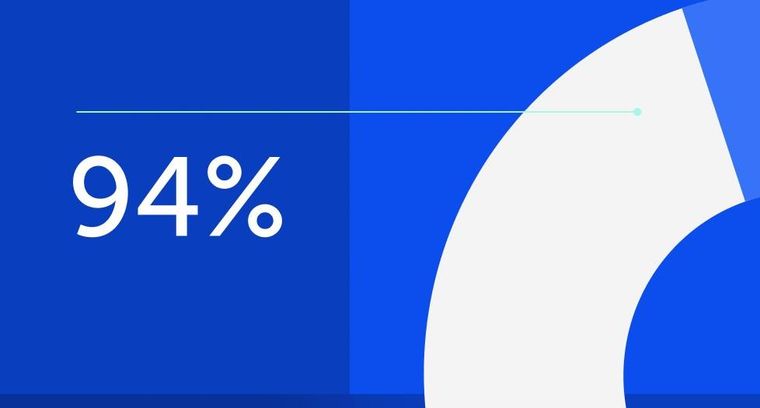
94% of researchers rate our articles as excellent or good
Learn more about the work of our research integrity team to safeguard the quality of each article we publish.
Find out more
ORIGINAL RESEARCH article
Front. Oncol., 22 April 2024
Sec. Thoracic Oncology
Volume 14 - 2024 | https://doi.org/10.3389/fonc.2024.1357583
This article is part of the Research TopicKRAS in Stage IV Non-Small Cell Lung CancerView all 10 articles
A correction has been applied to this article in:
Corrigendum: TP53 co-mutations in advanced lung adenocarcinoma: comparative bioinformatic analyses suggest ambivalent character on overall survival alongside KRAS, STK11 and KEAP1 mutations
Background: Recently, we could show that the co-mutations of KRAS + KEAP1, STK11 + KEAP1 and KRAS + STK11 + KEAP1 lead to a significantly shorter median overall survival (mOS) in patients with lung cancer across treatments by analyzing multiple dataset. TP53, a tumor suppressor gene, plays a crucial role in regulating cell cycle progression. Its mutations occur in approximately 40-50% of non-small lung cancer (NSCLC). Co-occurrence of all four mentioned mutations has been a matter of debate for years. The aim of this study was to assess the distribution of these four mutations and the influence of the different co-mutational patterns on survival.
Methods: We present a comparative bioinformatic analysis and refer to data of 4,109 patients with lung adenocarcinoma (LUAD).
Results: Most of the mutations within the LUAD belong to TP53-only (29.0%), quadruple-negative (25.9%) and KRAS-only (13.4%). Whereas TP53-mutations seem to have protective effects in the context of further KEAP1- and KRAS + KEAP1-alterations (improved mOS), their role seems contrary if acquired in an already existing combination of mutations as KRAS + STK11, KRAS + STK11 + KEAP1 and STK11 + KEAP1. TP53 co-mutations had a negative influence on KRAS-only mutated LUAD (mOS reduced significantly by more than 30%).
Discussion: These data underline the need for complex mutational testing to estimate prognosis more accurately in patients with advanced LUAD.
Lung Cancer is the leading cause of cancer death worldwide, with non-small lung cancer (NSCLC) representing the largest group. Lung adenocarcinoma (LUAD) belongs to the most common and best studied histological subgroups (1). Besides the common treatment strategies consisting of surgery, radiation and chemotherapy, the development and approval of targeted therapies and immune checkpoint inhibitors (ICI) explicitly improved therapy options and patients’ outcome within the past decade. However, treatment responses still vary in a wide range even for the personalized treatment options available (2). Therefore, amongst others, one major need is to acknowledge the significance of genetic co-alterations and their influence on therapy responses.
KRAS (Kirsten rat sarcoma viral oncogene homolog) plays a key role in the development and progression of various cancers, including NSCLC. Mutations occur in about 25% of cases, leading to the constitutive activation of KRAS signaling pathways, promoting uncontrolled cell growth and survival (3). KRAS-altered NSCLC frequently show co-mutations within the genes Kelch-like ECH-associated protein 1 (KEAP1) and serine/threonine kinase 11 (STK11), also known as liver kinase B1 (LKB1) (4). Recently, we could show, by analyzing multiple datasets, that the co-mutations of KRAS + KEAP1, STK11 + KEAP1 and KRAS + STK11 + KEAP1 lead to a significantly shorter median overall survival (mOS) across treatments. In contrast, patients with tumors harboring only KRAS mutations or being negative for all above-mentioned alterations show a significantly improved mOS in a multivariate analysis. Furthermore, triple co-mutated primary tumors showed a significantly increased frequency of distant metastases to bone and adrenal glands (5). Thus, analysis of the complex mutational network seems inevitable in the clinical routine setting.
TP53 (tumor protein p53), a tumor suppressor gene, plays a crucial role in regulating cell cycle progression, DNA repair, as well as apoptosis and is one of the most common alterations among all cancers, and among LUAD in particular (6). Its mutations occur in approximately 40-50% of NSCLC cases, leading mainly to a loss of function, allowing cells to evade normal regulatory mechanisms and promoting tumorigenesis (7).
Co-occurrence of all four mentioned mutations has been a matter of debate for years: Aredo et al. (8) described concurrent pathogenic mutations of KRAS with TP53 (39%), STK11 (12%) and KEAP1 (8%), discussing distinct molecular subtypes (study with a total of 186 patients). Furthermore, they could show that combined KRAS G12C and TP53 mutations predict benefit from immunotherapy.
Frost et al. focused on 119 patients with lung adenocarcinoma receiving pembrolizumab monotherapy as first-line palliative treatment. Here, rates for KRAS, TP53 and combined mutations were 52.1%, 47.1% and 21.9%, respectively. Whereas, TP53 mutations alone had no impact on response and survival, a subgroup (12 patients) with KRAS G12C + TP53 co-mutations defined long-term responders to immunotherapy (9).
Recently, Proulx-Rocray et al. described 100 patients with known KRAS status. They postulated that KRAS mutation in NSCLC might be associated with a favorable response to ICI therapy in the absence of a concurrent mutation in the STK11 and/or KEAP1 tumor suppressor genes (10). A survival advantage associated with TP53 mutation in NSCLC treated with ICIs has been reported in current literature (9, 11–13).
However, the above-mentioned studies only encompass a low number of patients. Furthermore, besides ICI, “classical” chemotherapy still presents the main cornerstone of therapy. Therefore, we here present a comprehensive bioinformatic analysis encompassing two datasets retrieved from cBioPortal and tested the influence of TP53 co-mutations depending on the KRAS, STK11 and KEAP1 status.
The aim of this study was to assess the distribution of the four mutations KRAS, STK11, KEAP1, and TP53 as single mutations as well as co-mutations in patients with advanced LUAD in a large dataset. Furthermore, we want to study the influence of the different co-mutational combinations on survival.
For this study, the following two datasets from the MSK institute were retrieved from cBioPortal (14, 15): the “MSK-IMPACT Clinical Sequencing Cohort (MSKCC, Nat Med 2017)” (16) and the “MSK MetTropism (MSK, Cell 2021)” dataset (17). To create one dataset across treatments the two datasets were merged into one “MSK across treatments” dataset (N = 4,855 NSCLC patients, 4,109 LUAD patients, 542 lung squamous cell carcinoma (LSCC) patients). Therapy details were not annotated. Due to the more recent data, data from the “MSK MetTropism (MSK, Cell 2021)” were preferred over the “MSK-IMPACT Clinical Sequencing Cohort (MSKCC, Nat Med 2017)”. All data comprised patients with advanced tumor stages (mainly stage IV). Analyses were performed on LUAD data.
In total, 16 combinatory groups of patients were established based on the four genes KRAS, STK11, KEAP1, and TP53 (Table 1, Figures 1, 2): quadruple negative, KRAS-only, STK11-only, KEAP1-only, TP53-only, KRAS + TP53, STK11 + TP53, KEAP1 + TP53, KRAS + STK11, KRAS + STK11 + TP53, KRAS + KEAP1, KRAS + KEAP1 + TP53, STK11 + KEAP1, STK11 + KEAP1 + TP53, KRAS + STK11 + KEAP1, KRAS + STK11 + KEAP1 + TP53.
Table 1. Distribution of co-mutations in the genes of KRAS, STK11, KEAP1, and TP53, and overall survival in patients with LUAD.
Statistical analyses were performed in Python (v.3.9.). All p-values were corrected for multiple testing using false discovery rates (q-value) and q-values < 0.05 were defined as significant. The Kaplan-Meier method was performed to calculate OS curves and medians. Pairwise differences were calculated by log-rank tests.
The total dataset consisted of 4,855 NSCLC patients (male: 41.7%; female: 58.3%). Thereby, 84.6% (N = 4,109) were lung adenocarcinoma, 11.2% (N = 542) lung squamous cell carcinoma, and 4.2% (N = 204) other histologic types of lung cancer: e.g. adenosquamous carcinoma, sarcomatoid lung cancer, lung neuroendocrine tumors (large cell neuroendocrine carcinoma, carcinoids), or not otherwise specified NSCLC. For the following analyses we concentrated on lung adenocarcinoma data. Thereby, we found the following mutation frequencies: 45.6% TP53, 34.17% KRAS, 16.82% STK11, 14.35% KEAP1. Further, KRAS mutations showed the subsequent distribution of point mutations: G12C 40.17%, G12V 17.32%, G12D 13.76%, G12A 7.59%, Q61H 4.26%, G13C 3.62%.
TP53-only mutation (29%; N = 1193), the absence of the four mutations (quadruple-negative: 25.9%; N = 1,062) and KRAS-only (13.4%; N = 552) were the most prevalent (co-)mutational patterns. The least prevalent (co-)mutational patterns were KEAP1-only (0.6%; N = 25) and the quadruple mutation (KRAS + STK11 + KEAP1 + TP53; 0.8%; N = 33). Full data are shown in Table 1 and Figure 1.
While mutations in KRAS, STK11 and KEAP1 significantly co-occurred among themselves (q < 0.05), there was neither a significant co-occurrence nor a significant mutual exclusivity between mutations in one of the three genes and TP53 mutations.
Kaplan-Meier curves were calculated for all 16 mutation groups and are shown in Figure 2. Quadruple negative (mOS = 64 months), KRAS-only (56.5 months) and KRAS + STK11 (mOS = 53 months) mutated patients had the longest mOS, while patients mutated in KRAS + STK11 + KEAP1 + TP53 (mOS = 8.6 months), KRAS + STK11 + KEAP1 (mOS = 12.4 months) and STK11 + KEAP1 + TP53 (mOS = 14.8 months) showed the shortest mOS (Figure 2, Table 1). To determine the influence of TP53 co-mutations on KRAS-, STK11- and/or KEAP1-mutated LUAD, pairwise tests were performed (Figure 3).
Figure 3. Kaplan Meier curves showing pairwise comparison of mutation groups with (blue) and without (yellow) additional TP53 mutation. Log-rank tests were performed (p-values) and p-values corrected for multiple testing using false discovery rates (q-values). Panel A compares KRAS-only with KRAS+TP53, panel B TP53-only with KRAS-TP53, panel C KEAP1-only with KEAP1+TP53, panel D STK11-only with STK11+TP53, panel E KRAS+KEAP1 with KRAS+KEAP1+TP53, panel F KRAS+STK11 with KRAS+STK11+TP53, panel G STK11+KEAP1 with STK11+KEAP1+TP53, and panel H KRAS+STK11+KEAP1 with KRAS+STK11+KEAP1+TP53.
Co-mutations in TP53 led to significantly reduced mOS in LUAD patients harboring only a KRAS mutation (mOS: 56.5 vs. 38.3 months; p = 0.0026; q = 0.021; Figure 3A) or a co-mutation in KRAS + STK11 (mOS: 53.0 vs. 23.0 months; p = 0.032; q = 0.085; Figure 3E), albeit, significance for the latter does not remain after correcting for multiple testing. The well-known negative impact of the KRAS + STK11 + KEAP1 (mOS: 12.4 months) and STK11 + KEAP1 mutation co-occurrence (mOS: 25.1 months) worsened mOS by trend through an add-on TP53 mutation (mOS: 8.6 or 14.8 months, respectively; Figures 3G, H); however, not statistically significant.
In contrast, concurrent TP53 mutations to KEAP1-only and to KRAS + KEAP1 mutations showed an opposite effect and led to an improved mOS: 21.1 months for KEAP1-only improved to 52.2 months for KEAP1 + TP53 (p = 0.03; q = 0.085; Figure 3C) and 16.1 months for KRAS + KEAP1 improved to a mOS which did not reach the median for KRAS + KEAP1 + TP53 (p = 0.053; q = 0.1; Figure 3F).
When considering only KRAS mutations harboring the G12C alteration, the add-on TP53 mutation still led to reduced mOS (85.7 vs. 36.5 months; p = 0.02; q = 0.08), albeit without significance after correcting for multiple testing, while for KRAS (G12C) + KEAP1, the TP53 co-mutation still did not significantly change mOS (20 months vs. NR; p-value = 0.25; q = 0.5) (Table 2). The occurrence of TP53 co-mutation in KRAS (G12C) + STK11 altered LUAD did not lead to a reduced mOS anymore (49 vs. 54 months; p = 0.87; q = 0.87). This is also true for KRAS G12C + STK11 + KEAP1 (18.7 vs. 8.6 months; p = 0.48; q = 0.64). These results must be interpreted with caution due to partly small group sizes (N < 20; Table 2).
Table 2. Log-rank test comparing overall survival of KRAS G12C mutation groups with and without TP53 co-mutation.
Here, we presented a comparative bioinformatic analysis referring to data of 4,109 patients with the aim of analyzing the influence of TP53 mutations in KRAS, STK11 and KEAP1 (co-) mutated LUAD on the patients’ overall survival. By employing this database approach, we were able to show that TP53 mutations had an influence on mOS for better or worse depending on the concurrent mutational pattern.
Therapy and prognosis of NSCLC has changed in the last 15 years as several treatable targets have been detected within the concept of so-called personalized therapies. In daily practice, these targets encompass testing for rearrangements (ALK, ROS, RET, NTRK, MET) and mutations (KRAS, EGFR, BRAF, ERBB2) (18–20). For a long time KRAS-mutations were called “untreatable targets”. Since early 2022 a specific (second-line) therapy for KRAS G12C has been available in Europe. However, the majority of NSCLC do not harbor the above-mentioned mutations and thus do not qualify for these treatment options. Thus, therapy is still based on different chemotherapy protocols with or without ICIs.
In our study, the mutation frequencies of the four genes (TP53: 45.6%, KRAS: 34.17%, STK11: 16.82%, KEAP1: 14.35%) correspond to the generally described values in LUAD (6). Further, as already shown (5), KRAS-only and KRAS + STK11 mutated patients have the longest mOS (56.5 and 53 months). This seems also true for the quadruple negative group as presented here (64 months). Nevertheless, it must be noticed that the overall survival of quadruple negative patients might be biased due to further common mutations or genetic rearrangements in genes like EGFR, ALK or ROS1 and their already approved targeted therapies. However, the comparable long survival times of these groups, especially for KRAS-only, further underlines the fact that the time has passed to describe KRAS as a prognostically unfavorable factor. Moreover, it seems more appropriate to analyze the complex surrounding mutational landscape, as patients mutated in KRAS + STK11 + KEAP1 + TP53, KRAS + STK11 + KEAP1 and STK11 + KEAP1 + TP53 show the shortest overall survival. So far, several studies described STK11 and KEAP1 alone or co-mutated with KRAS having a negative impact on OS, response to ICI-therapy (4, 5, 21–26) and also across treatment classes independent of immunotherapy (4, 5, 24, 27). However, especially KEAP1 mutations seemed to be the driving factor being the only one significant in a multivariate model (4). This is also reflected in the current analyses of the CodeBreak 100 clinical trial. Here, KEAP1 mutations also appear to be a negative prognostic marker for sotorasib (28).
Therefore, it is particularly interesting that this role seems only true if TP53-mutations are absent, as patients with the combination of KRAS + KEAP1 + TP53 or KEAP1 + TP53 co-mutations show an improved mOS in comparison to KEAP1-only and KRAS + KEAP1 mutated patients. Thus, somewhat surprisingly, in this setting TP53 mutations seem to have a protective effect as long as STK11 is not co-mutated. So far, survival advantage of TP53 mutations could be shown under therapy with ICI (9, 11–13), however not across treatments. Regardless, it must be noticed that these studies did not include KEAP1 co-mutations into their analyses.
Vice versa, TP53 will have a negative influence on KRAS-only mutated LUAD. Here, the patients’ mOS was reduced significantly more than 30% (from 56.5 to 38.3 months, see Table 1, Figure 3A). The negative influence of TP53 mutations were also visible for the following co-mutations: KRAS + STK11, KRAS + STK11 + KEAP1 and STK11 + KEAP1 (Figure 3). Therefore, the potential positive or negative impact of the altered tumor suppressor p53 seems to depend on the surrounding mutational network. This effect had already been described by Saleh et al. and Scalera et al., pointing out that molecular stratification of both alterations should be implemented for localized and advanced-stage NSCLC to optimize and modify clinical decision-making (29, 30), even though both studies did not include KRAS and/or STK11 in their investigations.
For KRAS G12C, sotorasib, a targeted therapy, is approved and has shown that its efficacy is influenced by the co-mutations of STK11 and KEAP1. While the co-mutation with STK11 leads to a slightly improved efficacy, KEAP1 and the co-mutation with both genes result in a reduced response (31). Therefore, we performed our analyses in the context of KRAS G12C. Comparable results were observed with a reduced overall survival when KRAS G12C (mOS = 85.7 month) is co-mutated with TP53 (mOS = 36.5; p = 0.02; q = 0.08). The benefits of TP53 co-mutations shown under ICI were subsequently not apparent across treatments (8, 9). The reported tendency was not shown in the context of KRAS G12C + STK11 + TP53. Here again, a somewhat protective effect might be discussed for TP53 co-mutations in KRAS G12C + KEAP1 mutated patients, albeit group sizes are small and results not significant. This underlines the importance of the now available G12C-targeted therapy and the need for more druggable options in KRAS-mutated LUAD and should be kept in mind when interpreting the results of the recently published phase III CodeBreaK 200 trial (32) and the still ongoing phase III CodeBreaK 202 trial (NCT05920356) evaluating sotorasib for the second-line or first-line treatment, respectively.
Finally, we here present a bioinformatic analysis of merged data sets. This might be a limitation at first sight, as we did not refer to our own data. However, the sample sizes of the molecular subgroups, as summarized here, are too small within the single studies to obtain group sizes sufficient for robust statistical results. Thus, integrating database approaches, as presented here, are needed to draw first preliminary conclusions and to generate new hypotheses. These hypotheses must be then tested in future multi-center investigations. This study is further limited by the given annotations. Analyzing the mutation groups in correlation with further clinical variables like age, sex, smoking history, and in particular different treatment patterns is an important task for future studies. Another point of interest is the assessment of progression-free survival in addition to the overall survival. Nevertheless, our study gives important insights into the mutual influence of co-mutations and provides a starting point for future research approaches.
To conclude, the more mutations are analyzed to a greater extent, the greater will be the complexity of the mutational network of lung cancer and cancer in general. In the daily clinical routine setting, referring to panel-based sequencing (as e.g. suggested by the national network of genomic medicine/nNGM) seems mandatory and focusing on different combinations of mutations can help define different prognostic groups and might be the starting point for new treatment strategies.
The original contributions presented in the study are included in the article/supplementary material. Further inquiries can be directed to the corresponding authors.
Ethical approval was not required for the study involving humans in accordance with the local legislation and institutional requirements. Written informed consent to participate in this study was not required from the participants or the participants’ legal guardians/next of kin in accordance with the national legislation and the institutional requirements.
AF: Conceptualization, Data curation, Formal Analysis, Funding acquisition, Investigation, Methodology, Project administration, Resources, Software, Supervision, Validation, Visualization, Writing – original draft, Writing – review & editing. MB: Conceptualization, Data curation, Formal Analysis, Funding acquisition, Investigation, Methodology, Project administration, Resources, Software, Supervision, Validation, Visualization, Writing – original draft, Writing – review & editing. HW: Writing – review & editing. MS: Methodology, Writing – review & editing. HB: Funding acquisition, Supervision, Writing – review & editing. ML: Conceptualization, Data curation, Formal Analysis, Funding acquisition, Investigation, Methodology, Project administration, Resources, Software, Supervision, Validation, Visualization, Writing – original draft, Writing – review & editing.
The authors declare that financial support was received for the publication of this article, which was funded by the Open Access Publishing Fund of Leipzig University, supported by the German Research Foundation (DFG). The funder was not involved in the study design, collection, analysis, interpretation of data, the writing of this article or the decision to submit it for publication.
AF was supported by the postdoctoral fellowship “MetaRot program” from the Federal Ministry of Education and Research (BMBF), Germany (FKZ 01EO1501, IFB Adiposity Diseases), a research grant from the “Mitteldeutsche Gesellschaft für Pneumologie (MDGP) e.V.” (2018-MDGP-PA-002), a junior research grant from the Medical Faculty, University of Leipzig (934100-012), a graduate fellowship of the “Novartis Foundation”.
The authors declare that the research was conducted in the absence of any commercial or financial relationships that could be construed as a potential conflict of interest.
This publication was funded by the Open Access Publishing Fund of Leipzig University, supported by the German Research Foundation (DFG). The funder was not involved in the study design, collection, analysis, interpretation of data, the writing of this article or the decision to submit it for publication.
All claims expressed in this article are solely those of the authors and do not necessarily represent those of their affiliated organizations, or those of the publisher, the editors and the reviewers. Any product that may be evaluated in this article, or claim that may be made by its manufacturer, is not guaranteed or endorsed by the publisher.
1. Hutchinson BD, Shroff GS, Truong MT, Ko JP. Spectrum of lung adenocarcinoma. Semin ultrasound CT MR. (2019) 40:255–64. doi: 10.1053/j.sult.2018.11.009
2. Kumar M, Sarkar A. CURRENT THERAPEUTIC STRATEGIES AND CHALLENGES IN NSCLC TREATMENT: A COMPREHENSIVE REVIEW. Exp Oncol. (2022) 44:7–16. doi: 10.32471/exp-oncology.2312-8852.vol-44-no-1.17411
3. Riely GJ, Marks J, Pao W. KRAS mutations in non–small cell lung cancer. Proc Am Thorac Soc. (2009) 6:201–5. doi: 10.1513/pats.200809-107LC
4. Arbour KC, Jordan E, Kim HR, Dienstag J, Yu HA, Sanchez-Vega F, et al. Effects of co-occurring genomic alterations on outcomes in patients with KRAS-mutant non-small cell lung cancer. Clin Cancer Res. (2018) 24:334–40. doi: 10.1158/1078-0432.CCR-17-1841
5. Boeschen M, Kuhn CK, Wirtz H, Seyfarth HJ, Frille A, Lordick F, et al. Comparative bioinformatic analysis of KRAS, STK11 and KEAP1 (co-)mutations in non-small cell lung cancer with a special focus on KRAS G12C. Lung Cancer. (2023) 184:107361. doi: 10.1016/j.lungcan.2023.107361
6. Cancer Genome Atlas Research Network. Comprehensive molecular profiling of lung adenocarcinoma. Nature. (2014) 511:543–50. doi: 10.1038/nature13385
7. Mogi A, Kuwano H. TP53 mutations in nonsmall cell lung cancer. J biomed Biotechnol. (2011) 2011:583929. doi: 10.1155/2011/583929
8. Aredo JV, Padda SK, Kunder CA, Han SS, Neal JW, Shrager JB, et al. Impact of KRAS mutation subtype and concurrent pathogenic mutations on non-small cell lung cancer outcomes. Lung Cancer. (2019) 133:144–50. doi: 10.1016/j.lungcan.2019.05.015
9. Frost N, Kollmeier J, Vollbrecht C, Grah C, Matthes B, Pultermann D, et al. KRASG12C/TP53 co-mutations identify long-term responders to first line palliative treatment with pembrolizumab monotherapy in PD-L1 high (≥50%) lung adenocarcinoma. Trans Lung Cancer Res. (2021) 10:737–52. doi: 10.21037/tlcr-20-958
10. Proulx-Rocray F, Routy B, Nassabein R, Belkaid W, Tran-Thanh D, Malo J, et al. The prognostic impact of KRAS, TP53, STK11 and KEAP1 mutations and their influence on the NLR in NSCLC patients treated with immunotherapy. Cancer Treat Res Commun. (2023) 37:100767. doi: 10.1016/j.ctarc.2023.100767
11. Skoulidis F, Byers LA, Diao L, Papadimitrakopoulou VA, Tong P, Izzo J, et al. Co-occurring genomic alterations define major subsets of KRAS-mutant lung adenocarcinoma with distinct biology, immune profiles, and therapeutic vulnerabilities. Cancer Discovery. (2015) 5:860–77. doi: 10.1158/2159-8290.CD-14-1236
12. Dong Z-Y, Zhong WZ, Zhang XC, Su J, Xie Z, Liu SY, et al. Potential predictive value of TP53 and KRAS mutation status for response to PD-1 blockade immunotherapy in lung adenocarcinoma. Clin Cancer Res. (2017) 23:3012–24. doi: 10.1158/1078-0432.CCR-16-2554
13. Assoun S, Theou-Anton N, Nguenang M, Cazes A, Danel C, Abbar B, et al. Association of TP53 mutations with response and longer survival under immune checkpoint inhibitors in advanced non-small-cell lung cancer. Lung Cancer. (2019) 132:65–71. doi: 10.1016/j.lungcan.2019.04.005
14. Cerami E, Gao J, Dogrusoz U, Gross BE, Sumer SO, Aksoy BA, et al. The cBio cancer genomics portal: an open platform for exploring multidimensional cancer genomics data. Cancer Discovery. (2012) 2:401–4. doi: 10.1158/2159-8290.CD-12-0095
15. Gao J, Aksoy BA, Dogrusoz U, Dresdner G, Gross B, Sumer SO, et al. Integrative analysis of complex cancer genomics and clinical profiles using the cBioPortal. Sci Signaling. (2013) 6:l1. doi: 10.1126/scisignal.2004088
16. Zehir A, Benayed R, Shah RH, Syed A, Middha S, Kim HR, et al. Erratum: Mutational landscape of metastatic cancer revealed from prospective clinical sequencing of 10,000 patients. Nat Med. (2017) 23:1004. doi: 10.1038/nm0817-1004c
17. Nguyen B, Fong C, Luthra A, Smith SA, DiNatale RG, Nandakumar S, et al. Genomic characterization of metastatic patterns from prospective clinical sequencing of 25,000 patients. Cell. (2022) 185:563–575.e11. doi: 10.1016/j.cell.2022.01.003
18. Dietel M, Jöhrens K, Laffert MV, Hummel M, Bläker H, Pfitzner BM, et al. A 2015 update on predictive molecular pathology and its role in targeted cancer therapy: a review focussing on clinical relevance. Cancer Gene Ther. (2015) 22:417–30. doi: 10.1038/cgt.2015.39
19. Dietel M, Bubendorf L, Dingemans AM, Dooms C, Elmberger G, García RC, et al. Diagnostic procedures for non-small-cell lung cancer (NSCLC): recommendations of the European Expert Group. Thorax. (2016) 71:177–84. doi: 10.1136/thoraxjnl-2014-206677
20. Garassino MC, Oskar S, Arunachalam A, Zu K, Kao YH, Chen C, et al. Real-world treatment patterns and outcomes of first-line immunotherapy among patients with advanced nonsquamous NSCLC harboring BRAF, MET, or HER2 alterations. JTO Clin Res Rep. (2023) 4:100568. doi: 10.1016/j.jtocrr.2023.100568
21. Biton J, Mansuet-Lupo A, Pécuchet N, Alifano M, Ouakrim H, Arrondeau J, et al. TP53, STK11, and EGFR mutations predict tumor immune profile and the response to anti-PD-1 in lung adenocarcinoma. Clin Cancer Res. (2018) 24:5710–23. doi: 10.1158/1078-0432.CCR-18-0163
22. Skoulidis F, Goldberg ME, Greenawalt DM, Hellmann MD, Awad MM, Gainor JF, et al. STK11/LKB1 mutations and PD-1 inhibitor resistance in KRAS-mutant lung adenocarcinoma. Cancer Discovery. (2018) 8:822–35. doi: 10.1158/2159-8290.CD-18-0099
23. Marinelli D, Mazzotta M, Scalera S, Terrenato I, Sperati F, D'Ambrosio L, et al. KEAP1-driven co-mutations in lung adenocarcinoma unresponsive to immunotherapy despite high tumor mutational burden. Ann Oncol. (2020) 31:1746–54. doi: 10.1016/j.annonc.2020.08.2105
24. Papillon-Cavanagh S, Doshi P, Dobrin R, Szustakowski J, Walsh AM. STK11 and KEAP1 mutations as prognostic biomarkers in an observational real-world lung adenocarcinoma cohort. ESMO Open. (2020) 5:e000706. doi: 10.1136/esmoopen-2020-000706
25. Pavan A, Bragadin AB, Calvetti L, Ferro A, Zulato E, Attili et I, et al. Role of next generation sequencing-based liquid biopsy in advanced non-small cell lung cancer patients treated with immune checkpoint inhibitors: impact of STK11, KRAS and TP53 mutations and co-mutations on outcome. Trans Lung Cancer Res. (2021) 10:202–20. doi: 10.21037/tlcr-20-674
26. Cordeiro de Lima VC, Corassa M, Saldanha E, Freitas H, Arrieta O, Raez L, et al. STK11 and KEAP1 mutations in non-small cell lung cancer patients: Descriptive analysis and prognostic value among Hispanics (STRIKE registry-CLICaP). Lung Cancer. (2022) 170:114–21. doi: 10.1016/j.lungcan.2022.06.010
27. Cho BC, Lopes G, Kowalski DM, Kasahara K, Wu YL, Castro G, et al. Abstract CT084: Relationship between STK11 and KEAP1 mutational status and efficacy in KEYNOTE-042: pembrolizumab monotherapy versus platinum-based chemotherapy as first-line therapy for PD-L1-positive advanced NSCLC. Cancer Res. (2020) 80:CT084–4. doi: 10.1158/1538-7445.AM2020-CT084
28. Dy GK, Govindan R, Velcheti V, Falchook GS, Italiano A, Wolf J, et al. Long-term outcomes and molecular correlates of sotorasib efficacy in patients with pretreated KRAS G12C-mutated non-small-cell lung cancer: 2-year analysis of codeBreaK 100. J Clin Oncol. (2023) 41:3311–7. doi: 10.1200/JCO.22.02524
29. Scalera S, Mazzotta M, Corleone G, Sperati F, Terrenato I, Krasniqi E, et al. KEAP1 and TP53 frame genomic, evolutionary, and immunologic subtypes of lung adenocarcinoma with different sensitivity to immunotherapy. J Thorac Oncol. (2021) 16:2065–77. doi: 10.1016/j.jtho.2021.08.010
30. Saleh MM, Scheffler M, Merkelbach-Bruse S, Scheel AH, Ulmer B, Wolf J, et al. Comprehensive analysis of TP53 and KEAP1 mutations and their impact on survival in localized- and advanced-stage NSCLC. J Thorac Oncol. (2022) 17:76–88. doi: 10.1016/j.jtho.2021.08.764
31. Skoulidis F, Li BT, Dy GK, Price TJ, Falchook GS, Wolf J, et al. Sotorasib for lung cancers with KRAS p.G12C mutation. New Engl J Med. (2021) 384:2371–81. doi: 10.1056/NEJMoa2103695
32. de Langen AJ, Johnson ML, Mazieres J, Dingemans AC, Mountzios G, Pless M, et al. Sotorasib versus docetaxel for previously treated non-small-cell lung cancer with KRASG12C mutation: a randomised, open-label, phase 3 trial. Lancet. (2023) 401:733–46. doi: 10.1016/S0140-6736(23)00221-0
Keywords: NSCLC, lung adenocarcinoma, KRAS, STK11, KEAP1, TP53, co-mutations, survival
Citation: Frille A, Boeschen M, Wirtz H, Stiller M, Bläker H and von Laffert M (2024) TP53 co-mutations in advanced lung adenocarcinoma: comparative bioinformatic analyses suggest ambivalent character on overall survival alongside KRAS, STK11 and KEAP1 mutations. Front. Oncol. 14:1357583. doi: 10.3389/fonc.2024.1357583
Received: 18 December 2023; Accepted: 05 February 2024;
Published: 22 April 2024.
Edited by:
Wouter H. Van Geffen, Medical Center Leeuwarden, NetherlandsReviewed by:
Renwang Liu, Tianjin Medical University General Hospital, ChinaCopyright © 2024 Frille, Boeschen, Wirtz, Stiller, Bläker and von Laffert. This is an open-access article distributed under the terms of the Creative Commons Attribution License (CC BY). The use, distribution or reproduction in other forums is permitted, provided the original author(s) and the copyright owner(s) are credited and that the original publication in this journal is cited, in accordance with accepted academic practice. No use, distribution or reproduction is permitted which does not comply with these terms.
*Correspondence: Armin Frille, YXJtaW4uZnJpbGxlQG1lZGl6aW4udW5pLWxlaXB6aWcuZGU=; Maximilian von Laffert, bWF4aW1pbGlhbi52b24tbGFmZmVydEBtZWRpemluLnVuaS1sZWlwemlnLmRl
†These authors have contributed equally to this work
Disclaimer: All claims expressed in this article are solely those of the authors and do not necessarily represent those of their affiliated organizations, or those of the publisher, the editors and the reviewers. Any product that may be evaluated in this article or claim that may be made by its manufacturer is not guaranteed or endorsed by the publisher.
Research integrity at Frontiers
Learn more about the work of our research integrity team to safeguard the quality of each article we publish.