- Department of Cell Stress Biology, Roswell Park Comprehensive Cancer Center, Buffalo, New York, NY, United States
The aryl hydrocarbon receptor (AhR) is a ligand-activated transcription factor that is widely recognized to play important, but complex, modulatory roles in a variety of tumor types. In this review, we comprehensively summarize the increasingly controversial role of AhR as a tumor regulator and the mechanisms by which it alters tumor progression based on the cancer cell type. Finally, we discuss new and emerging strategies to therapeutically modulate AhR, focusing on novel agents that hold promise in current human clinical trials as well as existing FDA-approved drugs that could potentially be repurposed for cancer therapy.
Introduction to AhR signaling
The aryl hydrocarbon receptor (AhR) is a ligand-activated transcription factor that was identified in 1976 as the receptor mediating the effects of 2,3,7,8-tetrachlorodibenzo-p-dioxin (TCDD) (1). AhR is a member of the basic helix-loop-helix PER-ARNT-SIM (bHLH-PAS) family of transcription factors, and as such, coordinates transcriptional activity in response to environmental signals. The AhR protein is comprised of the N-terminal bHLH DNA-binding domain, two PAS domains for dimerization (with the PAS-B domain also responsible for ligand binding), and a C-terminal transactivation domain (2) (see Figure 1).
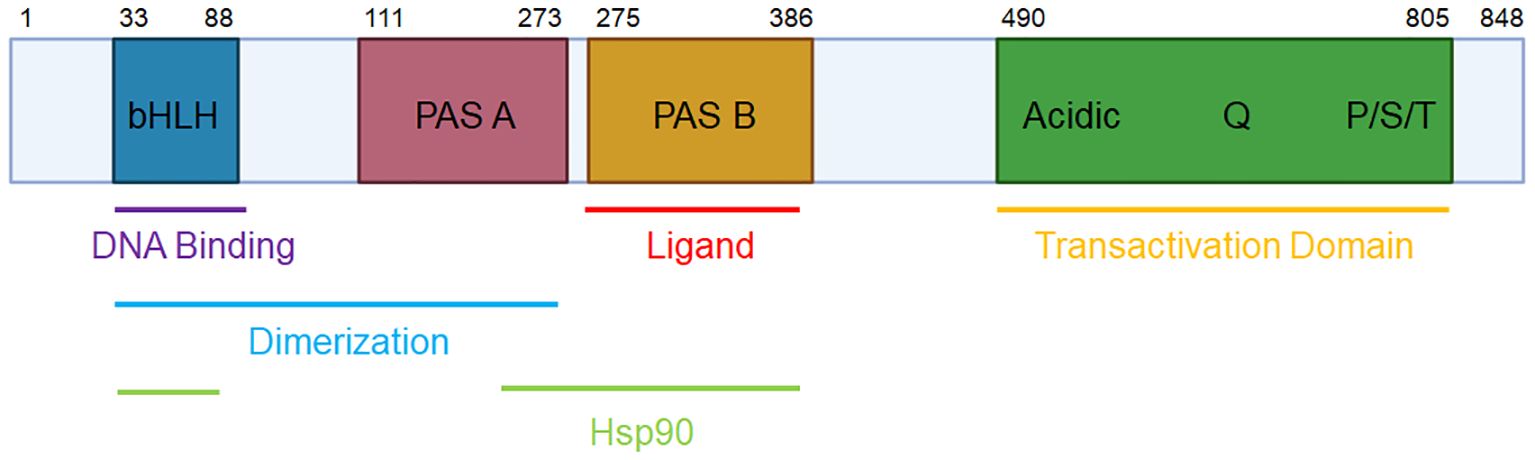
Figure 1 The domains of human AhR. The human AhR protein consists of an N-terminal basic helix-loop-helix (bHLH) domain for DNA binding, protein dimerization, and Hsp90 binding; a PAS-A domain for protein dimerization and Hsp90 binding; a PAS-B domain for ligand and Hsp90 binding; and a C-terminal transactivation domain.
AhR is normally sequestered in the cytoplasm where it is bound to chaperone proteins, notably heat shock protein 90 (Hsp90), X-associated protein 2 (XAP2), p23, and Src (3). Upon ligand binding, AhR translocates into the nucleus and forms a heterodimer with its canonical binding partner, AhR nuclear translocator (ARNT). The AhR-ARNT complex binds to xenobiotic response elements (XREs) within the DNA, leading to the induction of classical targets comprising the “AhR gene battery,” including cytochrome P450 enzymes CYP1A1 and CYP1A2, ROS scavenger NQO1, poly(ADP-ribose) polymerase TIPARP, and the AhR repressor AHRR, among many others (4). AhR signaling is regulated via several mechanisms, including control of ligand availability by CYP enzymes (5). In a negative feedback loop, AhRR binds to ARNT, limiting AhR/ARNT transcriptional activity (6). Finally, AhR is degraded by the 26S proteasome, which is also triggered by AhR activation (7) (see Figure 2).
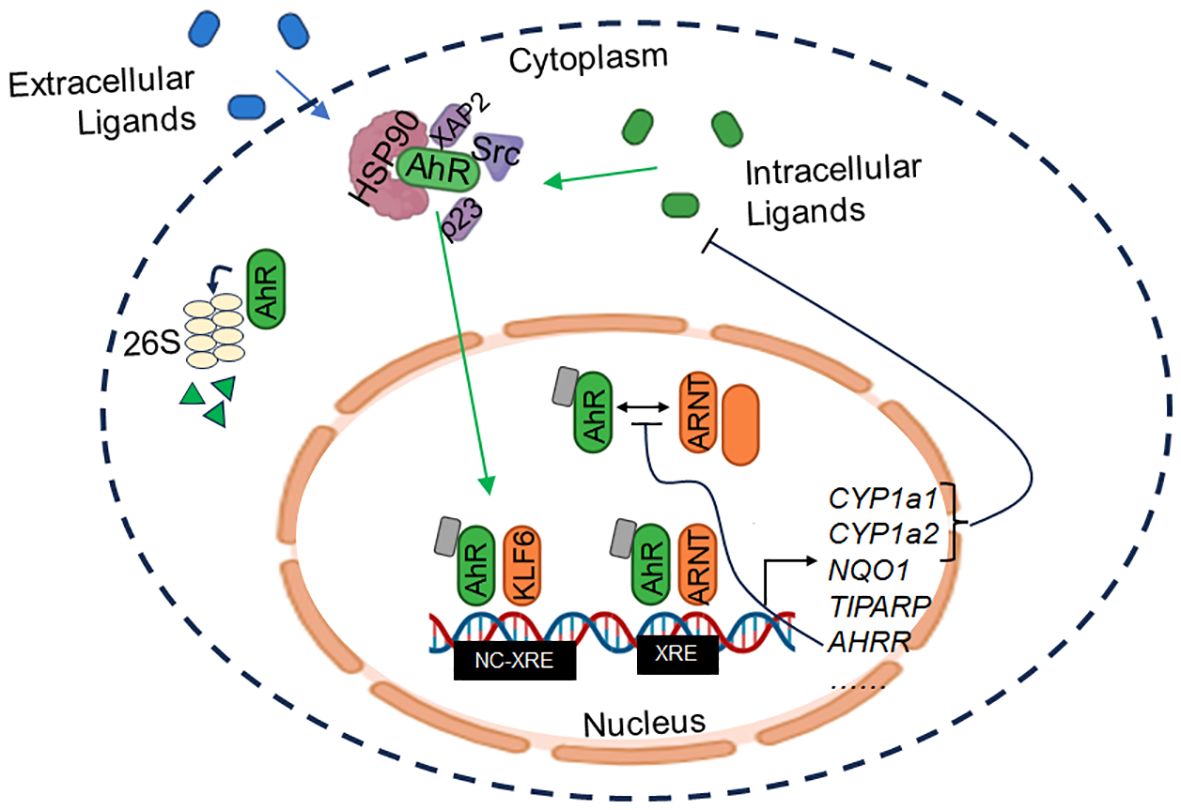
Figure 2 AhR signaling. AhR is normally sequestered in inactive form in the cytoplasm through interaction with chaperones (HSP90, XAP2, p23, and Src). Upon binding of exogenous or endogenous ligands, AhR dissociates from the complex and translocates to the nucleus where it dimerizes with ARNT or KLF6, among others, to induce transcription of target genes. XRE = xenobiotic response element; NC-XRE = non-canonical XRE.
In recent years, it has become well-appreciated that AhR can bind a multitude of exogenous and endogenous ligands, heterodimerize with several non-canonical binding partners, and regulate diverse transcriptional programs. While xenobiotics such as TCDD (1) and polycyclic aromatic hydrocarbons (PAHs) (8) represent prototypical AhR agonists, an ever-growing list of exogenous and endogenous ligands have been described. In particular, tryptophan derivatives including 2-(1’H-indole-3’-carbonyl)-thiazole-4-carboxylic acid methyl ester (ITE) (9) as well as kynurenine (10) are among the most well-characterized endogenous AhR ligands. Accordingly, differences in ligand binding may differentially modulate AhR functionality (11). Indeed, AhR interacts with a diverse set of binding partners, such as RelA (12), estrogen receptor α (ERα) (13), Kruppel-like factor 6 (KLF6) (14), among others, at both xenobiotic response elements (XRE) and non-canonical XRE (nc-XRE) elements within the DNA, resulting in distinct gene expression changes.
AHR-deficient murine models have provided critical insight into AhR’s endogenous functions, revealing its role in the immune system, hepatic growth and development, and fertility (15). AhR-null mice remain viable and fertile, making them ideal models to study cancer development and progression.
AhR in tumor biology
AhR is ubiquitously expressed and dysregulated in a wide range of cancer types. Its function as a tumor modulator is complex, as AhR can act as pro-tumorigenic or anti-tumorigenic factor depending on the cancer cell type, sometimes, with conflicting reports (Figure 3). Here, we summarize the current state of knowledge of the tumor modulatory roles of AhR based on varying cancer subsets.
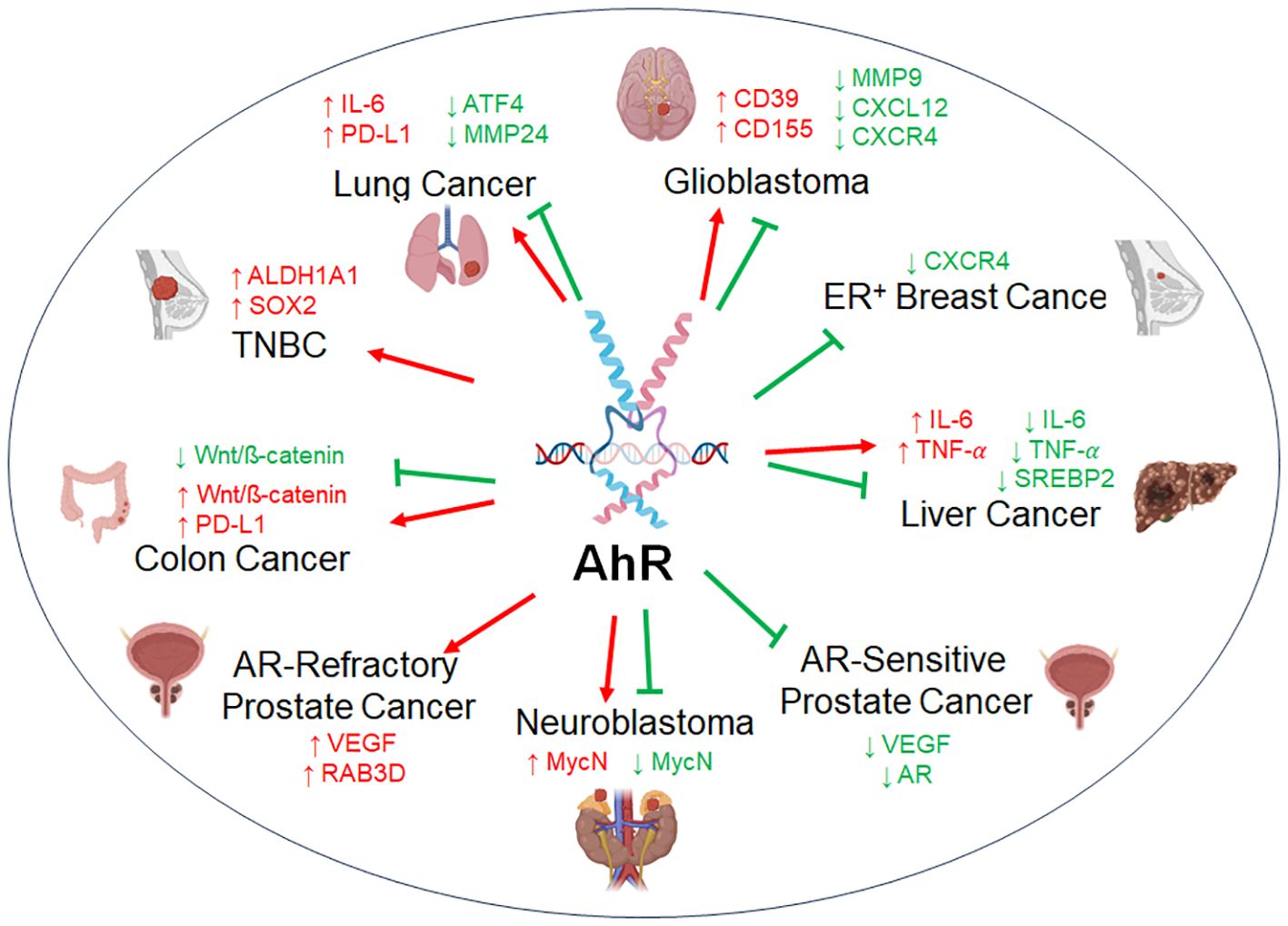
Figure 3 The dual role of AhR in tumor biology. AhR acts as a tumor promoter or as a tumor suppressor across representative cancer types. AhR regulates a variety of factors with either an oncogenic function (red) or anti-tumorigenic function (green), sometimes with opposing findings within the same subset of cancer.
Blood cancers
AhR is emerging as a tumor modulator in hematological malignancies, including acute myeloid leukemia (AML), multiple myeloma, chronic lymphocytic leukemia (CLL), and lymphomas, where its precise roles are just beginning to be understood. In AML, some reports suggest a tumor-promoting function of AhR, with high AhR expression and constitutive activity observed in AML patients (16). Scoville et al. (16) found that AML cells produce soluble ligands that activate AhR signaling in natural killer (NK) cells, resulting in the upregulation of miR-29b that impairs NK cell function. Consequently, AhR inhibition re-sensitizes AML cells to NK cell-induced cytotoxicity (16). On the contrary, other studies have shown reduced AhR signaling in AML cells, with AhR promoting AML differentiation, suppressing leukemic burden, and modulating AML resistance to bromodomain inhibitors (17). These discrepancies may be explained by differential ligands used among the studies; however, more work is needed to clarify how AhR alters AML disease progression.
Our group recently identified AhR as a poor prognostic factor in multiple myeloma patients that positively regulates the polyamine biosynthesis through transcriptional regulation of key players ornithine decarboxylase 1 (ODC1) and antizyme inhibitor 1 (AZIN1), and supports multiple myeloma cell proliferation (18). Subsequent corroborative studies by Hughes et al. (19) have demonstrated that AhR antagonism suppresses multiple myeloma cell viability, alters immune surface markers, and sensitizes multiple myeloma to NK cell cytotoxicity.
In CLL, microarray analysis revealed high AHR mRNA and target genes expression relative to other human B-cell lineage cancers, suggesting an oncogenic role for AhR in the disease (20). Consistently, CLL cells have been found to express indoleamine 2,3-dioxygenase 1 (IDO1) (21), which converts tryptophan to kynurenine, a ligand for AhR. IDO1-mediated kynurenine production rescues CLL cells from venetoclax-induced apoptosis and upregulates the pro-survival Mcl-1 in an AhR-dependent manner (21). Additionally, it was shown that the AhR-activating enzyme, IL4l1, is highly enriched in tumor-supportive monocytes in the Eµ-TCL1 mouse model of CLL and promotes CLL tumor progression (22). Thus, these findings imply that targeting AhR in the CLL tumor microenvironment could be an attractive therapeutic approach.
Studies in B and T-cell lymphomas have pointed to an oncogenic role for AhR. A recent report showed that AhR and its activating enzymes, IDO1 and tryptophan 2,3- dioxygenase (TDO), were highly expressed in diffuse large B-cell lymphoma (DLBCL) patient samples and were inversely correlated with patient survival (23). AhR localizes to the nucleus of DLBCL cell lines and drives expression of the germinal center oncogenes, MEF2B and BCL6 (24). These studies raise the question of how endogenous ligands generated by IDO1 and TDO could modulate AhR activity in lymphomas.
Breast cancer
Studies in breast cancer have revealed complex tumor regulatory functions for AhR. AhR is overexpressed and constitutively active in breast cancer (25), where it is thought to have varying effects depending on the ligand and cell type. It is well-established that there is an inhibitory crosstalk between AhR and estrogen receptor (ER) pathways, the mechanisms of which are described in great detail elsewhere (26) and also mentioned below. Consequently, ER status may influence the pro- or anti-tumorigenicity of AhR.
In triple-negative breast cancer – characterized by lack of expression of ER, progesterone receptor (PR), and lack of human epidermal growth factor receptor 2 (HER2) overexpression – TDO is particularly elevated and drives AhR activity to promote migration, anoikis resistance, and tumor metastasis (25, 27). Goode et al. (28) showed that shRNA-mediated depletion of AhR attenuates tumor growth in a xenograft human triple negative breast cancer (TNBC) MDA-MB-231 murine model. Stanford et al. (29) determined that AhR controls cancer stemness in human TNBC Hs578T cells, as AhR knockdown decreases tumor sphere formation and in vivo tumor growth via downregulation of ALDH1A1 and SOX2. While these reports provide evidence that AhR plays an oncogenic role in triple-negative breast cancer, other studies describe opposite results. siRNA-mediated AhR knockdown has been found to increase MDA-MB-231 invasion (30). These discrepancies are further exacerbated by conflicting results obtained with AhR ligands that suggest AhR acts a tumor suppressor in triple-negative breast cancer. A wide array of AhR agonists including TCDD (31), 6-methyl-1,3,8-trichlorodibenzo-furan (MCDF) (31), and omeprazole (30) have repressed viability, proliferation, invasion, and/or metastasis in MDA-MB-231 or MDA-MB-468 cells in an AhR-dependent manner. These findings suggest that ligand-mediated activation of AhR in TNBC has differing effects than genetic AhR manipulation. This highlights the complexity of AhR functions in triple-negative breast cancer and necessitate further work to understand these differences.
In breast cancer subtypes where ER is positively expressed, AhR has been described to exert tumor suppressive functions. Köhle et al. found that expression of a constitutively active mutant of AhR impairs the estrogen-dependent growth of MCF-7 cells (32). Consistent with these data, numerous reports have shown that AhR ligands have anti-tumorigenic effects in ER+ breast cancer cells. For example, in MCF-7 cells, TCDD counteracts estrogen-mediated proliferation and G1/S phase cell cycle progression and suppresses xenograft tumor growth in vivo (32, 33). Thus, there is consistent evidence from multiple laboratories that AhR is tumor suppressive in ER+ breast cancer cells. While this has generated efforts to therapeutically modulate AhR in ER+ patients, clinical trials have thus far not yielded success.
Colon cancer
An increasing multitude of reports suggest a tumor regulatory role for AhR in colorectal cancer. A study by Kawajiri et al. (34) detected low AhR protein levels in human cecal cancer specimens, and determined that AHR-deficient mice harbor more cecal tumors than wild-type mice. Moreover, in the APCMin/+ model of familial adenomatous polyposis, supplementation of the AhR ligands, indole-3-carbinol (I3C) and 3,3’-diindolylmethane (DIM), in the diet significantly delayed intestinal carcinogenesis (34). Other groups have corroborated a tumor suppressive function for AhR in colon cancer. Deletion of AHR in the APCS580/+; KRASG12D/+ mouse model of colorectal cancer promotes proliferation and tumor growth and decreases mouse survival rate (35). At the same time, various other publications suggest a tumor promoting role for AhR in colon cancer. Recently, Miyazaki et al. (36) found that patient-derived colon cancer spheroids express high levels of TDO2 and kynurenine. TDO2 promotes metastasis of colon cancer cells to the liver, upregulates programmed death ligand 1 (PD-L1) and suppresses immune responses, and maintains Wnt signaling in an AhR-dependent manner (36). Zhang et al. (37) showed that IDO1 expression in colon cancer cells stalls T cell proliferation. In a model of chronic colitis-associated cancer, IDO1-depleted mice have smaller and fewer tumors, reduced infiltrating regulatory T cells as well as increased CD8+ T cell abundance that is reversed with supplementation of kynurenine (37). Interestingly, gut microbiota such as Fusobacterium nucleatum produce formate, which drives metastatic dissemination, stemness, and increased Th17 cell infiltration via AhR signaling (38). Altogether, these findings reveal the complexity of AhR as a tumor modulator in colon cancer. While many in vivo studies suggest a tumor suppressive role, in vitro studies with ligands have generated opposing findings. This may be partly explained by the fact that tumor development in an AHR-deficient mouse model is distinct from AhR gain during tumorigenesis. Investigation into how the gut microbiome alters colorectal cancer progression is an emerging topic and further study will provide valuable insight into this research area.
Esophageal and stomach cancers
In upper gastrointestinal tract tumors of the esophagus and stomach, a growing body of literature addresses the role for AhR in cancer progression. In esophageal cancer, various reports suggest AhR acts as a tumor promoter. It has been shown that AhR is highly expressed in patient-derived esophageal squamous cell cancers and correlates with poor overall survival (39). Genetic AhR overexpression promotes esophageal carcinoma migration and invasion via upregulation of phosphorylated epidermal growth factor receptor (p-EGFR) and RhoA/ROCK1 (39). Studies in mice demonstrate that esophageal squamous cell carcinoma cells express TDO, which promotes tumor growth and induces monocyte differentiation into the pro-tumorigenic M2 macrophage via AhR (40). While collectively these studies support a role for AhR in promoting esophageal carcinogenesis, differing observations were noted with ligand AhR activation. Treatment of esophageal squamous cell carcinoma cells with the AhR ligand 3,3’-diindolylmethane (DIM) represses proliferation, invasion, migration, and tumor growth in xenograft models (39).
A pro-tumorigenic role for AhR has also been largely reported in gastric cancers. Transgenic mice expressing constitutively active AhR develop hamartomatous tumors in the glandular part of the stomach, which is accompanied by a downregulation of osteopontin (41). AhR is strongly expressed and localized in the nucleus of human gastric cancer tissues and cell lines (42). Genetic depletion of AhR suppresses viability, proliferation, migration, and invasion of gastric cancer cells, and in vivo administration of the AhR inhibitor, biseugenol, prevents gastric tumor growth, metastasis, and peritoneal dissemination (42).
Gynecologic malignancies
The role of AhR in gynecologic malignancies, including ovarian and uterine tumors, is just beginning to be understood. In human ovarian cancer tissues, immunohistochemistry analysis has shown positive AhR staining in a range of histological subtypes (43). IDO1 is also expressed by ovarian carcinomas where its levels are sustained by an autocrine AhR-IL-6-STAT3 signaling loop (44). In vivo experiments in mice have demonstrated that ovarian tumoral IDO1 mediates PD-1 upregulation on CD8+ T cells via AhR and causes infiltration of suppressive immune cells in the tumor microenvironment, augmenting ovarian tumor growth (45). These studies suggest a potential oncogenic role for AhR in ovarian cancer. However, treatment of ovarian cancer cell lines with AhR agonists appears to have varying effects depending on the ligand and cell type. For example, exposure of cells to the AhR agonist, ITE, decreases proliferation of OVCAR-3 cells and suppresses migration of SKOV-3 cells, but has no effect on the IOSE-385 cell line (43). As in breast cancer, these data may be partly explained by inhibitory crosstalk of the AhR signaling pathway with the estrogen receptor (ER) pathway (46). Further investigation is needed to clarify the tumorigenic role of AhR in ovarian cancers.
In endometrial cancer, mixed observations regarding the tumor regulatory role of AhR have been reported. Some studies suggest AhR plays an oncogenic role in endometrial cancer, as AhR is upregulated in human endometrial cancer lesions and its increased expression significantly correlates with higher tumor grade (47). Recent work by Li et al. (47) shows that genetic depletion of AHR reverts the growth, invasion, and motility induced by knockdown of the tumor suppressive transcription factor, nuclear factor 1-C (NF1C) in HHUA, HEC-6, and hEM cell lines, suggesting that NF1-C suppresses AhR-mediated tumorigenic functions. Ligand-induced AhR activation in endometrial cancer largely has anti-estrogenic and tumor suppressive effects, as seen in ER+ positive breast cancer. TCDD, B[a]P, and MCDF suppress estrogen-induced Ishikawa and ECC-1 cell proliferation. ITE reduces proliferation, migration, and in vivo tumor growth of AN3-CA, HCE-1B, and Ishikawa cells in an AhR-dependent manner (48). Again, these results highlight how AhR’s effects are strongly dependent on ligand and on cell type.
Head and neck cancers
An increasingly important role for AhR as a tumor promoter has been demonstrated in various head and neck cancers. AhR is constitutively active in head and neck squamous carcinoma cells where it promotes migration and invasive capability, and drives expression of IL-6 and growth factors, including amphiregulin (AREG), epiregulin (EREG), and platelet-derived growth factor A (PDGFA) (49). Recently, Frank et al. (50) showed that antibiotic treatment of a chemically-induced mouse model of oral squamous cell carcinoma reduced AhR activity, raising the possibility that Lactobacillus spp., enriched in this cancer type, activates AhR (50). Similarly, exposure of oral squamous cell carcinoma cells to the supernatants from Pseudomonas aeruginosa and Porphyromonas gingivalis, the latter commonly found in the oral cavity, induced AhR activity and augmented expression of ALDH1, a marker associated with chemoresistance (51). Consequently, AhR antagonism increases sensitivity to cisplatin, decreases tumor sphere formation, and reduces xenograft tumor growth in oral squamous cell carcinoma (51). AhR also modulates the tumor microenvironment of oral squamous cell carcinoma. Kenison et al. (52) showed that AhR deletion in murine orthotopic oral cancer cells prevents tumor growth and decreases expression of inhibitory immune checkpoints PD-L1, CD39, CTLA-4, PD-1, and Lag3 on multiple immune cell types.
Liver and pancreatic cancers
The tumor modulatory role of AhR is well-appreciated in liver cancers but remains controversial. Although most reports suggest a pro-tumorigenic function for AhR in hepatocellular carcinoma, some studies have documented a tumor suppressive role. It has been shown that AhR is highly expressed in human liver cancer tissues and cell lines (53). In the diethylnitrosamine (DEN)-induced mouse model of hepatic carcinogenesis, some groups found that constitutively active AhR expression promotes liver tumor formation (54). Conversely, another report showed that DEN-induced AhR-deficient mice have greater hepatic tumor incidence, increased proliferation, and higher IL-6 and TNF-α expression (55). Interestingly, there are also mixed findings regarding endogenous AhR activity in liver cancers. Some studies show the overexpression of TDO in human hepatocellular carcinoma patient tissues that drives cell growth, migration, invasion, and epithelial to mesenchymal transition (EMT) via the AhR pathway (56). However, a contrasting report found that TDO is downregulated in human hepatocellular carcinomas, inhibits cell proliferation, and represses tumor xenograft growth (57). Intriguingly, a recent study suggests that tryptophan metabolites produced by gut flora such as Lactobacillus reuteri attenuates expression of sterol regulatory element-binding protein 2 (SREBP2) and correspondingly prevents liver tumorigenesis in an AhR-dependent manner (58). In summary, these data illustrate that the tumor modulatory functions of AhR in liver cancers remain to be fully understood.
AhR has also been found to modulate pancreatic cancer progression. Early studies by Koliopanos et al. (59) demonstrated that AhR is overexpressed in human pancreatic cancer tissue specimens and cell lines. This work has shown that AhR activation by agonists – including TCDD, MCDF, and DIM – suppresses the growth of pancreatic cancer cells (59). However, more recent studies have suggested a tumor promoting role for AhR in pancreatic tumors. Human pancreatic cancers highly express IDO1 and TDO, which correlate with poor patient prognosis, and causes increased migration and invasion of cells and spheroids via kynurenine-mediated AhR activation (60). Consistently, metabolic profiling of pancreatic ductal adenocarcinoma patients revealed that higher kynurenine levels are associated with poorer overall survival of patients (61). Tumors expressing high levels of IDO1 are enriched for AHR pro-tumorigenic target genes, including NFE2L2 (Nrf2), SERPINB2, IL1b, IL6, and IL8 (61). Furthermore, AhR is expressed by tumor-associated macrophages where its activation by Lactobacillus-metabolized tryptophan metabolites drives immunosuppression and pancreatic tumor growth (62). Thus, while exogenous AhR activation appears to have a tumor suppressive effect, emerging evidence suggests that endogenous ligands may promote pancreatic cancer progression.
Lung cancer
An increasingly visible role for AhR as a regulator of lung cancer tumorigenicity is being appreciated, with most reports focused on non-small cell lung cancers. A solid body of data published by various laboratories supports a pro-tumorigenic role for AhR in lung cancer. Nuclear AhR expression is significantly associated with poor survival of patients with non-small cell lung carcinoma (63). Consistently, AhR inhibition or knockdown sensitizes non-small cell lung cancer to EGFR tyrosine kinase inhibitors in vitro and in vivo (63). Interestingly, Wang et al. (64) found that cigarette smoke upregulates PD-L1 via AhR in non-small cell lung cancer cells, and treatment with α-PD-L1 attenuates benzo-alpha-pyrene (BaP)-induced lung cancer in vivo. Accordingly, AhR inhibition with α-naphthoflavone (ANF) significantly enhances the efficacy of α-PD-L1 in lung cancer mouse models and prolongs the lifespan of mice (64). While these studies provide evidence that AhR acts as a tumor promoter, there are also reports suggesting a tumor suppressive function for AhR in lung cancer. Nothdurft et al. (65) determined that AHR depletion by shRNA augments invasiveness in vitro and metastatic capability of non-small cell lung cancer cells in vivo via regulation of the EMT pathway, ATF4 signaling, and MMP24 expression. AhR has also been shown to inhibit tumor growth and suppress the expansion of lung progenitor cells in a murine model of KRASG12D-induced non-small cell lung cancer (66). Thus, the role of AhR in lung cancer requires further study to fully understand its tumorigenic functions.
Nervous system tumors
AhR has been reported to play increasingly important roles in central and peripheral nervous system tumors. In the context of malignant gliomas, including glioblastoma, some groups have described AhR as a tumor promoter (10, 22, 67, 68), while others have shown that AhR acts as a tumor suppressor (69). Opitz et al. (10) originally established TDO-derived kynurenine produced by human gliomas as an oncometabolite that activates AhR, supports glioma cell survival and motility, and suppresses immune responses. AhR drives CD39 and CD155 expression in tumor-associated macrophages, thereby impairing T cell response in glioblastoma (68). Interestingly, R-2-hydroxyglutarate (R-2-HG), a metabolite that accumulates in isocitrate dehydrogenase (IDH)-mutant gliomas, enzymatically induces TDO in tumor-infiltrating myeloid cells, leading to AhR-dependent suppression of macrophage function and anti-tumor immunity (67). On the other hand, conflicting reports have demonstrated that kynurenine does not alter AhR activity or invasion of glioblastoma cells, and that the AhR antagonist, CH-223191, inhibits glioblastoma invasion in an AhR-independent manner in both AhR-expressing and AhR-silent cell lines (69). These studies show that AhR knockdown increases glioblastoma cell invasion and migration by induction of MMP9, CXCL12, and CXCR4 (69). Collectively, while these studies demonstrate the importance of AhR in malignant gliomas, more research is needed to understand its tumor modulatory roles that may shed light into contradictory data reported by different laboratories.
The role of AhR in neuroblastoma is largely understudied. Recent work from our laboratory suggests AhR acts as a tumor promoter in MYCN-amplified neuroblastoma (70). We have found that AhR transcriptional activity correlates with poor patient prognosis, positively regulates MycN, and represses differentiation of MYCN-amplified neuroblastoma cells by altering chromatin accessibility and modulating the retinoic acid receptor pathway (70). Accordingly, AhR antagonism with the AhR antagonist clofazimine (CLF) synergistically augments retinoic acid-induced differentiation (70), suggesting that modulation of AhR may be a potential and promising therapeutic approach for improving standard-of-care in this disease. We and others have also reported that AhR has a tumor suppressive role in non-MYCN-amplified neuroblastoma cells. Treatment of non-MYCN-amplified neuroblastoma cells with TCDD has been found to induce non-apoptotic cell death via an AhR-dependent mechanism (71). Wu et al. reported that AhR induces cell differentiation, reduces in vivo tumor burden, and downregulates MycN expression in the context of non-MYCN-amplified neuroblastoma (72, 73). Our work revealed that AhR suppresses cMYC in similar systems (70). Thus, current evidence lends support to the hypothesis that AhR has a dual tumor modulatory role depending on the neuroblastoma subtype.
Prostate cancer
In prostate cancer, the tumor modulatory effects of AhR appear to be context-dependent, differing based on androgen sensitivity or refractoriness. Multiple lines of evidence suggest AhR exerts tumor suppressive effects in androgen-sensitive prostate cancer, with some mixed reports. Studies in the transgenic, androgen-sensitive TRAMP model of prostate cancer show that AhR protects against prostate cancer development, as AHR+/+ TRAMP mice exhibit lower tumor formation than AHR-/- or AHR+/- TRAMP mice (74). In line with these findings, treatment of TRAMP mice with the selective AhR modulator, 6-methyl-1,3,8-trichlorodibenzo-furan (6-MCDF), which displays AhR agonist activity in prostate cell lines, inhibits prostate metastatic ability (75). In vitro experiments with AhR agonists in androgen-sensitive human prostate cancer cells have corroborated these murine studies. The AhR agonist, carbidopa, suppresses LNCaP proliferation and induces AhR-mediated proteasomal degradation of androgen receptor (AR) (76).
In androgen-refractory prostate cancer models, however, AhR has been suggested to act as a tumor promoter. AhR is highly expressed and constitutively active in androgen-independent (hormone-refractory) prostate cancer cells relative to androgen-sensitive prostate cancer cells (77). Immunohistochemistry of human prostate cancer tissues shows increasing AhR expression with tumor grade (77). AhR depletion or inhibition decreases cell proliferation, reduces AR protein levels, and inhibits AR target gene expression in androgen-independent cells (77). Further studies are needed to understand the crosstalk of the AhR and AR pathways and how they impact prostate cancer progression in both androgen-sensitive and androgen-refractory settings.
Skin cancers
Several studies imply a tumor regulatory role for AhR in melanoma. Genome-wide association studies have suggested that the AHR gene locus at 7p.21.1 confers susceptibility to cutaneous malignant melanoma (78). Recent work has shown that AhR drives resistance to BRAF inhibitors in melanoma, and that AhR inhibition with resveratrol and flavinoids re-sensitize melanoma to BRAF inhibition (79). Although these studies demonstrate an oncogenic role for AhR, there are also some reports indicating that AhR has a tumor suppressive role in melanoma. In particular, Contador-Troca et al. (80) found that AhR suppresses growth and metastasis of melanoma in vivo. Immunohistochemistry revealed that human melanoma patient samples express lower levels of AhR compared to human nevi (80). Interestingly, Bender et al. found that tryptophan-derived metabolites derived from Lactobacillus reuteri promote immune-checkpoint inhibitor efficacy in melanoma via activation of the AhR (81).
Urothelial tumors
Recent reports suggest a pro-tumorigenic role for AhR in urothelial cancers. Through analysis of whole-genome sequencing data, Vlaar et al. (82) identified recurrent in-frame deletions in exons 8 and 9 of AHR (AHRΔe8-9) in 10% of metastatic urothelial cancer patients. AHRΔe8-9 causes ligand-independent AhR activation and anchorage-independent growth of bladder organoids, acting as a novel driver mutation (82). In addition, Shi et al. (83) newly characterized AHRQ383H as an apolipoprotein B mRNA-editing enzyme catalytic polypeptide-like (APOBEC)-associated driver hotspot mutation in bladder cancer. AHRQ383H leads to higher sensitivity of AhR to ligand activation, greater AhR activity, and increased dependency of luminal bladder cancer cells on AhR for cell survival (82, 83). High expression of AhR and its target genes, CYP1A1 and CYP1A2, has been correlated with increased histological grade, tumor stage, and progression in muscle-invasive bladder cancer and upper urinary tract cancers (84).
AhR and immunity
Notably, AhR not only contributes to tumor growth and survival in a tumor-intrinsic fashion as highlighted in the chapters above, but multiple recent publications have revealed an AhR-dependent regulation of the tumor microenvironment, especially with regard to the immune system, to modulate tumor immune surveillance and allow escape from immunosuppression. A plethora of endogenous and exogenous AhR ligands can be found in the tumor microenvironment due to tumor cells’ related metabolism, the organismal microbiota metabolism, as well as dietary consumption and pollutants absorption. In most cases, AhR activation results in the alteration of the tumor-immune cell interactions, with suppression of anti-tumor functions and induction of tumor-permissive or tumor-promoting immune landscapes, as AhR has been shown to regulate the differentiation of multiple cell types in both the innate and the adaptive immune response compartments. Several recent reviews have extensively described the role of AhR in the regulation of the immune system during cancer progression (85–88); thus, here we will briefly summarize some of the recent key findings.
AhR activation has been found to promote the trans-differentiation of antigen-presenting cells (APCs), such as dendritic cells (DCs) and macrophages, toward a more tolerogenic or tumor-permissive phenotype, resulting in the generation of immune-suppressive regulatory T (Tregs) cells. Hezaveh et al. reported that the activation of AhR in tumor-associated macrophages (TAMs) from microbiome-produced tryptophan metabolites leads to immunosuppression in pancreatic ductal adenocarcinoma (PDAC) thus promoting tumor growth (62). In the same study, it was found that, conversely, AhR pharmacological antagonism, as well as AhR deletion from myeloid cells, results in improved T-cell tumor infiltration, leading to tumor suppression (62). Likewise, Sadik et al. revealed that interleukin 4-induced 1 (IL4I1) can generate AhR ligands such as indole metabolites and kynurenic acid, which result in the suppression of adaptive immunity (22). Interestingly, immune checkpoint inhibitors (ICIs) whose use has been incorporated in the standard-of-care of multiple cancer types, were found to induce IL4I1 and activate AhR, thus generating a negative feedback loop (22). Inadvertent AhR activation by chemotherapy, resulting in suppression of innate immunity responses (i.e interferon type I production) has been recently reported also in triple negative breast cancer (89).
Work by Wu et al. (90) revealed the presence of an ARID5A-IDO1-AhR axis that leads to chimeric antigen receptor T-cell (CAR-T) exhaustion and immune evasion in colorectal cancer. Additionally, the authors performed a pan-cancer analysis which revealed high AhR expression in tumor-infiltrating immune cells, where AhR antagonism with a novel compound (BAY-2416964 (91)), see section below) showed promising potential in restoring immune cell function and enhancing antigen-specific cytotoxic T cell responses (90).
The role that AhR plays in the development and cytotoxic capabilities of natural killer (NK) cells is still complex and controversial, with some groups proposing AhR activation to be critical for proper NK cells cytotoxicity and functions (92, 93) and others claiming that AhR activation dampens NK cells activity instead (94).
While more work needs to be done to address the discrepancies, the consensus seems to be that AhR antagonism would be beneficial to promote a tumor-suppressive microenvironment and relieve the immune-suppression often seen associated with the more aggressive tumors.
Therapeutic modulation of AhR in cancer
Given the well-recognized role of AhR as a regulator of tumor biology, many efforts are focused on developing therapeutic strategies to modulate AhR in cancer patients. Notably, this includes synthesizing novel AhR modulators as well as repurposing existing agents for the treatment of cancer patients. Certain agents, including the AhR agonist aminoflavone (AFP464) and the AhR antagonist StemRegenin-1 (SR1) (95), have been tested in clinical trials for breast neoplasms and solid tumors (NCT01015521, NCT00369200, NCT01233947) or hematological malignancies (NCT02765997), respectively. However, these trials were either withdrawn or terminated due to toxicity and poor side effect profiles. Currently, phase 1 clinical trials are ongoing for a novel AhR antagonist, BAY-2416964 (96), developed by Bayer and researchers at the German Cancer Research Center (DKFZ) for patients with advanced solid tumors (NCT04069026, NCT04999202). Preliminary reports presented at ASCO 2023 showed that BAY-2416964 seems to be well tolerated, suppresses AhR activation in vivo, and modulates immune functions. The authors noted that of 67 patients evaluable for response by RECIST, 22 (32.8%) had stable disease (91). Additionally, Ikena Oncology recently developed the novel AhR inhibitor, IK-175 (97), which is in phase 1 trials for urothelial cancers and solid tumors (NCT04200963 and NCT05472506) and received FDA Fast Track Designation. While not yet approved for cancer therapy, the AhR agonist tapinarof (98) is in clinical trials for atopic dermatitis and plaque psoriasis (NCT05142774, NCT05680740) and could potentially be tested for the treatment of dermatological malignancies in the future. These up-and-coming trials are promising and could represent the first AhR modulators to be clinically approved in patients for cancer therapy.
There are a growing number of FDA-approved drugs approved for other indications that have been recognized as AhR modulators, with demonstrated anti-tumor efficacy in pre-clinical studies (see Table 1). These include carbidopa (DOPA decarboxylase inhibitor) (76, 99, 100), dopamine (adrenergic receptor agonist) (101), flutamide (anti-androgen) (102), leflunomide (anti-rheumatic agent) (103, 104), nimodipine (anti-hypertensive agent) (30, 104), omeprazole (proton pump inhibitor) (30, 105, 106), raloxifene (estrogen modulator) (108), sorafenib (kinase inhibitor) (109), sulindac (nonsteroid anti-inflammatory) (30, 104, 110), transilast (anti-allergic agent) (107), and vemurafenib (BRAF inhibitor) (79). Our group previously identified clofazimine (CLF), an FDA-approved antibiotic approved for drug-resistant tuberculosis and lepromatous leprosy, as a novel AhR antagonist with anti-multiple myeloma and anti-neuroblastoma efficacy (18, 70). Importantly, the favorable safety profiles of these already FDA-approved agents warrant their testing in clinical trials for cancer patients, as they hold promise as anti-cancer drugs that could be rapidly translated into the clinic.
It is important to note, however, that AhR antagonism may not benefit all type of cancers as, as noted in the sections above, in a subset of diseases AhR has been shown to act as a tumor suppressor (i.e. ER+ breast cancer, androgen-sensitive prostate cancer, non-MYCN-amplified neuroblastoma, see Figure 3). Additionally, while not many severe side effects have been revealed so far by the use of AhR antagonists in current clinical trials, systemic inhibition of AhR may still end up causing co-morbidities later in life, as AhR has well-documented physiological roles in normal development, as well as still controversial roles in some of the immune cell populations (i.e. NK cells).
Conclusions and future perspectives
A tremendous body of literature continues to provide support for AhR as a critical modulator of tumor progression across a wide variety of cancer types – from solid and liquid tumors to adult and pediatric malignancies. Like many other transcription factors, AhR has a dual role in cancer biology, with either oncogenic or tumor suppressive effects that are highly contextual, depending on the specific ligand or cell type. While these context-specific effects have often produced conflicting results, they also provide avenues for further investigation. The ligand-specific effects of endogenous ligands, such as those produced by gut microbiota, on tumor growth is an exciting and emerging topic with potential therapeutic applications. Finally, the development and clinical testing of novel AhR modulating drugs, such as the FDA Fast Track-Designated AhR inhibitor, IK-175, are promising and could represent potential new cancer therapies.
Author contributions
AB-S: Writing – original draft, Writing – review & editing. KC: Writing – original draft, Writing – review & editing.
Funding
The author(s) declare financial support was received for the research, authorship, and/or publication of this article. This work has been partly supported by grants from the Roswell Park Alliance Foundation (to AB-S), the Kate Amato Foundation (to KC), the NIH T32 (to KC), and the NCI Cancer Center Support Grant P30CA16056 (to the Roswell Park Comprehensive Cancer Center).
Acknowledgments
Figures were made with BioRender.
Conflict of interest
The authors declare that the research was conducted in the absence of any commercial or financial relationships that could be construed as a potential conflict of interest.
Publisher’s note
All claims expressed in this article are solely those of the authors and do not necessarily represent those of their affiliated organizations, or those of the publisher, the editors and the reviewers. Any product that may be evaluated in this article, or claim that may be made by its manufacturer, is not guaranteed or endorsed by the publisher.
Glossary
References
1. Poland A, Glover E, Kende AS. Stereospecific, high affinity binding of 2,3,7,8 tetrachlorodibenzo p dioxin by hepatic cytosol. Evidence that the binding species is receptor for induction of aryl hydrocarbon hydroxylase. J Biol Chem. (1976) 251:4936–46. doi: 10.1016/s0021-9258(17)33205-2
2. Fukunaga BN, Probst MR, Reisz-Porszasz S, Hankinson O. Identification of functional domains of the aryl hydrocarbon receptor. J Biol Chem. (1995) 270:29270–8. doi: 10.1074/jbc.270.49.29270
3. Gruszczyk J, Grandvuillemin L, Lai-Kee-Him J, Paloni M, Savva CG, Germain P, et al. Cryo-EM structure of the agonist-bound Hsp90-XAP2-AHR cytosolic complex. Nat Commun. (2022) 13:1–13. doi: 10.2210/pdb7zub/pdb
4. Nebert DW, Roe AL, Zieter MZ, Solis WA, Yang Y, Dalton TP. Role of the aromatic hydrocarbon receptor and [Ah] gene battery in the oxidative stress response, cell cycle control, and apoptosis. Biochem Pharmacol. (2000) 59:66–85. doi: 10.1016/S0006-2952(99)00310-X
5. Schiering C, Wincent E, Metidji A, Iseppon A, Li Y, Alexandre J. Feedback control of AHR signaling regulates intestinal immunity. Nature. (2017) 542:242–5. doi: 10.1038/nature21080.Feedback
6. Mimura J, Ema M, Sogawa K, Fujii-Kuriyama Y. Identification of a novel mechanism of regulation of Ah (dioxin) receptor function. Genes Dev. (1999) 13:20–5. doi: 10.1101/gad.13.1.20
7. Davarinos NA, Pollenz RS. Aryl hydrocarbon receptor imported into the nucleus following ligand binding is rapidly degraded via the cytosplasmic proteasome following nuclear export. J Biol Chem. (1999) 274:28708–15. doi: 10.1074/jbc.274.40.28708
8. Shimada T, Inoue K, Suzuki Y, Kawai T, Azuma E, Nakajima T, et al. Arylhydrocarbon receptor-dependent induction of liver and lung cytochromes P450 1A1, 1A2, and 1B1 by polycyclic aromatic hydrocarbons and polychlorinated biphenyls in genetically engineered C57BL/6J mice. Carcinogenesis. (2002) 23:1199–207. doi: 10.1093/carcin/23.7.1199
9. Song J, Clagett-Dame M, Peterson RE, Hahn ME, Westler WM, Sicinski RR, et al. A ligand for the aryl hydrocarbon receptor isolated from lung. Proc Natl Acad Sci U S A. (2002) 99:14694–9. doi: 10.1073/pnas.232562899
10. Opitz CA, Litzenburger UM, Sahm F, Ott M, Tritschler I, Trump S, et al. An endogenous tumour-promoting ligand of the human aryl hydrocarbon receptor. Nature. (2011) 478:197–203. doi: 10.1038/nature10491
11. Denison MS, Soshilov AA, He G, Degroot DE, Zhao B. Exactly the same but different: Promiscuity and diversity in the molecular mechanisms of action of the aryl hydrocarbon (dioxin) receptor. Toxicol Sci. (2011) 124:1–22. doi: 10.1093/toxsci/kfr218
12. Kim DW, Gazourian L, Quadri SA, Raphaëlle, Sherr DH, Sonenshein GE. The RelA NF-κB subunit and the aryl hydrocarbon receptor (AhR) cooperate to transactivate the c-myc promoter in mammary cells. Oncogene. (2000) 19:5498–506. doi: 10.1038/sj.onc.1203945
13. Beischlag TV, Perdew GH. ERα-AHR-ARNT protein-protein interactions mediate estradiol-dependent transrepression of dioxin-inducible gene transcription. J Biol Chem. (2005) 280:21607–11. doi: 10.1074/jbc.C500090200
14. Wilson SR, Joshi AD, Elferink CJ. The tumor suppressor kruppel-like factor 6 is a novel aryl hydrocarbon receptor DNA binding partner. J Pharmacol Exp Ther. (2013) 345:419–29. doi: 10.1124/jpet.113.203786
15. Schmidt JV, Su GHT, Reddy JK, Simon MC, Bradfield CA. Characterization of a murine Ahr null allele: Involvement of the Ah receptor in hepatic growth and development. Proc Natl Acad Sci U S A. (1996) 93:6731–6. doi: 10.1073/pnas.93.13.6731
16. Scoville SD, Nalin AP, Chen L, Chen L, Zhang MH, McConnell K, et al. Human AML activates the aryl hydrocarbon receptor pathway to impair NK cell development and function. Blood. (2018) 132:1792–804. doi: 10.1182/blood-2018-03-838474
17. Romine KA, Nechiporuk T, Bottomly D, Jeng S, McWeeney SK, Kaempf A, et al. Monocytic differentiation and AHR signaling as primary nodes of BET inhibitor response in acute myeloid leukemia. Blood Cancer Discovery. (2021) 2:518–31. doi: 10.1158/2643-3230.bcd-21-0012
18. Bianchi-Smiraglia A, Bagati A, Fink EE, Affronti HC, Lipchick BC, Moparthy S, et al. Inhibition of the aryl hydrocarbon receptor/polyamine biosynthesis axis suppresses multiple myeloma. J Clin Invest. (2018) 128:4682–96. doi: 10.1172/JCI70712
19. Hughes T, Cottini F, Catton E, Ciarlariello D, Chen L, Yang Y, et al. Functional expression of aryl hydrocarbon receptor as a potential novel therapeutic target in human multiple myeloma. Leuk Lymphoma. (2021) 62:2968–80. doi: 10.1080/10428194.2021.1948033
20. Sherr DH, Monti S. The role of the aryl hydrocarbon receptor in normal and Malignant B cell development. Semin Immunopathol. (2013) 35:705–16. doi: 10.1007/s00281-013-0390-8
21. Atene CG, Fiorcari S, Mesini N, Alboni S, Martinelli S, Maccaferri M, et al. Indoleamine 2, 3-dioxygenase 1 mediates survival signals in chronic lymphocytic leukemia via kynurenine/aryl hydrocarbon receptor-mediated MCL1 modulation. Front Immunol. (2022) 13:832263. doi: 10.3389/fimmu.2022.832263
22. Sadik A, Somarribas Patterson LF, Öztürk S, Mohapatra SR, Panitz V, Secker PF, et al. IL4I1 is a metabolic immune checkpoint that activates the AHR and promotes tumor progression. Cell. (2020) 182:1252–1270.e34. doi: 10.1016/j.cell.2020.07.038
23. Chen X, Zang Y, Li D, Guo J, Wang Y, Lin Y, et al. IDO, TDO, and AHR overexpression is associated with poor outcome in diffuse large B-cell lymphoma patients in the rituximab era. Med (United States). (2020) 99:E19883. doi: 10.1097/MD.0000000000019883
24. Ding J, Dirks WG, Ehrentraut S, Geffers R, MacLeod RAF, Nagel S, et al. BCL6 – Regulated by AhR/ARNT and wild-type MEF2B – Drives expression of germinal center markers MYBL1 and LMO2. Haematologica. (2015) 100:801–9. doi: 10.3324/haematol.2014.120048
25. Novikov O, Wang Z, Stanford EA, Parks AJ, Ramirez-Cardenas A, Landesman , et al. An aryl hydrocarbon receptor-mediated amplification loop that enforces cell migration in ER-/PR-/Her2- human breast cancer cells. Mol Pharmacol. (2016) 90:674–88. doi: 10.1124/mol.116.105361
26. Tarnow P, Tralau T, Luch A. Chemical activation of estrogen and aryl hydrocarbon receptor signaling pathways and their interaction in toxicology and metabolism. Expert Opin Drug Metab Toxicol. (2019) 15:219–29. doi: 10.1080/17425255.2019.1569627
27. D’Amato NC, Rogers TJ, Gordon MA, Greene LI, Cochrane DR, Spoelstra NS, et al. A TDO2-AhR signaling axis facilitates anoikis resistance and metastasis in triple-negative breast cancer. Cancer Res. (2015) 75:4651–64. doi: 10.1158/0008-5472.CAN-15-2011
28. Goode GD, Ballard BR, Manning HC, Freeman ML, Kang Y, Eltom SE. Knockdown of aberrantly upregulated aryl hydrocarbon receptor reduces tumor growth and metastasis of MDA-MB-231 human breast cancer cell line. Int J Cancer. (2013) 133:2769–80. doi: 10.1002/ijc.28297
29. Stanford EA, Wang Z, Novikov O, Mulas F, Landesman-Bollag E, Monti S, et al. The role of the aryl hydrocarbon receptor in the development of cells with the molecular and functional characteristics of cancer stem-like cells. BMC Biol. (2016) 14:1–22. doi: 10.1186/s12915-016-0240-y
30. Jin UH, Lee SO, Pfent C, Safe S. The aryl hydrocarbon receptor ligand omeprazole inhibits breast cancer cell invasion and metastasis. BMC Cancer. (2014) 14. doi: 10.1186/1471-2407-14-498
31. Zhang S, Kim KH, Jin UH, Pfent C, Cao H, Amendt B, et al. Aryl hydrocarbon receptor agonists induce microRNA-335 expression and inhibit lung metastasis of estrogen receptor negative breast cancer cells. Mol Cancer Ther. (2012) 11:108–18. doi: 10.1158/1535-7163.MCT-11-0548
32. Köhle C, Hassepass I, Bock-Hennig BS, Walter Bock K, Poellinger L, McGuire J. Conditional expression of a constitutively active aryl hydrocarbon receptor in MCF-7 human breast cancer cells. Arch Biochem Biophys. (2002) 402:172–9. doi: 10.1016/S0003-9861(02)00076-0
33. Gierthy JF, Bennett JA, Bradley LM, Cutler DS. Correlation of in vitro and in vivo growth suppression of MCF-7 human breast cancer by 2,3,7,8-tetrachlorodibenzo-p-dioxin. Cancer Res. (1993) 53:3149–53.
34. Kawajiri K, Kobayashi Y, Ohtake F, Ikuta T, Matsushima Y, Mimura J, et al. Aryl hydrocarbon receptor suppresses intestinal carcinogenesis in Apc Min/+ mice with natural ligands. Proc Natl Acad Sci U S A. (2009) 106:13481–6. doi: 10.1073/pnas.0902132106
35. Han H, Davidson LA, Hensel M, Yoo G, Landrock K, Allred C, et al. Loss of aryl hydrocarbon receptor promotes colon tumorigenesis in ApcS580/þ; KrasG12D/þ mice. Mol Cancer Res. (2021) 19:771–83. doi: 10.1158/1541-7786.MCR-20-0789
36. Miyazaki T, Chung S, Sakai H, Ohata H, Obata Y, Shiokawa D, et al. Stemness and immune evasion conferred by the TDO2-AHR pathway are associated with liver metastasis of colon cancer. Cancer Sci. (2022) 113:170–81. doi: 10.1111/cas.15182
37. Zhang X, Liu X, Zhou W, Du Q, Yang M, Ding Y, et al. Blockade of IDO-kynurenine-ahR axis ameliorated colitis-associated colon cancer via inhibiting immune tolerance. Cmgh. (2021) 12:1179–99. doi: 10.1016/j.jcmgh.2021.05.018
38. Ternes D, Tsenkova M, Pozdeev VI, Meyers M, Koncina E, Atatri S, et al. The gut microbial metabolite formate exacerbates colorectal cancer progression. Nat Metab. (2022) 4:458–75. doi: 10.1038/s42255-022-00558-0
39. Zhu P, Yu H, Zhou K, Bai Y, Qi R, Zhang S. 3,3′-Diindolylmethane modulates aryl hydrocarbon receptor of esophageal squamous cell carcinoma to reverse epithelial-mesenchymal transition through repressing RhoA/ROCK1-mediated COX2/PGE2pathway. J Exp Clin Cancer Res. (2020) 39:1–18. doi: 10.1186/s13046-020-01618-7
40. Zhao Y, Sun J, Li Y, Zhou X, Zhai W, Wu Y, et al. Tryptophan 2,3-dioxygenase 2 controls M2 macrophages polarization to promote esophageal squamous cell carcinoma progression via AKT/GSK3β/IL-8 signaling pathway. Acta Pharm Sin B. (2021) 11:2835–49. doi: 10.1016/j.apsb.2021.03.009
41. Kuznetsov NV, Andersson P, Gradin K, von Stein P, Dieckmann A, Pettersson S, et al. The dioxin/aryl hydrocarbon receptor mediates downregulation of osteopontin gene expression in a mouse model of gastric tumourigenesis. Oncogene. (2005) 24:3216–22. doi: 10.1038/sj.onc.1208529
42. Lai DW, Liu SH, Karlsson AI, Lee WJ, Wang KB, Chen YC, et al. The novel Aryl hydrocarbon receptor inhibitor biseugenol inhibits gastric tumor growth and peritoneal dissemination. Oncotarget. (2014) 5:7788–804. doi: 10.18632/oncotarget.2307
43. Wang K, Li Y, Jiang YZ, Dai CF, Patankar MS, Song JS, et al. An endogenous aryl hydrocarbon receptor ligand inhibits proliferation and migration of human ovarian cancer cells. Cancer Lett. (2013) 340:63–71. doi: 10.1016/j.canlet.2013.06.026
44. Litzenburger UM, Opitz CA, Sahm F, Rauschenbach KJ, Trump S, Winter M, et al. Constitutive IDO expression in human cancer is sustained by an autocrine signaling loop involving IL-6, STAT3 and the AHR. Oncotarget. (2014) 5:1038–51. doi: 10.18632/oncotarget.1637
45. Amobi-McCloud A, Muthuswamy R, Battaglia S, Yu H, Liu T, Wang J, et al. IDO1 expression in ovarian cancer induces PD-1 in T cells via aryl hydrocarbon receptor activation. Front Immunol. (2021) 12:678999. doi: 10.3389/fimmu.2021.678999
46. Son DS, Roby KF, Rozman KK, Terranova PF. Estradiol enhances and estriol inhibits the expression of CYP1A1 induced by 2,3,7,8-tetrachlorodibenzo-p-dioxin in a mouse ovarian cancer cell line. Toxicology. (2002) 176:229–43. doi: 10.1016/S0300-483X(02)00162-2
47. Li D, Takao T, Tsunematsu R, Morokuma S, Fukushima K, Kobayashi H, et al. Inhibition of AHR transcription by NF1C is affected by a single-nucleotide polymorphism, and is involved in suppression of human uterine endometrial cancer. Oncogene. (2013) 32:4950–9. doi: 10.1038/onc.2012.509
48. Bian Y, Li Y, Shrestha G, Wen X, Cai B, Wang K, et al. ITE, an endogenous aryl hydrocarbon receptor ligand, suppresses endometrial cancer cell proliferation and migration. Toxicology. (2019) 421:1–8. doi: 10.1016/j.tox.2019.03.017
49. DiNatale BC, Smith K, John K, Krishnegowda G, Amin SG, Perdew GH. Ah receptor antagonism represses head and neck tumor cell aggressive phenotype. Mol Cancer Res. (2012) 10:1369–79. doi: 10.1158/1541-7786.MCR-12-0216
50. Frank DN, Qiu Y, Cao Y, Zhang S, Lu L, Kofonow JM, et al. A dysbiotic microbiome promotes head and neck squamous cell carcinoma. Oncogene. (2022) 41:1269–80. doi: 10.1038/s41388-021-02137-1
51. Stanford EA, Ramirez-Cardenas A, Wang Z, Novikov O, Alamoud K, Koutrakis P, et al. Role for the aryl hydrocarbon receptor and diverse ligands in oral squamous cell carcinoma migration and tumorigenesis. Mol Cancer Res. (2016) 14:696–706. doi: 10.1158/1541-7786.MCR-16-0069
52. Kenison JE, Wang Z, Yang K, Snyder M, Quintana FJ, Sherr DH. The aryl hydrocarbon receptor suppresses immunity to oral squamous cell carcinoma through immune checkpoint regulation. Proc Natl Acad Sci U S A. (2021) 118. doi: 10.1073/pnas.2012692118
53. Wang LT, Chiou SS, Chai CY, Hsi E, Wang SN, Huang SK, et al. Aryl hydrocarbon receptor regulates histone deacetylase 8 expression to repress tumor suppressive activity in hepatocellular carcinoma. Oncotarget. (2017) 8:7489–501. doi: 10.18632/oncotarget.9841
54. Moennikes O, Loeppen S, Buchmann A, Andersson P, Ittrich C, Poellinger L, et al. A constitutively active dioxin/aryl hydrocarbon receptor promotes hepatocarcinogenesis in mice. Cancer Res. (2004) 64:4707–10. doi: 10.1158/0008-5472.CAN-03-0875
55. Fan Y, Boivin GP, Knudsen ES, Nebert DW, Xia Y, Puga A. The aryl hydrocarbon receptor functions as a tumor suppressor of liver carcinogenesis. Cancer Res. (2010) 70:212–20. doi: 10.1158/0008-5472.CAN-09-3090
56. Li L, Wang T, Li S, Chen Z, Wu J, Cao W, et al. TDO2 promotes the EMT of hepatocellular carcinoma through kyn-ahR pathway. Front Oncol. (2021) 10:562823. doi: 10.3389/fonc.2020.562823
57. Yu C, Rao D, Zhu H, Liu Q, Huang W, Zhang L, et al. TDO2 Was Downregulated in Hepatocellular Carcinoma and Inhibited Cell Proliferation by Upregulating the Expression of p21 and p27. BioMed Res Int. (2021) 2021:8–13. doi: 10.1155/2021/4708439
58. Chen W, Wen L, Bao Y, Tang Z, Zhao J, Zhang X, et al. Gut flora disequilibrium promotes the initiation of liver cancer by modulating tryptophan metabolism and up-regulating SREBP2. Proc Natl Acad Sci. (2022) 119. doi: 10.1073/pnas.2203894119
59. Koliopanos A, Kleeff J, Xiao Y, Safe S, Zimmermann A, Büchler MW, et al. Increased arylhydrocarbon receptor expression offers a potential therapeutic target for pancreatic cancer. Oncogene. (2002) 21:6059–70. doi: 10.1038/sj.onc.1205633
60. Liang H, Li T, Fang X, Zhang S, Shi L, Li W, et al. IDO1/TDO dual inhibitor RY103 targets Kyn-AhR pathway and exhibits preclinical efficacy on pancreatic cancer. Cancer Lett. (2021) 522:32–43. doi: 10.1016/j.canlet.2021.09.012
61. Wang L, Tang W, Yang S, He P, Wang J, Gaedcke J, et al. NO•/RUNX3/kynurenine metabolic signaling enhances disease aggressiveness in pancreatic cancer. Int J Cancer. (2020) 146:3160–9. doi: 10.1002/ijc.32733
62. Hezaveh K, Shinde RS, Klötgen A, Halaby MJ, Lamorte S, Ciudad MT, et al. Tryptophan-derived microbial metabolites activate the aryl hydrocarbon receptor in tumor-associated macrophages to suppress anti-tumor immunity. Immunity. (2022) 55:324–340.e8. doi: 10.1016/j.immuni.2022.01.006
63. Ye M, Zhang Y, Gao H, Xu Y, Jing P, Wu J, et al. Activation of the aryl hydrocarbon receptor leads to resistance to EGFR TKIs in non–small cell lung cancer by activating src-mediated bypass signaling. Clin Cancer Res. (2018) 24:1227–39. doi: 10.1158/1078-0432.CCR-17-0396
64. Wang GZ, Zhang L, Zhao XC, Gao SH, Qu LW, Yu H, et al. The Aryl hydrocarbon receptor mediates tobacco-induced PD-L1 expression and is associated with response to immunotherapy. Nat Commun. (2019) 10:1–13. doi: 10.1038/s41467-019-08887-7
65. Nothdurft S, Thumser-Henner C, Breitenbücher F, Okimoto RA, Dorsch M, Opitz CA, et al. Functional screening identifies aryl hydrocarbon receptor as suppressor of lung cancer metastasis. Oncogenesis. (2020) 9:1–12. doi: 10.1038/s41389-020-00286-8
66. Nacarino-Palma A, Rejano-Gordillo CM, González-Rico FJ, Ordiales-Talavero A, Román ÁC, Cuadrado M, et al. Loss of aryl hydrocarbon receptor favors k-rasg12d-driven non-small cell lung cancer. Cancers (Basel). (2021) 13. doi: 10.3390/cancers13164071
67. Friedrich M, Sankowski R, Bunse L, Kilian M, Green E, Ramallo Guevara C, et al. Tryptophan metabolism drives dynamic immunosuppressive myeloid states in IDH-mutant gliomas. Nat Cancer. (2021) 2:723–40. doi: 10.1038/s43018-021-00201-z
68. Takenaka MC, Gabriely G, Rothhammer V, Mascanfroni ID, Wheeler MA, Chao CC, et al. Control of tumor-associated macrophages and T cells in glioblastoma via AHR and CD39. Nat Neurosci. (2019) 22:1533. doi: 10.1038/s41593-019-0446-8
69. Jin UH, Karki K, Cheng Y, Michelhaugh SK, Mittal S, Safe S. The aryl hydrocarbon receptor is a tumor suppressor-like gene in glioblastoma. J Biol Chem. (2019) 294:11342–53. doi: 10.1074/jbc.RA119.008882
70. Chaudhry KA, Jacobi JJ, Gillard BM, Karasik E, Martin JC, da Silva Fernandes T, et al. Aryl hydrocarbon receptor is a tumor promoter in MYCN-amplified neuroblastoma cells through suppression of differentiation. iScience. (2023) 26:108303. doi: 10.1016/j.isci.2023.108303
71. Morales-Hernández A, Corrales-Redondo M, Marcos-Merino JM, González-Rico FJ, Sánchez-Martín FJ, Merino JM. AhR-dependent 2,3,7,8-tetrachlorodibenzo-p-dioxin toxicity in human neuronal cell line SHSY5Y. Neurotoxicology. (2016) 56:55–63. doi: 10.1016/j.neuro.2016.07.001
72. Wu PY, Liao YF, Juan HF, Huang HC, Wang BJ, Lu YL, et al. Aryl hydrocarbon receptor downregulates MYCN expression and promotes cell differentiation of neuroblastoma. PloS One. (2014) 9:3–11. doi: 10.1371/journal.pone.0088795
73. Wu PY, Yu IS, Lin YC, Chang YT, Chen CC, Lin KH, et al. Activation of aryl hydrocarbon receptor by kynurenine impairs progression and metastasis of neuroblastoma. Cancer Res. (2019) 79:5550–62. doi: 10.1158/0008-5472.CAN-18-3272
74. Fritz WA, Lin TM, Cardiff RD, Peterson RE. The aryl hydrocarbon receptor inhibits prostate carcinogenesis in TRAMP mice. Carcinogenesis. (2007) 28:497–505. doi: 10.1093/carcin/bgl179
75. Fritz WA, Lin TM, Safe S, Moore RW, Peterson RE. The selective aryl hydrocarbon receptor modulator 6-methyl-1,3,8-trichlorodibenzofuran inhibits prostate tumor metastasis in TRAMP mice. Biochem Pharmacol. (2009) 77:1151–60. doi: 10.1016/j.bcp.2008.12.015
76. Chen Z, Cai A, Zheng H, Huang H, Sun R, Cui X, et al. Carbidopa suppresses prostate cancer via aryl hydrocarbon receptor-mediated ubiquitination and degradation of androgen receptor. Oncogenesis. (2020) 9:1–13. doi: 10.1038/s41389-020-0236-x
77. Richmond O, Ghotbaddini M, Allen C, Walker A, Zahir S, Powell JB. The aryl hydrocarbon receptor is constitutively active in advanced prostate cancer cells. PloS One. (2014) 9. doi: 10.1371/journal.pone.0095058
78. Law MH, Bishop DT, Lee JE, Brossard M, Martin NG, Moses EK, et al. Genome-wide meta-analysis identifies five new susceptibility loci for cutaneous Malignant melanoma. Nat Genet. (2015) 47:987–95. doi: 10.1038/ng.3373
79. Corre S, Tardif N, Mouchet N, Leclair HM, Boussemart L, Gautron A, et al. Sustained activation of the Aryl hydrocarbon Receptor transcription factor promotes resistance to BRAF-inhibitors in melanoma. Nat Commun. (2018) 9:1–13. doi: 10.1038/s41467-018-06951-2
80. Contador-Troca M, Alvarez-Barrientos A, Barrasa E, Rico-Leo EM, Catalina-Fernández I, Menacho-Márquez M, et al. The dioxin receptor has tumor suppressor activity in melanoma growth and metastasis. Carcinogenesis. (2013) 34:2683–93. doi: 10.1093/carcin/bgt248
81. Bender MJ, McPherson AC, Phelps CM, Pandey SP, Laughlin CR, Shapira JH, et al. Dietary tryptophan metabolite released by intratumoral Lactobacillus reuteri facilitates immune checkpoint inhibitor treatment. Cell. (2023) 186:1846–1862.e26. doi: 10.1016/j.cell.2023.03.011
82. Vlaar JM, Borgman A, Kalkhoven E, Westland D, Besselink N, Shale C, et al. Recurrent exon-deleting activating mutations in AHR act as drivers of urinary tract cancer. Sci Rep. (2022) 12:1–12. doi: 10.1038/s41598-022-14256-0
83. Shi MJ, Meng XY, Fontugne J, Chen CL, Radvanyi F, Bernard-Pierrot I. Identification of new driver and passenger mutations within APOBEC-induced hotspot mutations in bladder cancer. Genome Med. (2020) 12:1–20. doi: 10.1186/s13073-020-00781-y
84. Ishida M, Mikami S, Kikuchi E, Kosaka T, Miyajima A, Nakagawa K, et al. Activation of the aryl hydrocarbon receptor pathway enhances cancer cell invasion by upregulating the MMP expression and is associated with poor prognosis in upper urinary tract urothelial cancer. Carcinogenesis. (2010) 31:287–95. doi: 10.1093/carcin/bgp222
85. Griffith BD, Frankel TL. The aryl hydrocarbon receptor: impact on the tumor immune microenvironment and modulation as a potential therapy. Cancers (Basel). (2024) 16:1–19. doi: 10.3390/cancers16030472
86. Basson C, Serem JC, Hlophe YN, Bipath P. The tryptophan–kynurenine pathway in immunomodulation and cancer metastasis. Cancer Med. (2023) 12:18691–701. doi: 10.1002/cam4.6484
87. Stone TW, Williams RO. Modulation of T cells by tryptophan metabolites in the kynurenine pathway. Trends Pharmacol Sci. (2023) 44:442–56. doi: 10.1016/j.tips.2023.04.006
88. Congues F, Wang P, Lee J, Lin D, Shahid A. Targeting aryl hydrocarbon receptor to prevent cancer in barrier organs. Biochem Pharmacol Published Online. (2024), 116156. doi: 10.1016/j.bcp.2024.116156
89. Martin JC, da Silva Fernandes T, Chaudhry KA, Oshi M, Abrams SI, Takabe K, et al. Aryl hydrocarbon receptor suppresses STING-mediated type I IFN expression in triple-negative breast cancer. Sci Rep. (2024) 14:1–13. doi: 10.1038/s41598-024-54732-3
90. Wu D, Wang G, Wen S, Liu X, He Q. ARID5A stabilizes Indoleamine 2,3-dioxygenase expression and enhances CAR T cell exhaustion in colorectal cancer. Transl Oncol. (2024) 42:101900. doi: 10.1016/j.tranon.2024.101900
91. Dumbrava EE, Cecchini M, Zugazagoitia J, Lopez JS, Jager D, Olivia M, et al. Initial results from a first-in-human, phase I study of immunomodulatory aryl hydrocarbon receptor (AhR) inhibitor BAY2416964 in patients with advanced solid tumors. J Clin Oncol. (2023) 41:2502–2. doi: 10.1200/jco.2023.41.16_suppl.2502
92. Shin JH, Zhang L, Murillo-Sauca O, Kim J, Kohrt HEK, Bui JD, et al. Modulation of natural killer cell antitumor activity by the aryl hydrocarbon receptor. Proc Natl Acad Sci U S A. (2013) 110:12391–6. doi: 10.1073/pnas.1302856110
93. Sato K, Ohira M, Imaoka Y, Imaoka K, Bekki T, Doskali M, et al. The aryl hydrocarbon receptor maintains antitumor activity of liver resident natural killer cells after partial hepatectomy in C57BL/6J mice. Cancer Med. (2023) 12:19821–37. doi: 10.1002/cam4.6554
94. Trikha P, Moseman JE, Thakkar A, Campbell AR, Elmas E, Foltz JA, et al. Defining the AHR-regulated transcriptome in NK cells reveals gene expression programs relevant to development and function. Blood Adv. (2021) 5:4605–18. doi: 10.1182/bloodadvances.2021004533
95. Boitano AE. Aryl hydrocarbon receptor antagonists promote the expansion of human hematopoietic stem cells (Science (2010) (1345)). Sci (80-). (2011) 332:664. doi: 10.1126/science.1191536
96. Kober C, Roewe J, Schmees N, Roese L, Roehn U, Bader B, et al. Targeting the aryl hydrocarbon receptor (AhR) with BAY 2416964: a selective small molecule inhibitor for cancer immunotherapy. J Immunother Cancer. (2023) 11:e007495. doi: 10.1136/jitc-2023-007495
97. McGovern K, Castro AC, Cavanaugh J, Coma S, Walsh M, Tchaicha J, et al. Discovery and characterization of a novel aryl hydrocarbon receptor inhibitor, IK-175, and its inhibitory activity on tumor immune suppression. Mol Cancer Ther. (2022) 4:1261–72. doi: 10.1158/1535-7163.MCT-21-0984
98. Lebwohl MG, Stein Gold L, Strober B, Papp KA, Armstrong AW, Bagel J, et al. Phase 3 trials of tapinarof cream for plaque psoriasis. N Engl J Med. (2021) 385:2219–29. doi: 10.1056/nejmoa2103629
99. Chen Z, Xia X, Chen H, Huang H, An X, Sun M, et al. Carbidopa suppresses estrogen receptor-positive breast cancer via AhR-mediated proteasomal degradation of ERα. Invest New Drugs. (2022) 40:1216–30. doi: 10.1007/s10637-022-01289-5
100. Ogura J, Miyauchi S, Shimono K, Yang S, Gonchigar S, Ganapathy V, et al. Carbidopa is an activator of aryl hydrocarbon receptor with potential for cancer therapy. Biochem J. (2017) 474:3391–402. doi: 10.1042/BCJ20170583
101. Park H, Jin UH, Karki K, Jayaraman A, Allred C, Michelhaugh SM, et al. Dopamine is an aryl hydrocarbon receptor agonist. Biochem J. (2020) 477:3899–910. doi: 10.1042/BCJ20200440
102. Koch DC, Jang HS, O’Donnell EF, Punj S, Kopparapu PR, Bisson WH, et al. Anti-androgen flutamide suppresses hepatocellular carcinoma cell proliferation via the aryl hydrocarbon receptor mediated induction of transforming growth factor-β1. Oncogene. (2015) 34:6092–104. doi: 10.1038/onc.2015.55
103. O’Donnell EF, Kopparapu PR, Koch DC, Jang HS, Phillips JL, Tanguay, et al. The Aryl hydrocarbon receptor mediates leflunomide-induced growth inhibition of melanoma cells. PloS One. (2012) 7. doi: 10.1371/journal.pone.0040926
104. Jin UH, Lee SO, Safe S. Aryl hydrocarbon receptor (AHR)-active pharmaceuticals are selective AHR modulators in MDA-MB-468 and BT474 breast cancer cells. J Pharmacol Exp Ther. (2012) 343:333–41. doi: 10.1124/jpet.112.195339
105. Jin UH, Michelhaugh SK, Polin LA, Shrestha R, Mittal S, Safe S. Omeprazole inhibits glioblastoma cell invasion and tumor growth. Cancers (Basel). (2020) 12:1–15. doi: 10.3390/cancers12082097
106. Bai Y, Zhu P, Zhou K, Zhang S-G. Effect of the acid suppressor omeprazole on the proliferation, migration, invasion and cell cycle of esophageal squamous cell carcinoma cells via the aryl hydrocarbon receptor pathway. Exp Ther Med. (2021) 22. doi: 10.3892/etm.2021.10621
107. Jin UH, Kim SB, Safe S. Omeprazole inhibits pancreatic cancer cell invasion through a nongenomic aryl hydrocarbon receptor pathway. Chem Res Toxicol. (2015) 28:907–18. doi: 10.1021/tx5005198
108. O’Donnell EF, Koch DC, Bisson WH, Jang HS, Kolluri SK. The aryl hydrocarbon receptor mediates raloxifene-induced apoptosis in estrogen receptor-negative hepatoma and breast cancer cells. Cell Death Dis. (2014) 5:1–12. doi: 10.1038/cddis.2013.549
109. Wei KL, Gao GL, Chou YT, Lin CY, Chen SC, Chen YL, et al. Sorafenib is an antagonist of the aryl hydrocarbon receptor. Toxicology. (2022) +):153118. doi: 10.1016/j.tox.2022.153118
Keywords: AhR, cancer biology, therapeutics, immune regulation, xenobiotic, tumor promoter, tumor suppressor
Citation: Chaudhry KA and Bianchi-Smiraglia A (2024) The aryl hydrocarbon receptor as a tumor modulator: mechanisms to therapy. Front. Oncol. 14:1375905. doi: 10.3389/fonc.2024.1375905
Received: 24 January 2024; Accepted: 03 May 2024;
Published: 14 May 2024.
Edited by:
Zhe-Sheng Chen, St. John’s University, United StatesReviewed by:
Giorgia Manni, University of Perugia, ItalyGuoyi Tang, The University of Hong Kong, Hong Kong SAR, China
Copyright © 2024 Chaudhry and Bianchi-Smiraglia. This is an open-access article distributed under the terms of the Creative Commons Attribution License (CC BY). The use, distribution or reproduction in other forums is permitted, provided the original author(s) and the copyright owner(s) are credited and that the original publication in this journal is cited, in accordance with accepted academic practice. No use, distribution or reproduction is permitted which does not comply with these terms.
*Correspondence: Anna Bianchi-Smiraglia, Anna.Bianchi-Smiraglia@RoswellPark.org