiogenesis. Fg α component enhances mesenchymal cell-endothelial cell interactions, actively participating in angiogenesis through the VEGA-VEGFR-FAK signaling axis (104).Exploring the intricate landscape of Fg binding sites involved in tumor angiogenesis reveals a nuanced interplay of molecular interactions that influence endothelial cell behavior. One reported interaction spotlights arginine-glycine-aspartic acid (RGD) site in at α252-254 or α572–574 region, showcasing its direct engagement with integrin αvβ3. This interaction holds the potential to function as a survival signal for endothelial cells, orchestrating pivotal roles in the angiogenic process (105).
Contrastingly, some studies challenge the notion of Fg’s indispensability for angiogenesis, suggesting potential anti-angiogenic roles. Integrin αvβ3 serves as a pivotal receptor that mediates the interaction between Fg and angiogenesis, with different fragments of Fg exhibiting distinct effects upon binding to this receptor. Interestingly, while certain Fg fragments have been associated with pro-angiogenic effects through integrin αvβ3 binding, others have been linked to the inhibition of tumor angiogenesis. An intriguing find lies in the β43-63 region, identified as a novel anti-tumor peptide due to its anti-angiogenic activity mediated by integrin αvβ3 (106). Additionally, the γC component and its truncation mutant (γC399tr) emerge as determinants in the binding of Fg to endothelial cells via integrin αvβ3. This interaction, in turn, contributes to endothelial cell apoptosis and a reduction in tube formation (107) (Figure 2).
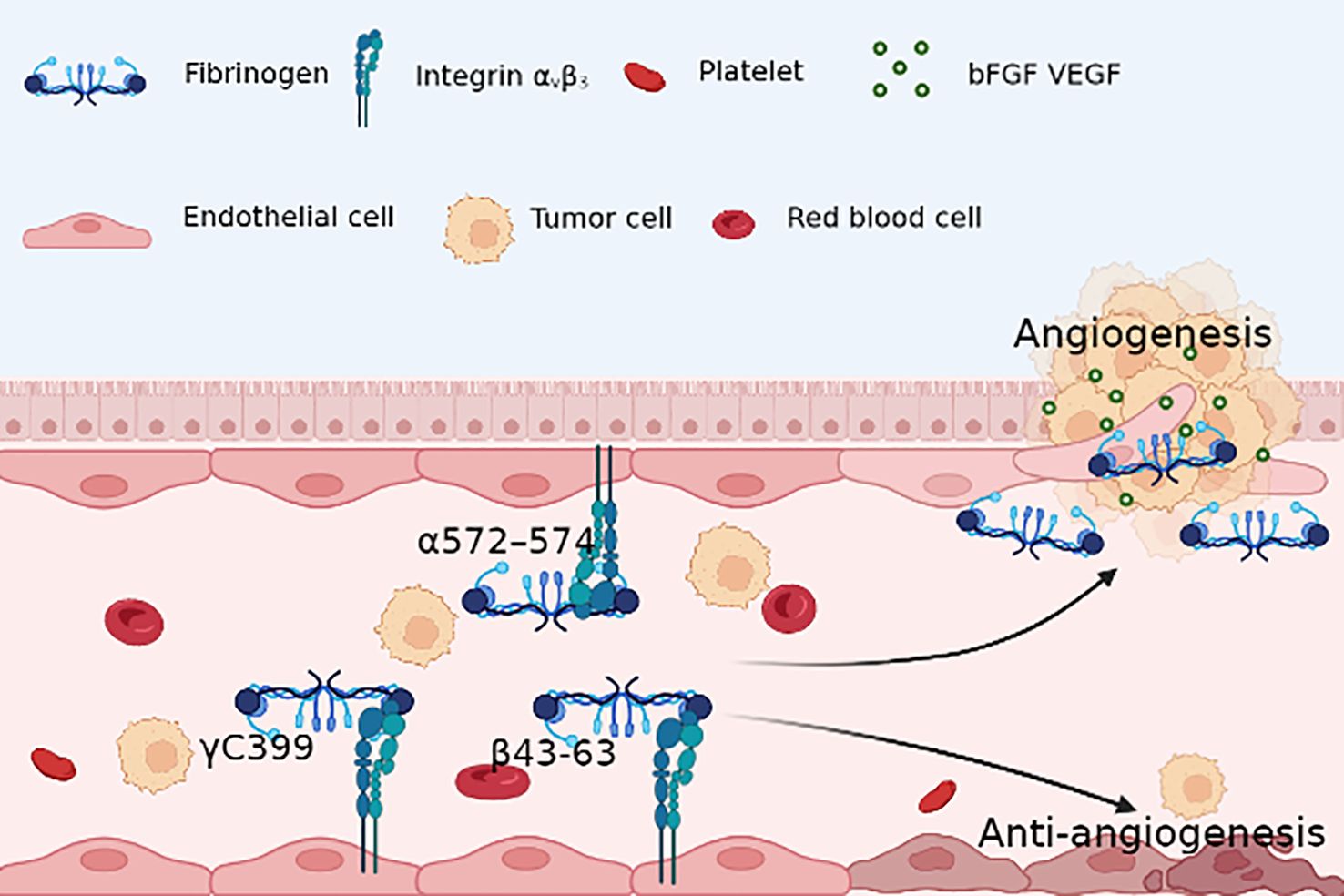
Figure 2 Fg RGD sequence(α572–574) with endothelial cells integrin αvβ3 provides survival signal for ECs and activates microvascular ECs, contributing to angiogenesis; however, Fg γC399 and β43-63 induce apoptosis of ECs, which results in an anti-angiogenesis environment.
Moreover, certain fragments of Fg have been reported to be linked to the inhibition of tumor angiogenesis. A key functional fragment, Fg α1-24, known as alphastatin, exhibits inhibitory effects on bFGF- and VEGF-induced migration, proliferation, and tubule formation of microvascular endothelial cells (108). This inhibition is mediated through the suppression of the JNK and ERK kinase pathways (109). Alphastatin’s localized effects extend to cellular necrosis, thrombosis, and vessel rupture (110), collectively acting as a potent inhibitor of angiogenesis and tumor growth (111). Notably, the down-regulation of Fg E-fragment in tumors (112) hinted at its detrimental impact on tumor growth. Subsequent investigations propose that the Fg E fragment might be linked to the inhibition of tumor angiogenesis.
Despite numerous studies reporting either promotion or inhibition of angiogenesis by Fg and its fragments, there are also studies indicating that Fg may not be significantly associated with angiogenesis in certain contexts. Tumor analysis from Fg-deficient mice and normal controls exhibited no genotype-dependent variations in vessel density and pattern (113). In regions with heightened Fg leakage, quantifying vascular density revealed no alterations in blood vessel size or density, dissociating elevated plasma Fg from angiogenesis (114).
2.5 Fg and metastasis
The coagulation cascade and platelet activation stand as pivotal drivers of metastasis, orchestrating a multifaceted interplay in tumor progression. Fibrinogen(Fg), a crucial constituent of the tumor microenvironment, facilitates tumor metastasis via both platelet-dependent and platelet-independent pathways.
Initially, Fg may foster tumor metastasis through platelet-dependent mechanisms. Platelets, circulating in the bloodstream, emerge as essential accomplices in facilitating tumor metastasis, underscoring their intricate involvement in the metastatic cascade. Platelet membranes host a myriad of adhesion molecules, including integrin α6β1, αvβ3and αIIbβ3, emerges as a critical mediator in platelet-tumor cell interactions, particularly through its facilitation of integrin αvβ3 binding to tumor cells (115). Moreover, he interaction between platelet integrin αIIbβ3 and specific Fg sequences, such as γA400-411 and arginine-glycine-aspartic acid(RGD) (116), enhances cancer cell adhesion to endothelial cells, fostering the dissemination of tumor cells (116, 117). Notably, studies in mice lacking Fg and Gαq, a G protein pivotal for platelet activation, showcased significantly reduced tumor cell survival compared to control counterparts (118, 119). This underscores the potential of Fg in bolstering platelet-tumor cell interactions, thereby modulating the metastatic potential mediated by platelets.
However, divergent perspectives exist regarding the role of Fg in cancer metastasis independent of platelets. Fg β15-42 emerges as a key player in augmenting vascular permeability and facilitating tumor cell migration across endothelial cells by binding to VE-cadherin (60). Furthermore, Fg serves as a mediator for matrix metalloproteinase (MMP)-2 and MMP-9, pivotal enzymes involved in tumor metastasis, by participating in extracellular matrix remodeling (74). These findings underscore Fg’s intricate involvement in regulating tumor cell migration during metastasis by integrating into the ECM and modulating matrix composition (120) (Figure 3).
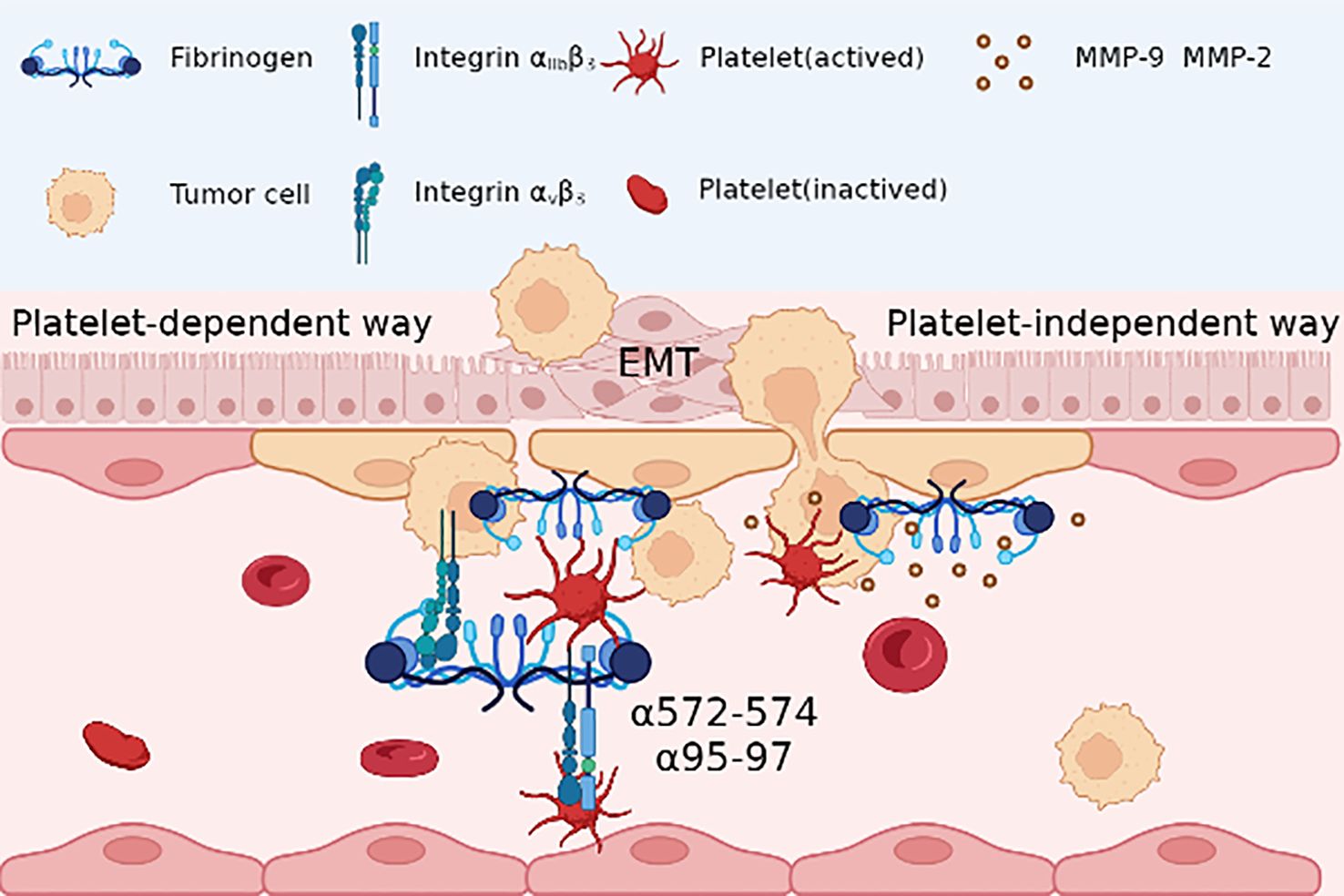
Figure 3 In platelet dependent way, Fg acts as a bridge between the reaction between tumor cells and platelet, with adhesion to tumor integrin αvβ3 and RGD sequence(α572-574, α95-97) binding to platelet integrin αIIbβ3; in platelet-independent way, Fg may be together with matrix metalloproteinase (MMP)-2 and MMP-9, promoting the epithelial-mesenchymal transition (EMT) and migration of tumor cells.
Furthermore, Fg can directly interact with tumor cells, thereby enhancing their metastatic potential. Fg plays a pivotal role in fostering persistent adherence, survival of metastatic emboli post-tumor cell intravasation, and contributes significantly to spontaneous metastasis (25, 27). Interactions between cancer cells and Fg, particularly in cases where cancer cells express ICAM-1, expedite endothelial penetration for metastasis by forming Fg-dependent bridges (121). Furthermore, the citrullination of Fg by lung endothelial cells promotes tumor cell aggregation and enhances metastatic potential (122).
Insights derived from studies involving Fg-deficient mice provide valuable illumination into the multifaceted role of Fg in metastasis. These investigations have yielded evidence showcasing a measurable reduction in lung micro-metastasis or overall tumor burden in the absence of Fg (113, 123). Crucially, this quantitative decline doesn’t correlate with observable changes in tumor stroma development or the general growth pattern of tumors. It’s noteworthy that the reduction in metastatic occurrences, though significant, falls short of achieving complete elimination. This intriguing observation was consistent across models involving Lewis lung carcinoma and the B16-BL6 melanoma, indicating a nuanced relationship between Fg and the metastatic process (124). This nuanced interplay suggests that while Fg deficiency exerts a discernible impact, it does not entirely eradicate metastatic events, prompting a deeper exploration into the specific mechanisms and contexts governing the complex interaction between Fg and metastasis.
Contrastingly, evidence supporting an anti-metastatic role for Fg and its fragments also exists. In a Met-1 breast cancer nude mouse model injected with the γC truncation mutant (γC399tr), reduced metastasis was observed compared to control mice, indicating the potential of γC399tr in impeding tumor growth (107). Additional findings revealed that in vivo subcutaneous injection of fibrinogen Aα(FGA) knockout (KO) A549 cells into immunodeficient BALB/c nude mice led to increased A549 cell proliferation and colonization, suggesting an inhibitory role of Fg in this context (125). Moreover, a unique perspective posits Fg’s anti-metastatic influence in anatomical sites characterized by low Fg concentrations, such as lymphatics and body cavities. Schneider et al.’s hypothesis suggesting the suppression of tumor cell migration by Fg, acting as a counterforce to free vitronectin, adds a layer of complexity to our understanding of Fg’s diverse roles in metastasis (126).
These multifaceted observations underscore the intricate and context-dependent nature of Fg’s impact on metastatic processes, warranting further exploration to decipher the nuanced interplay between Fg and tumor metastasis.
2.6 Molecular pathways of Fg in regulation of tumor progression
Currently, investigations into the relationship between Fibrinogen (Fg) and tumors predominantly center around clinical data analysis, with a limited number of studies delving into the intricate molecular mechanisms at play. In diverse tumor microenvironments, the molecular pathways involving Fg exhibit variability, indicating the multifaceted role of Fg in tumorigenesis.
In the context of lung adenocarcinoma (LUAD), Fg typically promotes tumor proliferation and metastasis, Fibrinogen Aα(FGA) exerts inhibitory effects on tumor growth, metastasis, and invasion while fostering apoptosis through the suppression of the PI3K-AKT-mTOR pathway (125).
Shifting focus to esophageal squamous cell carcinoma (ESCC), Fg demonstrates a distinctive impact. Here, Fg is implicated in the promotion of cell migration and invasion, albeit without a significant influence on proliferation. This nuanced effect is achieved through the induction of Epithelial-Mesenchymal Transition (EMT) via the p-AKT/p-mTOR pathway (8). In some ESCC patients with elevated Fg, TP53, KMT2D, and NOTCH1 actionable gene variants were detected, but the specific signaling pathways remain to be studied (20).
Moving to colon cancer, the interaction between Fg and tumor cells is facilitated by the activation of Focal Adhesion Kinase (FAK), concurrently inhibiting the tumor suppressor p53 and its downstream targets, such as 14-3-3σ and p21. This concerted action promotes unbridled cell growth while inhibiting senescence (67).
Within the intricacies of the hepatocellular carcinoma (HCC) microenvironment, Fg emerges as a pivotal player, exerting its influence through interactions with integrin αvβ5 and subsequent activation of hepatic stellate cells (HSCs). This interaction establishes a robust correlation with both the incidence and recurrence of HCC in affected individuals (5). Fg’s impact extends beyond mere association, actively promoting migration and invasion of hepatocellular carcinoma cells. These pro-metastatic effects are mediated through the activation of the PTEN/AKT/mTOR pathway, coupled with the induction of epithelial-mesenchymal transition (EMT) (127). Furthermore, in hepatoma cell lines, the expression of Fg undergoes significant modulation. This modulation is intricately linked to the degradation of signal transducer and activator of transcription (STAT), providing a glimpse into the regulatory mechanisms governing Fg expression (128). Significantly, the targeted inhibition of p38 MAPK demonstrates a marked ability to downregulate Fg expression, thereby illuminating a promising avenue for precision therapeutic interventions (128). This finding underscores the potential interplay between Fg and key signaling pathways, including JAK/STAT and p38 MAPK, hinting at intricate regulatory mechanisms within the cellular milieu. In addition, in HCC, fibrinogen Aα(FGA) can act as a tumor suppressor with opposite effects. It has been shown that FGA mutations can promote hepatocellular carcinoma development by activating TYK2-STAT3 signaling and increasing IL-6 expression (129). Additionally, FGA can impede AKT phosphorylation and its downstream effectors, such as Bcl-2, by binding to HBsAg, thereby diminishing pro-survival protein levels while augmenting pro-apoptotic protein expression (130). The intricate interplay of these molecular events highlights Fg’s multifaceted role in shaping the aggressive behavior of hepatocellular carcinoma.
Within gallbladder cancer (GBC) cells, Fg orchestrates a multifaceted interplay, aggregating macrophages to propel angiogenesis. This effect is mediated through the upregulation of Intercellular Adhesion Molecule-1 (ICAM-1) expression (131). Moreover, Fg induces a phenotypic shift in GBC cells, typified by an increase in the mesenchymal marker vimentin, coupled with a concomitant decrease in the epithelial marker E-cadherin. This dual modulation strongly implies that Fg plays a contributory role in cell migration and invasion, potentially through the induction of Epithelial-Mesenchymal Transition (EMT) signaling (132).
Within the intricate milieu of ovarian cancer, Fg emerges as a dynamic orchestrator, stimulating fibroblasts to enhance the production of Type I alpha 1 collagen (COL1A1). Simultaneously, Fg acts as a catalyst, activating the AKT signaling pathway (133). These concerted actions contribute to the facilitation of cancer metastasis, marking Fg as a key player in shaping the metastatic landscape of ovarian cancer.
In breast cancer, the extracellular matrix, including Fg, plays a pivotal role in modulating tumor cell behavior, particularly proliferation and quiescence, through mechanical stimuli. Lower mechanical forces stimulate the cytoskeleton/AIRE axis via integrin β1/3 receptor activation, enhancing tumor cell potential. Conversely, excessive mechanical forces activate DDR2/STAT1/P27 signaling, inducing cell cycle arrest and transitioning stem cell-like cancer cells into a quiescent state (134).
This nuanced understanding of Fg regulation offers valuable insights into the potential synergies between different signaling cascades (Figure 4), providing a foundation for the development of targeted therapeutic strategies aimed at modulating Fg-mediated pathways in disease states.
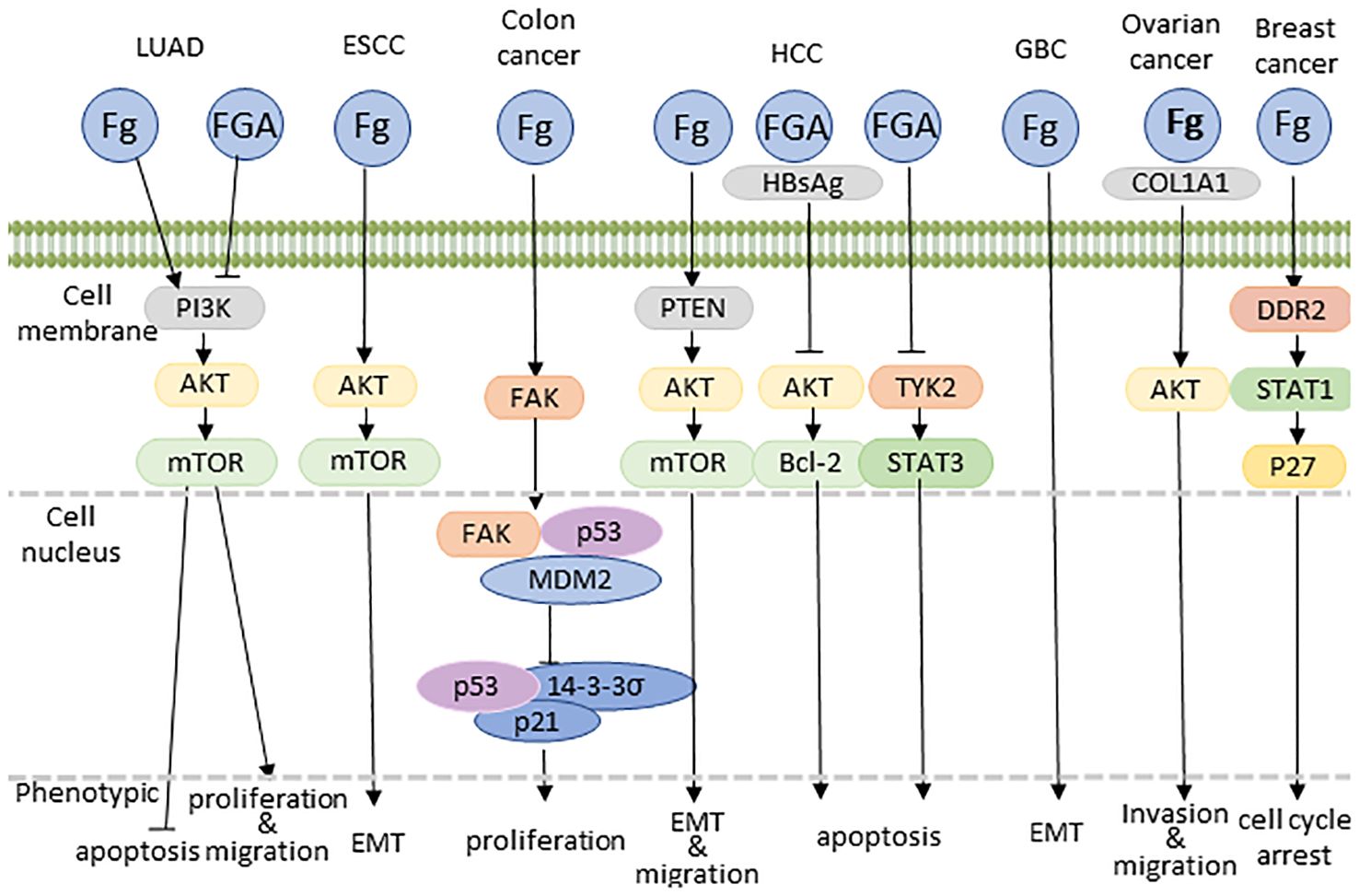
Figure 4 Proposed model explaining Fg-driven tumor growth. COL1A1, type I alpha 1 collagen; EMT, epithelial to mesenchymal transition.
3 Discussion
The existing comprehension of the relationships between Fibrinogen (Fg) and tumors primarily rests on clinical data, consistently indicating that elevated plasma Fg levels serve as independent predictors for an unfavorable prognosis across various tumor types. A mounting body of evidence underscores Fg’s multifaceted role in promoting tumorigenesis, encompassing pro-inflammatory, metastatic, and angiogenic effects that collectively contribute to tumor progression.
Based on our thorough literature review, we anticipate significant potential for further development in understanding the relationship between Fg and tumors. Primarily, in clinical contexts, Fg determination methods need not be confined solely to plasma analysis, such as the traditional Clauss method; exploring liquid biopsy (135) or salivary Fg detection (136) could shed light on Fg’s prognostic implications for tumors. There is a pressing need for more comprehensive studies on how Fg levels may interfere with various chemotherapy regimens and impact the development of therapy resistance. Furthermore, future investigations could delve into the prognostic significance of plasma Fg ratios when combined with other tumor prognostic markers, such as the fibrinogen-to-albumin ratio (137, 138), F-NLR (fibrinogen and neutrophil-lymphocyte ratio) (139), among others. Additionally, there is scope for further exploration of the roles played by Fg fragments, including FGA, FGB, and FGG, in the context of tumor prognosis.
In terms of basic research, while the prognostic significance of plasma Fg in various tumors has been extensively investigated, several areas warrant further exploration. These include elucidating the mechanisms underlying the association between elevated Fg levels prior to treatment and the subsequent poor prognosis of cancer patients post-surgery or chemotherapy. Additionally, the mechanisms governing Fg deposition within the microenvironment of diverse tumor types, as well as its involvement in processes such as inflammation, angiogenesis, and metastasis, require thorough investigation. Future studies could focus on uncovering the intricate molecular mechanisms through which Fg influences tumor progression across different cancer types. This could involve identifying specific integrins that interact with Fg, elucidating downstream effector molecules modulated by Fg, and assessing the potential relevance of these Fg-mediated signaling pathways in various human tumors. Furthermore, in terms of experimental protocol development, research targeting Fg as a therapeutic target has primarily been limited to a few tumor cell lines or murine models, neglecting the broader spectrum of tumor types. Given the challenges associated with Fg deficiency and its implications for hemostasis, refining experimental protocols is imperative. More precise methodologies are urgently needed to facilitate in situ injection of cancer cell lines into Fg-knockout mice, enabling a more comprehensive understanding of Fg’s role in tumor biology. Lastly, there is scope to expand investigations into the interactions between Fg-related fragments and tumors, which may yield novel insights. Exploring these interactions could uncover unexpected findings and contribute to a deeper understanding of the complex interplay between Fg and tumorigenesis.
The translation of relevant research findings on Fg and tumors into clinical applications is a promising endeavor that can significantly impact tumor treatment protocols. Firstly, our review findings suggest a strong association between elevated pretreatment Fg levels and poor prognosis in most tumor patients. This association can serve as a valuable guide for determining treatment regimens and post-treatment management strategies for cancer patients. Additionally, compared to current tumor markers that often involve cumbersome testing steps like immunohistochemistry, assessing Fg levels through pre-treatment coagulation is more convenient, accessible, and provides a quicker reference value. Secondly, Fg can serve as a tool for stratifying and assessing individual tumor risk. Particularly in cases where tumors lack precise and specific prognostic biomarkers, plasma Fg offers significant clinical utility. Furthermore, specific fragments of Fg, such as FGA, FGB, and FGG, may exert distinct effects on tumors compared to intact Fg. For instance, FGA may function as an inhibitory factor, presenting itself as a potential target for clinical interventions and tumor treatments. Lastly, targeting Fg to mitigate its role in promoting tumorigenesis and development holds promise for innovative cancer treatments. Strategies like partial inhibition of the Fg gene or blocking downstream signaling pathways activated by Fg could prevent its involvement in tumor metastasis and angiogenesis. These approaches offer new avenues for cancer treatment and have the potential to enhance the effectiveness and customization of therapeutic strategies.
Author contributions
XW: Writing – review & editing, Writing – original draft. XY: Writing – review & editing, Writing – original draft. CC: Writing – review & editing, Writing – original draft. CIC: Writing – review & editing, Writing – original draft. YW: Writing – review & editing, Writing – original draft. DS: Writing – review & editing, Writing – original draft. LZ: Writing – review & editing, Writing – original draft.
Funding
The author(s) declare financial support was received for the research, authorship, and/or publication of this article. This work was supported by grant 82272784 from the National Natural Science Foundation of China and grant LY22H200004 from the Natural Science Foundation of Zhejiang Province.
Conflict of interest
The authors declare that the research was conducted in the absence of any commercial or financial relationships that could be construed as a potential conflict of interest.
Publisher’s note
All claims expressed in this article are solely those of the authors and do not necessarily represent those of their affiliated organizations, or those of the publisher, the editors and the reviewers. Any product that may be evaluated in this article, or claim that may be made by its manufacturer, is not guaranteed or endorsed by the publisher.
References
1. Kant JA, Fornace AJ Jr., Saxe D, Simon MI, McBride OW, Crabtree GR. Evolution and organization of the fibrinogen locus on chromosome 4: gene duplication accompanied by transposition and inversion. Proc Natl Acad Sci U.S.A. (1985) 82:2344–8. doi: 10.1073/pnas.82.8.2344
2. Mosesson MW. Fibrinogen and fibrin structure and functions. J Thromb Haemost. (2005) 3:1894–904. doi: 10.1111/j.1538-7836.2005.01365.x
3. Luyendyk JP, Schoenecker JG, Flick MJ. The multifaceted role of fibrinogen in tissue injury and inflammation. Blood. (2019) 133:511–20. doi: 10.1182/blood-2018-07-818211
4. Perisanidis C, Psyrri A, Cohen EE, Engelmann J, Heinze G, Perisanidis B, et al. Prognostic role of pretreatment plasma fibrinogen in patients with solid tumors: A systematic review and meta-analysis. Cancer Treat Rev. (2015) 41:960–70. doi: 10.1016/j.ctrv.2015.10.002
5. Yan C, Yang Q, Gong Z. Activation of hepatic stellate cells during liver carcinogenesis requires fibrinogen/integrin Avβ5 in Zebrafish. Neoplasia. (2018) 20:533–42. doi: 10.1016/j.neo.2018.02.002
6. Halder SK, Milner R. The impact of chronic mild hypoxia on cerebrovascular remodelling; uncoupling of angiogenesis and vascular breakdown. Fluids Barriers CNS. (2021) 18:50. doi: 10.1186/s12987-021-00284-x
7. Wolberg AS. Fibrinogen and fibrin: synthesis, structure, and function in health and disease. J Thromb Haemost. (2023) 21:3005–15. doi: 10.1016/j.jtha.2023.08.014
8. Zhang F, Wang Y, Sun P, Wang ZQ, Wang DS, Zhang DS, et al. Fibrinogen promotes Malignant biological tumor behavior involving epithelial-mesenchymal transition via the P-akt/P-mtor pathway in esophageal squamous cell carcinoma. J Cancer Res Clin Oncol. (2017) 143:2413–24. doi: 10.1007/s00432-017-2493-4
9. Beleva EA, Deneva TI, Stoencheva SS, Grudeva-Popova ZG. Longitudinal dynamics of coagulation and angiogenesis markers in cancer patients during and after chemotherapy. Clin Appl Thromb Hemost. (2021) 27:10760296211056637. doi: 10.1177/10760296211056637
10. Cheng F, Zeng C, Zeng L, Chen Y. Clinicopathological and prognostic value of preoperative plasma fibrinogen in gastric cancer patients: A meta-analysis. Med (Baltimore). (2019) 98:e17310. doi: 10.1097/md.0000000000017310
11. Lee JH, Hyun JH, Kim DY, Yoo BC, Park JW, Kim SY, et al. The role of fibrinogen as a predictor in preoperative chemoradiation for rectal cancer. Ann Surg Oncol. (2015) 22:209–15. doi: 10.1245/s10434-014-3962-5
12. Cai H, Zhang ZH, Zhou YJ, Liu J, Chen HQ, Lin RY. The prognostic value of preoperative plasma fibrinogen and neutrophil-to-lymphocyte ratio in patients with laryngeal squamous cell carcinoma. Ear Nose Throat J. (2021) 100:731–6. doi: 10.1177/0145561320920746
13. He SS, Wang Y, Yang L, Chen HY, Liang SB, Lu LX, et al. Plasma fibrinogen correlates with metastasis and is associated with prognosis in human nasopharyngeal carcinoma. J Cancer. (2017) 8:403–9. doi: 10.7150/jca.17028
14. Fan S, Guan Y, Zhao G, An G. Association between plasma fibrinogen and survival in patients with small-cell lung carcinoma. Thorac Cancer. (2018) 9:146–51. doi: 10.1111/1759-7714.12556
15. Mitsui S, Tanaka Y, Doi T, Hokka D, Maniwa Y. Prognostic value of preoperative plasma fibrinogen levels in resected stage I non-small cell lung cancer. Thorac Cancer. (2022) 13:1490–5. doi: 10.1111/1759-7714.14419
16. Sinn K, Mosleh B, Grusch M, Klepetko W, Hoetzenecker K, Klikovits T, et al. Impact of fibrinogen levels and modified glasgow prognostic score on survival of stage iii/N2 non-small cell lung cancer patients treated with neoadjuvant therapy and radical resection. BMC Cancer. (2022) 22:1197. doi: 10.1186/s12885-022-10298-9
17. Su Z, Huang J, Chen W, Sun A, Bai X, Huang L, et al. High diagnostic value of plasma fibrinogen for osteomyelitis of the jaws after oral cancer surgery. Oral Dis. (2022) 28:1907–10. doi: 10.1111/odi.13911
18. Wu M, Ye P, Zhang W, Zhu H, Yu H. Prognostic role of an inflammation scoring system in radical resection of oral squamous cell carcinoma. BMC Oral Health. (2022) 22:226. doi: 10.1186/s12903-022-02261-8
19. Wakatsuki K, Matsumoto S, Migita K, Ito M, Kunishige T, Nakade H, et al. Preoperative plasma fibrinogen is associated with lymph node metastasis and predicts prognosis in resectable esophageal cancer. World J Surg. (2017) 41:2068–77. doi: 10.1007/s00268-017-3991-x
20. Hoshino S, Matsuda S, Kawakubo H, Yamaguchi S, Nakamura K, Aimono E, et al. Elevation of the prognostic factor plasma fibrinogen reflects the immunosuppressive tumor microenvironment in esophageal squamous cell carcinoma. Ann Surg Oncol. (2022) 29:6894–904. doi: 10.1245/s10434-022-11974-7
21. Wang H, Yin X, Ma K, Wang Y, Fang T, Zhang Y, et al. Nomogram based on preoperative fibrinogen and systemic immune-inflammation index predicting recurrence and prognosis of patients with borrmann type iii advanced gastric cancer. J Inflammation Res. (2023) 16:1059–75. doi: 10.2147/jir.S404585
22. Parisi R, Panzera T, Russo L, Gamba S, De Curtis A, Di Castelnuovo A, et al. Fibrinogen levels in relation to colorectal cancer onset: A nested case-cohort study from the moli-sani cohort. Front Cardiovasc Med. (2022) 9:1009926. doi: 10.3389/fcvm.2022.1009926
23. Huang G, Jiang H, Lin Y, Wu Y, Cai W, Shi B, et al. Prognostic value of plasma fibrinogen in hepatocellular carcinoma: A meta-analysis. Cancer Manag Res. (2018) 10:5027–41. doi: 10.2147/cmar.S175780
24. Xu J, An S, Lu Y, Li L, Wu ZQ, Xu HG. Preoperative alpha fetoprotein, total bilirubin, fibrinogen, albumin, and lymphocytes predict postoperative survival in hepatocellular carcinoma. Cancer Med. (2023) 12:13319–28. doi: 10.1002/cam4.6030
25. Guo Q, Zhang B, Dong X, Xie Q, Guo E, Huang H, et al. Elevated levels of plasma fibrinogen in patients with pancreatic cancer: possible role of a distant metastasis predictor. Pancreas. (2009) 38:e75–9. doi: 10.1097/MPA.0b013e3181987d86
26. Chung KH, Lee JC, Lee J, Cho IK, Kim J, Jang W, et al. Serum fibrinogen as a diagnostic and prognostic biomarker for pancreatic ductal adenocarcinoma. Pancreatology. (2020) 20:1465–71. doi: 10.1016/j.pan.2020.06.010
27. Ye XW, Hu HJ, Xiong XZ, Lu J, Cheng NS. The preoperative elevated plasma fibrinogen level is associated with the prognosis of hilar cholangiocarcinoma. Surg Today. (2021) 51:1352–60. doi: 10.1007/s00595-021-02249-x
28. Cao P, Jiang L, Zhou LY, Chen YL. The clinical significance of preoperative serum fibrinogen levels and platelet counts in patients with gallbladder carcinoma. BMC Gastroenterol. (2021) 21:366. doi: 10.1186/s12876-021-01943-x
29. Lu J, Chen S, Li X, Qiu G, He S, Wang H, et al. Gastrointestinal stromal tumors: fibrinogen levels are associated with prognosis of patients as blood-based biomarker. Med (Baltimore). (2018) 97:e0568. doi: 10.1097/md.0000000000010568
30. Troppan KT, Melchardt T, Wenzl K, Schlick K, Deutsch A, Bullock MD, et al. The clinical significance of fibrinogen plasma levels in patients with diffuse large B cell lymphoma. J Clin Pathol. (2016) 69:326–30. doi: 10.1136/jclinpath-2015-203356
31. Zhang Z, Zhang R, Qi J, Miao W, Fang K, Ruan C, et al. The prognostic value of plasma fibrinogen level in patients with acute myeloid leukemia: A systematic review and meta-analysis. Leuk Lymphoma. (2020) 61:2682–91. doi: 10.1080/10428194.2020.1780587
32. Holtzman NG, Xie H, Bentzen S, Kesari V, Bukhari A, El Chaer F, et al. Immune effector cell-associated neurotoxicity syndrome after chimeric antigen receptor T-cell therapy for lymphoma: predictive biomarkers and clinical outcomes. Neuro Oncol. (2021) 23:112–21. doi: 10.1093/neuonc/noaa183
33. Tian Y, Hong M, Jing S, Liu X, Wang H, Wang X, et al. Clinical and prognostic effect of plasma fibrinogen in renal cell carcinoma: A meta-analysis. BioMed Res Int. (2017) 2017:9591506. doi: 10.1155/2017/9591506
34. Liu R, Zhou X, Zou L, Chen Q, Hu Y, Hu J, et al. Clinicopathological and prognostic significance of preoperative plasma fibrinogen level in patients with upper urinary tract urothelial carcinoma: A retrospective tumor marker prognostic study. Int J Surg. (2019) 65:88–93. doi: 10.1016/j.ijsu.2019.03.022
35. Polterauer S, Seebacher V, Hefler-Frischmuth K, Grimm C, Heinze G, Tempfer C, et al. Fibrinogen plasma levels are an independent prognostic parameter in patients with cervical cancer. Am J Obstet Gynecol. (2009) 200:647. doi: 10.1016/j.ajog.2009.01.008
36. Seebacher V, Aust S, D’Andrea D, Grimm C, Reiser E, Tiringer D, et al. Development of a tool for prediction of ovarian cancer in patients with adnexal masses: value of plasma fibrinogen. PloS One. (2017) 12:e0182383. doi: 10.1371/journal.pone.0182383
37. Farzaneh F, Salimnezhad M, Hosseini MS, Ashraf Ganjouee T, Arab M, Talayeh M. D-dimer, fibrinogen and tumor marker levels in patients with benign and Malignant ovarian tumors. Asian Pac J Cancer Prev. (2023) 24:4263–8. doi: 10.31557/apjcp.2023.24.12.4263
38. Hefler-Frischmuth K, Lafleur J, Hefler L, Polterauer S, Seebacher V, Reinthaller A, et al. Plasma fibrinogen levels in patients with benign and Malignant ovarian tumors. Gynecol Oncol. (2015) 136:567–70. doi: 10.1016/j.ygyno.2014.12.041
39. Bekos C, Grimm C, Brodowicz T, Petru E, Hefler L, Reimer D, et al. Prognostic role of plasma fibrinogen in patients with uterine leiomyosarcoma - a multicenter study. Sci Rep. (2017) 7:14474. doi: 10.1038/s41598-017-13934-8
40. Seebacher V, Polterauer S, Grimm C, Husslein H, Leipold H, Hefler-Frischmuth K, et al. The prognostic value of plasma fibrinogen levels in patients with endometrial cancer: A multi-centre trial. Br J Cancer. (2010) 102:952–6. doi: 10.1038/sj.bjc.6605547
41. Wang Y, Yin W, Wang Z, Huang J, Pan J, Zhu Y, et al. Pretreatment plasma fibrinogen as an independent prognostic indicator of prostate cancer patients treated with androgen deprivation therapy. Prostate Cancer Prostatic Dis. (2016) 19:209–15. doi: 10.1038/pcan.2016.6
42. Li X, Shu K, Zhou J, Yu Q, Cui S, Liu J, et al. Preoperative plasma fibrinogen and D-dimer as prognostic biomarkers for non-muscle-invasive bladder cancer. Clin Genitourin Cancer. (2020) 18:11–9.e1. doi: 10.1016/j.clgc.2019.10.025
43. Graf ME, Sookthai D, Johnson T, Schübel R, Maldonado SG, Pletsch-Borba L, et al. Pre-diagnostic plasma concentrations of fibrinogen, sgpiib/iiia, sp-selectin, sthrombomodulin, thrombopoietin in relation to cancer risk: findings from a large prospective study. Int J Cancer. (2018) 143:2659–67. doi: 10.1002/ijc.31623
44. Wang Y, Wang Y, Chen R, Tang Z, Peng Y, Jin Y, et al. Plasma fibrinogen acts as a predictive factor for pathological complete response to neoadjuvant chemotherapy in breast cancer: A retrospective study of 1004 Chinese breast cancer patients. BMC Cancer. (2021) 21:542. doi: 10.1186/s12885-021-08284-8
45. Jianyong L, Zhihui L, Rixiang G, Jingqiang Z. Using a nomogram based on preoperative serum fibrinogen levels to predict recurrence of papillary thyroid carcinoma. BMC Cancer. (2018) 18:390. doi: 10.1186/s12885-018-4296-7
46. Wang PF, Meng Z, Song HW, Yao K, Duan ZJ, Li SW, et al. Higher plasma fibrinogen levels are associated with Malignant phenotype and worse survival in patients with glioblastomas. J Cancer. (2018) 9:2024–9. doi: 10.7150/jca.24714
47. Ghanim B, Hoda MA, Klikovits T, Winter MP, Alimohammadi A, Grusch M, et al. Circulating fibrinogen is a prognostic and predictive biomarker in Malignant pleural mesothelioma. Br J Cancer. (2014) 110:984–90. doi: 10.1038/bjc.2013.815
48. Asanuma K, Matsumine A, Nakamura T, Matsubara T, Asanuma Y, Oi T, et al. Impact of plasma fibrinogen levels in benign and Malignant soft tissue tumors. Cancer biomark. (2016) 16:453–8. doi: 10.3233/cbm-160584
49. Pu Y, Wang J, Wang J, Wang S. Association of preoperative plasma D-dimer and fibrinogen and osteosarcoma outcome. Front Oncol. (2022) 12:699295. doi: 10.3389/fonc.2022.699295
50. Peschek LS, Hobusch GM, Funovics PT, Willegger M, Schmid MP, Amann G, et al. High fibrinogen levels are associated with poor survival in patients with liposarcoma. Sci Rep. (2023) 13:8608. doi: 10.1038/s41598-023-31527-6
51. Nenclares P, Gunn L, Soliman H, Bover M, Trinh A, Leslie I, et al. On-treatment immune prognostic score for patients with relapsed and/or metastatic head and neck squamous cell carcinoma treated with immunotherapy. J Immunother Cancer. (2021) 9(6). doi: 10.1136/jitc-2021-002718
52. Liang YJ, Mei XY, Zeng B, Zhang SE, Yang L, Lao XM, et al. Prognostic role of preoperative D-dimer, fibrinogen and platelet levels in patients with oral squamous cell carcinoma. BMC Cancer. (2021) 21:122. doi: 10.1186/s12885-021-07841-5
53. Mori S, Taki T, Murakami Y, Urata T, Okumura M, Akanabe H, et al. Low plasma fibrinogen levels are associated with poor prognosis in cutaneous angiosarcoma of the head and neck. Cancer Sci. (2021) 112:3924–7. doi: 10.1111/cas.15037
54. Chu T, Wang H, Lv X, Qi J, Tang Y, Fan Y, et al. Investigation of fibrinogen in early bleeding of patients with newly diagnosed acute promyelocytic leukemia. Platelets. (2021) 32:677–83. doi: 10.1080/09537104.2020.1799969
55. Zhang X, Long Q. Elevated serum plasma fibrinogen is associated with advanced tumor stage and poor survival in hepatocellular carcinoma patients. Med (Baltimore). (2017) 96:e6694. doi: 10.1097/md.0000000000006694
56. Dvorak HF. Tumors: wounds that do not heal. Similarities between tumor stroma generation and wound healing. N Engl J Med. (1986) 315:1650–9. doi: 10.1056/nejm198612253152606
57. Rybarczyk BJ, Simpson-Haidaris PJ. Fibrinogen assembly, secretion, and deposition into extracellular matrix by mcf-7 human breast carcinoma cells. Cancer Res. (2000) 60:2033–9.
58. Lee SY, Lee KP, Lim JW. Identification and biosynthesis of fibrinogen in human uterine cervix carcinoma cells. Thromb Haemost. (1996) 75:466–70.
59. Chan JP, Merlini M, Gao HX, Mendiola AS, Akassoglou K, Rubenstein JL, et al. Blood coagulation factor fibrinogen in tumor pathogenesis of central nervous system B-cell lymphoma. Am J Pathol. (2021) 191:575–83. doi: 10.1016/j.ajpath.2020.12.010
60. Sahni A, Arévalo MT, Sahni SK, Simpson-Haidaris PJ. The ve-cadherin binding domain of fibrinogen induces endothelial barrier permeability and enhances transendothelial migration of Malignant breast epithelial cells. Int J Cancer. (2009) 125:577–84. doi: 10.1002/ijc.24340
61. Lip GY, Chin BS, Blann AD. Cancer and the prothrombotic state. Lancet Oncol. (2002) 3:27–34. doi: 10.1016/s1470-2045(01)00619-2
62. Wang C, Wang Y, Zuo N, Fang S, Shi J. Cd44-fibrinogen binding promotes bleeding in acute promyelocytic leukemia by in situ fibrin(Ogen) deposition. Blood Adv. (2022) 6:4617–33. doi: 10.1182/bloodadvances.2022006980
63. Guadiz G, Sporn LA, Simpson-Haidaris PJ. Thrombin cleavage-independent deposition of fibrinogen in extracellular matrices. Blood. (1997) 90:2644–53. doi: 10.1182/blood.V90.7.2644
64. Redman CM, Xia H. Fibrinogen biosynthesis. Assembly, intracellular degradation, and association with lipid synthesis and secretion. Ann N Y Acad Sci. (2001) 936:480–95. doi: 10.1111/j.1749-6632.2001.tb03535.x
65. Zhang W, Gao Z, Zeng G, Xie H, Liu J, Liu N, et al. Clinical significance of urinary plasminogen and fibrinogen gamma chain as novel potential diagnostic markers for non-small-cell lung cancer. Clin Chim Acta. (2020) 502:55–65. doi: 10.1016/j.cca.2019.11.022
66. Liu G, Xu X, Geng H, Li J, Zou S, Li X. Fga inhibits metastases and induces autophagic cell death in gastric cancer via inhibiting itga5 to regulate the fak/erk pathway. Tissue Cell. (2022) 76:101767. doi: 10.1016/j.tice.2022.101767
67. Sharma BK, Mureb D, Murab S, Rosenfeldt L, Francisco B, Cantrell R, et al. Fibrinogen activates focal adhesion kinase (Fak) promoting colorectal adenocarcinoma growth. J Thromb Haemost. (2021) 19:2480–94. doi: 10.1111/jth.15440
68. Adams GN, Rosenfeldt L, Frederick M, Miller W, Waltz D, Kombrinck K, et al. Colon cancer growth and dissemination relies upon thrombin, stromal par-1, and fibrinogen. Cancer Res. (2015) 75:4235–43. doi: 10.1158/0008-5472.Can-15-0964
69. Zhang X, Wang F, Huang Y, Ke K, Zhao B, Chen L, et al. Fgg promotes migration and invasion in hepatocellular carcinoma cells through activating epithelial to mesenchymal transition. Cancer Manag Res. (2019) 11:1653–65. doi: 10.2147/cmar.S188248
70. Masamune A, Kikuta K, Watanabe T, Satoh K, Hirota M, Hamada S, et al. Fibrinogen induces cytokine and collagen production in pancreatic stellate cells. Gut. (2009) 58:550–9. doi: 10.1136/gut.2008.154401
71. Verheul HM, van Erp K, Homs MY, Yoon GS, van der Groep P, Rogers C, et al. The relationship of vascular endothelial growth factor and coagulation factor (Fibrin and fibrinogen) expression in clear cell renal cell carcinoma. Urology. (2010) 75:608–14. doi: 10.1016/j.urology.2009.05.075
72. Chen Y, Zhu Y, Sheng Y, Xiao J, Xiao Y, Cheng N, et al. Sirt1 downregulated fgb expression to inhibit rcc tumorigenesis by destabilizing stat3. Exp Cell Res. (2019) 382:111466. doi: 10.1016/j.yexcr.2019.06.011
73. Uccella S, Cromi A, Vigetti D, Cimetti L, Deleonibus S, Casarin J, et al. Endometrial cancer cells can express fibrinogen: immunohistochemistry and Rt-Pcr analysis. J Obstet Gynaecol. (2016) 36:353–8. doi: 10.3109/01443615.2015.1065231
74. Dzikowski L, Mirzaei R, Sarkar S, Kumar M, Bose P, Bellail A, et al. Fibrinogen in the glioblastoma microenvironment contributes to the invasiveness of brain tumor-initiating cells. Brain Pathol. (2021) 31:e12947. doi: 10.1111/bpa.12947
75. Teitelbaum DH, Tuttle S, Carey LC, Clausen KP. Fibrolamellar carcinoma of the liver. Review of three cases and the presentation of a characteristic set of tumor markers defining this tumor. Ann Surg. (1985) 202:36–41. doi: 10.1097/00000658-198507000-00005
76. Greten FR, Grivennikov SI. Inflammation and cancer: triggers, mechanisms, and consequences. Immunity. (2019) 51:27–41. doi: 10.1016/j.immuni.2019.06.025
77. Cervantes-Villagrana RD, Albores-García D, Cervantes-Villagrana AR, García-Acevez SJ. Tumor-induced neurogenesis and immune evasion as targets of innovative anti-cancer therapies. Signal Transduct Target Ther. (2020) 5:99. doi: 10.1038/s41392-020-0205-z
78. Zhang C, Chen H, He Q, Luo Y, He A, Tao A, et al. Fibrinogen/akt/microfilament axis promotes colitis by enhancing vascular permeability. Cell Mol Gastroenterol Hepatol. (2021) 11:683–96. doi: 10.1016/j.jcmgh.2020.10.007
79. Hahn J, Bressler J, Domingo-Relloso A, Chen MH, McCartney DL, Teumer A, et al. DNA methylation analysis is used to identify novel genetic loci associated with circulating fibrinogen levels in blood. J Thromb Haemost. (2023) 21:1135–47. doi: 10.1016/j.jtha.2023.01.015
80. Tsakadze NL, Zhao Z, D’Souza SE. Interactions of intercellular adhesion molecule-1 with fibrinogen. Trends Cardiovasc Med. (2002) 12:101–8. doi: 10.1016/s1050-1738(01)00157-8
81. Davalos D, Ryu JK, Merlini M, Baeten KM, Le Moan N, Petersen MA, et al. Fibrinogen-induced perivascular microglial clustering is required for the development of axonal damage in neuroinflammation. Nat Commun. (2012) 3:1227. doi: 10.1038/ncomms2230
82. Han CY, Pichon TJ, Wang X, Ringgold KM, St John AE, Stern SA, et al. Leukocyte activation primes fibrinogen for proteolysis by mitochondrial oxidative stress. Redox Biol. (2022) 51:102263. doi: 10.1016/j.redox.2022.102263
83. Millien VO, Lu W, Shaw J, Yuan X, Mak G, Roberts L, et al. Cleavage of fibrinogen by proteinases elicits allergic responses through toll-like receptor 4. Science. (2013) 341:792–6. doi: 10.1126/science.1240342
84. Jensen T, Kierulf P, Sandset PM, Klingenberg O, Joø GB, Godal HC, et al. Fibrinogen and fibrin induce synthesis of proinflammatory cytokines from isolated peripheral blood mononuclear cells. Thromb Haemost. (2007) 97:822–9. doi: 10.1160/th07-01-0039
85. Saraiva AL, Peres RS, Veras FP, Talbot J, de Lima KA, Luiz JPM, et al. Citrullinated human fibrinogen triggers arthritis through an inflammatory response mediated by il-23/il-17 immune axis. Int Immunopharmacol. (2021) 101:108363. doi: 10.1016/j.intimp.2021.108363
86. Smiley ST, King JA, Hancock WW. Fibrinogen stimulates macrophage chemokine secretion through toll-like receptor 4. J Immunol. (2001) 167:2887–94. doi: 10.4049/jimmunol.167.5.2887
87. Guo M, Sahni SK, Sahni A, Francis CW. Fibrinogen regulates the expression of inflammatory chemokines through nf-kappab activation of endothelial cells. Thromb Haemost. (2004) 92:858–66. doi: 10.1160/th04-04-0261
88. Sulimai N, Brown J, Lominadze D. The role of nuclear factor-kappa B in fibrinogen-induced inflammatory responses in cultured primary neurons. Biomolecules. (2022) 12(12). doi: 10.3390/biom12121741
89. Alouffi S, Khanam A, Husain A, Akasha R, Rabbani G, Ahmad S. D-ribose-mediated glycation of fibrinogen: role in the induction of adaptive immune response. Chem Biol Interact. (2022) 367:110147. doi: 10.1016/j.cbi.2022.110147
90. Poole LG, Kopec AK, Flick MJ, Luyendyk JP. Cross-linking by tissue transglutaminase-2 alters fibrinogen-directed macrophage proinflammatory activity. J Thromb Haemost. (2022) 20:1182–92. doi: 10.1111/jth.15670
91. Kim JW, Jeong MH, Yu HT, Park YJ, Kim HS, Chung KH. Fibrinogen on extracellular vesicles derived from polyhexamethylene guanidine phosphate-exposed mice induces inflammatory effects via integrin B. Ecotoxicol Environ Saf. (2023) 252:114600. doi: 10.1016/j.ecoenv.2023.114600
92. Flick MJ, Du X, Witte DP, Jirousková M, Soloviev DA, Busuttil SJ, et al. Leukocyte engagement of fibrin(Ogen) via the integrin receptor Alphambeta2/Mac-1 is critical for host inflammatory response in vivo. J Clin Invest. (2004) 113:1596–606. doi: 10.1172/jci20741
93. Wright SD, Weitz JI, Huang AJ, Levin SM, Silverstein SC, Loike JD. Complement receptor type three (Cd11b/Cd18) of human polymorphonuclear leukocytes recognizes fibrinogen. Proc Natl Acad Sci U.S.A. (1988) 85:7734–8. doi: 10.1073/pnas.85.20.7734
94. Rubel C, Fernández GC, Rosa FA, Gómez S, Bompadre MB, Coso OA, et al. Soluble fibrinogen modulates neutrophil functionality through the activation of an extracellular signal-regulated kinase-dependent pathway. J Immunol. (2002) 168:3527–35. doi: 10.4049/jimmunol.168.7.3527
95. Ryu JK, Petersen MA, Murray SG, Baeten KM, Meyer-Franke A, Chan JP, et al. Blood coagulation protein fibrinogen promotes autoimmunity and demyelination via chemokine release and antigen presentation. Nat Commun. (2015) 6:8164. doi: 10.1038/ncomms9164
96. Steinbrecher KA, Horowitz NA, Blevins EA, Barney KA, Shaw MA, Harmel-Laws E, et al. Colitis-associated cancer is dependent on the interplay between the hemostatic and inflammatory systems and supported by integrin Alpha(M)Beta(2) engagement of fibrinogen. Cancer Res. (2010) 70:2634–43. doi: 10.1158/0008-5472.Can-09-3465
97. Wichaiyo S, Svasti S, Supharattanasitthi W, Morales NP. Dasatinib induces loss of vascular integrity and promotes cutaneous wound repair in mice. J Thromb Haemost. (2021) 19:3154–67. doi: 10.1111/jth.15499
98. Yang W, Feng J, Xiang F, Xie Z, Zhang G, Sabatier JM, et al. Endogenous animal toxin-like human B-defensin 2 inhibits own K(+) channels through interaction with channel extracellular pore region. Cell Mol Life Sci. (2015) 72:845–53. doi: 10.1007/s00018-014-1715-z
99. Sahni A, Khorana AA, Baggs RB, Peng H, Francis CW. Fgf-2 binding to fibrin(Ogen) is required for augmented angiogenesis. Blood. (2006) 107:126–31. doi: 10.1182/blood-2005-06-2460
100. Sahni A, Simpson-Haidaris PJ, Sahni SK, Vaday GG, Francis CW. Fibrinogen synthesized by cancer cells augments the proliferative effect of fibroblast growth factor-2 (Fgf-2). J Thromb Haemost. (2008) 6:176–83. doi: 10.1111/j.1538-7836.2007.02808.x
101. Sahni A, Francis CW. Stimulation of endothelial cell proliferation by Fgf-2 in the presence of fibrinogen requires alphavbeta3. Blood. (2004) 104:3635–41. doi: 10.1182/blood-2004-04-1358
102. Maciel J, Oliveira MI, Colton E, McNally AK, Oliveira C, Anderson JM, et al. Adsorbed fibrinogen enhances production of bone- and angiogenic-related factors by monocytes/macrophages. Tissue Eng Part A. (2014) 20:250–63. doi: 10.1089/ten.TEA.2012.0439
103. Tang H, Fu Y, Lei Q, Han Q, Ploplis VA, Castellino FJ, et al. Fibrinogen facilitates the anti-tumor effect of nonnative endostatin. Biochem Biophys Res Commun. (2009) 380:249–53. doi: 10.1016/j.bbrc.2009.01.045
104. Li H, Cai E, Cheng H, Ye X, Ma R, Zhu H, et al. Fga controls vegfa secretion to promote angiogenesis by activating the vegfr2-fak signalling pathway. Front Endocrinol (Lausanne). (2022) 13:791860. doi: 10.3389/fendo.2022.791860
105. Thiagarajan P, Rippon AJ, Farrell DH. Alternative adhesion sites in human fibrinogen for vascular endothelial cells. Biochemistry. (1996) 35:4169–75. doi: 10.1021/bi952532b
106. Krajewska E, Lewis CE, Chen YY, Welford A, Tazzyman S, Staton CA. A novel fragment derived from the beta chain of human fibrinogen, beta43-63, is a potent inhibitor of activated endothelial cells in vitro and in vivo. Br J Cancer. (2010) 102:594–601. doi: 10.1038/sj.bjc.6605495
107. Akakura N, Hoogland C, Takada YK, Saegusa J, Ye X, Liu FT, et al. The cooh-terminal globular domain of fibrinogen gamma chain suppresses angiogenesis and tumor growth. Cancer Res. (2006) 66:9691–7. doi: 10.1158/0008-5472.Can-06-1686
108. Staton CA, Lewis CE. Angiogenesis inhibitors found within the haemostasis pathway. J Cell Mol Med. (2005) 9:286–302. doi: 10.1111/j.1582-4934.2005.tb00356.x
109. Guo SW, Che HM, Li WZ. Anti-tumor effect of lentivirus-mediated gene transfer of alphastatin on human glioma. Cancer Sci. (2011) 102:1038–44. doi: 10.1111/j.1349-7006.2011.01879.x
110. Staton CA, Brown NJ, Rodgers GR, Corke KP, Tazzyman S, Underwood JC, et al. Alphastatin, a 24-amino acid fragment of human fibrinogen, is a potent new inhibitor of activated endothelial cells in vitro and in vivo. Blood. (2004) 103:601–6. doi: 10.1182/blood-2003-07-2192
111. Liang C, Wei T, Zhang T, Niu C. Adipose−Derived stem cell−Mediated alphastatin targeting delivery system inhibits angiogenesis and tumor growth in glioma. Mol Med Rep. (2023) 28(5). doi: 10.3892/mmr.2023.13102
112. Chahed K, Kabbage M, Ehret-Sabatier L, Lemaitre-Guillier C, Remadi S, Hoebeke J, et al. Expression of fibrinogen E-fragment and fibrin E-fragment is inhibited in the human infiltrating ductal carcinoma of the breast: the two-dimensional electrophoresis and maldi-tof-mass spectrometry analyses. Int J Oncol. (2005) 27:1425–31. doi: 10.3892/ijo
113. Palumbo JS, Potter JM, Kaplan LS, Talmage K, Jackson DG, Degen JL. Spontaneous hematogenous and lymphatic metastasis, but not primary tumor growth or angiogenesis, is diminished in fibrinogen-deficient mice. Cancer Res. (2002) 62:6966–72.
114. Heinolainen K, Karaman S, D’Amico G, Tammela T, Sormunen R, Eklund L, et al. Vegfr3 modulates vascular permeability by controlling vegf/vegfr2 signaling. Circ Res. (2017) 120:1414–25. doi: 10.1161/circresaha.116.310477
115. Lavergne M, Janus-Bell E, Schaff M, Gachet C, Mangin PH. Platelet integrins in tumor metastasis: do they represent a therapeutic target? Cancers (Basel). (2017) 9(10). doi: 10.3390/cancers9100133
116. Huang J, Li X, Shi X, Zhu M, Wang J, Huang S, et al. Platelet integrin Aiibβ3: signal transduction, regulation, and its therapeutic targeting. J Hematol Oncol. (2019) 12:26. doi: 10.1186/s13045-019-0709-6
117. Schlesinger M. Role of platelets and platelet receptors in cancer metastasis. J Hematol Oncol. (2018) 11:125. doi: 10.1186/s13045-018-0669-2
118. Palumbo JS, Talmage KE, Massari JV, La Jeunesse CM, Flick MJ, Kombrinck KW, et al. Platelets and fibrin(Ogen) increase metastatic potential by impeding natural killer cell-mediated elimination of tumor cells. Blood. (2005) 105:178–85. doi: 10.1182/blood-2004-06-2272
119. Zheng S, Shen J, Jiao Y, Liu Y, Zhang C, Wei M, et al. Platelets and fibrinogen facilitate each other in protecting tumor cells from natural killer cytotoxicity. Cancer Sci. (2009) 100:859–65. doi: 10.1111/j.1349-7006.2009.01115.x
120. Simpson-Haidaris PJ, Rybarczyk B. Tumors and fibrinogen. The role of fibrinogen as an extracellular matrix protein. Ann N Y Acad Sci. (2001) 936:406–25. doi: 10.1111/j.1749-6632.2001.tb03525.x
121. Angelidakis E, Chen S, Zhang S, Wan Z, Kamm RD, Shelton SE. Impact of fibrinogen, fibrin thrombi, and thrombin on cancer cell extravasation using in vitro microvascular networks. Adv Healthc Mater. (2023) 12:e2202984. doi: 10.1002/adhm.202202984
122. Han Y, Tomita T, Kato M, Ashihara N, Higuchi Y, Matoba H, et al. Citrullinated fibrinogen-saas complex causes vascular metastagenesis. Nat Commun. (2023) 14:4960. doi: 10.1038/s41467-023-40371-1
123. Camerer E, Qazi AA, Duong DN, Cornelissen I, Advincula R, Coughlin SR. Platelets, protease-activated receptors, and fibrinogen in hematogenous metastasis. Blood. (2004) 104:397–401. doi: 10.1182/blood-2004-02-0434
124. Palumbo JS, Kombrinck KW, Drew AF, Grimes TS, Kiser JH, Degen JL, et al. Fibrinogen is an important determinant of the metastatic potential of circulating tumor cells. Blood. (2000) 96:3302–9. doi: 10.1182/blood.V96.10.3302.h8003302_3302_3309
125. Wang M, Zhang G, Zhang Y, Cui X, Wang S, Gao S, et al. Fibrinogen alpha chain knockout promotes tumor growth and metastasis through integrin-akt signaling pathway in lung cancer. Mol Cancer Res. (2020) 18:943–54. doi: 10.1158/1541-7786.Mcr-19-1033
126. Schneider G, Bryndza E, Poniewierska-Baran A, Serwin K, Suszynska M, Sellers ZP, et al. Evidence that vitronectin is a potent migration-enhancing factor for cancer cells chaperoned by fibrinogen: A novel view of the metastasis of cancer cells to low-fibrinogen lymphatics and body cavities. Oncotarget. (2016) 7:69829–43. doi: 10.18632/oncotarget.12003
127. Qian X, Cai J, Qi Q, Han J, Zhu X, Xia R, et al. Preoperative fibrinogen is associated with the clinical survival of primary liver cancer patients and promotes hepatoma metastasis via the pten/akt/mtor pathway. Heliyon. (2023) 9:e16696. doi: 10.1016/j.heliyon.2023.e16696
128. Westra J, Bijzet J, Doornbos-van der Meer B, van Rijswijk MH, Limburg PC. Differential influence of P38 mitogen activated protein kinase (Mapk) inhibition on acute phase protein synthesis in human hepatoma cell lines. Ann Rheum Dis. (2006) 65:929–35. doi: 10.1136/ard.2005.043232
129. Chen L, Zhang C, Xue R, Liu M, Bai J, Bao J, et al. Deep whole-genome analysis of 494 hepatocellular carcinomas. Nature. (2024) 627(8004):586–93. doi: 10.1038/s41586-024-07054-3
130. Li P, Xiao L, Li YY, Chen X, Xiao CX, Liu JJ, et al. Fibrinogen alpha chain acts as a hbsag binding protein and their interaction promotes hepg2 cell apoptosis. Curr Proteomics. (2014) 11(1). doi: 10.2174/1570164611666140412003740
131. Jiang C, Li Y, Li Y, Liu L, Wang XA, Wu W, et al. Fibrinogen promotes gallbladder cancer cell metastasis and extravasation by inducing icam1 expression. Med Oncol. (2022) 40:10. doi: 10.1007/s12032-022-01874-x
132. Shu YJ, Weng H, Bao RF, Wu XS, Ding Q, Cao Y, et al. Clinical and prognostic significance of preoperative plasma hyperfibrinogenemia in gallbladder cancer patients following surgical resection: A retrospective and in vitro study. BMC Cancer. (2014) 14:566. doi: 10.1186/1471-2407-14-566
133. Li M, Wang J, Wang C, Xia L, Xu J, Xie X, et al. Microenvironment remodeled by tumor and stromal cells elevates fibroblast-derived col1a1 and facilitates ovarian cancer metastasis. Exp Cell Res. (2020) 394:112153. doi: 10.1016/j.yexcr.2020.112153
134. Li C, Qiu S, Liu X, Guo F, Zhai J, Li Z, et al. Extracellular matrix-derived mechanical force governs breast cancer cell stemness and quiescence transition through integrin-ddr signaling. Signal Transduct Target Ther. (2023) 8:247. doi: 10.1038/s41392-023-01453-0
135. Lapitz A, Azkargorta M, Milkiewicz P, Olaizola P, Zhuravleva E, Grimsrud MM, et al. Liquid biopsy-based protein biomarkers for risk prediction, early diagnosis, and prognostication of cholangiocarcinoma. J Hepatol. (2023) 79:93–108. doi: 10.1016/j.jhep.2023.02.027
136. Chu HW, Chang KP, Hsu CW, Chang IY, Liu HP, Chen YT, et al. Identification of salivary biomarkers for oral cancer detection with untargeted and targeted quantitative proteomics approaches. Mol Cell Proteomics. (2019) 18:1796–806. doi: 10.1074/mcp.RA119.001530
137. Zhong J. Fibrinogen-to-albumin ratios are related to mortality in patients with secondary Malignant neoplasm of bone and bone marrow. Heliyon. (2023) 9:e18797. doi: 10.1016/j.heliyon.2023.e18797
138. Li B, Deng H, Lei B, Chen L, Zhang X, Sha D. The prognostic value of fibrinogen to albumin ratio in Malignant tumor patients: A meta-analysis. Front Oncol. (2022) 12:985377. doi: 10.3389/fonc.2022.985377
Keywords: fibrinogen, tumor, pro-inflammatory, angiogenesis, metastasis, molecular pathway
Citation: Wu X, Yu X, Chen C, Chen C, Wang Y, Su D and Zhu L (2024) Fibrinogen and tumors. Front. Oncol. 14:1393599. doi: 10.3389/fonc.2024.1393599
Received: 29 February 2024; Accepted: 25 April 2024;
Published: 08 May 2024.
Edited by:
Robson Q. Monteiro, Federal University of Rio de Janeiro, BrazilReviewed by:
Haibo Yu, Zhengzhou University, ChinaAnca Maria Cimpean, Victor Babes University of Medicine and Pharmacy, Romania
Copyright © 2024 Wu, Yu, Chen, Chen, Wang, Su and Zhu. This is an open-access article distributed under the terms of the Creative Commons Attribution License (CC BY). The use, distribution or reproduction in other forums is permitted, provided the original author(s) and the copyright owner(s) are credited and that the original publication in this journal is cited, in accordance with accepted academic practice. No use, distribution or reproduction is permitted which does not comply with these terms.
*Correspondence: Liqing Zhu, wzzhuliqing@126.com