- 1Section for Cancer Cytogenetics, Institute for Cancer Genetics and Informatics, The Norwegian Radium Hospital, Oslo University Hospital, Oslo, Norway
- 2Department of Pathology, The Norwegian Radium Hospital, Oslo University Hospital, Oslo, Norway
- 3Institute of Clinical Medicine, Faculty of Medicine, University of Oslo, Oslo, Norway
- 4Department of Haematology, Oslo University Hospital, Oslo, Norway
- 5Department of Immunology, Oslo University Hospital, Oslo, Norway
Introduction: Alterations of the NUP214 gene (9q34) are recurrent in acute leukemias. Rearrangements of chromosomal band 9q34 targeting this locus can be karyotypically distinct, for example t(6;9)(p22;q34)/DEK::NUP214, or cryptic, in which case no visible change of 9q34 is seen by chromosome banding.
Methods: We examined 9 cases of acute leukemia with NUP214 rearrangement by array Comparative Genomic Hybridization (aCGH), reverse-transcription polymerase chain reaction (RT-PCR), and cycle sequencing/Sanger sequencing to detect which fusion genes had been generated.
Results: The chimeras DEK::NUP214, SET::NUP214, and NUP214::ABL1 were found, only the first of which can be readily detected by karyotyping.
Discussion: The identification of a specific NUP214 rearrangement is fundamental in the management of these patients, i.e., AMLs with DEK::NUP214 are classified as an adverse risk group and might be considered for allogenic transplant. Genome- and/or transcriptome-based next generation sequencing (NGS) techniques can be used to screen for these fusions, but we hereby present an alternative, step-wise procedure to detect these rearrangements.
Introduction
Leukemias are a highly heterogeneous - both phenotypically and pathogenetically - group of aggressive neoplasias that affect the production and maturation of bone marrow cells (1, 2). They are classified into two major groups: myeloid leukemias that involve more or less mature granulocytes, erythrocytes, megakaryocytes, and/or monocytes, and the lymphoid ones that involve lymphocytes and their precursor cells (3). Based on disease development (classically) or the degree of cellular maturation (currently), leukemias are additionally classified as acute or chronic. Acute myeloid leukemia (AML) is the most common leukemia in adults (4). Depending on phenotypic features such as cellular morphology, but increasingly in later years on which acquired genetic change brought about neoplastic transformation, the various leukemias are subdivided further into entities with distinctive prognostic features dependent on the therapeutic regimens that experience has shown to be preferable (1). For these reasons, one currently pays more attention to (patho)genetic features when diagnosing and treating leukemia patients; a precise understanding of what lies behind leukemogenesis in individual patients has become adamant in clinical state-of-the-art medicine. To do this, studies of the leukemic cells with different techniques are necessary.
In this study, we selected for examination 9 acute leukemia cases with rearrangements of chromosomal band 9q34 detected either by G-banding and/or FISH, but excluding cases displaying the translocation t(9;22)(q34;q11) that characterizes Philadelphia (Ph)-positive leukemias. We used array comparative genomic hybridization (aCGH), reverse-transcription polymerase chain reaction (RT-PCR), and cycle sequencing/Sanger sequencing to identify the fusion genes generated by the different genomic rearrangements.
Materials and methods
Material
Nine acute leukemias, from 6 males and 3 females aged between 8 and 78 years (Table 1), were investigated diagnostically in our laboratory during 2011 and 2023. Bone marrow aspirated and/or blood sampleswere found by karyotype/FISH analysis to carry cells with rearrangement of chromosomal band 9q34 other than the t(9;22) specific for Ph-positive leukemias. The study was approved by the Regional Committee for Medical and Health Research Ethics (REK, project number S-0747a; http://helseforskning.etikkom.no.
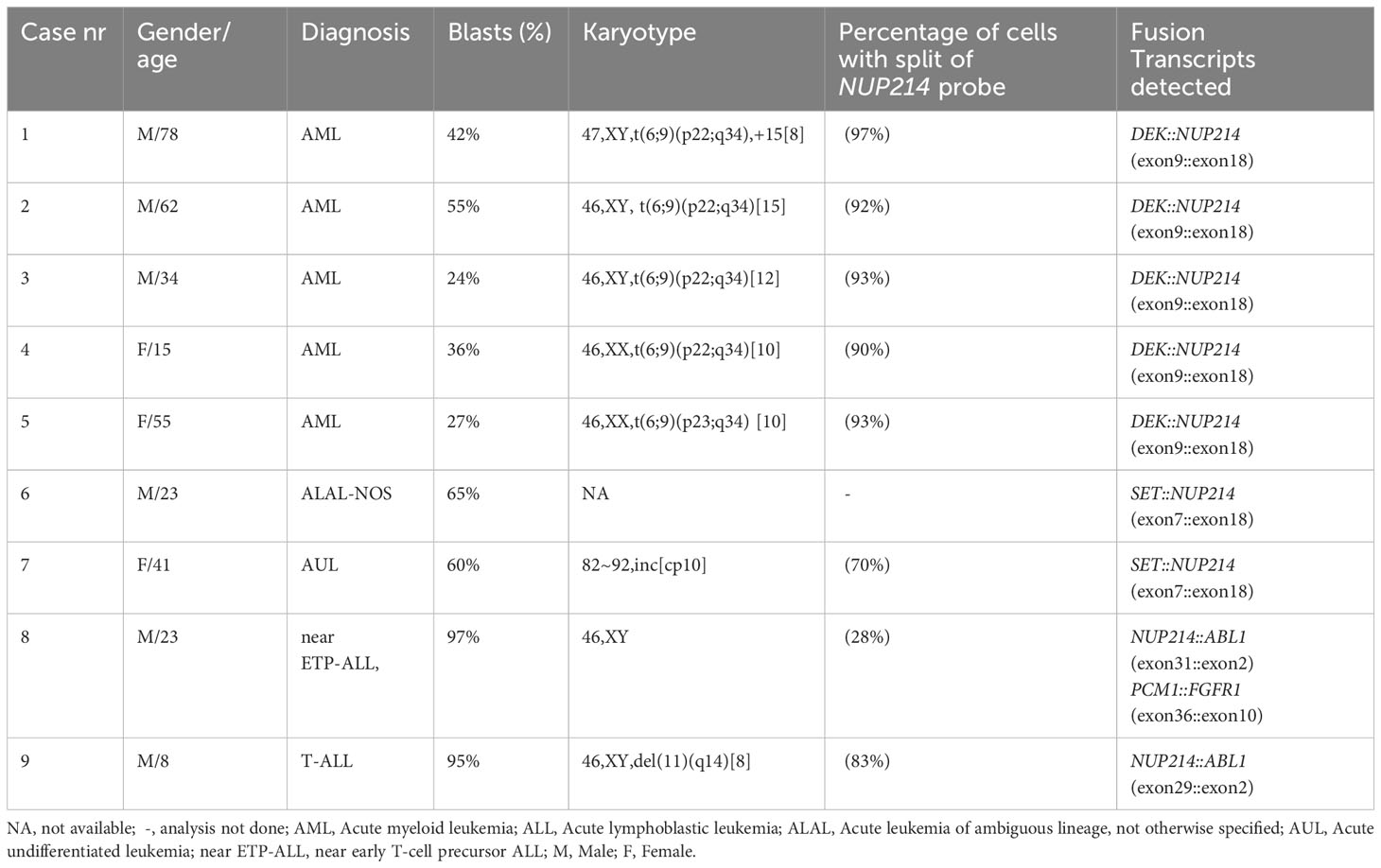
Table 1 Overview of clinical diagnostic parameters and molecular analysis of the nine acute leukemias.
G-banding and fluorescence in situ hybridization analyses
Hematopoietic cells were short-term cultured, harvested, stained for G-banding analysis, and analyzed cytogenetically as previously described (5, 6). The karyotype description followed the recommendations of the International System of Cytogenomic Nomenclature (7). In addition, routine leukemia-relevant FISH analyses were performed according to standard protocols. For acute myeloid leukemia (AML), tests were performed using commercial probes (Cytocell, Oxford Gene Technology, Begbroke, Oxfordshire, UK) to detect deletions of 5q, 7q, 20q, and the TP53 locus (17p13), splitting of the KMT2A locus (previously called MLL; 11q23), as well as for the possible presence of fusion genes PML::RARA, RUNX1::RUNX1T1, and CBFB::MYH11. For acute lymphoblastic leukemia (ALL), FISH investigations (probes from Cytocell, Oxford Gene Technology) aimed to detect deletion of CDKN2A (9p21), fusion genes ETV6::RUNX1 and BCR::ABL1, rearrangement of ABL1 (9q34), MYC (8q24), KMT2A (11q23.3), TCF3 (19p13.3), and IGH (14q32.33), as well as the possible presence of additional chromosomes typical of a hyperdiploid karyotype.
Additional FISH investigations to detect rearrangement of the NUP214 locus (9q34) were performed using the Dual Color Break Apart Probe (Zytovision, ZytoLight, Bremerhaven, Germany) on those cases from which suitable material was available. Chromosome preparations were counterstained with 0.2 μg/ml DAPI and overlaid with a 24×50 mm2 coverslip. Fluorescent signals were captured and analyzed using the CytoVision system (Leica Biosystems, Newcastle, UK).
DNA and RNA extraction and sequencing
Fresh frozen bone marrow or blood was used to extract DNA and RNA. DNA was extracted using the Maxwell 16 extractor (Promega, Madison, Wisconsin, United States) and purified using the Maxwell 16 Cell DNA Purification kit (Promega) according to the manufacturer’s recommendations. RNA was extracted using a miRNeasy kit (Qiagen, Hilden, Germany) or the Monarch Total RNA miniprep kit (New England Biolabs, Ipswich, Massachusetts, USA). The concentrations were measured using QIAxel microfluidic UV/VIS spectrophotometer (Qiagen) and Quantus fluorometer (Promega).
Array comparative genomic hybridization
Array Comparative Genomic Hybridization (aCGH) using CytoSure Consortium Cancer + SNP arrays (Oxford Gene Technology, Oxford, UK) was performed to screen for genomic imbalances. Data were analyzed using Agilent Feature Extraction Software (Agilent Technologies; version 10.7.3.1) and CytoSure Interpret Software (Oxford Gene Technology; version 4.9.40). Copy number aberrations (CNA) were identified using the Circular Binary Segmentation (CBS) algorithm, adding a custom-made aberration filter defining imbalances as a region with a minimum of five gained/lost probes (8). Imbalances were scored according to (9); however, also those smaller than 5Mb were considered. Annotations were based on the human reference sequence GRCh37/hg19 (10).
Reverse transcription polymerase chain reaction
One µg of total RNA was reverse-transcribed in a 20 µL reaction volume using iScript Advanced cDNA Synthesis Kit for RT-PCR according to the manufacturer’s instructions (Bio-Rad, Hercules, CA, USA). Primers used for PCR reactions are listed in Supplementary Table 3. The PCR amplifications were run on a C-1000 Thermal cycler (Bio-Rad) with an initial denaturation at 94°C for 30 sec followed by 35 cycles at 98°C for 7 sec, 60°C for 30 sec, and a final extension at 72°C for 1 min. Three µL of the PCR product were stained with GelRed (Biotium, Hayward, CA, USA), analyzed by electrophoresis through 1.0% agarose gel, and photographed. The remaining PCR products were purified using the MinElute PCR Purification Kit (Qiagen) and sequenced using an Applied Biosystems SeqStudio Genetic Analyzer system with BigDye™ Terminator v1.1 Cycle Sequencing Kit (ThermoFisher Scientific, Waltham, MA, USA) according to the company’s recommendations. The basic local alignment search tool (BLAST) software (BLAST; https://blast.ncbi.nlm.nih.gov/Blast.cgi; accessed on 15 April 2023) was used for computer analysis of sequence data (11). The BLAT alignment tool and the human genome browser at the University of California, Santa Cruz (UCSC) were also used to map the sequences on the Human GRCh37/hg19 assembly (BLAT; http://genome.ucsc.edu/cgi-bin/hgBlat; accessed on 15 April 2023) (12).
Quantitative RT-PCR analyses
qRT-PCR was carried out to determine the expression level of T-cell leukemia homeobox 3 (TLX3) in T-ALL patients using the CFX96 Touch Real-Time PCR detection system (Bio-Rad Laboratories). Reactions were carried out in triplicate using TaqMan Assays and the TaqMan Universal Master Mix II with UNG (Applied Biosystems, Foster City, CA) according to the manufacturer’s protocol. The thermal cycling parameters consisted of 40 cycles of an initial denaturation step of 95°C for 10 minutes, followed by 60°C for 1 min. The primers used for the qRT-PCR reaction were TLX3 (Hs00253271_m1; ThermoFisher) and, as an endogenous control, actin beta (ACTB; Hs01060665_g1, ThermoFisher). Human universal reference total RNA (Clontech, Mountain View, CA) was used as internal reaction control.
Results
Four of the nine leukemias (cases 2, 3, 4, and 5) had t(6;9)(p22;q34) as a sole aberration by G-banding. Case 1 had trisomy 15 in addition to the t(6;9). Altogether, five of the leukemias were diagnosed as AML (cases 1-5). One acute undifferentiated leukemia (AUL; case 7) showed a complex, near-tetraploid karyotype. Cases 8 and 9, diagnosed as near ETP-ALL and T-ALL, showed a normal karyotype and the chromosomal deletion del(11)(q14), respectively. The karyotype from the ALAL-NOS patient (case 6) was not available. All karyotype descriptions as well as FISH profiles are listed in Table 1; Supplementary Table 1. The FISH analyses were those recommended by the WHO (1), national (https://www.helsedirektoratet.no/retningslinjer/maligne-blodsykdommer–handlingsprogram), ALLTogether (ClinicalTrials.gov Identifier: NCT04307576), and NOPHO (2008) protocols (13) as standard for the diagnosis of AML and ALL. Signal patterns detected by FISH did not show any aberrations for any probes except for ABL1 probe (9q34). These FISH data, together with the NUP214 signal pattern, are summarized in Table 1; Supplementary Table 1. Mutation analysis and flow cytometry data are shown in Supplementary Table 1.
The imbalances detected by aCGH analyses are presented in Supplementary Table 2. Genomic imbalances were detected in cases 1, 5, 6, 7, 8, and 9. A total of 69 imbalances were scored (range 1 to 29), with losses being more frequent than gains.
In cases 2, 3, and 4, no imbalances were seen by aCGH, confirming the balanced rearrangement t(6;9)(p22;q34), seen byG-banding. Cases 1 and 5 showed a similar karyotypic t(6;9); however, these genomes were found to be imbalanced by aCGH (Supplementary Table 2). The remaining cases showed imbalances affecting 9q34, the chromosome band where the gene NUP214 is located demonstrating that cryptic rearrangements undetected by G-banding were present. Of note, cases 6, 7, 8, and 9 showed loss and/or gain of genetic material from 9q33.3-q34.1 less than 5Mb (Figure 1) (9). aCGH identified gains on chromosome band 9q34.12-q34.1 (415 Kb) in cases 8 and 9, and losses on 8p22 (415 Kb) and 8p11.23-p11.22 (520 Kb) in case 8. (Supplementary Table 1).
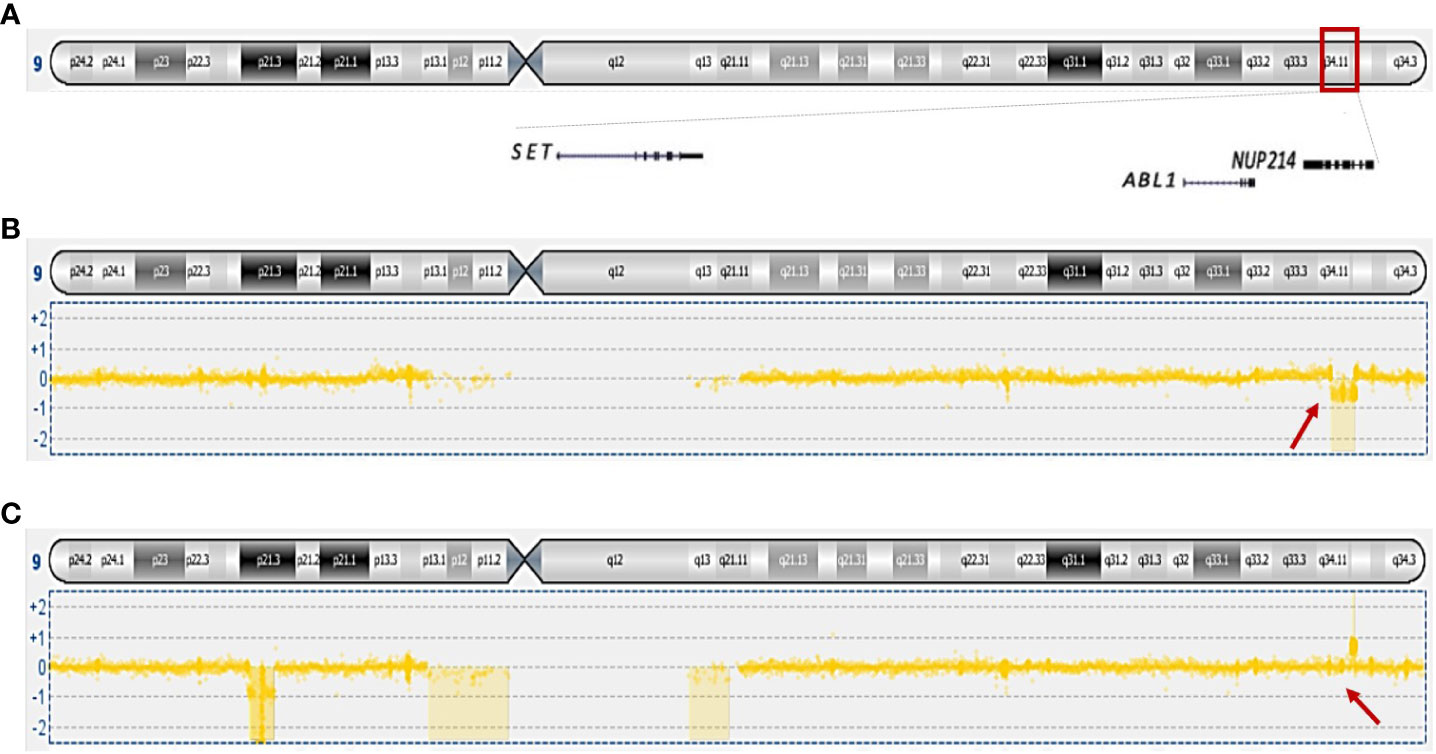
Figure 1 Overview of genes mapping on 9q34 and genomic imbalances scored on this chromosomal band. (A) Ideogram of chromosome 9 showing the mapping position of SET, ABL1, and NUP214; (B) loss of 33.62 kb genomic material from case 8. (C) gain of 97.54 kb genomic material from case 9; in addition, loss of 9p is shown. Arrows are pointing to the imbalances at 9q34.
A DEK::NUP214 fusion was identified in five leukemias (cases 1-5), whereas SET::NUP214 and NUP214::ABL1 were found in two cases each (cases 6 and 7, and 8 and 9, respectively). Of interest, an additional in-frame fusion was detected between PCM1 (8p22; exon 36) and FGFR1 (8p11.23-p11.22; exon 10) in case 8 (Table 1; Figure 2).
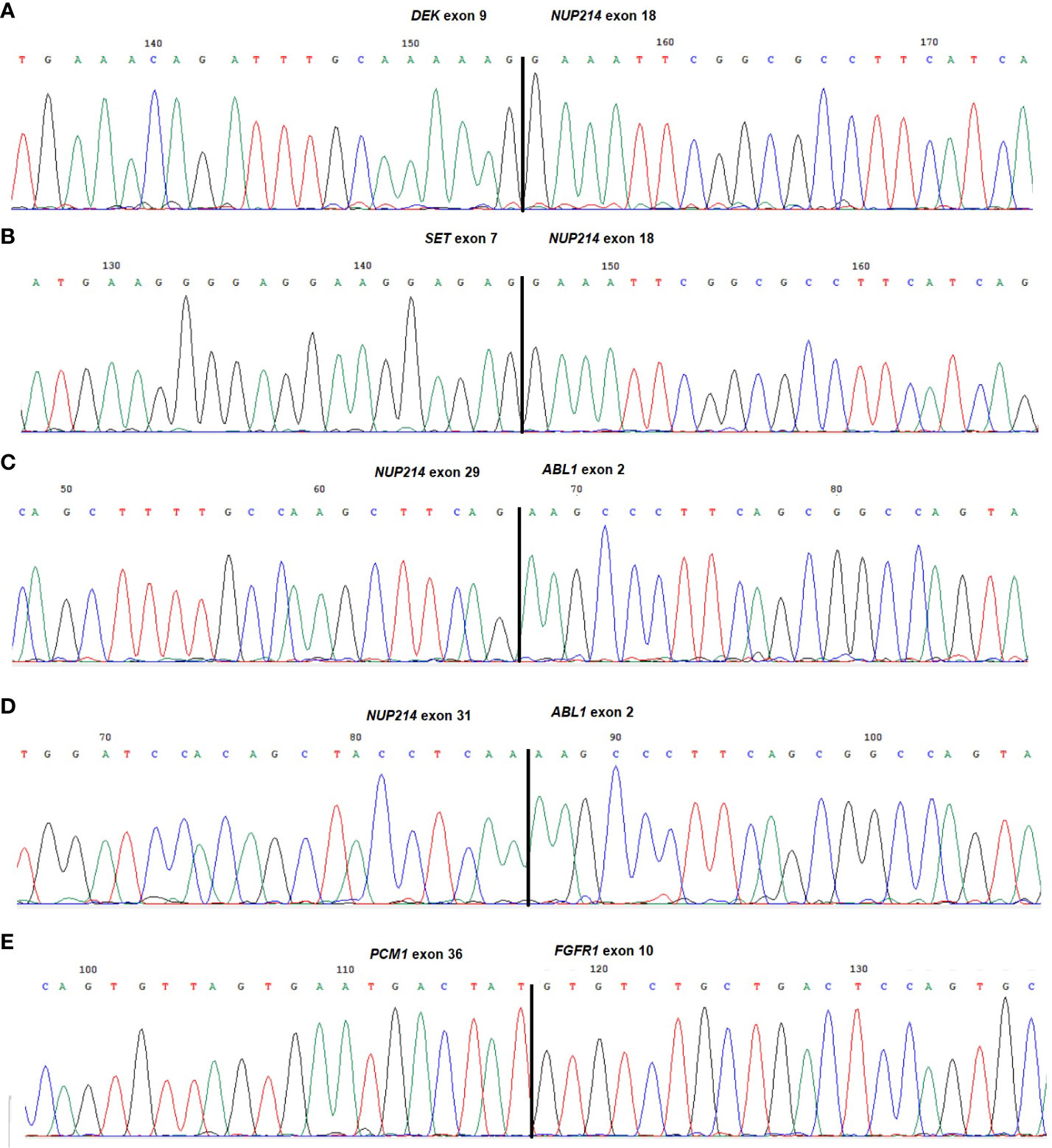
Figure 2 Partial chromatograms of the different chimeric transcripts. (A) DEK::NUP214 junction found in cases 1-5; (B) SET::NUP214 junction found in cases 6 and 7; (C) NUP214::ABL1 junction found in case 8; (D) NUP214::ABL1 variant junction found in case 9; (E) PCM1::FGFR1 junction found in case 8. The exons involved in the breakpoints are reported.
FISH was performed to validate NUP214-rearrangements using a break-apart probe (Table 1). The expression level of TLX3 was investigated in the T-ALL cases. The mean quantification cycle (Cq Mean) of TLX3 was found to be 18.65 and 21.53 for cases 8 and 9, respectively. The Cq Mean for ACTB was 18.90 and 22.37 for cases 8 and 9, respectively (both T-ALL); the human reference control gave a Cq Mean of 20.01.
Discussion
Rearrangements of chromosomal band 9q34 are common and important in the genesis and classification of leukemias (‘https://mitelmandatabase.isb-cgc.org/’). The most common gene-fusion result by far is the BCR::ABL1 generated by the t(9;22)(q34;q11) translocation that is specific for chronic myeloid leukemias but also occurs in Ph-positive acute leukemias. Other leukemia-relevant genes mapping to 9q34 include SET and NUP214. The differentiation between these rearrangements has important therapeutic implications. G-banding and/or FISH investigations can hint at gene fusions generated by inter-chromosomal rearrangements, e.g., the t(6;9) leading to DEK::NUP214 fusion; however, this is not the case with cryptic intra-chromosomal rearrangements such as SET::NUP214 and NUP214::ABL1 (all three genes map within 9q34). Furthermore, standard diagnostic FISH analyses using commercial dual fusion probes for BCR::ABL1 (used whenever CML or ALL is suspected) may sometimes show an abnormal signal pattern for ABL1 (mostly the presence of an extra signal) but no fusion with BCR. Next generation sequencing, at both DNA and RNA level, is an effective method to screen for presence of cryptic rearrangements/fusion genes. However, the commercial panels available for diagnostic purposes have specific targets and may therefore miss less common gene-fusions. Furthermore, although the screening ability of NGS technologies is indisputable; its use in standard leukemia diagnostics is still limited. We here present a combination of cytogenetic and molecular methods that can be used in a stepwise manner to identify cryptic rearrangements in neoplastic cells.
NUP214 codes for a nuclear pore complex (NPC) component with a key role in the cell cycle, including mRNA and nuclear protein export between the nucleus and cytoplasm (2, 14). The gene has been found rearranged with several partners in leukemias. The most frequent partner is DEK which maps to 6p22 and codes for a chromatin-remodeling protein participating in transcription regulation (15). DEK::NUP214 fusion (16) as a result of a seemingly balanced t(6;9)(p22;q34) is typical of AML preceded by a short myelodysplastic phase (‘https://mitelmandatabase.isb-cgc.org/’). Alternative partners for NUP214 are SET, which maps to 9q34 and codes for a chromatin-remodeling protein with roles in transcription regulation (2), and the proto-oncogene ABL1 which encodes a protein tyrosine kinase involved in many cellular processes, including cell growth and survival (17–21 ). The SET::NUP214 fusion (22) can be generated by either the cryptic translocation t(9;9)(q34;q34) or an intra-chromosomal rearrangement such as the deletion del(9)(q34.11q34.13); it is mostly found in patients with AUL and T-ALL (1, 2, 19, 22). A NUP214::ABL1 (23) chimeric transcript usually corresponds to a T-lineage ALL phenotype (23, 24), and patients with this fusion gene can be effectively treated with tyrosine kinase inhibitors (TKI) (25, 26). Less frequent partners for NUP214 are the Sequestosome 1 (SQSTM1) gene at 5q35, involved mostly in T-ALLs (27), and the Ras-related C3 botulinum toxin substrate 1 gene (RAC1) at 7p22 in AML (28).
Patients with DEK::NUP214 account for around 1% of all AML cases (1) and include both children (mean age 10 years) and adults (mean age 45 years) (29, 30); our data are in accordance with previous literature as DEK::NUP214fusion was present in four adults and a 15-year-old girl with AML. The exact role of DEK::NUP214 in leukemogenesis is still unknown, but Ageberg et al. showed that it could promote phosphorylation of the eukaryotic translation initiation factor 4E (eIF4E) and activation of the mTOR signaling pathway, increasing myeloid cell proliferation (31, 32).
SET::NUP214 was found in two patients, with ALAL-NOS and AUL, respectively, (cases 6 and 7). In both cases, FISH analysis using an ABL1 probe showed only one fluorescent signal on 9q34/ABL1. The genomic imbalances detected by aCGH included loss of a genomic region at 9q34 with breakpoints inside the above-mentioned genes (SET and NUP214). RT-PCR, followed by sequencing, detected the SET::NUP214 fusion gene in both cases. The mechanism by which SET::NUP214 mediates leukemogenesis has not been fully elucidated, but the chimeric gene encodes a fusion protein that inhibits cell apoptosis by cytotoxic T-cells (33).
NUP214 showed the same breakpoint (exon 18) in both types of fusion (DEK::NUP214 and SET::NUP214), indicating that a similar pathogenetic mechanism, namely deregulation of the DEK and SET histone binding proteins, could be critical leading to abnormal histone hypoacetylation and silencing of genes important for lineage commitment and hematopoietic differentiation (2).
FISH analysis in case 8 and 9 using a commercial ABL1 probe showed amplification of the gene. Graux et al. (23) were the first to show that, in such cases, the ABL1 gene is involved in a cryptic rearrangement with NUP214 creating from one to multiple extrachromosomal episome(s) containing the NUP214::ABL1 fusion genes not detectable by karyotyping. RT-PCR confirmed the presence of a 9q34 rearrangement resulting in the fusion of exon 29 of NUP214 with exon 2 of ABL1 in case 9, and fusion of exon 31 of NUP214 with exon 2 of ABL1 in case 8. This is the first time that a NUP214::ABL1 fusion gene was found together with a PCM1::FGFR1 transcript (case 8). The patient was a 23-year-old previously healthy man diagnosed with near, early precursor T-cell leukemia (near ETP-ALL) after 2-3 weeks with night sweats, weight loss, and enlarging lymph nodes on his neck. Upon admission, anemia and thrombocytopenia were noted and leukocytes were increased to 106 x 109/L (normal range 3-10).
The final diagnosis in this case was made based on examination of the bone marrow and a lymph node biopsy. Treatment with dexamethasone, vincristine, doxorubicine, asparginase, methotrexate, and mercaptopurine according to European ALL Together protocol was then started (34), whereas imatinib was added afterwards. The patient became minimal residual disease (MRD) negative and achieved complete remission on day 78. The second gene fusion in this case, PCM1::FGFR1, was also described by Kasbeker et al. (35 ) in a patient suffering from a rare, aggressive myeloid neoplasia with eosinophilia; treatment with futibatinib, an oral selective small molecule inhibitor of FGFR1-4, resulted in complete hematologic and cytogenetic remission (35). The PCM1 gene as partner in the chimera PCM1::JAK2 has been implicated in the pathogenesis of also other acute myeloid/lymphoid neoplasms (36) whereas rearrangements of FGFR1 are rare (37). The use of selective TKIs can potentially affect outcomes in these patients (35, 37). Because FGFR inhibitors may be used as first-line therapy for patients with myeloid/lymphoid neoplasms with FGFR1 rearrangement, gene-level diagnosis testing for such aberrations is clinically important (35). Finally, the detection of both NUP214::ABL1 and PCM1::FGFR1 fusions in the near ETP-ALL of case 8 leaves no information as to which fusion was the primary and which was secondary in this leukemia.
Aberrant expression of transcription factor oncogenes such as TLX3 plays a major role in the pathogenesis of T-ALL and defines distinct molecular groups with prognostic significance (38). Our two T-ALL cases were also characterized by aberrant expression of TLX3. T-ALL patients co-expressing TLX3 and NUP214::ABL1 fusion transcript may belong to a subgroup of poor survival (39). However, data are still too limited to conclude.
Finally, some methodological comments on the scoring principles of aCGH analyses may be warranted. Many laboratories, including ours, follow standard guidelines/recommendations (9, 40, 41) as to the size of which imbalances to score and which not. If one sticks to a requirement of size more than 5 Mb for any imbalance to be paid attention to, one risks overlooking small but important changes. This would have been the case for patients 6, 7, 8, and 9 of our series. Therefore, when determining the scoring of imbalances, each clinical laboratory applies its own professional judgment to the specific circumstances presented by individual patients or specimens, reporting all the imbalances deemed clinically relevant; this is also fundamental to avoid false positives in case of low-quality results. Based on our experience and the results reported here, we recommend checking and reporting all imbalances, irrespective of their size. One should also note which genes are affected so as to identify different pathways leading to the same phenotypic disease, or even identify possible therapeutic approaches associated with the rearrangement of specific genes in other contexts.
In conclusion, the use of different methodological approaches - whenever possible a combination of karyotyping, FISH and molecular methods - is often necessary to detect genomic NUP214 rearrangements in leukemic cells. The identification of a specific NUP214 rearrangement is fundamental to choosing an optimal therapeutic strategy: by way of example, patients with DEK::NUP214 are classified as an adverse risk group within AML and might be considered candidates for allogenic bone marrow transplantation.
Data availability statement
The raw data supporting the conclusions of this article will be made available by the authors, without undue reservation.
Ethics statement
The studies were conducted in accordance with the local legislation and institutional requirements. Written informed consent for participation in this study was provided by the participants’ legal guardians/next of kin.
Author contributions
MB: Writing – review & editing, Writing – original draft, Validation, Supervision, Resources, Methodology, Formal analysis, Data curation, Conceptualization. KA: Writing – review & editing, Methodology, Formal analysis. SS: Writing – review & editing, Data curation. AL: Writing – review & editing, Data curation. LO: Writing – review & editing, Data curation. HV: Writing – review & editing, Methodology. SH: Writing – review & editing. FM: Writing – review & editing, Writing – original draft, Visualization, Supervision, Project administration, Funding acquisition, Data curation, Conceptualization.
Funding
The author(s) declare that financial support was received for the research, authorship, and/or publication of this article. This research was funded by The Norwegian Radium Hospital Foundation (Radiumhospitalets legater).
Acknowledgments
The authors wish to thank the diagnostic group at the Section for Cancer Cytogenetics, Institute for Cancer Genetics and Informatics, Oslo University Hospital, for technical assistance.
Conflict of interest
The authors declare that the research was conducted in the absence of any commercial or financial relationships that could be construed as a potential conflict of interest.
Publisher’s note
All claims expressed in this article are solely those of the authors and do not necessarily represent those of their affiliated organizations, or those of the publisher, the editors and the reviewers. Any product that may be evaluated in this article, or claim that may be made by its manufacturer, is not guaranteed or endorsed by the publisher.
Supplementary material
The Supplementary Material for this article can be found online at: https://www.frontiersin.org/articles/10.3389/fonc.2024.1371980/full#supplementary-material
References
1. Swerdlow SH, Campo E, Harris NL, Jaffe ES, Pileri SA, Stein H, et al. WHO classification of tumours of haematopoietic and lymphoid tissues, revised 4th edition ed. Lyon, France (2017).
2. Mendes A, Fahrenkrog B. NUP214 in leukemia: it’s more than transport. Cells. (2019) 8. doi: 10.3390/cells8010076
3. Rose-Inman H, Kuehl D. ‘Acute leukemia’. Hematology/oncology Clinics North America. (2017) 31:1011–28. doi: 10.1016/j.hoc.2017.08.006
4. Fleischmann M, Schnetzke U, Hochhaus A, Scholl S. Management of acute myeloid leukemia: current treatment options and future perspectives. Cancers (Basel). (2021) 13. doi: 10.3390/cancers13225722
5. Czepulkowski B, Bhatt B, Rooney D. ‘Basic techniques for the preparation and analysis of’. Hum cytogenetics: Malignancy acquired abnormalities. (1992) 2:1.
6. Brunetti M, Zeller B, Tierens A, Heim S, Micci F, Panagopoulos I. ‘TYRO3 truncation resulting from a t(10;15)(p11;q15) chromosomal translocation in pediatric acute myeloid leukemia’. Anticancer Res. (2020) 40:6115–21. doi: 10.21873/anticanres.14632
7. McGowan-Jordan J, Hastings RJ, Moore S. ISCN 2020: An International system for human cytogenomic nomenclature. Basel, Switzerland (2020). p. 164.
8. Olshen AB, Venkatraman ES, Lucito R, Wigler M. ‘Circular binary segmentation for the analysis of array-based DNA copy number data’. Biostatistics. (2004) 5:557–72. doi: 10.1093/biostatistics/kxh008
9. Schoumans J, Suela J, Hastings R, Muehlematter D, Rack K, van den Berg E, et al. ‘Guidelines for genomic array analysis in acquired haematological neoplastic disorders’. Genes Chromosomes Cancer. (2016) 55:480–91. doi: 10.1002/gcc.22350
10. Brunetti M, Panagopoulos I, Kostolomov I, Davidson B, Heim S, Micci F. ‘Mutation analysis and genomic imbalances of cells found in effusion fluids from patients with ovarian cancer’. Oncol Lett. (2020) 20:2273–9. doi: 10.3892/ol
11. Altschul SF, Gish W, Miller W, Myers EW, Lipman DJ. ‘Basic local alignment search tool’. J Mol Biol. (1990) 215:403–10. doi: 10.1016/S0022-2836(05)80360-2
13. Quist-Paulsen P, Toft N, Heyman M, Abrahamsson J, Griškevičius L, Hallböök H, et al. ‘T-cell acute lymphoblastic leukemia in patients 1-45 years treated with the pediatric NOPHO ALL2008 protocol’. Leukemia. (2020) 34:347–57. doi: 10.1038/s41375-019-0598-2
14. Borden KLB. The nuclear pore complex and mRNA export in cancer. Cancers (Basel). (2020) 13. doi: 10.3390/cancers13010042
15. Alexiadis V, Waldmann T, Andersen J, Mann M, Knippers R, Gruss C. ‘The protein encoded by the proto-oncogene DEK changes the topology of chromatin and reduces the efficiency of DNA replication in a chromatin-specific manner’. Genes Dev. (2000) 14:1308–12. doi: 10.1101/gad.14.11.1308
16. Soekarman D, von Lindern M, Daenen S, de Jong B, Fonatsch C, Heinze B, et al. ‘The translocation (6;9) (p23;q34) shows consistent rearrangement of two genes and defines a myeloproliferative disorder with specific clinical features’. Blood. (1992) 79:2990–7. doi: 10.1182/blood.V79.11.2990.bloodjournal79112990
17. Sandahl JD, Coenen EA, Forestier E, Harbott J, Johansson B, Kerndrup G, et al. ‘t(6;9)(p22;q34)/DEK-NUP214-rearranged pediatric myeloid leukemia: an international study of 62 patients’. Haematologica. (2014) 99:865–72. doi: 10.3324/haematol.2013.098517
18. Ommen HB, Touzart A, MacIntyre E, Kern W, Haferlach T, Haferlach C, et al. ‘The kinetics of relapse in DEK-NUP214-positive acute myeloid leukemia patients’. Eur J Haematol. (2015) 95:436–41. doi: 10.1111/ejh.12511
19. Zhu HH, Zhao XS, Qin YZ, Lai YY, Jiang H. B-cell acute lymphoblastic leukemia associated with SET-NUP214 rearrangement: A case report and review of the literature. Oncol Lett. (2016) 11:2644–50. doi: 10.3892/ol.2016.4260
20. Panagopoulos I, Gorunova L, Torkildsen S, Tjønnfjord GE, Micci F, Heim S. ‘DEK-NUP214-fusion identified by RNA-sequencing of an acute myeloid leukemia with t(9;12)(q34;q15)’. Cancer Genomics Proteomics. (2017) 14:437–43. doi: 10.21873/cgp
21. Kim B, Kim E, Lee ST, Cheong JW, Lyu CJ, Min YH, et al. ‘Detection of recurrent, rare, and novel gene fusions in patients with acute leukemia using next-generation sequencing approaches’. Hematol Oncol. (2020) 38:82–8. doi: 10.1002/hon.2709
22. Van Vlierberghe P, van Grotel M, Tchinda J, Lee C, Beverloo HB, van der Spek PJ, et al. ‘The recurrent SET-NUP214 fusion as a new HOXA activation mechanism in pediatric T-cell acute lymphoblastic leukemia’. Blood. (2008) 111:4668–80. doi: 10.1182/blood-2007-09-111872
23. Graux C, Cools J, Melotte C, Quentmeier H, Ferrando A, Levine R, et al. ‘Fusion of NUP214 to ABL1 on amplified episomes in T-cell acute lymphoblastic leukemia’. Nat Genet. (2004) 36:1084–9. doi: 10.1038/ng1425
24. Mitelman F, Johansson B, Mertens F. Mitelman database of chromosome aberrations and gene fusions in cancer. (2023).
25. Quintás-Cardama A, Tong W, Manshouri T, Vega F, Lennon PA, Cools J, et al. ‘Activity of tyrosine kinase inhibitors against human NUP214-ABL1-positive T cell Malignancies’. Leukemia. (2008) 22:1117–24. doi: 10.1038/leu.2008.80
26. Clarke S, O’Reilly J, Romeo G, Cooney J. ‘NUP214-ABL1 positive T-cell acute lymphoblastic leukemia patient shows an initial favorable response to imatinib therapy post relapse’. Leuk Res. (2011) 35:e131–3. doi: 10.1016/j.leukres.2011.03.025
27. Gorello P, La Starza R, Di Giacomo D, Messina M, Puzzolo MC, Crescenzi B, et al. ‘SQSTM1-NUP214: a new gene fusion in adult T-cell acute lymphoblastic leukemia’. Haematologica. (2010) 95:2161–3. doi: 10.3324/haematol.2010.029769
28. Abe A, Yamamoto Y, Iba S, Okamoto A, Tokuda M, Inaguma Y, et al. ‘NUP214-RAC1 and RAC1-COL12A1 fusion in complex variant translocations involving chromosomes 6, 7 and 9 in an acute myeloid leukemia case with DEK-NUP214’. Cytogenet Genome Res. (2015) 146:279–84. doi: 10.1159/000441464
29. von Lindern M, Fornerod M, van Baal S, Jaegle M, de Wit T, Buijs A, et al. ‘The translocation (6;9), associated with a specific subtype of acute myeloid leukemia, results in the fusion of two genes, dek and can, and the expression of a chimeric, leukemia-specific dek-can mRNA’. Mol Cell Biol. (1992) 12:1687–97. doi: 10.1128/MCB.12.4.1687
30. Heim S, Mitelman F. Acute myeloid leukemia. In: Chromosomal and molecular genetic aberration of tumor cells. Chichester, West Sussex: Wiley-Blackwell (2015).
31. Ageberg M, Drott K, Olofsson T, Gullberg U, Lindmark A. ‘Identification of a novel and myeloid specific role of the leukemia-associated fusion protein DEK-NUP214 leading to increased protein synthesis’. Genes Chromosomes Cancer. (2008) 47:276–87. doi: 10.1002/gcc.20531
32. Sandén C, Ageberg M, Petersson J, Lennartsson A, Gullberg U. ‘Forced expression of the DEK-NUP214 fusion protein promotes proliferation dependent on upregulation of mTOR’. BMC Cancer. (2013) 13:440. doi: 10.1186/1471-2407-13-440
33. Zhou MH, Yang QM. ‘NUP214 fusion genes in acute leukemia (Review)’. Oncol Lett. (2014) 8:959–62. doi: 10.3892/ol.2014.2263
34. ClinicalTrials.gov. A treatment protocol for participants 0-45 years with acute lymphoblastic leukaemia. (2023).
35. Kasbekar M, Nardi V, Dal Cin P, Brunner AM, Burke M, Chen YB, et al. ‘Targeted FGFR inhibition results in a durable remission in an FGFR1-driven myeloid neoplasm with eosinophilia’. Blood Adv. (2020) 4:3136–40. doi: 10.1182/bloodadvances.2020002308
36. Kaplan HG, Jin R, Bifulco CB, Scanlan JM, Corwin DR. ‘PCM1-JAK2 fusion tyrosine kinase gene-related neoplasia: A systematic review of the clinical literature’. Oncologist. (2022) 27:e661–70. doi: 10.1093/oncolo/oyac072
37. Strati P, Tang G, Duose DY, Mallampati S, Luthra R, Patel KP, et al. ‘Myeloid/lymphoid neoplasms with FGFR1 rearrangement’. Leuk Lymphoma. (2018) 59:1672–6. doi: 10.1080/10428194.2017.1397663
38. Van Vlierberghe P, Ferrando A. ‘The molecular basis of T cell acute lymphoblastic leukemia’. J Clin Invest. (2012) 122:3398–406. doi: 10.1172/JCI61269
39. Ballerini P, Landman-Parker J, Cayuela JM, Asnafi V, Labopin M, Gandemer V, et al. ‘Impact of genotype on survival of children with T-cell acute lymphoblastic leukemia treated according to the French protocol FRALLE-93: the effect of TLX3/HOX11L2 gene expression on outcome’. Haematologica. (2008) 93:1658–65. doi: 10.3324/haematol.13291
40. Cooley LD, Lebo M, Li MM, Slovak ML, Wolff DJ. ‘American College of Medical Genetics and Genomics technical standards and guidelines: microarray analysis for chromosome abnormalities in neoplastic disorders’. Genet Med. (2013) 15:484–94. doi: 10.1038/gim.2013.49
41. Mikhail FM, Biegel JA, Cooley LD, Dubuc AM, Hirsch B, Horner VL, et al. ‘Technical laboratory standards for interpretation and reporting of acquired copy-number abnormalities and copy-neutral loss of heterozygosity in neoplastic disorders: a joint consensus recommendation from the American College of Medical Genetics and Genomics (ACMG) and the Cancer Genomics Consortium (CGC)’. Genet Med. (2019) 21:1903–16. doi: 10.1038/s41436-019-0545-7
Keywords: acute leukemias, rare leukemias, cytogenetics, 9q34-NUP214, fluorescence in situ hybridization, array comparative genomic hybridization, fusion gene
Citation: Brunetti M, Andersen K, Spetalen S, Lenartova A, Osnes LTN, Vålerhaugen H, Heim S and Micci F (2024) NUP214 fusion genes in acute leukemias: genetic characterization of rare cases. Front. Oncol. 14:1371980. doi: 10.3389/fonc.2024.1371980
Received: 17 January 2024; Accepted: 08 March 2024;
Published: 20 March 2024.
Edited by:
Mohamed A. Yassin, Qatar University, QatarReviewed by:
Gisela Barbany, Karolinska University Laboratory, SwedenIrina Golovleva, Umeå University, Sweden
Copyright © 2024 Brunetti, Andersen, Spetalen, Lenartova, Osnes, Vålerhaugen, Heim and Micci. This is an open-access article distributed under the terms of the Creative Commons Attribution License (CC BY). The use, distribution or reproduction in other forums is permitted, provided the original author(s) and the copyright owner(s) are credited and that the original publication in this journal is cited, in accordance with accepted academic practice. No use, distribution or reproduction is permitted which does not comply with these terms.
*Correspondence: Marta Brunetti, brunetti.marta90@gmail.com