- 1Department of Radiation Oncology, Miami Cancer Institute, Baptist Health South Florida, Miami, FL, United States
- 2Herbert Wertheim College of Medicine, Florida International University, Miami, FL, United States
In the current era of high-precision radiation therapy, real-time magnetic resonance (MR)-guided tracking of the tumor and organs at risk (OARs) is a novel approach that enables accurate and safe delivery of high-dose radiation. Organ tracking provides a general sense of the need for daily online adaptation but lacks precise information regarding exact dosimetry. To overcome this limitation, we developed the methodology for monitoring intrafraction motion with real-time MR-guided isodose line-based tracking of an OAR in combination with anatomic tumor-based tracking and reported the first case treated with this approach. An isolated para-aortic (PA) nodal recurrence from carcinosarcoma of the endometrium was treated with an ablative dose of 50 Gy in five fractions using MR-guided radiotherapy (MRgRT). This report demonstrates the feasibility, workflow, dosimetric constraints, and treatment paradigm for real-time isodose line-based OAR tracking and gating to enable an isotoxicity delivery approach. This innovative treatment strategy effectively tracked the intrafraction motion of both the target and OAR independently and enhanced the accuracy of structure localization in time and space with a more precise dosimetric evaluation.
Introduction
Radiation dose escalation has been highly effective in achieving high rates of durable local control for several tumors, which can further cause improved overall survival (1–3). However, the delivery of ablative doses is often compromised by the proximity of certain organs at risk (OARs), and this has been a major limiting factor, especially when intrafraction organ motion adds a layer of dosimetric uncertainty, as is frequently observed in the thorax and abdomen. Given the lack of correlation between surface changes and internal organ position, location, and movement, patient surface anatomy cannot be used as a reliable surrogate for the internal motion of the thorax and upper abdominal organs (4).
Real-time tracking and automatic gating of radiation delivery is an effective solution to spare the OARs and mitigate treatment-related toxicity. This enables the safe delivery of ablative doses to the gastrointestinal (GI)/pelvic structures, which was previously impractical due to limited visualization and intrafraction motion. Magnetic resonance-guided radiation therapy (MRgRT) enables online adaptation to customize the radiation to the anatomy of the day, addressing both intra- and interfraction anatomic variation while providing excellent soft tissue visualization. The ability to continuously track in real time with MR imaging addresses the uncertainty associated with intrafraction motion (5), while eliminating the need for invasive procedures such as internal fiducials (required for x-ray-based real-time tracking) or electromagnetic transponders (6). It also eliminates the need to expand the true target to account for motion using the concept of an internal target volume (ITV) and thereby reduces the required setup margin, therefore exposing less normal tissue to unnecessary radiation doses (7).
In contrast to conventional radiation therapy, where homogeneous target coverage is the primary objective, isotoxic dose escalation increases the dose to the target volume until the pre-selected adjacent OAR dose constraint is reached. An isotoxic approach is generally applied when certain OARs are in proximity to the target volume, and these OARs are constrained to a lower dose level than the target volume. As such, this isotoxicity approach is characterized by heterogeneous target coverage, with a higher dose covering the core of the target [usually the gross tumor volume (GTV)] (8). Furthermore, this approach necessitates ensuring a rapid dose falloff of the ablative doses from the core to the periphery of the target, so as to further minimize OAR doses in close proximity. Because of the anatomically constrained dosimetric gradients between the OARs and target volumes in such cases, it is essential to ensure that the motion of the OAR-target geometry is minimized and/or accounted for in isotoxicity planning and delivery.
Liu et al. implemented a multitarget MLC-based motion tracking system for MRgRT that can simultaneously track two independently moving structures and gate the radiation beam in real time, compensating for motion (9). This approach can be used to track the tumor and adjacent dose-limiting OARs concurrently. Such an approach makes isotoxic dose escalation in real time feasible, thus broadening the therapeutic window. MRgRT provides continuous real-time cine MR imaging of the radiation field and is deployed utilizing a simple target margin, i.e., a boundary, for standard anatomic tracking and gating. The addition of isodose line-based tracking can further enhance the intrafraction motion accuracy beyond the use of simple anatomic margin expansion, as has been used historically in MRgRT.
This is the first report to describe the feasibility and workflow of this innovative approach to dose-guided tracking of GI OAR using MRgRT. Additionally, we report the institutional dose constraints for abdominal and pelvic targets to ablative doses (50 Gy in five fractions) and time analysis for the current workflow.
Case description
A 63-year-old woman was initially diagnosed with stage IB carcinosarcoma of the endometrium in September 2020. She was treated with robotic total laparoscopic hysterectomy with bilateral salpingo-oophorectomy and bilateral pelvic sentinel lymph node dissection, followed by high-dose-rate intracavitary brachytherapy to the upper vagina (21 Gy in three fractions of 7 Gy prescribed to 0.5 cm depth), and completed six cycles of adjuvant carboplatin/paclitaxel chemotherapy in February 2021. A follow-up positron emission tomography–computed tomography (PET‐CT) scan in June 2022 revealed an oligometastatic disease in the left para-aortic (PA) node (1.1 cm), which has further enlarged to 1.3 cm by October 2022, with an increase in maximum standardized uptake value (SUVmax) from 5.75 to 10.9.
A multi-disciplinary discussion at our institutional gynecology tumor board recommended treating isolated nodal recurrence with ablative radiation therapy. Clinically, she reported intermittent rectal pain and sporadic rectal bleeding, with the last occurrence 1 month before. She was also diagnosed with co-existing acute inflammatory bowel disease (IBD), which raised the concern of severe acute GI toxicity if treated with conventional abdominal and pelvic radiation fields. Therefore, online adaptive stereotactic body radiation therapy (SBRT) was recommended at a dose of 50 Gy in five fractions on the MRIdian system (ViewRay, Cleveland, OH, USA). The MRIdian system combines a 0.35-T MR scanner with a 6-MV flattening filter-free Linac and a double-stacked, double-focused MLC offset by half a leaf width, enabling a leaf resolution of 4.15 mm at the isocenter (10). The patient was included in an institutional review board (IRB)-approved protocol.
Treatment description
Simulation and initial planning
The simulation was performed under mid-inspiration breath hold in the supine position with both arms at her sides to ensure patient comfort. The planning MR scan was acquired on the MRIdian at 50 × 50 × 35.8 cm3 field of view (FOV) with a resolution of 1.5 × 1.5 × 3.0 mm3. The planning MR scan acquired for simulation and also the scan at each fraction for adaptive re-planning were a balanced steady-state free precession (TrueFISP) sequence with a maximum distortion of <1.0 mm and <2.0 mm within 5 cm and 17.5 cm of the isocenter, respectively (11). Patient devices included a foam pad and wing board since continuous MR imaging is available for motion management.
Segmentation and treatment planning were performed on the MR simulation scan. The GTV was defined as the tumor visualized on the TrueFISP MR, and the clinical target volume (CTV) was delineated as GTV with a 2-mm isotropic margin, excluding extension into the vertebral body posteriorly, and then uniformly expanded by a 3-mm margin to create the planning target volume (PTV), which was prescribed to 50 Gy (PTV50). A simultaneous integrated boost (SIB) technique was used, and a second CTV prescribed to 30 Gy (CTV30) was delineated by the PA chain as defined from the renal vessels (superiorly) to the aortic bifurcation (inferiorly), following the RTOG consensus (12). A 3-mm uniform setup margin expansion of the CTV30 was used to create the PTV30. Relevant OARs segmented included the duodenum, small bowel, large bowel, stomach, kidneys, liver, spinal canal, and cauda equina. Figure 1 depicts the simulation anatomy and dose distribution on the TrueFISP MR scan.
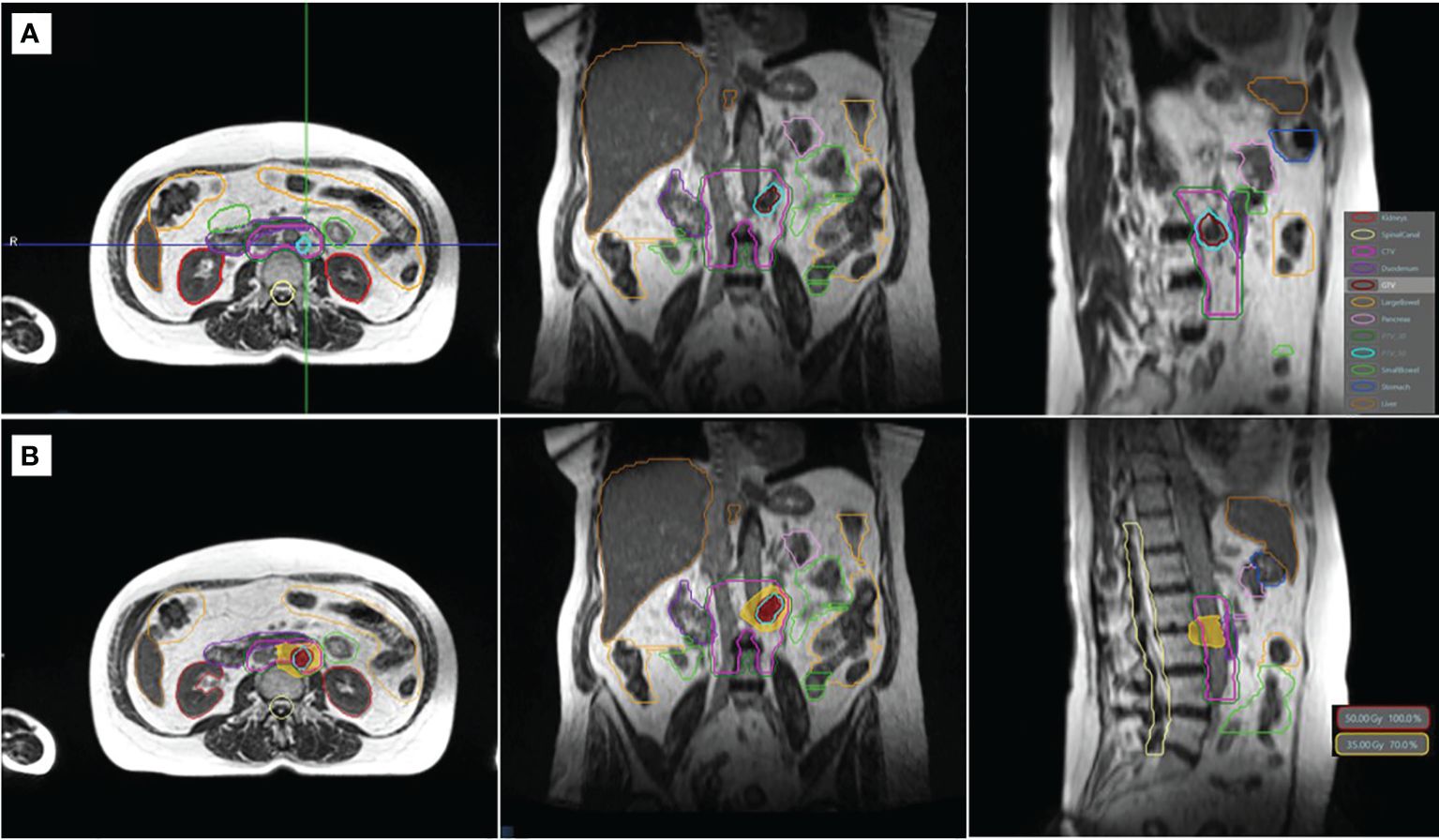
Figure 1 TrueFISP MR scan showing the simulation anatomy (A) and the dose distribution of the original plan (B). Relevant organs at risk and targets are shown in outline (A). The isodose lines of the prescription (50 Gy) and gastrointestinal organ-at-risk constraint (35 Gy) are shown in colorwash (B).
Our planning technique, which has been previously described in the literature, utilized subdivision of the PTV50 to differential dosing based on a nonoverlapping region of the PTV50 with a GI planning OAR volume (PRV) (8). To this end, a GI PRV was created as an optimization structure to define the dose falloff between the proximal GI OARs and the target. The GI PRV was defined by a 3-mm isotopic expansion of the union of the stomach, duodenum, small bowel, and large bowel. Any overlapping portion of the GTV and PTV50 by the GI PRV was optimized to achieve 25–35 Gy. The non-overlapping portion of the GTV and PTV50 with the GI PRV was defined as GTVopt and PTV50opt, respectively, and optimized to achieve at least 50 Gy. Further details on the optimization and adaptive robustness of this planning technique have been previously reported (8).
A 15-field step-and-shoot intensity-modulated radiation therapy (IMRT) arrangement with 40 segments was created with a Monte Carlo dose calculation algorithm using a 2-mm isotropic dose grid size. A deformed CT approach was used to map the electron density. Manual edits were applied as needed to correct for GI luminal gas as air and/or tissue. Note that the CT scan was performed in mid-inspiration breath hold and acquired on the same day as the simulation MR scan.
A recent hardware and software upgrade to the MRIdian system was released for clinical use (A3i, 510K approval, December 2021) and included an updated user interface with an online adaptive parallel workflow and updated gating protocols that enable multi-planar tracking capabilities. Specifically, the MR multi-planar tracking capabilities now include anatomical tracking and/or dose-guided tracking for real-time gating. With these novel A3i features, we chose to implement “two planar” tracking for this patient: dose-guided tracking of the GI luminal OAR and anatomical tracking of the target in the sagittal and coronal planes, respectively. Owing to the patient’s history of IBD and its close proximity TO the target volume, duodenum was the prioritized OAR in this case to minimize the risk of acute inflammatory flare-ups or late effects like ulceration, perforation, or fibrosis.
Online adaptation and isotoxicity planning
Our institutional online adaptive MRgRT workflow has been reported previously (1). Target volumes were rigidly registered, and OARs were deformably registered from the simulation MR to the daily volumetric MR scan. All OARs within 2 cm axially and 3 cm craniocaudally of the PTV were reviewed and manually edited by the radiation oncologist. Manual electron density edits were performed on the deformed CT to match the anatomy of the daily MR scan.
Following segmentation, a predicted plan was calculated using the original plan, generated using the simulated anatomy, and superimposed on the current anatomy and contours of the day to evaluate the indication for adaptation. The predicted plan was considered sufficient if all OARs and target coverage metrics were achieved based on the individualized treatment planning directive. If the predicted plan failed for any metric (i.e., under-coverage of the target volume or overdose to any OAR), an adaptive plan was then generated to meet OAR constraints and/or improve target coverage. The first priority was to ensure that the OAR constraints were met, and target coverage was a secondary goal for isotoxic planning.
Gated treatment
Real-time tracking was performed on the coronal and sagittal planes. For this particular patient, a novel gating scheme of dose-guided tracking utilized the MRIdian A3i features. To this end, we chose to track the duodenum, the most proximal GI OAR, in the sagittal plane, since it seemed ideal to track the OAR with respect to the pre-specified border of the selected isodose line. Meanwhile, the coronal plane was used to track and ensure the target coverage.
Specifically for the sagittal plane tracking, a boundary was defined such that the gating threshold would be that no more than 5% of the duodenum overlapping within the 35-Gy isodose line. This technique was selected for this case since we utilized a dose escalation approach with isotoxicity normalization to the proximal GI luminal OAR, i.e., the duodenum. Figure 2 demonstrates the intrafraction gating with respiration tracked in the sagittal plane with an earlier overlap between the 35-Gy isodose line and the duodenum (A), and a later separation is observed when the patient is in an adequate breath hold (B).
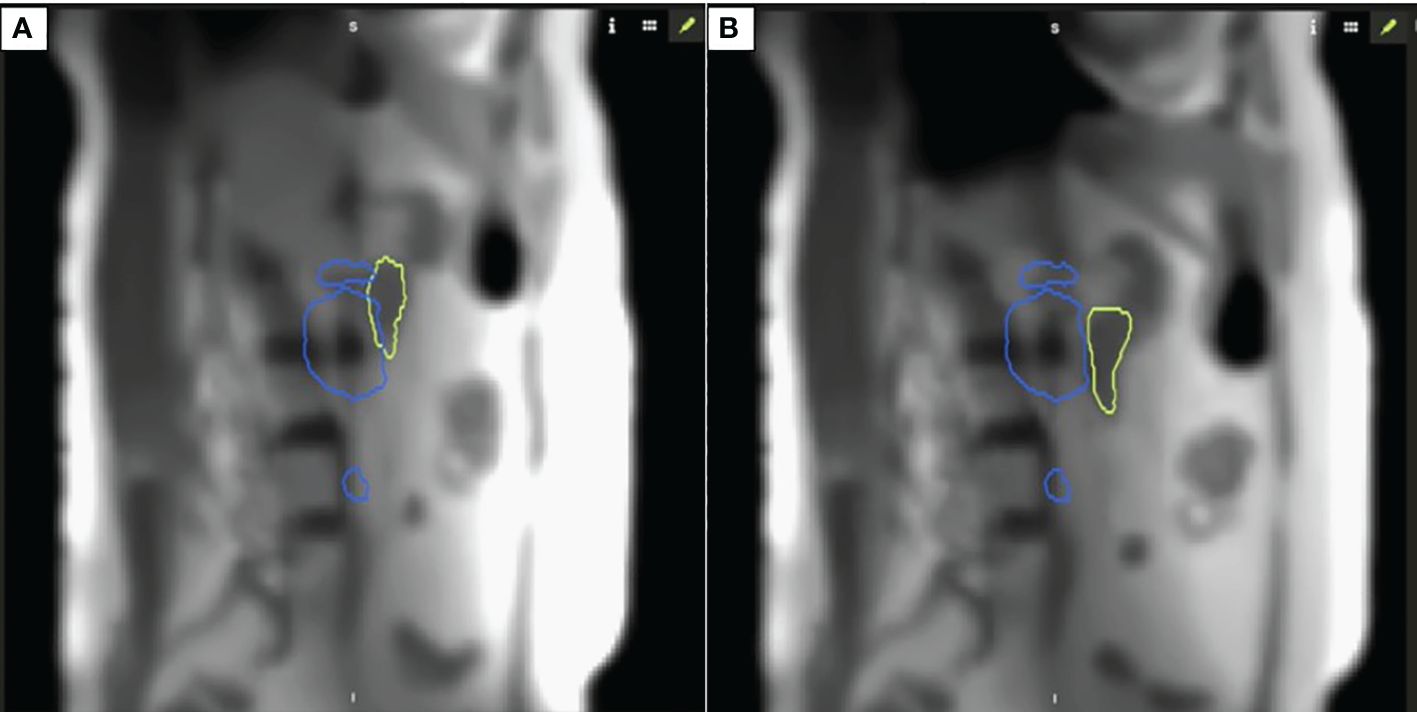
Figure 2 Intrafraction motion with respiration (A) demonstrates an overlap between 35 Gy isodose line and duodenum; (B) demonstrates separation between the organ-at-risk and respective constraint isodose line when patient is in appropriate breath hold for isotoxic delivery.
Additionally, we tracked the gross target on the coronal plane through a tracking structure that approximated the GTV. The target tracking region of interest (ROI) was contoured daily based on the contrast differences of the daily MR to enable the highest accuracy for the deformable image registration algorithm during cine imaging. The sagittal target tracking ROI had a 3-mm boundary margin such that when the deformed tracking ROI moved outside the boundary more than 5%, the beam would automatically be withheld.
The cine image acquisition was selected as a cartesian sequence at a nominal 4 frames per second (FPS). Note that the cine-temporal resolution is dependent on the FOV. For this case, the achieved frame rate was 3 FPS after FOV adjustments. An FOV of 27.5 × 42 × 0.70 cm and a spatial resolution of 0.35 × 0.35 cm were used, which enabled a frame rate of at least 3 FPS during treatment for each respective plane. Cine imaging was acquired in repeated sequential order between sagittal and coronal orientations. The treatment delivery was a step-and-shoot IMRT, and as such, continuous MR cine imaging occurred for each gantry treatment position. Note that in this isotoxic gating approach, the treatment beam is automatically gated on/off based on the tumor position and duodenum position relative to the high dose gradient. No real-time intra-fraction adaptation was performed based on the duodenum position during treatment delivery. Online adaptation was only performed once per fraction based on the daily volumetric MR scan prior to delivery. Figure 3 demonstrates the timeline for this patient for each fraction. The median total in-room time was 58 min [range (R): 46–76 min] with the longest on fraction 1 and the shortest on fraction 5. The multi-planar tracking and the breath hold did not substantially increase the treatment time compared to prior literature, with the observed median duration of radiation delivery being 15 min (R: 13–20 min).
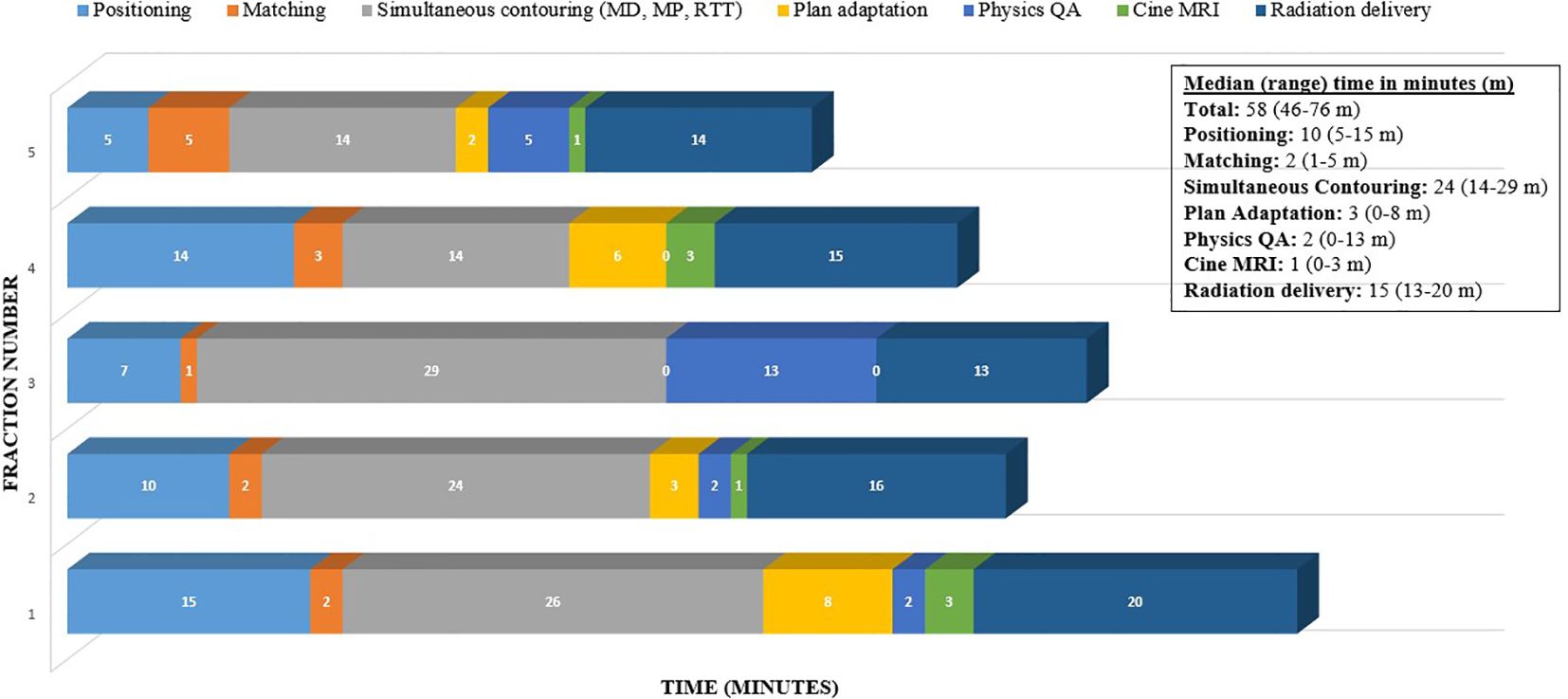
Figure 3 Time (minutes) taken through the workflow for online adaptive MRgRT for this patient case example (5 fractions).
Follow-up
She completed the planned treatment course, with Common Terminology Criteria for Adverse Events (CTCAE) grade 1 nausea being the only acute toxicity. The follow-up PET-CT 3 at months and 6 months post-treatment confirmed complete metabolic and anatomic resolution of the node. With a follow-up at 12 months post-radiation, the patient had durable local control of the treated lesion and the entire PA chain. She has not developed any further late GI toxicity but had one out-of-field failure (left external iliac lymph node). This was also treated with SBRT using MRIdian; however, owing to its quite posterior position and the absence of any abutting GI OAR with respiratory-induced motion, no GI OAR management was needed during cine tracking, and only multi-planar anatomical tumor tracking was used. She continues to have no late toxicity and no evidence of disease on the latest scans in November 2023.
Discussion
Although multi-object tracking has been technically described, to our knowledge, this is the first report that describes the clinical workflow, successful implementation, and treatment timeline of an isotoxicity approach with dose-guided tracking of a GI OAR (9, 13). With the MRIdian A3i 510K approved for clinical use, several features now facilitate modern SBRT, including multi-planar tracking (up to three planes simultaneously at 4 FPS), dose-guided and anatomical tracking capabilities, and a parallel adaptive workflow that significantly reduces the on-table treatment times. This novel technology was applied to this patient’s case, in which a high-risk OAR was in close proximity to the target in a patient who had a higher predisposition for GI toxicity due to active IBD. The challenge of delivering an ablative dose in this case was that the intrafraction motion of the GI luminal OAR was not synchronous with the target motion. This approach was deemed successful because the patient did not have any significant GI toxicity (only grade 1 nausea), despite being at higher risk and she had complete resolution of the tumor after treatment.
Radiotherapy in the setting of IBD may be at increased risk of severe toxicity, with estimates upwards of 40%–50% in some series (14). In this cohort, 21% of patients required cessation of treatment due to toxicity, which, in the context of curative intent therapy, may result in suboptimal overall survival. There are other cohorts, including a systematic review, suggesting lower rates of toxicity, similar to the baseline risk following pelvic radiation, and some showed a similar risk of toxicity in the setting of IMRT vs. 3D-CRT techniques (15, 16). There is a paucity of data reporting dose constraints for the SBRT technique in the setting of IBD, and for consistency within our institution, similar OAR constraints were used as compared to other indications for adaptive abdominal radiotherapy.
As previously detailed, real-time tracking was performed for the target on the coronal plane and the duodenum, with dose-guided tracking on the sagittal plane. The institutional target coverage and dose constraints for abdominal targets at ablative doses (50 Gy in five fractions) are presented in Table 1. A prophylactic dose of 30 Gy was selected in line with the SPARTACUS protocol for elective lymph node coverage (17). With the duodenum adjacent to the target in this case and considering its independent intrafraction motion with respiration, an isotoxicity approach was implemented with the 35-Gy isodose line selected for the dose-guided tracking.
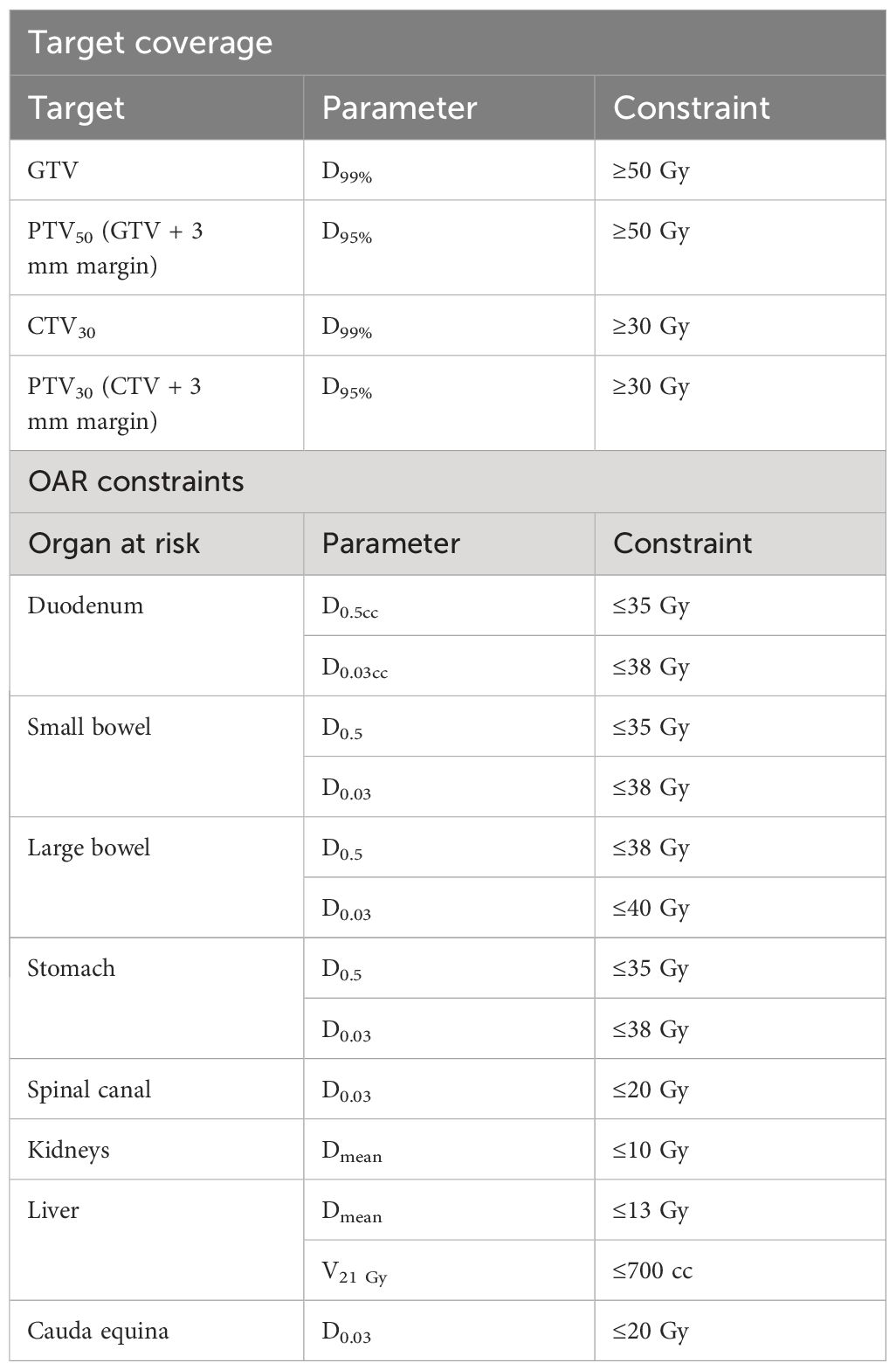
Table 1 Institutional target coverage and dose constraints for abdominal targets to ablative dose schedule (50 Gy in five fractions).
An in-room patient monitor was installed to guide patients regarding the adequacy of their breath hold (Figure 4). The monitor displays the real-time motion of the target volume on the right and the OAR on the left. Note that the user has the option to display all planes, a subset of planes, or none. A smiling emoji is displayed on the monitor as real-time feedback to the patient when the breath hold is adequate, and all gating parameters are satisfied.
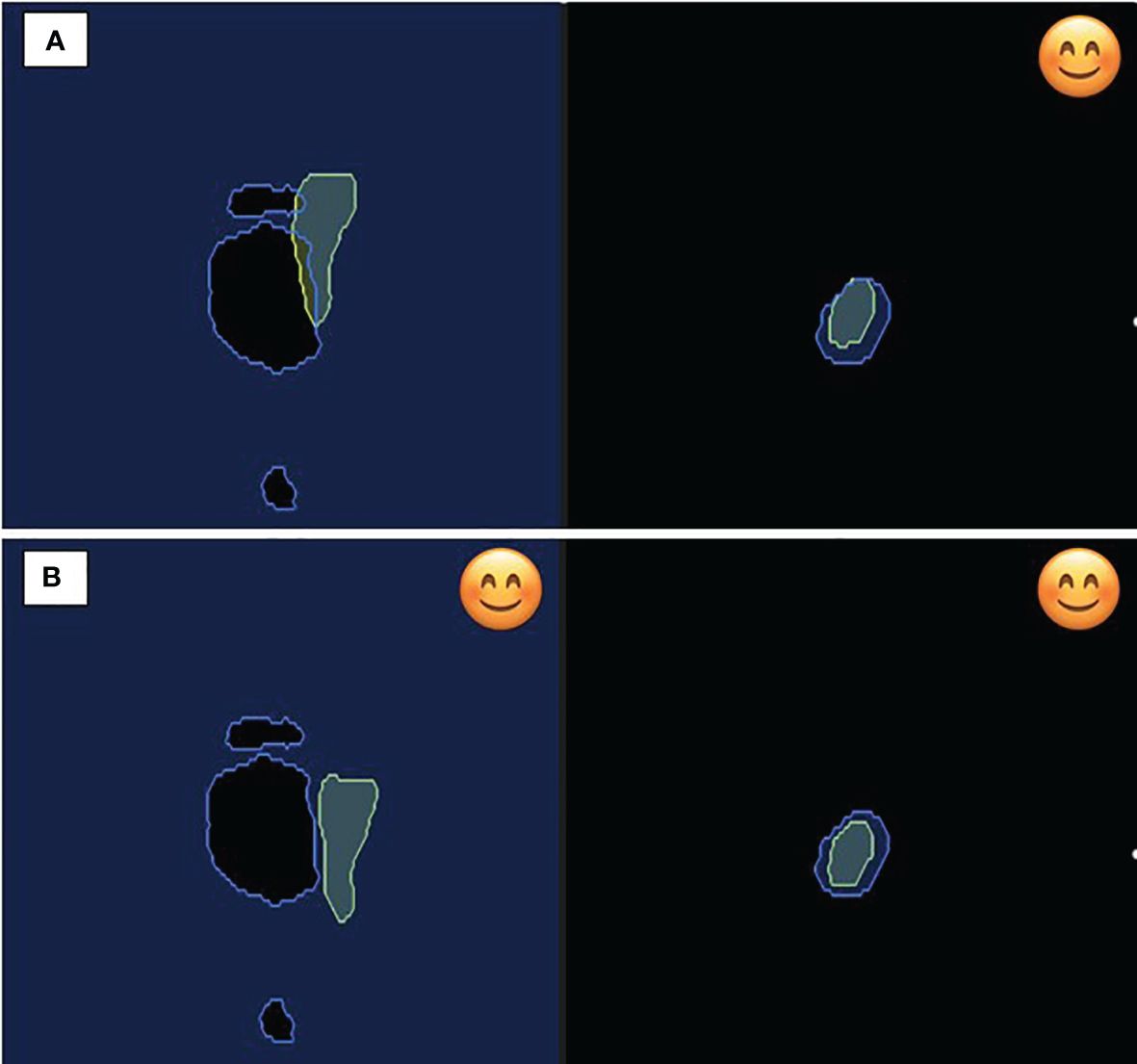
Figure 4 In-room patient monitor demonstrating on the left (A) free breathing with overlap between 35 Gy isodose constraint and duodenum; (B) breath hold demonstrating appropriate geometry for isotoxic delivery. On the right, figure displays the static target volume demonstrating favorable positioning during both respiration and breath hold. The smile emoji is the feedback to the patient during delivery that the patient is in the correct breath hold position for treatment delivery. Note both the organ-at-risk (left) and the target volume (right) have to be in the correct position for the beam to gate on.
In our case, we chose gating settings that required both planes to be satisfied for beam-on delivery. As the target and OAR motion are independent of each other with respiration, at times there can be good target coverage but not a separation with the OAR, and vice versa. This non-synchronous motion is also demonstrated in Figure 4, emphasizing the need for independent, simultaneous tracking of the target and OAR. The treatment beam is automatically gated off at such moments and delivered only when the target is inside and the OAR is outside of the defined boundary.
An increase in the gating events is generally expected, but note that in this case, the target was relatively static, improving the treatment delivery duty cycle. The in-room monitor that provides the visual position of the tumor in real time and feedback to the patient greatly enhanced the adequacy of the breath hold. Moreover, the patients felt actively involved in the treatment process, resulting in improved patient satisfaction, which might have compensated for the treatment time.
AAPM TG 76 recommends the use of appropriate respiratory motion management whenever the motion is >5 mm (18). In clinical practice, image-guided tracking and motion management are restricted to the target, while the OAR is considered secondary; there is no ideal solution to track two ROIs simultaneously in real time. However, SBRT for ablative doses relies on geometric accuracy even when the OAR and target are observed to have independent motion. The technique of multitarget tracking was demonstrated by Liu et al. to simultaneously track targets such as multiple primary lung tumors, prostate, and pelvic lymph nodes (9).
We have adapted this technique to track a target and the adjacent OAR, which have independent respiratory motion to enhance the accuracy of treatment delivery. Conventionally, abdominal and thoracic sites are treated using ITV and PRV, which can lead to inferior target coverage and/or a higher dose to the OAR. While our technique used a GI PRV to define the gradient of the ablative dose of 50 Gy, we performed plan normalization based on the isotoxicity to the nominal GI OAR wall rather than the GI PRV. Without adaptive planning and/or dose-guided tracking, performing isotoxicity to the GI PRV is recommended over the GI OAR wall. Therefore, our approach would rather allow tighter margins for both target and OAR without compromising on target coverage or OAR constraints. The median treatment time of 15 min was equivalent to other MRIdian A3i deliveries of tumor tracking only (19). MRIdian A3i parallel workflow efficiency gains of 30% reduction in online adaptive replanning times have been previously reported (19). The workflow presented here can be adopted for the definitive treatment of any thoracic, abdominal, or pelvic target with dose-limiting OAR in close proximity to ablative doses with an isotoxicity approach.
Beyond this case report, we have implemented dose-guided tracking for all MRgRT patients who have asynchronous motion between the proximal OAR and the tumor. In practice, we have found that the majority of applicable clinical sites are pelvic nodes, in which the target remains static with respiratory motion but the surrounding GI OARs have respiratory-induced motion. Criteria for the section are performed at the time of MRgRT simulation, upon which the geometry of the OAR and target motion is assessed in both the sagittal and coronal cine MR planes. Our future aim is to assess the toxicity profile of patients treated with tumor-based track alone versus dual tumor and isodose-based OAR tracking to quantify the potential reduced toxicity of this method.
Conclusion
Dose-guided tracking of a GI OAR for isotoxicity delivery is feasible on the MRIdian system for SBRT delivery of a target with an adjacent OAR having non-synchronous respiratory motion to ablative doses. This approach takes advantage of the soft tissue visualization of MR and the real-time multi-planar tracking capabilities of an MR Linac and aids in reducing toxicity while maintaining target coverage.
Data availability statement
The datasets presented in this article are not readily available because this is a case report. Requests to access the datasets should be directed to the corresponding author.
Ethics statement
This research was conducted as part of an institutional review board (IRB)-approved retrospective chart review of MR-guided radiotherapy that does not require prospective informed consent. There were no potentially identifiable images or data included in the article.
Author contributions
SY: Conceptualization, Data curation, Formal analysis, Investigation, Methodology, Writing – original draft, Writing – review & editing. YW: Conceptualization, Investigation, Methodology, Supervision, Writing – review & editing. MC: Investigation, Methodology, Supervision, Validation, Writing – review & editing. NB: Conceptualization, Supervision, Validation, Writing – review & editing. AG: Conceptualization, Supervision, Writing – review & editing. RK: Writing – review & editing. MM: Supervision, Writing – review & editing. KM: Conceptualization, Data curation, Formal analysis, Investigation, Methodology, Software, Supervision, Validation, Writing – original draft, Writing – review & editing.
Funding
The author(s) declare that no financial support was received for the research, authorship, and/or publication of this article.
Conflict of interest
NB: grants from ViewRay Inc. MC: Honoraria from ViewRay, Sirtex, IBA, and institutional research funding from ViewRay, Novocure, and StratPharma. AG: Honoraria from ViewRay, Inc., Elekta AB, IBA AB. Ownership interest of Atlantic Health Solutions uPlanÔ. RK: Honoraria from Accuray Inc., Elekta AB, ViewRay Inc., Novocure Inc., Elsevier Inc., Brainlab, Kazia Therapeutics, Castle Biosciences, and institutional research funding from Medtronic Inc., Blue Earth Diagnostics Ltd., Novocure Inc., GT Medical Technologies, AstraZeneca, Exelixis, ViewRay Inc., Brainlab, Cantex Pharmaceuticals, and Kazia Therapeutics. MM: Consulting Fees from Karyopharm, KAzia Therapeutics, Sapience, Zap, Mevion, Xoft; BOD Oncoceutics; Stock in Chimerix. KM: reports ownership interest in a company that provides consulting services on image guided radiation therapy technology MR Guidance, LLC.. She received travel reimbursement/consulting fees/speaking fees/grants from ViewRay Inc.
The remaining authors declare that the research was conducted in the absence of any commercial or financial relationships that could be construed as a potential conflict of interest.
The author(s) declared that they were an editorial board member of Frontiers, at the time of submission. This had no impact on the peer review process and the final decision.
Publisher’s note
All claims expressed in this article are solely those of the authors and do not necessarily represent those of their affiliated organizations, or those of the publisher, the editors and the reviewers. Any product that may be evaluated in this article, or claim that may be made by its manufacturer, is not guaranteed or endorsed by the publisher.
References
1. Chuong MD, Bryant J, Mittauer KE, Hall M, Kotecha R, Alvarez D, et al. Ablative 5-fraction stereotactic magnetic resonance–guided radiation therapy with on-xxx adaptive replanning and elective nodal irradiation for inoperable pancreas cancer. Pract Radiat Oncol. (2021) 11:134–47. doi: 10.1016/j.prro.2020.09.005
2. Spratt DE, Pei X, Yamada J, Kollmeier MA, Cox B, Zelefsky MJ. Long-term survival and toxicity in patients treated with high-dose intensity modulated radiation therapy for localized prostate cancer. Int J Radiat Oncol Biol Phys. (2013) 85:686–92. doi: 10.1016/j.ijrobp.2012.05.023
3. Gerard J-P, Barbet N, Schiappa R, Magné N, Martel I, Mineur L, et al. Neoadjuvant chemoradiotherapy with radiation dose escalation with contact x-ray brachytherapy boost or external beam radiotherapy boost for organ preservation in early cT2–cT3 rectal adenocarcinoma (OPERA): a phase 3, randomised controlled trial. Lancet Gastroenterol Hepatol. (2023) 8:356–67. doi: 10.1016/S2468-1253(22)00392-2
4. Dhont J, Harden SV, Chee LYS, Aitken K, Hanna GG, Bertholet J. Image-guided radiotherapy to manage respiratory motion: lung and liver. Clin Oncol. (2020) 32:792–804. doi: 10.1016/j.clon.2020.09.008
5. Chuong MD, Bryant JM, Herrera R, McCulloch J, Contreras J, Kotecha R, et al. Dose-escalated magnetic resonance image–guided abdominopelvic reirradiation with continuous intrafraction visualization, soft tissue tracking, and automatic beam gating. Adv Radiat Oncol. (2022) 7(2):100840. doi: 10.1016/j.adro.2021.100840
6. Bohoudi O, Bruynzeel AME, Senan S, Cuijpers JP, Slotman BJ, Lagerwaard FJ, et al. Fast and robust online adaptive planning in stereotactic MR-guided adaptive radiation therapy (SMART) for pancreatic cancer. Radiother Oncol J Eur Soc Ther Radiol Oncol. (2017) 125:439–44. doi: 10.1016/j.radonc.2017.07.028
7. Mittauer K, Paliwal B, Hill P, Bayouth JE, Geurts MW, Baschnagel AM, et al. A new era of image guidance with magnetic resonance-guided radiation therapy for abdominal and thoracic Malignancies. Cureus. (2018) 10:4. doi: 10.7759/cureus.2422
8. Mittauer KE, Yarlagadda S, Bryant JM, Bassiri N, Romaguera T, Gomez AG, et al. Online adaptive radiotherapy: Assessment of planning technique and its impact on longitudinal plan quality robustness in pancreatic cancer. Radiother Oncol J Eur Soc Ther Radiol Oncol. (2023) 188:109869. doi: 10.1016/j.radonc.2023.109869
9. Liu PZY, Dong B, Nguyen DT, Ge Y, Hewson EA, Waddington DEJ, et al. First experimental investigation of simultaneously tracking two independently moving targets on an MRI-linac using real-time MRI and MLC tracking. Med Phys. (2020) 47:6440–9. doi: 10.1002/mp.14536
10. Mittauer KE, Yadav P, Paliwal B, Bayouth JE. Characterization of positional accuracy of a double-focused and double-stack multileaf collimator on an MR-guided radiotherapy (MRgRT) Linac using an IC-profiler array. Med Phys. (2020) 47:317–30. doi: 10.1002/mp.13902
11. Ginn JS, Agazaryan N, Cao M, Baharom U, Low DA, Yang Y, et al. Characterization of spatial distortion in a 0.35 T MRI-guided radiotherapy system. Phys Med Biol. (2017) 62:4525. doi: 10.1088/1361-6560/aa6e1a
12. Small W, Mell LK, Anderson P, Creutzberg C, De Los Santos J, Gaffney D, et al. Consensus guidelines for delineation of clinical target volume for intensity-modulated pelvic radiotherapy in postoperative treatment of endometrial and cervical cancer. Int J Radiat Oncol Biol Phys. (2008) 71:428–34. doi: 10.1016/j.ijrobp.2007.09.042
13. Dhont J, Vandemeulebroucke J, Cusumano D, Boldrini L, Cellini F, Valentini V, et al. Multi-object tracking in MRI-guided radiotherapy using the tracking-learning-detection framework. Radiother Oncol. (2019) 138:25–9. doi: 10.1016/j.radonc.2019.05.008
14. Willett CG, Ooi CJ, Zietman AL, Menon V, Goldberg S, Sands BE, et al. Acute and late toxicity of patients with inflammatory bowel disease undergoing irradiation for abdominal and pelvic neoplasms. Int J Radiat Oncol Biol Phys. (2000) 46:995–8. doi: 10.1016/S0360-3016(99)00374-0
15. Trotta M, Patel KR, Singh S, Verma V, Ryckman J. Safety of radiation therapy in patients with prostate cancer and inflammatory bowel disease: A systematic review. Pract Radiat Oncol. (2023) 13:454–65. doi: 10.1016/j.prro.2023.04.006
16. White EC, Murphy JD, Chang DT, Koong AC. Low toxicity in inflammatory bowel disease patients treated with abdominal and pelvic radiation therapy. Am J Clin Oncol. (2015) 38:564–9. doi: 10.1097/COC.0000000000000010
17. Leung E, Gladwish AP, Davidson M, Taggar A, Velker V, Barnes E, et al. Quality-of-life outcomes and toxic effects among patients with cancers of the uterus treated with stereotactic pelvic adjuvant radiation therapy: the SPARTACUS phase 1/2 nonrandomized controlled trial. JAMA Oncol. (2022) 8:1–9. doi: 10.1001/jamaoncol.2022.0362
18. Keall PJ, Mageras GS, Balter JM, Emery RS, Forster KM, Jiang SB, et al. The management of respiratory motion in radiation oncology report of AAPM Task Group 76. Med Phys. (2006) 33:3874–900. doi: 10.1118/1.2349696
Keywords: MRgRT, dose-guided tracking, SBRT, ablative dose, GI OAR
Citation: Yarlagadda S, Weiss Y, Chuong MD, Bassiri N, Gutierrez AN, Kotecha R, Mehta MP and Mittauer KE (2024) Case report: Intrafraction dose-guided tracking for gastrointestinal organ-at-risk isotoxicity delivery on an MR-guided radiotherapy system. Front. Oncol. 14:1357916. doi: 10.3389/fonc.2024.1357916
Received: 18 December 2023; Accepted: 20 June 2024;
Published: 11 July 2024.
Edited by:
Enis Ozyar, Acıbadem University, TürkiyeReviewed by:
Nadia Saeed, Brigham/Dana Farber Cancer Institute, United StatesPhilipp Hoegen, Heidelberg University Hospital, Germany
Copyright © 2024 Yarlagadda, Weiss, Chuong, Bassiri, Gutierrez, Kotecha, Mehta and Mittauer. This is an open-access article distributed under the terms of the Creative Commons Attribution License (CC BY). The use, distribution or reproduction in other forums is permitted, provided the original author(s) and the copyright owner(s) are credited and that the original publication in this journal is cited, in accordance with accepted academic practice. No use, distribution or reproduction is permitted which does not comply with these terms.
*Correspondence: Kathryn Elizabeth Mittauer, a2F0aHJ5bm1AYmFwdGlzdGhlYWx0aC5uZXQ=