- 1Department of Radiotherapy, Centre François-Baclesse, Caen, France
- 2Corpuscular Physics Laboratory, IN2P3, Ensicaen, CNRS UMR 6534, Caen, France
- 3Faculté de Médecine de Caen, Université de Normandie, Caen, France
- 4Department of Otorhinolaryngology - Head and Neck Surgery, University Hospital of Poitiers, Poitiers, France
- 5Institut Curie, PSL Research University, University Paris Saclay, Inserm LITO, Orsay, France
- 6Gordon Center for Medical Imaging, Massachusetts General Hospital, Harvard Medical School, Boston, MA, United States
- 7Surgical Oncology Department, Centre Léon Bérard, UNICANCER, Lyon, France
- 8Inserm, U1296 Unit, “Radiation: Defense, Health and Environment”, Centre Léon Bérard, Lyon, France
- 9Polyclinics ELSAN Group, Department of Radiodiagnostics and Interventional Imaging, PolyClinics Les Fleurs, Ollioules, France
- 10Radiotherapy Department, S. Croce & Carle Teaching Hospital, Cuneo, Italy
- 11Radioophysics, Centre Lacassagne, Nice, France
- 12Departement of Maxillofacial Surgery, University Hospital of Amiens Picardy, Research Unit, UR7516 CHIMERE, University of Picardy Jules Verne, Institut Faire Faces, Amiens, France
Background: Major advances have been made in reconstructive surgery in the last decades to reduce morbidity in head and neck cancer. Flaps are now present in 80% of patients with oral cavity cancer to cover anatomic, functional, and cosmetic needs. However, gaps in interdisciplinary innovation transfer from surgery to postoperative radiotherapy (poRT) remain challenging. We aimed to provide an interdisciplinary view of the challenges encountered by radiation oncologists in planning head and neck postoperative radiotherapy.
Methods: A systematic and critical review was conducted to address areas of optimization in surgery and radiology that may be relevant to poRT.
Results: Despite extensive surgical literature on flap techniques and salvage surgery, 13 retrospective series were identified, where flap outcomes were indirectly compared between surgery alone or poRT. These low-evidence studies suggest that radiotherapy accelerates flap atrophy, fibrosis, and osteoradionecrosis and deteriorates functional outcomes. Preliminary evidence suggests that tumor spread occurs at the flap–tissue junction rather than in the flaps. One prospective 15-patient study showed 31.3% vs. 39.2% flap volume reduction without or with poRT. In an international consensus, experts recognized the needs for optimized flap-sparing poRT against flap-related functional deterioration and bone damage. CT, MRI, and PET-CT modalities show potential for the delineation of the junction area between native tissues and flap for flap segmentation and to characterize flap-specific changes quantitatively and correlate them with patterns of relapse or complications.
Conclusion: Flap management in poRT is insufficiently documented, but poRT seems to damage flaps. Current gaps in knowledge underscore the need for prospective flap assessment and interdisciplinary trials investigating flap morbidity minimization by flap-sparing poRT planning.
1 Introduction
In the last two decades, substantial improvements have been made in surgery, radiotherapy, systemic treatments, and supportive care of head and neck cancer (HNC). Over 75% of HNC patients have advanced stages at diagnosis and elect for combined treatments (1). Often inevitable to maximize the chance of cure, combined treatments are also responsible for cumulative late toxicities (2). Surgery is the mainstay of treatment, with radiotherapy advocated in about 60% of patients in the definitive (chemo)radiotherapy or postoperative (poRT) setting (3). Primary tumor resection is increasingly performed using reconstructive surgery and mini-invasive/mini-morbid approaches, with de-escalation when appropriate (4, 5). Reconstructive surgery aims to mitigate the effects of tumor resection and is standard practice in many cancer sites (6) to restore the anatomy and function. Flaps, i.e., vascularized autologous tissues repairing a tissue defect or grafts protecting or sustaining organs (7) and dental implants, fixating plates, and prosthetic materials, are frequent practice, yet interdisciplinary transfer of high-end technology suffers from gaps in knowledge at interfaces between disciplines. The choice of the reconstructive technique and materials depends on patient comorbidities, tumor bulk and site, and surgical expertise (8–10). Reconstructive surgery is no longer limited to surgical salvage in case of tumor relapse in irradiated tissues with a flap to protect the carotid artery from salivary leakage.
As a result, flaps are now present in over 80% in oral cavity cancer poRT series and 50% of all tumor sites (11, 12). Flaps can allow the anticipation of more generous margins (13, 14). The next challenge is high-end surgical technology transfer to the poRT planning to refine target volumes in the presence of a flap to retain full functionality. This critical review addresses the multidisciplinary issues of the management of flaps in HNC poRT planning and poRT outcomes.
2 Search strategy and selection criteria
PubMed was searched using the terms: (flap[Title]) AND (radiotherapy[Title]) for the 2008–2023 period. Free flaps do not hold the same radiotherapy planning challenges as local flaps on one hand or pedicled flaps on the other hand; all flaps were therefore included. The initial sifts focused on HNC. Further eligibility criteria excluded articles of salvage reconstructive surgery in irradiated areas and case reports (Supplementary Figure S1: flowchart). This search process was extended to comprehensively address aspects pertaining to flap reconstruction and poRT in HNC with articles sourced from the reference lists of the primary articles. It enabled us to cover surgical techniques, imaging strategies, and radiation toxicity. The final set of articles was reviewed, providing a comprehensive perspective on the complex interplay between flap reconstruction and poRT in HNC patients.
3 Surgical management of flaps
3.1 Flap reconstructive surgery
Primary closure and spontaneous healing may be sufficient for small tumors. In many other cases, curative resection requires a wide excision to obtain safe margins and for the subsequent functional repair of head and neck anatomy. Grafts are mostly made of non-vascularized fascia or skin and exert a mechanical role rather than restore a function. They can cover variably large surfaces of defect, such as the fascia lata graft to protect the dura during sinonasal surgery. Made of autologous tissues having their own vascular supply, flaps are often more appropriate than grafts to fulfil the functional needs associated with a volumetric substance loss (15). Flaps are transposed geometrically with their blood supply to the tissue resection site, the “tumor bed”, from a loco-regional non-tumoral area. Exploiting advances in microvascular surgery, free flaps are harvested from distant donor body parts, with vessels micro-anastomosed between the donor and recipient sites. Such flaps are expected to be versatile to contribute to vital functions with high fidelity. Chimeric flaps associate soft and bony tissues. In bone flaps, hardware, i.e., plaques and screws made of metal (or artefact-free carbon fiber), fix the bone flap to the native bone anatomy (16). In uncertain cases, however, reconstruction may be delayed after definitive negative margins are obtained. Anticipating flap reconstruction may allow for larger resections and may improve local control and progression-free survival (17). It also allows optimal flap choice based on the estimates of extent of the tissue defect clinically and by conventional preoperative imaging using CT or MRI of the head and neck. Preoperative assessments of comorbidities and vascular supply quality are also needed (18).
3.2 Flap types
The types of flaps include: (a) the local flap, with geometric reshaping/positioning to adjacent tissue; (b) the regional flap, with rotation of the flap vascular system, and (c) the distant free flap with transfer of microanastomosed tissue. Flaps can consist of one tissue type, such as cutaneous flaps, or, more frequently, several tissue types such as myocutaneous, fasciocutaneous, and bone flaps; the latter also often have a soft tissue part. Flaps vary depending on the complexity of the defect (19). The native tissue is not necessarily replaced by the same tissue, and the native mucosae is often substituted for skin from the donor site. Flap reconstruction is continuously evolving toward the use of more versatile flaps (agile to comply with functional requirements), including composite/chimeric (made of several tissue types), multiple (made of different flaps such as, for example, the fibula flap and the anterolateral thigh flap, transplanted sequentially during the same surgery, to both get bone and enough skin volume at the recipient site), and “free style” (highly customized operator dependent) flaps to cover unusual needs. Technical advances and new flaps are variably integrated by surgical teams in time.
3.3 Basic principles of flap selection
When the primary closure of a relatively small defect is not feasible and surgical margins are negative, local flap reconstruction is usually the preferred procedure. The benefits include ease of harvest, short operating room time with little patient morbidity constraints, short hospital length of stay, and low morbidity at the donor site. The drawbacks include limited size, risk for partial necrosis, or vascular pedicle damage during tumor resection and wound dehiscence. The facial artery myomucosal (FAMM) and buccinator local flaps can, as an example, reconstruct a small floor of mouth tumor.
Free flaps can be larger than local flaps and are more versatile. A free flap is chosen based on the size of the surgical defect and donor tissue bulk availability, need for bone or soft tissue only, thickness, and pliability along with mobility of the restored organ (such as the tongue). Surgical oncology with free flap reconstruction requires successive operative steps, e.g., tumor resection, flap harvesting at the donor site, flap transplantation to the recipient site, and therefore prolonged general anesthesia, which may be contraindicated in case of severe comorbidities. Morbidity of harvesting at the donor site is also considered, and feasibility of vascular microsurgery is dependent on the quality of both recipient and donor arteries, which may be inadequate (atherosclerosis) or depleted (previous local treatments). Common free flaps include (but are not limited to) the radial forearm, anterior lateral thigh, fibula, iliac, scapula, latissimus dorsi (in particular when team expertise with perforator flaps is limited), and jejunal free flaps (Table 1). The radial forearm flap, for example, may be used for reconstructions of the oral cavity, tongue, palate, nose, face, scalp, lip, and pharynx (20) (Figure 1). The anterolateral thigh flap can also be used for larger and thicker substance loss. The fibula flap may be used to reconstruct the upper maxillary or mandibular bone (20). Osteotomies can be prepared preoperatively with 3D planning software and cutting guides, while soft tissue components are usually shaped peri-operatively. The jejunal flap may be used for circumferential pharyngeal and esophageal defects (20). When local flaps do not adequately fulfill the needs and free flaps are not feasible, pedicle flaps, such as the pectoralis major flap, may be used. This is particularly the case in patients with severe comorbidities or after a first flap failure.
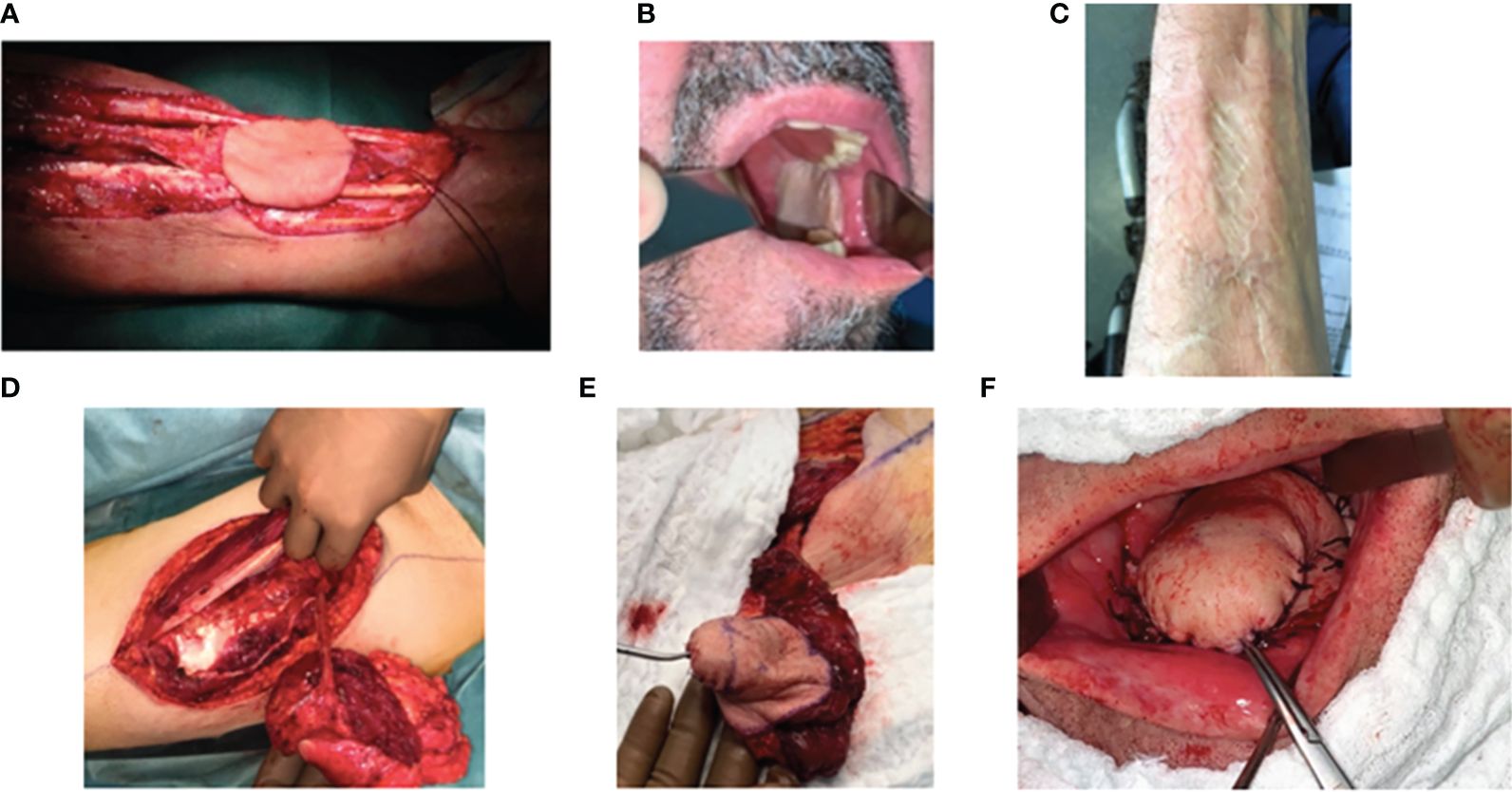
Figure 1 Surgical harvest and use of radial forearm and anterolateral thigh free flaps for reconstructive surgery. (A, B) Radial forearm free flap for the reconstruction of a retromolar trigone tumor. (A) Harvesting of the flap pedicle from the radial artery and vein, (B) flap positioning in the oral cavity, and (C) donor site. (D–F) Anterolateral thigh flap for the reconstruction of a mobile tongue tumor. (D) Harvesting of the flap pedicle on the peroneal artery, (E) flap during the modeling step, and (F) flap positioned in the oral cavity.
Although there is no consensual algorithm for the choice of a flap among many flaps (Table 1), the features that should constitute an ideal flap for head and neck soft tissue reconstruction may be summarized as follows: (1) the tissue harvested should be pliable so as to not impair movement and function in its recipient position in the head and neck, (2) the pedicle should be long, large, and consistent between source and recipient, (3) small and large flaps with variable thickness should be possible, (4) harvesting the flap and resecting the tumor should be feasible in the same position (usually supine), (5) the donor site morbidity should be low, both in terms of function and cosmesis, and (6) there should be sensitive nerves available with limited morbidity (21)—for example, the radial forearm was defined in the 1980s and is commonly used but may become less popular due to aesthetic donor site morbidity. For that reason, and for its larger size and versatility, the anterolateral thigh flap is also common. Perforator flaps are used since the 2000s and may be considered for their rich vascular supply. In bone reconstruction, the fibular flap is often considered to reconstruct mandibular defects (22). One might also consider the scapula in case of atherosclerosis of the inferior limbs or the iliac flap for its favorable curvature and shape of the bony part as well as muscular bulk to fill cavities to provide intraoral lining (23, 24).
It is beyond the scope of this article to standardize the choice between flap types (25) and perioperative regimens or the reporting of complications of patients undergoing free flap reconstructive surgery (26–28).
3.4 Vascular supply of flaps
The vascular supply of flaps originates directly from the native tissue artery in case of local (island artery) and regional/pedicled (axial artery) flaps. Free flaps rely on a transected reconnected source/recipient artery and vein. In the case of perforating flaps, harvesting includes a time of dissection of the perforating vessels from donor tissues, such as muscle, which will thus be left in place, preserving the function of the donor site. Perforating vessels originate in the source axial vessels, pass through tissues, besides interstitial connective tissue and fat, and allow the flap vascularization and thus shaping (such as thinning to only use the fatty and skin components of a thigh flap, for example) (29). The vascularization of the flap becomes progressively autonomous from that provided by its pedicle thanks to the angiogenesis of distal vascular branches formed from the surrounding recipient tissue.
3.5 Toward high-fidelity flap reconstruction
Reconstruction surgery is in constant progress to improve flap management and aesthetic/functional outcomes.
3.5.1 Flap innervation
While flap vascularization is critical to flap vitality, flap innervation has pros and cons (30, 31). Motor nerve innervation (conservation or transection–reconnection) could limit muscle atrophy (further to fatty involution of inactive muscle) but should avoid dysesthestic and uncomfortable aberrant contractions (32). Therefore, the nerves of pedicled flaps (such as the latissimus dorsi) are often transected (33), and nerve graft is rarely performed in free flaps. Sensitive innervation should achieve superior sensitive recovery in innervated than non-innervated flaps, which may translate in better swallowing and speech function (34) and better quality of life (29). It has also been suggested that bone flap innervation might promote bone regeneration and turnover and subsequently less bone resorption (35). The assessment of the benefits of sensitive innervation warrants further matched or randomized studies.
3.5.2 Overcompensation of soft tissue flap volume
Anticipating spontaneous flap atrophy over time, it is common surgical practice to overestimate the flap volume 1.6 times larger than the intraoperative defect resulting from the tumor resection (36). In the short term, patients are informed that they may experience transient difficulties swallowing and speaking in the first 6 months. Overcompensation of flap volume 20%–30% larger than the actual defects is often intuitively and empirically (with little inter-surgeons’ reproducibility) performed, yet with limited tools to predict ultimate flap volume (36). Some surgeons recommend to maintain body weight (37, 38) and use higher fat-to-muscle or no muscle (such as perforator flaps) (37–39). Skin atrophy is another possible evolution of flaps. Overcompensation is even more common in anticipation of poRT and has significant implications for poRT planning (see Section 5).
3.5.3 Bone flap shaping
Functional restoration following segmental mandibulectomy or maxillectomy remains challenging despite substantial technology advances. Instead of a bone flap, a soft tissue flap is occasionally associated with a reconstruction plate in patients unfit for bone reconstruction (particularly for anterior lesions). In a meta-analysis of 2,379 patients undergoing reconstruction using a soft tissue flap with a plate, the risk of plate fracture was 5% and of extrusion 20%, while after bone reconstruction, the risk of extrusion was 10%. Bone reconstruction is also expected to be more functional (40). Bone flaps may rely on the use of bone, soft tissues, and a metallic (usually titanium) reconstruction bridging plate. However, plate exposure, osteitis, and osteoradionecrosis often exceed 30% in the long term; alternate options to fixation plates have been evaluated. Miniplates may reduce the risks and be more appropriate for patients who need poRT (41), yet in anticipation of poRT planning and complications, unmet needs remain in the engineering of plates with non-metallic carbon fiber materials that would support hard mechanical constraints and avoid delineation and dose uncertainties.
High-end technology in the field of bone reconstruction is currently dominated by the purpose of high-fidelity bone shaping. Virtual surgical planning, 3D-printed osteotomy guides, and preoperatively bent or custom-milled mandibular reconstruction plates have been shown to ease intraoperative decision making and reduce operative time. With such a purpose in mind, the selection criteria for bone flaps include the possibility of multiple osteotomies while keeping in mind to not impair vascularization, to resist mechanical constraints, to harbor dental implants. The early promising results of such technologies should be confirmed in the mid/long term (42)—for example, the high number of osteotomies of hyperconformal mandibular reconstruction could, in theory, be associated with a substantial damage to the periosteum, a factor that might result in frail vascularization and a higher risk of osteoradionecrosis.
3.5.4 Dental rehabilitation
Common maxillary and mandibular composite free flaps may be used for dental implants (43). A systematic review of 2,626 implants placed into fibula, iliac crest, scapula, and radial forearm free flaps revealed a pooled 5-year survival rate of 94% of implants in the fibula and iliac crest. Factors affecting dental implant survival included implantation after poRT (HR 2.3, 95% CI: 1.2–4.6, P = 0.027). Immediate dental implantation has been advocated to promote more efficient rehabilitation, better patient acceptance, and aesthetics. According to a meta-analysis including randomized trials, immediate loading may, however, result in a higher incidence of implant failure compared to delayed loading (43). Immediate dental implants do not seem to increase the risks of osteonecrosis and osteoradionecrosis. However, case selection and publication biases seem to exist and should address the total number of immediate dental implants over the total number of HNC patients requiring dental implants with bone reconstruction. Another recent meta-analysis suggested that implants should be placed in the native bone rather than in bone grafts in case of poRT (44). Controversial issues remain in dental rehabilitation in the context of radiotherapy.
4 Flap changes and their clinical and radiological assessment
Flaps may be distinguished on imaging from native tissues based on their specific tissue components and anatomic asymmetry.
4.1 Imaging characteristics
Figure 2 illustrates the CT aspect of five common flaps. Myocutaneous (muscle, skin, and fat), fasciocutaneous (fascia, skin, and fat), mucosal (mucosa), visceral (viscera and fat), or bone (bone and soft tissues) flap components exhibit distinct features on imaging (45, 46). On CT, the fatty portion of a flap is hypodense in the [-140; -40] Hounsfield unit (HU) range. On MRI, fat tissues have a short relaxation time and T1/T2 hypersignal, which can hide tumor enhancement in T1 or be mistaken for edematous hypersignal in T2, suggesting the use of fat signal suppression (STIR) gadolinium enhancement T1-weighted sequences. With time, fat can show atrophy or slight augmentation/edema. On CT, the muscular flap component is usually striated, isodense to native muscle and relatively flat. On MR, its signal and enhancement pattern is moderate to intense (15, 47, 48). In the early postoperative period, the flap muscle may be edematous, T1-hypointense, T2-hyperintense, and diffusely T1 gadolinium-enhanced. Later, denervated muscles (such as in myocutaneous flaps) are susceptible to volume loss and fatty replacement of muscle (15, 47, 49, 50), with heterogeneous T1/T2 signal and CT hypodensity. The skin usually exhibits enhancement in fasciocutaneous flaps. These are less susceptible to atrophy (being without muscle) but more to skin fibrosis. Mucosa in flaps usually presents like native mucosa. Local mucosal (such as buccinator) and visceral (such as jejunum) flaps exhibit normally enhanced mucosa, with mesenteric fat carrying donor vessels and lymphatic nodes on CT. Some bone flaps may be distinguished from the native bone by shape and a higher corticated portion. Baseline postoperative CT assessment at 3 months should be proposed for later recurrence assessment (46).
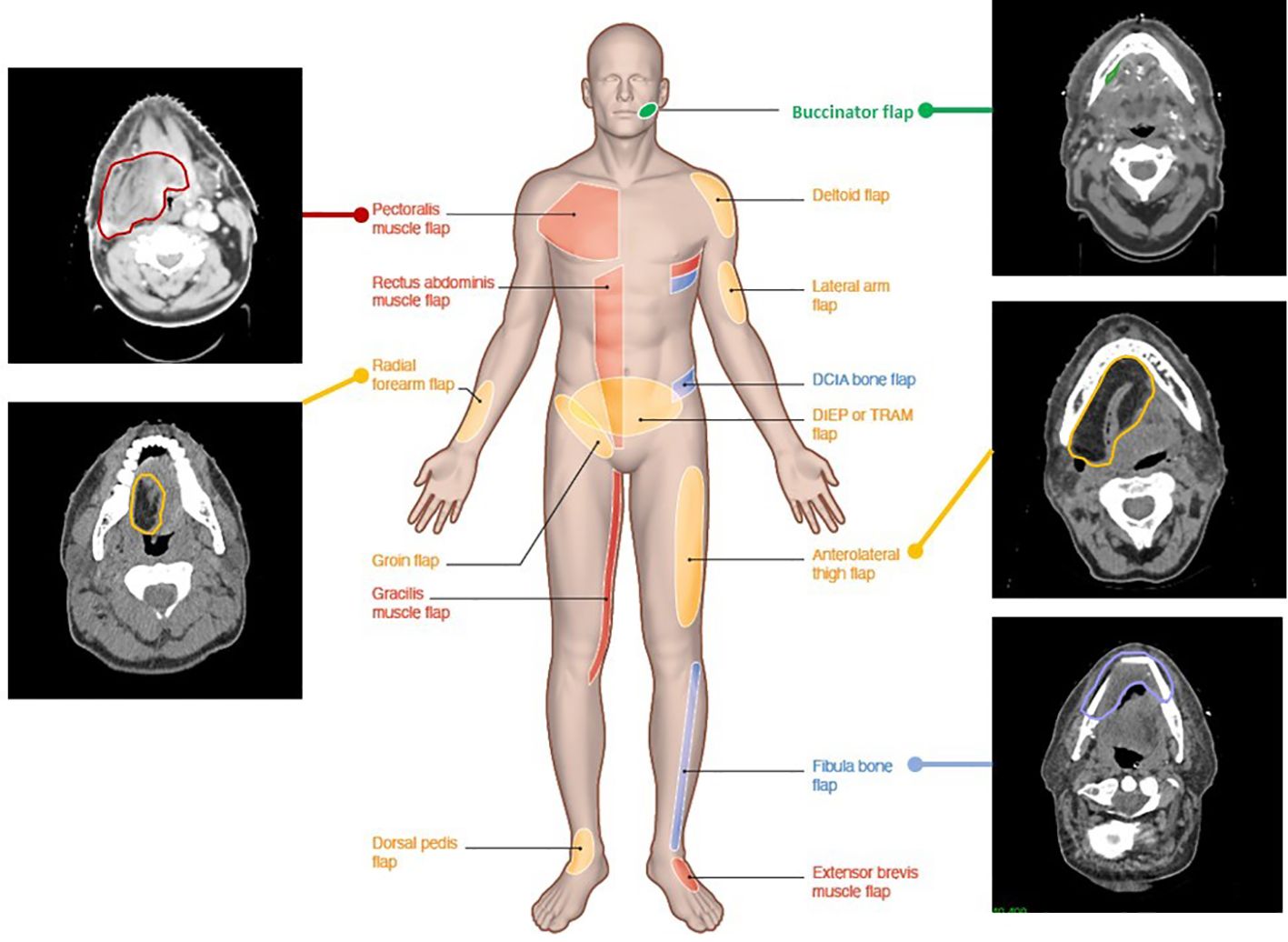
Figure 2 Computed tomography images illustrating various flap reconstructions in head and neck surgery. Flap donor sites from various donor body parts (middle male figure) and their aspect and contours on postoperative radiotherapy planning CT. Red, pedicled flaps; blue, bone free flaps; orange, soft tissue free flaps; green, local flaps.
4.2 Spontaneous flap changes over time
Various changes that are specific to flaps and distinct from native tissues may occur.
4.2.1 Flap failure
Patient factors, such as age and comorbidities (including diabetes mellitus and malnutrition), tumor site, red blood cell transfusion, wound healing and infectious complications, operating room duration, length of hospital stay, or risk of early postoperative readmission are important predictors of flap failure (51, 52). On one hand, flaps, being autologous tissues, are not susceptible to auto-immune rejection. On the other hand, recent isolated reports suggest that anti-cancer immunotherapy might compromise flap vitality (53), possibly due to a higher risk of thrombosis (54, 55).
Flap failure, defined as partial flap or total flap loss, is rare overall. The ultimate success rates of micro-anastomosed free flaps are about 85%–90% (56), but they refer to the final surgical outcome, which may have necessitated flap repair or replacement for early flap complications, which vary from 30% to 80% across surgical series (15, 57). The incidence of perioperative free flap compromise is low, with successful salvage in up to 70%. When the flap is again compromised, second salvage has been reported to be successful in 30% of free flaps (79 in 3,510 flaps in 2000–2020) (58).
Complications must be detected early because of the narrow window of opportunity for flap salvage. Early flap failure is often detected clinically by the loss of viability of a small superficial portion of the soft tissue flap (palor, color, temperature, etc.). Nevertheless, more in-depth (at the tissue–flap junction) complications may be missed by clinical observation. Delayed flap failure may also occur. It can be depicted on CT as intra- or peri-flap fluid collection >4 cm, intra- or peri-flap air collection >2 cm, and fistula to the adjacent aerodigestive tract or skin (59). Early free flap failure may primarily be due to veinous thrombosis (usually within 72 h). Arterial thrombosis can occur even earlier and can be severe (60). Prolonged ischemia time during surgery and revision of microvascular anastomosis are risk factors for flap failure (61, 62). Early thrombectomy, if feasible (short ischemia), leads to better survival than vein revision to neighboring branch veins (58). Local and pedicle flaps can also suffer vascular damage by vessel stretch or torsion. Finally, early flap loss due to necrosis is unlikely due to radiation-induced damage of vascular anastomosis or thrombosis (7).
Imaging is rarely prescribed for the suspicion of early flap failure as clinical suspicion may suffice. Early MRI might, however, be useful to assess deeper tissue damage (63).
Doppler monitoring, hyperspectral imaging (HSI), and microdialysis are gaining popularity as promising techniques for monitoring flap viability. Doppler monitoring is a minimally invasive method where a probe is situated near the vascular anastomosis, while HSI evaluates soft tissue parameters like temperature, re-capillarization time, and flap turgor (64), and microdialysis can be utilized for soft tissue or bone (65). Thiem et al. incorporated HSI as a complementary tool to traditional clinical evaluations for assessing free flap viability in reconstructive surgery (64). Their non-randomized clinical study, which included 54 primary and 11 secondary reconstructions, found that HSI detected perfusion compromise notably earlier than clinical assessments alone, leading to re-exploration decisions about 4.8 ± 5 h sooner (p < 0.001). With a flap salvage rate of 63%, the study implies HSI as a potentially valuable addition to postoperative flap monitoring procedures.
4.2.2 Atrophy
In flaps with a significant muscle component, motor nerve section is usually preferred to its preservation to avoid inadequate contractions. Loss of muscular activity usually results in fatty conversion and overall flap volume reduction of >40% 2 years after surgery (50, 66, 67). Flap volume reduction is related to its components (67, 68) and weight loss, while age is associated with less skin retraction (possibly due to less collagen) and atrophy (muscle/fat proportion). Muscle volume may decrease to less than a third of its immediate postoperative volume (50), while fat volume may be more stable (50, 68), with <20% volume reduction (39, 69). Fat volume reduction in flaps seems to correlate with poorer outcomes: fat volume has been shown to decrease by 70% in patients dying of their HNC and increase to >115% in patients free of disease (50). Fatty tissue atrophy might also be related with flap innervation, direct tissue damage, ischemia during surgery (39), or patency of the flap pedicle and anastomotic blood flow (50, 70). Motor innervation might contribute to preventing atrophy.
4.2.3 Fibrosis
Fibrosis refers to a dynamic, pathological process of parenchymal cell damage, stromal remodeling, and tissue contraction, particularly in the muscle component (71). It manifests as a delayed and progressive reduction in tissue elasticity and flexibility, associated with poorer function. Severe fibrosis can manifest as stiffened cervical skin, dense platysma, and flattened internal jugular vein, which becomes anterior to the common carotid artery.
Ultrasound, CT perfusion (blood flow, blood volume, and time maximum intensity projection), and functional MR [dynamic contrast-enhanced with fractional plasma volume, volume transfer constant, peak enhancement, and time to maximum enhancement and imaging diffusion-weighted imaging (DWI)] can also help to differentiate tumor recurrence from postoperative changes and fibrosis. Soft tissue density on CT and iso-hypointensity on T1/2 sequences represent a scar or fibrosis.
4.2.4 Other flap changes
Other changes include wound dehiscence (separation of skin edges 30%), native skin breakdown, and the presence of a pharyngocutaneous fistula or the conditions requiring re-operation such as venous congestion and ecchymosis, seroma or hematoma, infection (56, 63, 72). Heterotopic ossifications occur from osteogenesis from the vascularized periosteum. They are depicted as linear periosteal attenuations of the vascular pedicle on CT, i.e., ossified vessels. They are reported in up to 50% of patients with fibula flaps within 1 month to 2 years postoperatively (73, 74). Spontaneous bone resorption may occur by about 0.2 mm/year in the native mandible (edentulous being at a higher risk) and transplanted bone to a lesser degree (75). Bone flaps might be at a higher risk for osteoradionecrosis than native bone. Fixation screws and plates at the flap–native bone interface can be responsible for metallic artifacts (with image blurring) and backscatter radiation, which might increase their susceptibility to osteoradionecrosis (76) (see Section 5).
4.3 Tumor recurrence after reconstructive flap surgery
4.3.1 Dissemination pathways in the presence of flaps
Some local myomucosal flaps might, in theory, harbor microscopic disease according to the concept of field cancerization. However, this phenomenon seems to be exceptional (77). Excluding local flaps, flaps are non-tumoral tissues that should not contain tumor cells, in contrast to native tissues surrounding the resection area. Therefore, this junction area between residual native tissues post-resection and the flap would be the area where recurrence would more likely occur before colonizing the flap by contiguity.
Permeation through the flap skin directly has been reported (unpublished) but might indeed have been initiated centripetally from lateral surface mucosal margins in tongue carcinomas, for example. Other unusual patterns of spread have been anecdotally reported where tumor disseminates from the native tissue–flap junction along flap muscle fibers (72).
Apart from these anecdotical situations, this junction area between residual native tissues post-resection and the flap should be identified for careful assessment during follow-up. Based on preclinical or translational studies, flap composition (fat, fascia, smooth or striated muscle, and bone or deperiosted bone) might influence the patterns of tumor recurrence. Tissue transfer and integration into a different site on the same body relies on complex epithelial remodeling between the macroscopic or microscopic components of flaps and surrounding potentially tumorigenic native tissues. It is unknown whether typical “anatomic barriers”, such as fasciae, may retain a barrier effect in flaps. It is also unknown whether adipose tissue transfer from flaps could turn into cancer-activated adipocytes and whether controversies on lipotransfer apply (78). Similarly, it is unknown whether fibroblasts could turn into cancer-activated fibroblasts in their new environment. Anecdotical reports indicate that such interactions might be worth investigating (79). It is also possible that clotting and inflammatory processes contribute to tumor dissemination and local immunotolerance. However, free flaps allow a wider resection, and their use may be associated with fewer marginal resections and better oncologic outcomes by decreasing local recurrences (13, 14, 80, 81). Similarly, poRT studies suggest that relapses occur mainly at the junction between the flap and the native tissues (see chapter 5).
4.3.2 Imaging of recurrence
Imaging reveals sharp boundaries between the flap and normal tissues, indicative of benignity, while poorly limited contrast-enhanced edges of the surgical field may signal local tumor recurrence (14, 72, 81–84). CT characterizes recurrence as a slightly hyperattenuating contrast-enhanced infiltrative mass or nodule, with radionecrosis and infection being the primary differential diagnoses. The less accessible deep flap–tissue junction, especially areas of weakness like muscular perimysium and bone, necessitates a careful inspection.
In a retrospective study, Kim et al. analyzed 93 HNC patients, 82% of whom had a previous surgery (85). Among them, 79 (84.9%) patients had recurrent tumor at the primary site confirmed via surgical resection. The study’s strength lies in using histological evaluation of the neck specimen as the reference standard for both true-positive and true-negative findings. The authors reported the sensitivity and specificity of MRI to be 0.75 and 0.99, respectively, and for PET/CT to be 0.86 and 0.95.
MRI and PET-CT are typically prescribed when relapse is suspected. It is worth noting that flap enhancement is not specific for recurrence, varying from no to diffuse enhancement with neither T2 hypersignal nor contrast-enhanced T1 being specific for flap failure (47). MRI protocols should include 3DT1, axial fat-saturated T2, post-contrast fat-saturated T1, and diffusion-weighted (DWI) sequences. Low apparent diffusion coefficient (ADC) on DWI-MRI could be indicative (47, 86), but it is not specific for small foci of viable cancer cells along flap muscle fibers.
18FDG PET/CT improves sensitivity and specificity in recurrence detection. Ravanelli et al. evaluated [18F] FDG PET-CT’s usefulness in following up surgically treated oral tongue squamous cell carcinoma (87). Among the 87 included patients, 68 (78%) had a flap. The study classified PET uptakes in the oral cavity as functional, suspicious, or highly suggestive of neoplastic recurrence. FDG uptake was observed in the oral cavity during follow-up in 59 (68%) patients, primarily deep in the floor of the mouth, near the interface between the native tissue and the flap. This was explained as compensatory hyperactivation of the contralateral extrinsic oral muscles, which may retract the flap in distorted and asymmetric anatomies. Nodular regions of FDG uptake deep to the flap at the native flap–tissue junction warrant particular attention (88).
Finally, CT is the preferred initial imaging modality for recurrence detection, while PET-CT and MRI may detect early relapses, considering the false-positive rates of PET-CT for up to 12 weeks (88). The use of MRI to quantify flap changes and correlate them with functional outcomes is worth investigating (69, 86).
5 Effects of postoperative radiotherapy on flaps
5.1 Efficacy and toxicity of postoperative radiotherapy
Radiation-associated soft tissue injury can range from acute reversible toxicities to destructive degenerative processes such as osteoradionecrosis, which can contribute to flap failure and delay oral rehabilitation. The surgical literature suggests that postoperative radiotherapy (poRT) alters the functional outcomes of reconstructive flap surgery (89–91). Brachytherapy increases the rate of complications in patients undergoing microvascular free tissue transfer (92). With external beam radiotherapy, there is a clear imbalance between patients receiving surgery alone or poRT in patients with poor prognostic factors. Most studies have been small, non-randomized, and retrospective without propensity score matching (Table 2).
Surgically treated HNSCC patients undergo poRT based on the presence/absence of pathological risk factors for recurrence (93, 94). These risk factors include positive surgical margins and extranodal extension, lymphovascular invasion, perineural invasion (PNI), advanced T stage (pT3–4), high grade, advanced N stage (N2–3), involved nodal levels IV–V (NCCN), worst invasion pattern (WPOI), metastatic nodal burden, and ratio in neck dissection specimen (95–100). In patients with high risk factors such as extranodal extension (ENE) or positive surgical margins (PSM), post-operative radiation therapy (poRT) enhances loco-regional control, disease-free survival, and overall survival. Moreover, when concurrent cisplatin chemotherapy is implemented, the 5-year survival rates see an improvement from 40% to 50% (95). poRT is considered in intermediate-risk patients with pT3–4 disease or positive nodes without ENE or PSM (101). However, the benefit of poRT is less clear when the sole adverse factors present are perineural invasion (PNI), lymphovascular invasion, differentiation, or specific patterns of invasion (93). In a randomized trial updated for long-term outcomes, there was no dose–response above 63 Gy (102). The time interval between surgery and poRT has more impact on survival than dose and should be ≤6 weeks (NCCN), and flaps do not seem to compromise it (103). In high-risk patients, the primary tumor bed with PSM/ENE receives 60–66 Gy in 1.8–2-Gy fractions, and the areas at risk for microscopic involvement receive 50–54 Gy. Most evidence for poRT using modern RT techniques are retrospective, and adherence to guidelines is low in practice. In patients with HPV+ oropharyngeal cancer, minimally morbid surgery +/- poRT (21%–58% of cases) or chemo-poRT (16%–62% of cases) is often proposed (104). Deescalated poRT dose/volumes are being investigated in trials to achieve better long-term functional outcomes in patients with HPV+ oropharyngeal cancer (105). In the poRT with IMRT era (poIMRT), the patterns of failure are mostly in-field (≈65%) or marginal (≈25%). Marginal relapses in the presence of ENE appear to require generous margins around the tumor bed (≈1.5 cm) (106–108); some occurred at the match line at the field junction or near the spared parotids in steep dose gradients (109). Out-of-field relapses have been reported mostly as contralateral nodal relapses in pN2a-b oral cavity cases and seem unlikely to be salvaged (110, 111). In oral cavity cancers, most (55%) local failures appeared to occur in the central high-dose region and less frequently in central intermediate-low-dose regions (18.5%) and out-of-field-dose regions (16.7%) (80). Local patterns of failure after poIMRT are less reported.
Considerable gaps in knowledge exist on how to handle a flap when poRT is needed and the transfer of flap-related advances to poRT (7, 45, 104, 112–114). Flaps now represent a substantial proportion of patients operated on for mucosal HNC (11). The patterns of relapse have only been anecdotally reported, with relapses that occur being mostly marginal and not intra-flap (115). Flap reconstruction anticipation before poRT seems to improve the margin quality and oncologic outcomes (80).
5.2 Postoperative radiotherapy planning in the presence of flaps
5.2.1 Planning CT protocols
At the time of poRT planning, the operative bed often shows a dramatically modified anatomy [such as edema, fluid/air bubble collection (hematoma, lymphocele), thickening of the skin, and platysma or reticulation of the subcutaneous fat] secondary to tissue loss, deformation, and inflammatory changes of adjacent normal structures and, increasingly often, the presence of reconstructive tissues (flaps) and materials. The post-reconstruction imaging interpretation is challenging to define the clinical target volume of the operative bed. Moreover, not all radiotherapy departments perform optimal CT due to logistics constraints (lack of radiologists for customized protocols, lack of physicians to monitor allergic contra-indications to iodine agents, and longer acquisition times with contrast enhancement). Due to its benefit on delineation accuracy (116), contrast-enhanced poRT planning CT may be recommended using a split-bolus technique to visualize arteries, veins, native tissues, residual tumors, and flaps. The intravenous iodine agent is infused at 2.5 mL/s, with 55 mL injected first and another 55 mL after a 40-s delay. Thin-section (≈1 mm) image acquisition is performed after a 90-s delay, followed by multiplanar reconstructions. Image artefact blurring is often reduced using MAR algorithms but still warrants inaccurate manual overriding for dosimetry. Multi-energy/spectral CT is largely implanted in radiodiagnostics but less systematically in radiotherapy departments. Moreover, proprietary treatment planning system (TPS) formats often alter spatial resolution to limit computational times, resulting in blurred/indistinct images hampering flap visualization. Knowing flap-related findings on high-resolution imaging is critical to distinguish flaps from surrounding anatomy and assess their spontaneous changes over time and after poRT on the one hand and tumor recurrence or flap complications on the other hand (117).
5.2.2 Flap delineation
PoRT recommendations lack guidelines on flap delineation and dose constraints, a situation possibly attributed to the complexity and time-consuming nature of delineation, given factors such as heterogeneity, altered anatomy, and interpretation of operative and pathology reports (104, 114, 118, 119). However, an accurate definition of flap boundaries is critical for successful flap sparing (120).
The current approach often encompasses over 80% of flaps in target volumes, typically focusing on the hypodense fatty portion of the flap (112). Dose–volume effects, unfortunately, have been scarcely documented (112). To address this, automatic segmentation tools utilizing neural networks may facilitate a more systematic flap delineation. Moreover, virtual marking of resection borders using a navigation pointer and titanium ligature clips can aid in delineation, as suggested by a prospective study (120). Establishing a standardized approach to flap delineation could significantly assist in defining flap dose–volume effects (see Section 5.3).
With the use of IMRT, high dose conformity in at-risk areas can be achieved while preserving other areas through a technique known as dose painting. By utilizing dose painting by contours (DPBC), the incidence of late mucosal ulcers can be reduced, thereby enhancing the patients’ quality of life (121). This protocol could be adapted for flap sparing, maintaining the dose at the native tissue–flap junction and limiting the dose to 40 Gy for portions of the flap distant from this junction, such as the center and surface (122)—for example, in a reconstructed mobile tongue, only the deep and lateral junction and an area of 6 mm into the flap at risk may receive an intermediate or high dose of 60–66 Gy, guided by the pathological report (Figure 3). Longitudinal studies are required to evaluate if this strategy leads to improvements in morphology, volume, texture, and functional outcomes without raising the risk of local recurrence.
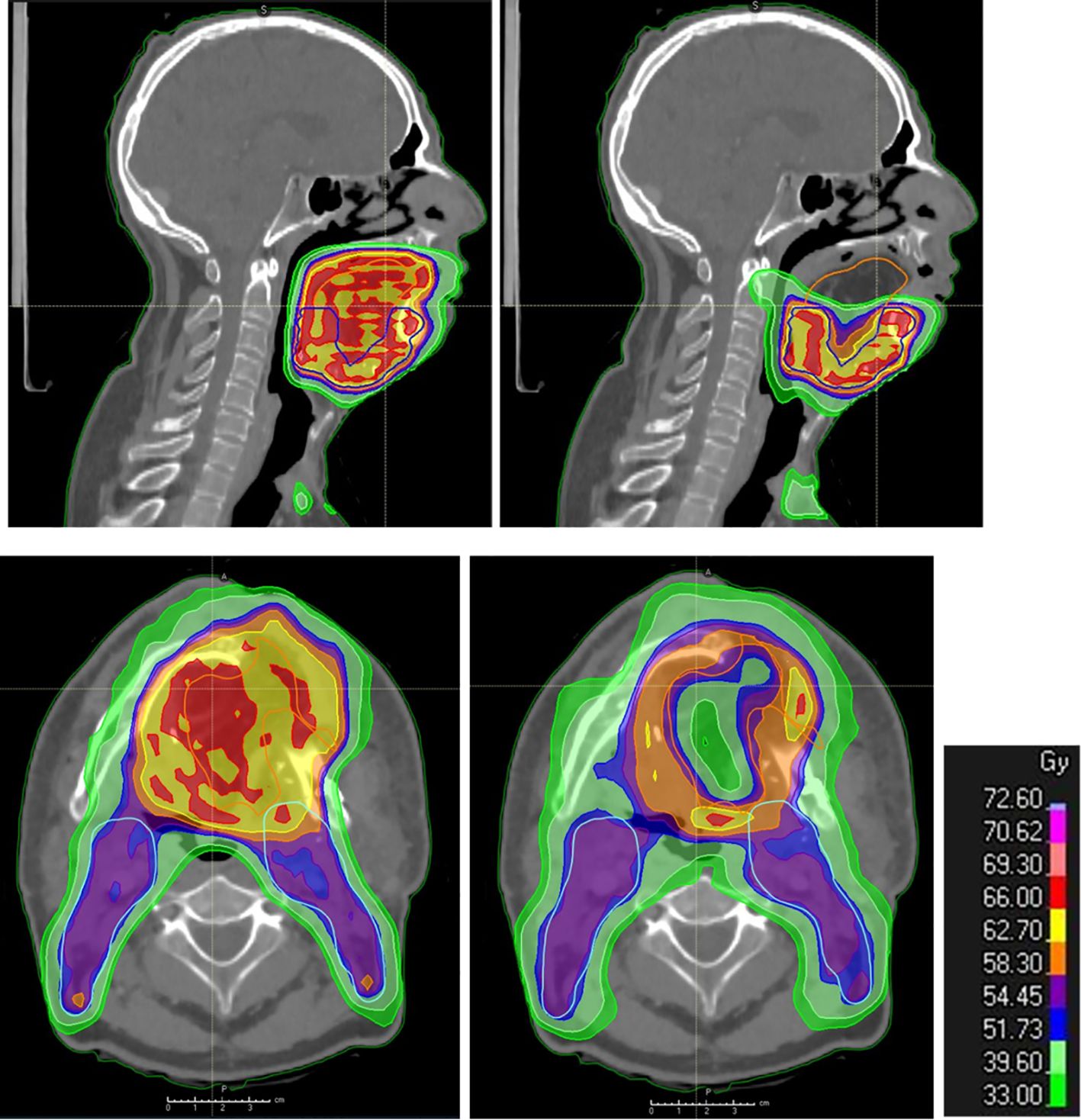
Figure 3 Post-operative radiation therapy planning in the presence of a flap. Dosimetry showing standard coverage (left: upper panel in sagittal view and lower panel in axial view) and flap sparing (right: upper panel in sagittal view and lower panel in axial view; the cranial part of the flap is spared as well as the central part, while the flap–native tissue junction receives full tumor dose) in flap (yellow contour) areas distant from the native tissues at risk of harboring microscopic tumor in a patient with reconstructive anterolateral thigh flap surgery for oral cavity cancer.
The identification of flaps as organs at risk and their accurate delineation would allow for specific sparing strategies. This would not only optimize the radiation dose but also minimize flap-related complications (Section 5.3), improving the overall patient outcomes. Future advancements may include the use of AI for precision delineation, but that remains a promising area for exploration.
5.3 Contribution of poRT to flap changes or complications
A total of 10 key articles primarily addressed radiation-induced changes or complications in the context of flap-based head and neck cancer (HNC) radiotherapy (Supplementary Data S2) (89, 90, 103, 112, 123–128). The reports indicated a significant mean flap reduction ranging from 8% to 70%.
5.3.1 Radiation-induced atrophy
In addition to spontaneous and host-dependent atrophy (50), poRT further contributes to flap volume reduction (68, 129) (Figure 4). With an average flap volume loss of 34%, 39% was reported in patients with poRT versus 31% in patients without poRT (130), probably by muscle atrophy, which itself is related to innervation (37, 39). This occurred despite efficient flap vascularization possibly through radiation endarteritis and chronic ischemia (39). PoIMRT may be offered to reduce high-dose irradiation to some parts of the flap (37).
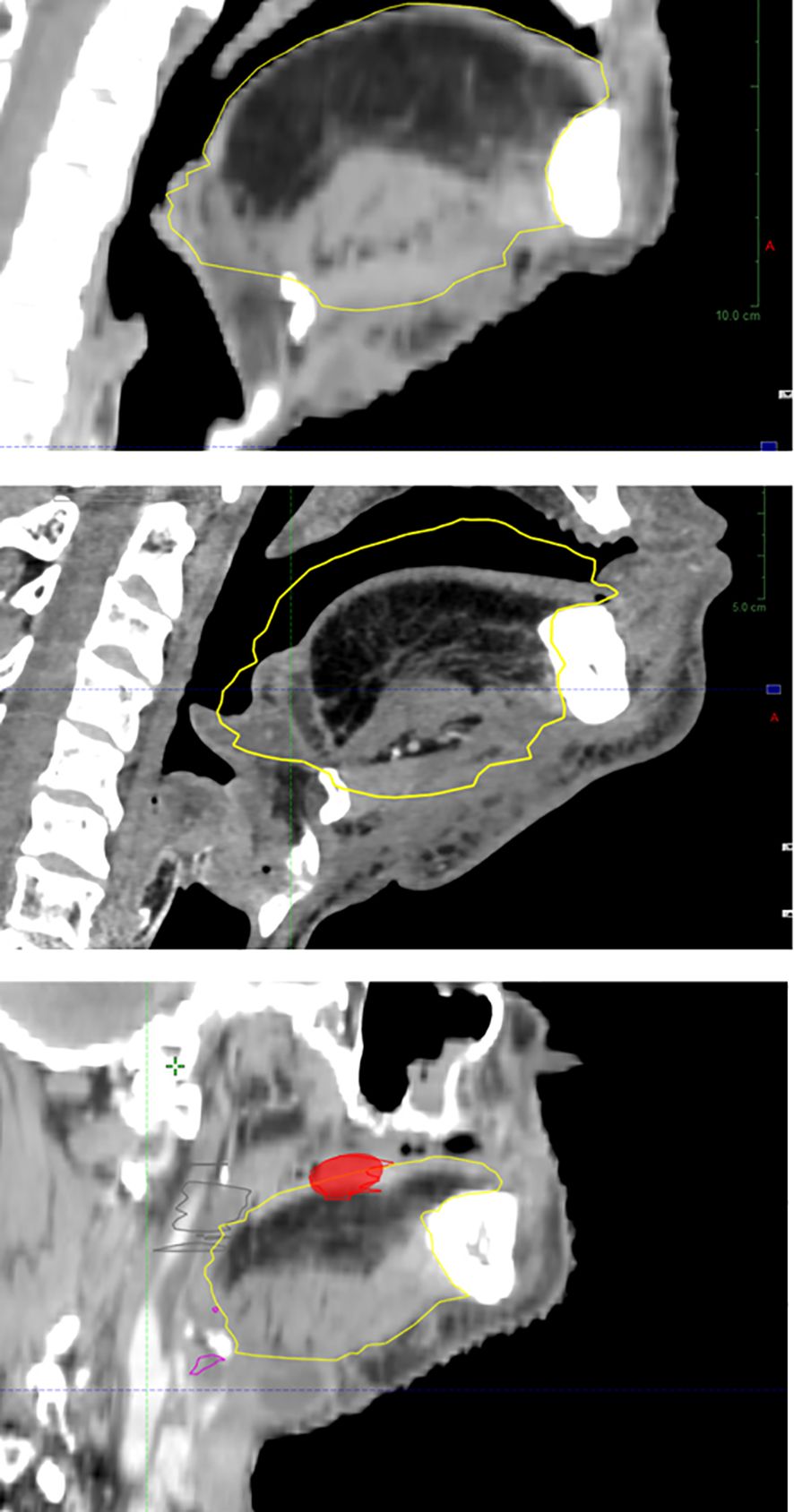
Figure 4 Flap atrophy over time with local relapse at the flap–native tissue junction (baseline, upper panel; atrophy at 1 year, intermediate panel; relapse at 2 years, lower panel).
5.3.2 Radiation-induced fibrosis
Radiation-induced fibrosis (RIF) is depicted clinically as a woody aspect (131, 132). Mild to moderate RIF is frequent (133). A vascularized fibrotic scar depicts low soft tissue T2 signal (lower than recurrence) and ill-defined margins and enhancement and might be difficult to differentiate from recurrence (134). Along with fibrosis, RIF might participate in altered soft tissue flap versatility (7). It may be more severe in flaps compared to other tissues (37, 39); it often accompanies subcutaneous fibrosis and mucosal edema (130).
5.3.3 Osteoradionecrosis
Osteoradionecrosis is a known complication following definitive IMRT, manifesting in 1%–10% of patients typically within 1 to 2 years post-treatment. In the context of reconstructed bone, osteoradionecrosis rates have reached up to 34% following reconstructive flap surgery and poRT (135). The potential risk factors include the number of osteotomies, which can lead to impaired vascularization due to the increased dissection of periosteum from the bone and decreased resistance to radiation-induced vascular damage, the exposure of hardware or plates and the potential for bacterial seeding, and the use of bone free flaps (in place of the native mandible), which are particularly susceptible as the bony margins have only undergone the initial stage of healing before being exposed to poRT. The presence of dental implants might also contribute to osteoradionecrosis. Therefore, in anticipation of poRT, the decision to perform a large number of osteotomies should be carefully balanced against the requirements of achieving a highly conformal reconstructed bone and maintaining long-term viability. The soft tissue provided must be sufficiently broad to allow perfect coverage of the osteosynthesis plates, even if it means harvesting a second soft free flap (136). Besides this, caution should be exerted with radiation fields passing through metals, especially for patients treated with proton therapy. Dose backscatter from plates and screws might result in increased dose to the native and flap segments at their junction, but lack of data prevents risk estimates (137). Above all, a poRT dose over 60 Gy (possibly through pericapillary fibrosis) is associated with an increased risk of developing osteoradionecrosis in the bony flap, along with large resection, large RT field, and chemotherapy (135). This issue must be considered, especially when reoperation for secondary bone reconstruction and dental rehabilitation is required after poRT. It is worth noting that dose escalation does not provide survival benefit (102, 138) but induces significant collateral tissue damage and acute and late toxicity (135), suggested to not exceed 66 Gy in the poRT setting in the absence of macroscopic tumor.
5.3.4 Other radiation-induced effects
PoRT may result in acute and delayed injury to the skin, including color, texture, and elasticity, increased enhancement and thickening of flap components such as skin and platysma, fat reticulation, and reduced vessel lumen (15). Facial nerve management (dissected when macroscopically involved and reconstructed using the hypoglossal nerve, for example) has been extensively described in parotid tumors to restore facial palsy. poRT did not compromise functional outcomes, suggesting that flap reinnervation is feasible before poRT if needed (139).
5.3.5 Impact of postoperative radiotherapy on flap failure
There is a persistent ambiguity surrounding the risks associated with prior RT and poRT. Long-term radiation-induced adverse effects are well documented, including alterations in smooth muscle density, endothelial cell dehiscence, vessel wall fibrosis, connective tissue scarring, and numerous vascular lesions exhibiting microthrombi and heightened proadhesive and prothrombotic properties (140). In the short term, small local flaps can typically attain autonomy from their pedicle within 3 weeks (up to 12 weeks for larger free flaps) through neovascularization by adjacent recipient tissues. As poRT should be administered within 42 days, by which time the vascular supply has usually been consolidated, there is scant evidence to suggest that poRT could jeopardize the flap’s vascular supply in the short term. Consequently, early flap loss might be more attributable to the surgical procedure rather than poRT. Nonetheless, poRT can independently induce an increased expression of cytokines and leukocyte adhesion molecules and promote clotting via mechanisms such as leukocyte or platelet endothelial adherence, thrombus formation, a sustained increase in plasminogen activator inhibitor PAI-1, persistent inflammation due to NF-κB, and atherosclerosis (141, 142). Hence, there is ongoing debate regarding the immediate effects of poRT on the incidence and severity of flap complications (122). The need for prospective controlled studies to elucidate this matter is pressing.
5.3.6 Impact of postoperative radiotherapy on functional flap outcomes
Functional deleterious impact of poRT on flaps occurs by volume reduction, muscle fibrosis, subcutaneous tissues, and skin retraction (39). However, prospective data showed that some patients recovered, while others did not (143). Interestingly, functional kinetics were highly correlated with survival. Tissue effects and their reversibility are less understood. Therefore, imaging and functional data on poRT/no poRT series are needed to correlate flap changes with outcomes and investigate the causality and responsibility of poRT. PoRT may delay sensory recovery, while motor recovery does not seem to be altered if one considers facial nerve outcomes in irradiated parotid tumors. Further data are, however, warranted (34).
6 Conclusion and perspectives
Reconstructive flap surgery in HNC patients represents a significant interdisciplinary challenge that facilitates the personalization of multimodal treatment de-escalation. The present review has some limitations. It is not systematic or quantitative, and only 10 studies specifically focusing on flap and poRT were found and meticulously analyzed in this review. Despite this, the narrative approach allowed us to cover a broader spectrum of information than a systematic review could. The poRT planning process could be improved by integrating interdisciplinary definitions of spread patterns and standardizing multimodal imaging for flap delineation both prior to and during the follow-up stages of poRT (144–147). Moreover, the evaluation of functional flap outcomes should be incorporated into the treatment process, and dedicated trials are needed to optimize patient care.
Author contributions
JT: Writing – original draft, Writing – review & editing. FC: Writing – original draft, Writing – review & editing. AB: Writing – original draft, Writing – review & editing. SD: Writing – original draft, Writing – review & editing. P-YM: Writing – original draft, Writing – review & editing. AM: Writing – original draft, Writing – review & editing. CD: Writing – original draft, Writing – review & editing. BD: Writing – original draft, Writing – review & editing.
Funding
The author(s) declare that no financial support was received for the research, authorship, and/or publication of this article.
Conflict of interest
The authors declare that the research was conducted in the absence of any commercial or financial relationships that could be construed as a potential conflict of interest.
Publisher’s note
All claims expressed in this article are solely those of the authors and do not necessarily represent those of their affiliated organizations, or those of the publisher, the editors and the reviewers. Any product that may be evaluated in this article, or claim that may be made by its manufacturer, is not guaranteed or endorsed by the publisher.
Supplementary material
The Supplementary Material for this article can be found online at: https://www.frontiersin.org/articles/10.3389/fonc.2024.1379861/full#supplementary-material
References
1. Siegel RL, Miller KD, Wagle NS, Jemal A. Cancer statistics, 2023. CA: A Cancer J Clin. (2023) 73:17–48. doi: 10.3322/caac.21763
2. Machtay M, Moughan J, Trotti A, Garden AS, Weber RS, Cooper JS, et al. Factors associated with severe late toxicity after concurrent chemoradiation for locally advanced head and neck cancer: an RTOG analysis. J Clin Oncol. (2008) 26:3582–9. doi: 10.1200/JCO.2007.14.8841
3. Mody MD, Rocco JW, Yom SS, Haddad RI, Saba NF. Head and neck cancer. Lancet. (2021) 398:2289–99. doi: 10.1016/S0140-6736(21)01550-6
4. Mehanna H, Wong WL, Dunn J. Management of advanced nodal disease in patients treated with primary chemoradiotherapy. Curr Opin Oncol. (2016) 28:201–4. doi: 10.1097/CCO.0000000000000283
5. Nutting CM, Morden JP, Shreerang Urbano Harrington TGuerrero KJ, Bhide A, Clark C, et al. Parotid-sparing intensity modulated versus conventional radiotherapy in head and neck cancer (PARSPORT): a phase 3 multicentre randomised controlled trial. Lancet Oncol. (2011) 12:127–36. doi: 10.1016/S1470-2045(10)70290-4
6. Thiruchelvam PTR, Leff DR, Godden AR, Cleator S, Wood SH, Kirby AM, et al. Primary radiotherapy and deep inferior epigastric perforator flap reconstruction for patients with breast cancer (PRADA): a multicentre, prospective, non-randomised, feasibility study. Lancet Oncol. (2022) 23:682–90. doi: 10.1016/S1470-2045(22)00145-0
7. Carsuzaa F, Lapeyre M, Gregoire V, Maingon P, Beddok A, Marcy P-Y, et al. Recommendations for postoperative radiotherapy in head & neck squamous cell carcinoma in the presence of flaps: A GORTEC internationally-reviewed HNCIG-endorsed consensus. Radiother Oncol. (2021) 160:140–7. doi: 10.1016/j.radonc.2021.04.026
8. Brown JS, Shaw RJ. Reconstruction of the maxilla and midface: introducing a new classification. Lancet Oncol. (2010) 11:1001–8. doi: 10.1016/S1470-2045(10)70113-3
9. Seth R, Khan AA, Pencavel T, Harrington KJ, Harris PA. Targeted gene delivery by free-tissue transfer in oncoplastic reconstruction. Lancet Oncol. (2012) 13:e392–402. doi: 10.1016/S1470-2045(12)70235-8
10. Cannon RB, Houlton JJ, Mendez E, Futran ND. Methods to reduce postoperative surgical site infections after head and neck oncology surgery. Lancet Oncol. (2017) 18:e405–13. doi: 10.1016/S1470-2045(17)30375-3
11. Marhic A, Guerlain J, Benmoussa N, Breuskin I, Honart J-F, Janot F, et al. Replacement of lip-split mandibulotomy by pull-through approach for T3-4 oral carcinomas. Int J Oral Maxillofac Surg. (2021) 50:1123–30. doi: 10.1016/j.ijom.2020.10.011
12. Lam L, Samman N. Speech and swallowing following tongue cancer surgery and free flap reconstruction–a systematic review. Oral Oncol. (2013) 49:507–24. doi: 10.1016/j.oraloncology.2013.03.001
13. Hanasono MM, Friel MT, Klem C, Hsu PW, Robb GL, Weber RS, et al. Impact of reconstructive microsurgery in patients with advanced oral cavity cancers. Head Neck. (2009) 31:1289–96. doi: 10.1002/hed.21100
14. de Vicente JC, Rodríguez-Santamarta T, Rosado P, Peña I, de Villalaín L. Survival after free flap reconstruction in patients with advanced oral squamous cell carcinoma. J Oral Maxillofac Surg. (2012) 70:453–9. doi: 10.1016/j.joms.2011.02.020
15. Saito N, Nadgir RN, Nakahira M, Takahashi M, Uchino A, Kimura F, et al. Posttreatment CT and MR imaging in head and neck cancer: what the radiologist needs to know. RadioGraphics. (2012) 32:1261–82. doi: 10.1148/rg.325115160
16. Offodile AC, Lin JA-J, Chang K-P, Abdelrahman M, Kou H-W, Loh CYY, et al. Anterolateral thigh flap combined with reconstruction plate versus double free flaps for composite mandibular reconstruction: A propensity score-matched study. Ann Surg Oncol. (2018) 25:829–36. doi: 10.1245/s10434-017-6309-1
17. Quinlan-Davidson SR, Mohamed ASR, Myers JN, Gunn GB, Johnson FM, Skinner H, et al. Outcomes of oral cavity cancer patients treated with surgery followed by postoperative intensity modulated radiation therapy. Oral Oncol. (2017) 72:90–7. doi: 10.1016/j.oraloncology.2017.07.002
18. Eskander A, Kang SY, Tweel B, Sitapara J, Old M, Ozer E, et al. Quality indicators: measurement and predictors in head and neck cancer free flap patients. Otolaryngol Head Neck Surg. (2018) 158:265–72. doi: 10.1177/0194599817742373
19. Raghuram AC, Manfro G, Teixeira GV, Cernea CR, Dias FL, Marco De M, et al. Use of single chimeric free flaps or double free flaps for complex head and neck reconstruction. J Reconstr Microsurg. (2021) 37:791–8. doi: 10.1055/s-0041-1727188
20. Wehage IC, Fansa H. Complex reconstructions in head and neck cancer surgery: decision making. Head Neck Oncol. (2011) 3:14. doi: 10.1186/1758-3284-3-14
21. Deneuve S, Majoufre C, Testelin S, Barry B, Louis MY, Longis J, et al. Donor site sequelae and patient satisfaction after head and neck reconstruction with a radial forearm free flap. Eur Arch Otorhinolaryngol. (2021) 278:4051–8. doi: 10.1007/s00405-021-06649-0
22. Löfstrand J, Nyberg M, Karlsson T, Thórarinsson A, Kjeller G, Lidén M, et al. Quality of life after free fibula flap reconstruction of segmental mandibular defects. J Reconstr Microsurg. (2018) 34:108–20. doi: 10.1055/s-0037-1606537
23. Hilven PH, Vranckx JJ. The iliac crest osteomuscular flap for bony reconstruction: beast or beauty? A reassessment of the value and donor site morbidity in the CAD/CAM era. J Reconstr Microsurg. (2021) 37:671–81. doi: 10.1055/s-0041-1724129
24. Lonie S, Herle P, Paddle A, Pradhan N, Birch T, Shayan R. Mandibular reconstruction: meta-analysis of iliac- versus fibula-free flaps. ANZ J Surg. (2016) 86:337–42. doi: 10.1111/ans.13274
25. Urken ML. Advances in head and neck reconstruction. Laryngoscope. (2003) 113:1473–6. doi: 10.1097/00005537-200309000-00008
26. Kovatch KJ, Hanks JE, Stevens JR, Stucken CL. Current practices in microvascular reconstruction in otolaryngology-head and neck surgery. Laryngoscope. (2019) 129:138–45. doi: 10.1002/lary.27257
27. Abouyared M, Katz AP, Ein L, Ketner J, Sargi Z, Nicolli E, et al. Controversies in free tissue transfer for head and neck cancer: A review of the literature. Head Neck. (2019) 41:3457–63. doi: 10.1002/hed.25853
28. Mady LJ, Poonia SK, Baddour K, Snyder V, Kurukulasuriya C, Frost AS, et al. Consensus of free flap complications: Using a nomenclature paradigm in microvascular head and neck reconstruction. Head Neck. (2021) 43:3032–41. doi: 10.1002/hed.26789
29. Lyons AJ. Perforator flaps in head and neck surgery. Int J Oral Maxillofac Surg. (2006) 35:199–207. doi: 10.1016/j.ijom.2005.07.015
30. Loewen IJ, Boliek CA, Harris J, Seikaly H, Rieger JM. Oral sensation and function: a comparison of patients with innervated radial forearm free flap reconstruction to healthy matched controls. Head Neck. (2010) 32:85–95. doi: 10.1002/hed.21155
31. Mishra S, Rao VUS, Thakur S, Subash A. ‘Volume and location of the defect as predictors of speech outcome after glossectomy: correlation with a classification’-Would collateral reinnervation improve the outcome? Int J Oral Maxillofac Surg. (2023) 52:619–20. doi: 10.1016/j.ijom.2022.04.021
32. Takayama Y, Yokoo S, Makiguchi T, Komori T. Motor nerve preservation and muscle atrophy after pectoralis major musculocutaneous flap surgery for oromandibular reconstruction. J Craniofac Surg. (2016) 27:2055–60. doi: 10.1097/SCS.0000000000002969
33. Dvořák Z, Pink R, Michl P, Heinz P, Tvrdý P. Pedicled pectoralis major flap in head and neck reconstruction - technique and overview. Acta Chir Plast. (2019) 60:14–21.
34. Yu P. Reinnervated anterolateral thigh flap for tongue reconstruction. Head Neck. (2004) 26:1038–44. doi: 10.1002/hed.20106
35. Wang L, Wei J-H, Yang X, Yang Z-H, Sun M-Y, Cheng X-B, et al. Preventing early-stage graft bone resorption by simultaneous innervation: innervated iliac bone flap for mandibular reconstruction. Plast Reconstr Surg. (2017) 139:1152e–61e. doi: 10.1097/PRS.0000000000003263
36. Jeong HH, Jeong WS, Choi JW, Jeong SE, Nam SY, Choi SH, et al. 3D computer simulation analysis of the flap volume change in total tongue reconstruction flaps. J Craniomaxillofac Surg. (2018) 46:844–50. doi: 10.1016/j.jcms.2018.02.013
37. Sakakibara A, Kusumoto J, Sakakibara S, Hasegawa T, Akashi M, Minamikawa T, et al. Long-term effects on volume change in musculocutaneous flaps after head and neck reconstruction. J Reconstr Microsurg. (2019) 35:235–43. doi: 10.1055/s-0038-1672134
38. Kamizono K-I, Yoshida S, Yasumatsu R, Kadota H. Volumetric changes of transferred free anterolateral thigh flaps in head and neck lesions. Auris Nasus Larynx. (2021) 48:751–7. doi: 10.1016/j.anl.2020.12.006
39. Fujioka M, Masuda K, Imamura Y. Fatty tissue atrophy of free flap used for head and neck reconstruction. Microsurgery. (2011) 31:32–5. doi: 10.1002/micr.20811
40. Bauer E, Mazul A, Zenga J, Graboyes EM, Jackson R, Puram SV, et al. Complications after soft tissue with plate vs bony mandibular reconstruction: A systematic review and meta-analysis. Otolaryngol Head Neck Surg. (2021) 164:501–11. doi: 10.1177/0194599820949223
41. Chang T-Y, Lai Y-S, Lin C-Y, Wang JD, Pan SC, Shieh SJ, et al. Plate-related complication and health-related quality of life after mandibular reconstruction by fibula flap with reconstruction plate or miniplate versus anterolateral thigh flap with reconstruction plate. Microsurgery. (2023) 43:131–41. doi: 10.1002/micr.30893
42. Annino DJ, Hansen EE, Sethi RK, Horne S, Rettig EM, Uppaluri R, et al. Accuracy and outcomes of virtual surgical planning and 3D-printed guides for osseous free flap reconstruction of mandibular osteoradionecrosis. Oral Oncol. (2022) 135:106239. doi: 10.1016/j.oraloncology.2022.106239
43. Khadembaschi D, Brierly GI, Chatfield MD, Beech N, Batstone MD. Systematic review and pooled analysis of survival rates, success, and outcomes of osseointegrated implants in a variety of composite free flaps. Head Neck. (2020) 42:2669–86. doi: 10.1002/hed.26238
44. Schiegnitz E, Reinicke K, Sagheb K, König J, Al-Nawas B, Grötz KA. Dental implants in patients with head and neck cancer-A systematic review and meta-analysis of the influence of radiotherapy on implant survival. Clin Oral Implants Res. (2022) 33:967–99. doi: 10.1111/clr.13976
45. Le Guevelou J, Bastit V, Marcy PY, Lasne-Cardon A, Guzene L, Gerard M, et al. Flap delineation guidelines in postoperative head and neck radiation therapy for head and neck cancers. Radiother Oncol. (2020) 151:256–65. doi: 10.1016/j.radonc.2020.08.025
46. McCarty JL, Corey AS, El-Deiry MW, Baddour HM, Cavazuti BM, Hudgins PA. Imaging of surgical free flaps in head and neck reconstruction. AJNR Am J Neuroradiol. (2019) 40:5–13. doi: 10.3174/ajnr.A5776
47. Chong J, Chan LL, Langstein HN, Ginsberg LE. MR imaging of the muscular component of myocutaneous flaps in the head and neck. Am J Neuroradiology. (2001) 22:170–4.
48. Hsu D, Chokshi FH, Hudgins PA, Kundu S, Beitler JJ, Patel MR, et al. Predictive value of first posttreatment imaging using standardized reporting in head and neck cancer. Otolaryngol Head Neck Surg. (2019) 161:978–85. doi: 10.1177/0194599819865235
49. Genden EM, Rinaldo A, Suárez C, Wei WI, Bradley PJ, Ferlito A. Complications of free flap transfers for head and neck reconstruction following cancer resection. Oral Oncol. (2004) 40:979–84. doi: 10.1016/j.oraloncology.2004.01.012
50. Yamaguchi K, Kimata Y, Onoda S, Mizukawa N, Onoda T. Quantitative analysis of free flap volume changes in head and neck reconstruction. Head Neck. (2012) 34:1403–7. doi: 10.1002/hed.21944
51. Eskander A, Kang S, Tweel B, Sitapara J, Old M, Ozer E, et al. Predictors of complications in patients receiving head and neck free flap reconstructive procedures. Otolaryngol Head Neck Surg. (2018) 158:839–47. doi: 10.1177/0194599818757949
52. Sweeny L, Curry JM, Crawley MB, DiLeo M, Bonaventure CA, Luginbuhl AJ, et al. Age and comorbidities impact medical complications and mortality following free flap reconstruction. Laryngoscope. (2022) 132:772–80. doi: 10.1002/lary.29828
53. Mays AC, Yarlagadda B, Achim V, Jackson R, Pipkorn P, Huang AT, et al. Examining the relationship of immunotherapy and wound complications following flap reconstruction in patients with head and neck cancer. Head Neck. (2021) 43:1509–20. doi: 10.1002/hed.26601
54. Wang T-F, Khorana AA, Carrier M. Thrombotic complications associated with immune checkpoint inhibitors. Cancers (Basel). (2021) 13:4606. doi: 10.3390/cancers13184606
55. Roopkumar J, Swaidani S, Kim AS, Thapa B, Gervaso L, Hobbs BP, et al. Increased incidence of venous thromboembolism with cancer immunotherapy. Med. (2021) 2:423–34. doi: 10.1016/j.medj.2021.02.002
56. Spoerl S, Schoedel S, Spanier G, Mueller K, Meier JK, Reichert TE, et al. A decade of reconstructive surgery: outcome and perspectives of free tissue transfer in the head and neck. Experience of a single center institution. Oral Maxillofac Surg. (2020) 24:173–9. doi: 10.1007/s10006-020-00838-7
57. Sweeny L, Curry J, Crawley M, Cave T, Stewart M, Luginbuhl A, et al. Factors impacting successful salvage of the failing free flap. Head Neck. (2020) 42:3568–79. doi: 10.1002/hed.26427
58. Slijepcevic AA, Young G, Shinn J, Cannady SB, Hanasono M, Old M, et al. Success and outcomes following a second salvage attempt for free flap compromise in patients undergoing head and neck reconstruction. JAMA Otolaryngol Head Neck Surg. (2022) 148:555–60. doi: 10.1001/jamaoto.2022.0793
59. Kim B, Yoon DY, Seo YL, Park MW, Kwon KH, Rho Y-S, et al. Value of the post-operative CT in predicting delayed flap failures following head and neck cancer surgery. Korean J Radiol. (2017) 18:536–42. doi: 10.3348/kjr.2017.18.3.536
60. Kitano D, Yonezawa K, Iwae S, Sakakibara S. Internal jugular vein thrombosis and pulmonary thromboembolism after head and neck reconstructive surgery. J Plast Reconstr Aesthet Surg. (2021) 74:1239–45. doi: 10.1016/j.bjps.2020.11.007
61. Crawley MB, Sweeny L, Ravipati P, Heffelfinger R, Krein H, Luginbuh A, et al. Factors associated with free flap failures in head and neck reconstruction. Otolaryngol Head Neck Surg. (2019) 161:598–604. doi: 10.1177/0194599819860809
62. Clavien PA, Barkun J, de Oliveira ML, Vauthey JN, Dindo D, Schulick RD, et al. The Clavien-Dindo classification of surgical complications: five-year experience. Ann Surg. (2009) 250:187–96. doi: 10.1097/SLA.0b013e3181b13ca2
63. Ota Y, Moore AG, Spector ME, Casper K, Stucken C, Malloy K, et al. Prediction of wound failure in patients with head and neck cancer treated with free flap reconstruction: utility of CT perfusion and MR perfusion in the early postoperative period. AJNR Am J Neuroradiol. (2022) 43:585–91. doi: 10.3174/ajnr.A7458
64. Thiem DGE, Römer P, Blatt S, Al-Nawas B, Kämmerer PW. New approach to the old challenge of free flap monitoring—Hyperspectral imaging outperforms clinical assessment by earlier detection of perfusion failure. J Pers Med. (2021) 14:(11):1101. doi: 10.3390/jpm11111101
65. Dakpé S, Colin E, Bettoni J, Davrou J, Diouf M, Devauchelle B, et al. Intraosseous microdialysis for bone free flap monitoring in head and neck reconstructive surgery: A prospective pilot study. Microsurgery. (2020) 40:315–23. doi: 10.1002/micr.30529
66. Kimura M, Takada K, Ishibashi K, Ohto H, Shibata A, Yamada H, et al. Quantitative analysis of chronological changes in the volume of flaps used for reconstruction of oral cavity defects. J Oral Sci. (2017) 59:499–503. doi: 10.2334/josnusd.16-0683
67. Razavi CR, Hostetter J, Shukla A, Cheng Z, Aygun N, Boahene K, et al. Predictors of free flap volume loss in nonosseous reconstruction of head and neck oncologic defects. Ear Nose Throat J. (2022) 101:48–53. doi: 10.1177/0145561320938903
68. Strohl MP, Junn JC, House AE, Heaton CM, Seth R, Park AM, et al. Long-term stability of vascularized adipofascial flaps in facial reconstruction. Facial Plast Surg Aesthet Med. (2020) 22:262–7. doi: 10.1089/fpsam.2019.0015
69. Sakamoto Y, Takahara T, Ota Y, Aoki T, Yamazaki H, Otsuru M, et al. MRI analysis of chronological changes in free-flap volume in head and neck reconstruction by volumetry. Tokai J Exp Clin Med. (2014) 39:44–50.
70. Salgado CJ, Smith A, Kim S, Higgins J, Behnam A, Herrera HR, et al. Effects of late loss of arterial inflow on free flap survival. J Reconstr Microsurg. (2002) 18:579–84. doi: 10.1055/s-2002-35095
71. Versluis B, Tuinder S, Boetes C, Van Der Hulst R, Lataster A, Van Mulken T, et al. Equilibrium-phase high spatial resolution contrast-enhanced MR angiography at 1.5T in preoperative imaging for perforator flap breast reconstruction. PloS One. (2013) 8:e71286. doi: 10.1371/journal.pone.0071286
72. Hudgins PA. Flap reconstruction in the head and neck: expected appearance, complications, and recurrent disease. Semin Ultrasound CT MR. (2002) 23:492–500. doi: 10.1016/S0887-2171(02)90039-9
73. Glastonbury CM, van Zante A, Knott PD. Ossification of the vascular pedicle in microsurgical fibular free flap reconstruction of the head and neck. AJNR Am J Neuroradiol. (2014) 35:1965–9. doi: 10.3174/ajnr.A3979
74. Wood CB, Rohde SL, Sinard RJ, Mannion K, Bigcas J-LM. Incidence of pedicle ossification in osseous free flap reconstruction in the head and neck. Oral Oncol. (2020) 103:104611. doi: 10.1016/j.oraloncology.2020.104611
75. Shokri T, Stahl LE, Kanekar SG, Goyal N. Osseous changes over time in free fibular flap reconstruction. Laryngoscope. (2019) 129:1113–6. doi: 10.1002/lary.27337
76. Witjes MJH, Schepers RH, Kraeima J. Impact of 3D virtual planning on reconstruction of mandibular and maxillary surgical defects in head and neck oncology. Curr Opin Otolaryngol Head Neck Surg. (2018) 26:108–14. doi: 10.1097/MOO.0000000000000437
77. Ahn D, Lee GJ, Sohn JH. Reconstruction of oral cavity defect using versatile buccinator myomucosal flaps in the treatment of cT2-3, N0 oral cavity squamous cell carcinoma: Feasibility, morbidity, and functional/oncological outcomes. Oral Oncol. (2017) 75:95–9. doi: 10.1016/j.oraloncology.2017.11.007
78. Kumar R, Griffin M, Adigbli G, Kalavrezos N, Butler PEM. Lipotransfer for radiation-induced skin fibrosis. Br J Surg. (2016) 103:950–61. doi: 10.1002/bjs.10180
79. Iwami K, Momota H, Fujii M, Natsume A, Yagi S, Toriyama K, et al. Anaplastic meningioma with rapid growth after omental flap transposition: a case report and experimental study. Brain Tumor Pathol. (2015) 32:137–44. doi: 10.1007/s10014-014-0190-4
80. Mohamed ASR, Wong AJ, Fuller CD, Kamal M, Gunn GB, Phan J, et al. Patterns of locoregional failure following post-operative intensity-modulated radiotherapy to oral cavity cancer: quantitative spatial and dosimetric analysis using a deformable image registration workflow. Radiat Oncol. (2017) 12:129. doi: 10.1186/s13014-017-0868-y
81. Marchetti C, Pizzigallo A, Cipriani R, Campobassi A, Badiali G. Does microvascular free flap reconstruction in oral squamous cell carcinoma improve patient survival? Otolaryngol Head Neck Surg. (2008) 139:775–80. doi: 10.1016/j.otohns.2008.08.019
82. Hudgins PA, Burson JG, Gussack GS, Grist WJ. CT and MR appearance of recurrent Malignant head and neck neoplasms after resection and flap reconstruction. AJNR Am J Neuroradiol. (1994) 15:1689–94.
83. Offiah C, Hall E. Post-treatment imaging appearances in head and neck cancer patients. Clin Radiol. (2011) 66:13–24. doi: 10.1016/j.crad.2010.09.004
84. Abdel Razek AAK, Saleh GA, Denever AT, Mukherji SK. Preimaging and postimaging of graft and flap in head and neck reconstruction. Magn Reson Imaging Clin N Am. (2022) 30:121–33. doi: 10.1016/j.mric.2021.07.004
85. Kim ES, Yoon DY, Moon JY, Baek S, Han YM, Seo YL, et al. Detection of loco-regional recurrence in Malignant head and neck tumors: a comparison of CT, MRI, and FDG PET-CT. Acta Radiol. (2019) 60:186–95. doi: 10.1177/0284185118776504
86. Thoeny HC. Diffusion-weighted MRI in head and neck radiology: applications in oncology. Cancer Imaging. (2011) 10:209–14. doi: 10.1102/1470-7330.2010.0030
87. Ravanelli M, Grammatica A, Squassina G, Bertagna F, Albano D, Lancini D, et al. Value of [18F]FDG PET-CT in the follow-up of surgically treated oral tongue squamous cell carcinoma: single centre cohort analysis on 87 patients. Nucl Med Rev. (2021) 24:58–62. doi: 10.5603/NMR.2021.0016
88. Guzmán Pérez-Carrillo GJ, Ivanidze J. PET/CT and PET/MR imaging of the post-treatment head and neck: traps and tips. Neuroimaging Clin N Am. (2022) 32:111–32. doi: 10.1016/j.nic.2021.09.003
89. Tarsitano A, Vietti MV, Cipriani R, Marchetti C. Functional results of microvascular reconstruction after hemiglossectomy: free anterolateral thigh flap versus free forearm flap. Acta Otorhinolaryngol Ital. (2013) 33:374–9.
90. Shin YS, Koh YW, Kim S-H, Jeong JH, Ahn S, Hong HJ, et al. Radiotherapy deteriorates postoperative functional outcome after partial glossectomy with free flap reconstruction. J Oral Maxillofac Surg. (2012) 70:216–20. doi: 10.1016/j.joms.2011.04.014
91. Choi S, Schwartz DL, Farwell DG, Austin-Seymour M, Futran N. Radiation therapy does not impact local complication rates after free flap reconstruction for head and neck cancer. Arch Otolaryngol Head Neck Surg. (2004) 130:1308–12. doi: 10.1001/archotol.130.11.1308
92. Ross DA, Hundal JS, Son YH, Ariyan S, Shin J, Lowlicht R, et al. Microsurgical free flap reconstruction outcomes in head and neck cancer patients after surgical extirpation and intraoperative brachytherapy. Laryngoscope. (2004) 114:1170–6. doi: 10.1097/00005537-200407000-00007
93. Ang KK, Trotti A, Brown BW, Garden AS, Foote RL, Morrison WH, et al. Randomized trial addressing risk features and time factors of surgery plus radiotherapy in advanced head-and-neck cancer. Int J Radiat Oncol Biol Phys. (2001) 51:571–8. doi: 10.1016/S0360-3016(01)01690-X
94. Langendijk JA, Slotman BJ, van der Waal I, Doornaert P, Berkof J, Leemans CR. Risk-group definition by recursive partitioning analysis of patients with squamous cell head and neck carcinoma treated with surgery and postoperative radiotherapy. Cancer. (2005) 104:1408–17. doi: 10.1002/cncr.21340
95. Bernier J, Domenge C, Ozsahin M, Matuszewska K, Lefèbvre JL, Greiner RH, et al. Postoperative irradiation with or without concomitant chemotherapy for locally advanced head and neck cancer. N Engl J Med. (2004) 350:1945–52. doi: 10.1056/NEJMoa032641
96. Cooper JS, Pajak TF, Forastiere AA, Jacobs J, Campbell BH, Saxman SB, et al. Postoperative concurrent radiotherapy and chemotherapy for high-risk squamous-cell carcinoma of the head and neck. N Engl J Med. (2004) 350:1937–44. doi: 10.1056/NEJMoa032646
97. Koyfman SA, Ismaila N, Crook D, D'Cruz A, Rodriguez CP, Sher DJ, et al. Management of the neck in squamous cell carcinoma of the oral cavity and oropharynx: ASCO clinical practice guideline. J Clin Oncol. (2019) 37:1753–74. doi: 10.1200/JCO.18.01921
98. Noble AR, Greskovich JF, Han J, Reddy CA, Nwizu TI, Khan MF, et al. Risk factors associated with disease recurrence in patients with stage III/IV squamous cell carcinoma of the oral cavity treated with surgery and postoperative radiotherapy. Anticancer Res. (2016) 36:785–92.
99. Chen C-C, Lin J-C, Chen K-W. Lymph node ratio as a prognostic factor in head and neck cancer patients. Radiat Oncol. (2015) 10:181. doi: 10.1186/s13014-015-0490-9
100. Mermod M, Tolstonog G, Simon C, Monnier Y. Extracapsular spread in head and neck squamous cell carcinoma: A systematic review and meta-analysis. Oral Oncol. (2016) 62:60–71. doi: 10.1016/j.oraloncology.2016.10.003
101. Fan K-H, Wang H-M, Kang C-J, Lee LY, Huang SF, Lin CY, et al. Treatment results of postoperative radiotherapy on squamous cell carcinoma of the oral cavity: coexistence of multiple minor risk factors results in higher recurrence rates. Int J Radiat Oncol Biol Phys. (2010) 77:1024–9. doi: 10.1016/j.ijrobp.2009.06.064
102. Rosenthal DI, Mohamed ASR, Garden AS, Morrison WH, El-Naggar AK, Kamal M, et al. Final report of a prospective randomized trial to evaluate the dose-response relationship for postoperative radiation therapy and pathologic risk groups in patients with head and neck cancer. Int J Radiat Oncol Biol Phys. (2017) 98:1002–11. doi: 10.1016/j.ijrobp.2017.02.218
103. Lee CW, Dupré S, Marlborough F, Iqbal MS, Kelly C, Bashir MA, et al. Postoperative radiotherapy delay in head and neck cancer patients undergoing major resection and free flap reconstruction. J Plast Reconstr Aesthet Surg. (2022) 75:2084–9. doi: 10.1016/j.bjps.2022.02.038
104. Evans M, Beasley M. Target delineation for postoperative treatment of head and neck cancer. Oral Oncol. (2018) 86:288–95. doi: 10.1016/j.oraloncology.2018.08.011
105. Ferris RL, Flamand Y, Weinstein GS, Weinstein GS, Quon H, Mehra R, et al. Transoral robotic surgical resection followed by randomization to low- or standard-dose IMRT in resectable p16+ locally advanced oropharynx cancer: A trial of the ECOG-ACRIN Cancer Research Group (E3311). JCO. (2020) 38:6500–0. doi: 10.1200/JCO.2020.38.15_suppl.6500
106. Daly ME, Le Q-T, Kozak MM, Maxim PG, Murphy JD, Hsu A, et al. Intensity-modulated radiotherapy for oral cavity squamous cell carcinoma: patterns of failure and predictors of local control. Int J Radiat Oncol Biol Phys. (2011) 80:1412–22. doi: 10.1016/j.ijrobp.2010.04.031
107. Metcalfe E, Aspin L, Speight R, Ermiş E, Ramasamy S, Cardale K, et al. Postoperative (Chemo)Radiotherapy for oral cavity squamous cell carcinomas: outcomes and patterns of failure. Clin Oncol (R Coll Radiol). (2017) 29:51–9. doi: 10.1016/j.clon.2016.09.008
108. Chao KSC, Ozyigit G, Tran BN, Cengiz M, Dempsey JF, Low DA. Patterns of failure in patients receiving definitive and postoperative IMRT for head-and-neck cancer. Int J Radiat Oncol Biol Phys. (2003) 55:312–21. doi: 10.1016/S0360-3016(02)03940-8
109. Chen AM, Farwell DG, Luu Q, Chen LM, Vijayakumar S, Purdy JA. Marginal misses after postoperative intensity-modulated radiotherapy for head and neck cancer. Int J Radiat Oncol Biol Phys. (2011) 80:1423–9. doi: 10.1016/j.ijrobp.2010.04.011
110. Chan AK, Huang SH, Le LW, Yu E, Dawson LA, Kim JJ, et al. Postoperative intensity-modulated radiotherapy following surgery for oral cavity squamous cell carcinoma: patterns of failure. Oral Oncol. (2013) 49:255–60. doi: 10.1016/j.oraloncology.2012.09.006
111. Geretschläger A, Bojaxhiu B, Crowe S, Arnold A, Manser P, Hallermann W, et al. Outcome and patterns of failure after postoperative intensity modulated radiotherapy for locally advanced or high-risk oral cavity squamous cell carcinoma. Radiat Oncol. (2012) 7:175. doi: 10.1186/1748-717X-7-175
112. Gérard M, Le Guevelou J, Jacksic N, Lequesne J, Bastit V, Géry B, et al. Postoperative radiotherapy after flap reconstructive surgery in patients with head and neck cancer: A retrospective monocentric study with flap delineation to assess toxicity and relapse. Cancer Radiother. (2020) 24:851–9. doi: 10.1016/j.canrad.2020.06.024
113. Gregoire V, Eisbruch A, Hamoir M, Levendag P. Proposal for the delineation of the nodal CTV in the node-positive and the post-operative neck. Radiotherapy Oncol. (2006) 79(1):15–20. doi: 10.1016/j.radonc.2006.03.009
114. Margalit DN, Sacco AG, Cooper JS, Ridge JA, Bakst RL, Beadle BM, et al. Systematic review of postoperative therapy for resected squamous cell carcinoma of the head and neck: Executive summary of the American Radium Society appropriate use criteria. Head Neck. (2021) 43:367–91. doi: 10.1002/hed.26490
115. Cho Y, Yoon HI, Lee IJ, Kim JW, Lee CG, Choi ECv, et al. Patterns of local recurrence after curative resection and reconstruction for oropharyngeal and oral cancers: Implications for postoperative radiotherapy target volumes. Head Neck. (2019) 41:3916–23. doi: 10.1002/hed.25928
116. Thariat J, Marcy P-Y, Lacout A, Ramus L, Girinsky T, Pointreau Y, et al. Radiotherapy and radiology: joint efforts for modern radiation planning and practice. Diagn Interv Imaging. (2012) 93:342–50. doi: 10.1016/j.diii.2012.02.004
117. Raghavan P, Vakharia K, Morales RE, Mukherjee S. Surgical free flaps and grafts in head and neck reconstruction: principles and postoperative imaging. Neuroimaging Clin N Am. (2022) 32:75–91. doi: 10.1016/j.nic.2021.09.002
118. Essig H, Rana M, Kokemueller H, von See C, Ruecker M, Tavassol F, et al. Pre-operative planning for mandibular reconstruction - a full digital planning workflow resulting in a patient specific reconstruction. Head Neck Oncol. (2011) 3:45. doi: 10.1186/1758-3284-3-45
119. Bittermann G, Scheifele C, Prokic V, Bhatt V, Henke M, Grosu AL, et al. Description of a method: computer generated virtual model for accurate localisation of tumour margins, standardised resection, and planning of radiation treatment in head & neck cancer surgery. J Craniomaxillofac Surg. (2013) 41:279–81.
120. Bittermann G, Ermer M, Voss P, Duttenhoefer F, Zimmerer R, Schmelzeisen R, et al. Comparison of virtual and titanium clip marking of tumour resection margins for improved radiation planning in head and neck cancer surgery. Int J Oral Maxillofac Surg. (2015) 44:1468–73. doi: 10.1016/j.ijom.2015.07.013
121. Evensen ME, Furre T, Malinen E, Løndalen AM, Dale E. Mucosa-sparing dose painting of head and neck cancer. Acta Oncol. (2022) 61:141–5. doi: 10.1080/0284186X.2021.2022200
122. Bittermann G, Wiedenmann N, Bunea A, Schwarz SJ, Grosu AL, Schmelzeisen R, et al. Clipping of tumour resection margins allows accurate target volume delineation in head and neck cancer adjuvant radiation therapy. Radiother Oncol. (2015) 116:82–6. doi: 10.1016/j.radonc.2015.04.025
123. Airoldi M, Garzaro M, Raimondo L, Pecorari G, Giordano C, Varetto A, et al. Functional and psychological evaluation after flap reconstruction plus radiotherapy in oral cancer. Head Neck. (2011) 33:458–68. doi: 10.1002/hed.21471
124. Higgins KM, Erovic BM, Ravi A, Yeung R, Lee JW, Yao C, et al. Volumetric changes of the anterolateral thigh free flap following adjuvant radiotherapy in total parotidectomy reconstruction. Laryngoscope. (2012) 122:767–72. doi: 10.1002/lary.22509
125. Haymerle G, Enzenhofer E, Lechner W, Stock M, Schratter-Sehn A, Vyskocil E, et al. The effect of adjuvant radiotherapy on radial forearm free flap volume after soft palate reconstruction in 13 patients. Clin Otolaryngol. (2018) 43:742–5. doi: 10.1111/coa.13042
126. Lilja M, Markkanen-Leppanen M, Viitasalo S, Saarilahti K, Lindford A, Lassus P, et al. Olfactory and gustatory functions after free flap reconstruction and radiotherapy for oral and pharyngeal cancer: a prospective follow-up study. Eur Arch Otorhinolaryngol. (2018) 275:959–66. doi: 10.1007/s00405-018-4883-x
127. Yamazaki M, Suzuki T, Hiraga C, Yoshida Y, Baba A, Saitou H, et al. Effect of postoperative radiotherapy for free flap volume changing after tongue reconstruction. Oral Radiol. (2021) 37(3):518–23. doi: 10.1007/s11282-020-00489-0
128. Chang LS, Wang H, Ahn HC, Lee TH, Tae K, Park SO. The impact of postoperative radiotherapy on dietary function of head and neck cancer patients after pharyngoesophageal reconstruction with free jejunal flap. J Clin Med. (2022) 11:2860. doi: 10.3390/jcm11102860
129. Zenke J, Alenazi A, Sommerfeld C, Pyne J, Idris S, Darwish A, et al. Long-term results of a pedicled and innervated sternocleidomastoid muscle flap to immediately reconstruct superficial parotidectomy defects. Facial Plast Surg Aesthet Med. (2020) 22:420–6. doi: 10.1089/fpsam.2020.0073
130. Bokhari WA, Wang SJ. Tongue reconstruction: recent advances. Curr Opin Otolaryngol Head Neck Surg. (2007) 15:202–7. doi: 10.1097/MOO.0b013e3281fbd406
131. Djabali EJ, Rotter J, Chheda NN, Amdur RJ, Hitchcock K, Mendenhall W, et al. Woody hardness classification impact on salvage laryngectomy functional outcomes. Am J Otolaryngol. (2021) 42:102877. doi: 10.1016/j.amjoto.2020.102877
132. Colbert SD, Mitchell DA, Brennan PA. Woody hardness - a novel classification for the radiotherapy-treated neck. Br J Oral Maxillofac Surg. (2015) 53:380–3. doi: 10.1016/j.bjoms.2015.02.019
133. Straub JM, New J, Hamilton CD, Lominska C, Shnayder Y, Thomas SM. Radiation-induced fibrosis: mechanisms and implications for therapy. J Cancer Res Clin Oncol. (2015) 141:1985–94. doi: 10.1007/s00432-015-1974-6
134. Ailianou A, Mundada P, De Perrot T, Pusztaszieri M, Poletti P-A, Becker M. MRI with DWI for the detection of posttreatment head and neck squamous cell carcinoma: why morphologic MRI criteria matter. AJNR Am J Neuroradiol. (2018) 39:748–55. doi: 10.3174/ajnr.A5548
135. Dziegielewski PT, Bernard S, Mendenhall WM, Hitchock KE, Parker Gibbs C, Wang J, et al. Osteoradionecrosis in osseous free flap reconstruction: Risk factors and treatment. Head Neck. (2020) 42:1928–38. doi: 10.1002/hed.26118
136. Gazyakan E, Wu C-W, Huang J-J, Engel H, Valerio IL, Cheng M-H. Minimizing osteoradionecrosis after mandibular reconstruction and radiation in advanced head and neck cancer patients. J Surg Oncol. (2016) 114:399–404. doi: 10.1002/jso.v114.4
137. Falek S, Regmi R, Herault J, Dore M, Vela A, Dutheil P, et al. Dental management in head and neck cancers: from intensity-modulated radiotherapy with photons to proton therapy. Support Care Cancer. (2022) 30:8377–89. doi: 10.1007/s00520-022-07076-5
138. Avkshtol V, Handorf EA, Ridge JA, Leachman BK, Liu JC, Bauman J, et al. Examining adjuvant radiation dose in head and neck squamous cell carcinoma. Head Neck. (2019) 41:2133–42. doi: 10.1002/hed.25680
139. Yi CR, Oh TM, Jeong WS, Choi J-W, Oh TS. Quantitative analysis of the impact of radiotherapy on facial nerve repair with sural nerve grafting after parotid gland surgery. J Craniomaxillofac Surg. (2020) 48:724–32. doi: 10.1016/j.jcms.2020.05.012
140. Tasch C, Pattiss A, Maier S, Lanthaler M, Pierer G. Free flap outcome in irradiated recipient sites: A systematic review and meta-analysis. Plast Reconstr Surg Glob Open. (2022) 10:e4216. doi: 10.1097/GOX.0000000000004216
141. le Nobel GJ, Higgins KM, Enepekides DJ. Predictors of complications of free flap reconstruction in head and neck surgery: Analysis of 304 free flap reconstruction procedures. Laryngoscope. (2012) 122:1014–9. doi: 10.1002/lary.22454
142. Hohlweg-Majert B, Ristow O, Gust K, Kehl V, Wolff K-D, Pigorsch S. Impact of radiotherapy on microsurgical reconstruction of the head and neck. J Cancer Res Clin Oncol. (2012) 138:1799–811. doi: 10.1007/s00432-012-1263-6
143. Eldridge RC, Pugh SL, Trotti A, Hu K, Spencer S, Yom SS, et al. Changing functional status within 6 months posttreatment is prognostic of overall survival in patients with head and neck cancer: NRG Oncology Study. Head Neck. (2019) 41:3924–32. doi: 10.1002/hed.25922
144. Graboyes EM, Garrett-Mayer E, Sharma AK, Lentsch EJ, Day TA. Adherence to National Comprehensive Cancer Network guidelines for time to initiation of postoperative radiation therapy for patients with head and neck cancer: NCCN Guidelines Time to Initiation PORT. Cancer. (2017) 123:2651–60. doi: 10.1002/cncr.30651
145. Bates JE, Hitchcock KE, Mendenhall WM, Dziegielewski PT, Amdur RJ. Comparing national practice versus standard guidelines for the use of adjuvant treatment following robotic surgery for oropharyngeal squamous cell carcinoma. Head Neck. (2020) 42:2602–6. doi: 10.1002/hed.26311
146. Graboyes EM, Zhan KY, Garrett-Mayer E, Lentsch EJ, Sharma AK, Day TA. Effect of postoperative radiotherapy on survival for surgically managed pT3N0 and pT4aN0 laryngeal cancer: Analysis of the National Cancer Data Base. Cancer. (2017) 123:2248–57. doi: 10.1002/cncr.30586
Keywords: head and neck cancer, reconstructive surgery/flap, radiotherapy, delineation, target volumes, relapse, functional outcomes, guidelines
Citation: Thariat J, Carsuzaa F, Beddok A, Deneuve S, Marcy P-Y, Merlotti A, Dejean C and Devauchelle B (2024) Reconstructive flap surgery in head and neck cancer patients: an interdisciplinary view of the challenges encountered by radiation oncologists in postoperative radiotherapy. Front. Oncol. 14:1379861. doi: 10.3389/fonc.2024.1379861
Received: 31 January 2024; Accepted: 21 March 2024;
Published: 11 April 2024.
Edited by:
Guy Andry, Université libre de Bruxelles, BelgiumReviewed by:
Allen Lee Feng, Massachusetts Eye & Ear Infirmary and Harvard Medical School, United StatesLentiona Basiari, University Hospital of Ioannina, Greece
Copyright © 2024 Thariat, Carsuzaa, Beddok, Deneuve, Marcy, Merlotti, Dejean and Devauchelle. This is an open-access article distributed under the terms of the Creative Commons Attribution License (CC BY). The use, distribution or reproduction in other forums is permitted, provided the original author(s) and the copyright owner(s) are credited and that the original publication in this journal is cited, in accordance with accepted academic practice. No use, distribution or reproduction is permitted which does not comply with these terms.
*Correspondence: Juliette Thariat, jthariat@gmail.com