- 1Department of Gastroenterology, Hainan General Hospital (Affiliated Hainan Hospital of Hainan Medical University), Haikou, China
- 2Department of Gastroenterology and Hepatology, Zhongshan Hospital of Fudan University, Shanghai, China
Hepatocellular carcinoma (HCC) is a prevalent malignant tumor worldwide, characterized by high malignancy and rapid progression. Most cases are diagnosed at intermediate to advanced stages. Current treatment methods have limited efficacy, resulting in high recurrence rates and poor prognosis. Radical hepatectomy remains the primary treatment for HCC, complemented by radiotherapy, chemotherapy, targeted therapy, and immunotherapy. Despite significant improvement in patient prognosis with radical hepatectomy, the five-year survival rate post-surgery remains low; thus necessitating exploration of more effective therapeutic approaches. Ferroptosis is a recently discovered form of cell death that can modulate the occurrence and development of HCC through various mechanisms. This article aims to elucidate the mechanism of ferroptosis and its impact on HCC development to provide novel insights for diagnosis and treatment.
1 Introduction
Hepatocellular carcinoma (HCC) is the most common pathological type of primary liver cancer. Based on the 2020 global Cancer statistics, HCC has a global incidence of 4.7% and a mortality rate of 8.3%. In China, the incidence and mortality rates are higher at 9.0% and 13% respectively (1). Currently, the diagnosis of HCC relies primarily on imaging techniques, including abdominal ultrasound (US), contrast-enhanced computed tomography (CT), and magnetic resonance imaging (MRI), as well as serological markers such as Alpha-fetoprotein (AFP), liquid biopsy (such as circulating tumor DNA gene sequencing and methylation), metabolomics, and glycomicomics (2). The most commonly used methods for detecting HCC are US and serological content analysis of AFP. However, their accuracy is limited, US has an overall sensitivity of 84% in clinical screening and diagnosis of HCC patients, but its sensitivity is affected by the tumor diameter (3); the sensitivity of serum AFP in the diagnosis of early HCC ranges from 45.3% to 62.0%, with a specificity of 87% to 93% (4). Furthermore, due to the insidious nature and lack of specific symptoms in the early stages of HCC, it is difficult to screen using routine examinations. The clinical diagnosis of HCC is mostly made at an advanced stage. Therefore, it is essential to explore more effective treatments for liver cancer to reduce the medical and economic burden on society. In 2012, a mode of cell death that is dependent on iron was officially named ‘ferroptosis’. Studies have shown that ferroptosis plays a significant role in HCC (5). This article aims to discuss the regulatory mechanism of ferroptosis and its application in the treatment of HCC, providing new ideas for improving the level of HCC treatment.
2 Characteristics of ferroptosis
The morphological manifestations of ferroptosis are as follows: the mitochondrial volume of cells in which ferroptosis occurs shrinks and the cells become smaller. The mitochondrial crista decrease or disappear, the density of the membrane increases, and the inner membrane aggregates, resulting in rupture of the mitochondrial outer membrane. Biochemically, the aggregation of iron ions, the accumulation of reactive oxygen species (ROS) and the excessive accumulation of lipid peroxides are observed (6). When iron metabolism is disordered, it can mediate lipid peroxidation and eventually generate lipid peroxides, resulting in the accumulation of lipid peroxides, damage to the body’s cell membrane system, stability of the lipid bilayer is destroyed, the cell membrane is ruptured, and finally cells die due to ferroptosis (7).
3 Regulation of ferroptosis and its role in HCC
It has been found that ferroptosis plays an important regulatory role in HCC. Here, we will take three regulatory pathways of ferroptosis as the starting point, and briefly describe the action mechanisms of these three pathways, namely the relevant regulators (Figure 1; Table 1).
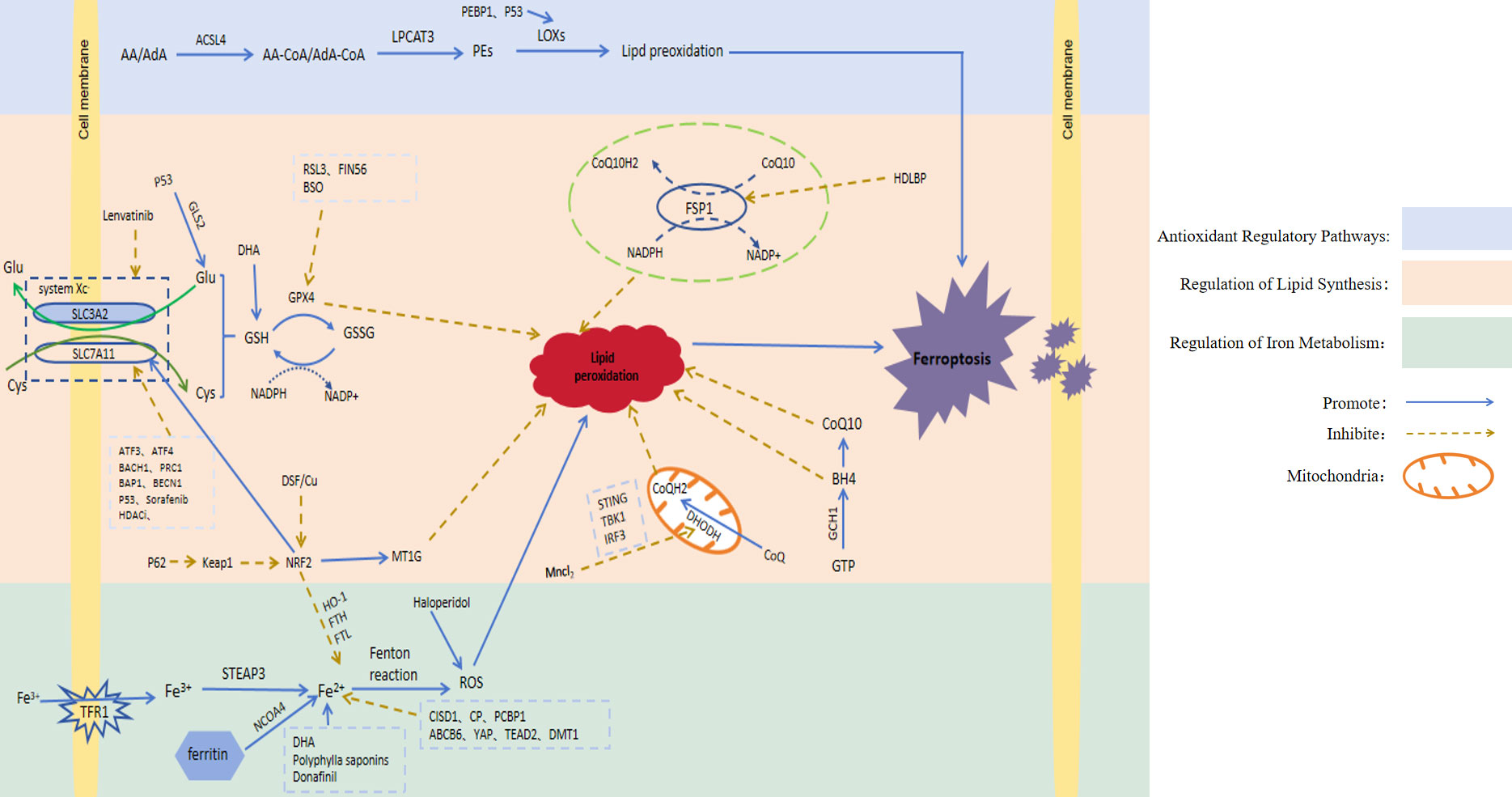
Figure 1 Signaling pathways involved in ferroptosis regulation. AA, Arachidonic acid; AdA, Adrenoic acid; LPCAT3, Lysophosphatidylcholine acyltransferase 3; PEs, Containing phosphatidylethanolamine from AA or AdA; Glu, Glutamate; Cys, Cysteine; System Xc-, Cystine/glutamate countertransporter system Xc; SLC7A11, Solute carrier family 7 member 11; SLC3A2, Solute carrier family 3 member 2; GLS2, Glutaminase 2; GSH, Glutathione; GPX4, Glutathione peroxidase 4; GSSG, Oxidised glutathione; NAD(P)H, Nicotinamide adenine dinucleotide (phosphate); RSL3, GPX4 inhibitor; FIN56, Ferroptosis inducers; ATF3, Activatingtranscriptionfactor 3; ATF4, Activatingtranscriptionfactor 4; BACH1, Basic leucine zipper transcription factor 1; PRC1, Protein regulator of cytokinesis 1; BECN1, Recombinant Human Beclin 1; BAP1, BRCA1-associated protein 1; Keap1, Kelch-like ECH-associated protein 1; NRF2, Nuclear factor erythroid-derived 2; MT-1G, Metallothionein; CoQ10H2, Ubiquinol; CoQ10, Coenzyme Q10; FSP1, Ferroptosis suppressor protein 1; HDLBP, High-density lipoprotein binding protein; IFN, Type-I interferon; DHODH, Dihydroorotate dehydrogenase; GTP, Guanosine triphosphate; GCH1, Guanosine triphosphate cyclohydrolase 1; BH4, Tetrahydrobiopterin; TFR1, Transferrin receptor 1; STEAP3, Six-transmembrane epithelial antigen of the prostate3; NCOA4, Nuclear receptor coactivator 4; FTH, Ferritin heavy chain; FTL, Ferritin light chain; HO-1, Heme oxygenase-1; CISD1, CDGSH iron-sulfur domain 1; CP, Ceruloplasmin; PCBP1, Poly C binding protein 1; ABCB6, ATP-binding cassette sub-family B member 6; YAP, Yes-associated protein; TEAD2, Transcriptional enhanced associate domain 2; DMT1, Divalent metal transporter1; ROS, Reactive oxygen species; DHA, Dihydroartemisinin; BSO, Auranofin and buthionine sulfoxide; HDACi, Histone deacetylase inhibitor; DSF/Cu, Disulfiram/copper.
3.1 Regulation of iron metabolism
Iron is an essential trace element in cells. It participates in the body’s metabolic processes in many ways and plays a crucial role in development of the body. Studies have shown that cancer cells increase intracellular iron concentration to promote cells through a variety of mechanisms (27). Ferrum is a redox-active metal due to its ability to easily gain or lose electrons. In the body, ferrum exists in two forms: A. Fe3+ is bound to ferritin and is responsible for participating in transport in the body. B. Fe2+ is absorbed by the body [5]. Specifically, Fe3+ binds to transferrin receptor 1 (TFR1) located on the cell membrane in order to enter the cell. In the cell, Fe3+ is reduced by iron oxidoreductase (six-transmembrane epithelial antigen of the prostate 3, STEAP3) to Fe2 + with higher catalytic activity, which is extremely unstable and can transfer electrons to intracellular oxygen. Peroxidation of lipids to lipid peroxides; On the other hand, in a weak acidic environment, Fe2+ can also generate lipid peroxides through the Fenton reaction by activating hydrogen peroxide (H2O2) in the body and generating hydroxyl radicals. Hydroxyl radicals are the most active free radicals of ROS, which can peroxidize lipids and generate lipid peroxides. Excessive lipid peroxides destroy the integrity of the cell membrane and eventually induce ferroptosis (28). As the most important organ for iron storage and iron metabolism in the body, the liver can regulate the iron content and the occurrence of ferroptosis in HCC cells, which may be a new treatment strategy for HCC patients. For example, CDGSH iron-sulfur domain 1 (CISD1) is an iron-containing mitochondrial outer membrane iron-sulfur protein. Studies have shown that this protein can further inhibit the occurrence of lipid peroxidation and reduce the accumulation of lipid peroxides by inhibiting mitochondrial iron uptake in HCC cells (8). Ceruloplasmin (CP) is a Fe2+ chelating glycoprotein. The depletion of CP can lead to Fe2+ accumulation and promote ferroptosis induced by erastin and RSL3 (9). Poly C binding protein 1 (PCBP1) is a multifunctional protein. One of its functions is to act as an iron chaperone for cellular solutes, binding iron and transferring it to receptor proteins in mammalian cells, thereby affecting iron content. PCBP1 deficiency mediates ferroptosis in HCC cells by increasing iron accumulation and ROS production (10). ABCB6, a member of the ATP-binding cassette (ABC) transporters, is one of the largest families of membrane proteins in most organisms. It has been shown that ABCB6 is highly expressed in HCC cells, and iron-containing porphyrin can be transported to the outside of cells under the action of ABCB6, thereby reducing intracellular iron content. At this time, ferroptosis in HCC cells was inhibited (11). The increased activity of Yes-associated protein (YAP) induces the expression of transferrin receptor (TFRC), and the overexpression of TFRC promotes iron absorption and increases iron content in HCC cells, thereby enhancing the sensitivity of HCC to ferroptosis (12). TEAD2 is a member of the transcriptional enhanced associate domain (TEAD) family (13). TEAD2 is significantly up-regulated in HCC, which plays an inhibitory role in the ferroptosis of HCC cells mainly by inhibiting the accumulation of iron. Ferritin can bind to nuclear receptor coactivator 4 (NCOA4) of autophagy and be transported to lysosomes, where ferritin is degraded and a large amount of Fe2+ is released, a process called ferroautophagy (14). Therefore, increasing the degradation of ferritin can increase the content of free iron, thereby promoting ferroptosis of cells. Divalent metal transporter1 (DMT1) shuttles iron across the plasma membrane, and most cells uptake iron through endocytosis; inhibition of DMT1 results in the production of ROS, lipid peroxidation, and cell death (15). Regulation of the iron metabolism pathway is the initiating factor of ferroptosis, and the accumulation of iron is one of the key factors in the ferroptosis of HCC cells. The increase in iron intake and the decrease in iron consumption or loss in the body due to various reasons can cause excessive intracellular iron accumulation, thereby promoting ferroptosis.
3.2 Regulation of lipid synthesis
The formation of lipid peroxides by lipid peroxidation is a key process in the induction of ferroptosis. Thus, lipid peroxides are the most dominant feature of ferroptosis. In vivo, the substrates of cell peroxidation are mainly lipids containing polyunsaturated fatty acids (PUFA). Lipid substances containing acyl groups of unsaturated fatty acids can be formed into lipid peroxides by enzymatic or non-enzymatic reactions. The first enzymatic reaction pathway: Arachidonic acid (AA) and adrenoic acid (AdA) are the most common polyunsaturated fatty acids in the body. These two compounds can first be catalyzed by acyl-CoA synthetase long-chain family member4 (ACSL4) to produce acyl-CoA derivatives. Then lysophosphatidylcholine acyltransferase 3 (LPCAT3) catalyzes the formation of phosphatidylethanolamine (PE). Finally, it is oxidized to toxic lipid peroxides by lipoxygenases (LOXs). Second, in a weak acid environment, PUFA is catalyzed by Fe2+ through the Fenton reaction to generate ROS, which then promote lipid peroxidation to generate lipid peroxides (16). In the body, the accumulation of lipid peroxides exceeds the body’s tolerance, which promotes the occurrence of ferroptosis. Other studies have reported that monounsaturated fatty acids (MUFA), unlike PUFA, have been shown to negatively regulate ferroptosis (29). However, the mechanism by which exogenous MUFA inhibit ferroptosis has not been fully elucidated. Liver is an important organ for lipid synthesis and metabolism. Ferroptosis of HCC cells is closely related to abnormal lipid metabolism in the liver. Previous studies (30) have shown that in HCC cells, ACSL4 can be activated by protein kinase Cβ2. Activated ACSL4 can initiate PUFA peroxidation, thereby triggering a series of lipid peroxidation reactions, resulting in increased production of lipid peroxides and the induction of ferroptosis in HCC cells. The above research provides us with inspiration: First, we can increase the content of PUFA such as AA and adrenal acid in the body. For example, AA is easily absorbed from meat intake, but AA can also be converted from other compounds. For example, the PUFA from plant food is mainly PUFA phospholipid containing linoleic acid (LA). LA can be mediated by fatty acid desaturase 1 and 2 (FADS1, FADS2) and elongase of very long chain fatty acids 5 (ELOVL5). After a series of enzymatic reactions, AA is finally generated (31, 32). Second, it can promote lipid peroxidation and increase the generation of lipid peroxides by increasing the content of key enzymes of peroxidation or catalyzing the reactions in which key enzymes are involved, such as phosphatidylethanolamine binding protein 1 (PEBP1). Ying et al. found that PEBP1 expression was low in liver cancer, indicating that this protein was negatively correlated with the degree of malignancy in human liver cancer, further reflecting the important role of PEBP1 in the occurrence and development of HCC (17).
3.3 Antioxidant regulatory pathways
The occurrence of ferroptosis is inseparable from the peroxidation reaction, which is closely related to the antioxidant regulatory pathway. Several antioxidant pathways have been found to be involved in ferroptosis.
3.3.1 GSH and GPX4 pathways
The glutathione (GSH)/glutathione peroxidase 4 (GPX4) pathway is the first identified molecular pathway that can regulate ferroptosis through antioxidants, which is also known as the classical pathway of ferroptosis. GSH is a small molecule with antioxidant activity in the body, and is composed of glutamate, glycine and cysteine. GSH protects cells from oxidative damage through antioxidant action. GSH is converted to glutathione oxidized (GSSG) under the catalytic action of GPX4, which reduces ROS in the body and reduces cell damage caused by excessive accumulation of ROS. From this point of view, GPX4 is used as a reducing agent in the anti-peroxidation reaction, and GSH has an antioxidant effect on GPX4, the antioxidant effect of the two complement each other and depend on each other. In addition, some studies have found that GPX4 can directly reduce lipid peroxide to the corresponding alcohol or reduce H2O2 to water. It is well known that H2O2 is a strong oxidant, if H2O2 is depleted, the peroxidation reaction in the body will be weakened, the formation of lipid peroxidation will be reduced, and the occurrence of ferroptosis will be inhibited (18). Therefore, the activities of GPX4 and GSH are inhibited by various pathways, the ability of anti-oxidation in cells is weakened, oxidation is dominant, and lipid is peroxidized, thus inducing ferroptosis of cells (33). GPX4 is the only enzyme found to protect mammalian biofilms from peroxidation-induced cell membrane damage because it directly reduces lipid peroxides to the related product, glutathione peroxidase (34). Li et al. (35) showed that compounds such as DSF/Cu can directly inhibit GPX4 activity and induce ferroptosis.
System Xc- is a type of amino acid reverse transporter attached to the body’s cell membrane, and its main function is the reverse transport of cystine and glutamate. The main components of System Xc- are SLC7A11 and SLC3A2. Of these, SLC7A11 is mainly responsible for transporting cystine and glutamate and is associated with System XC-transport activity, while SLC3A2 mainly functions as a chaperone protein. Glutamate is mainly found in the cell, whereas cystine is mainly found outside the cell. System Xc- can expel one molecule of glutamate from the cell, while also transporting one molecule of cystine from the outside to the cell. The reversed cystine is rapidly reduced to oxidation-active cysteine, which reacts with glutamate and glycine as substrates to produce GSH (36). Thus, when the amount of System Xc- in the cell is insufficient, it leads to insufficient GSH production, resulting in a consequent decrease in GPX4 oxidative activity. This provided us with new insight and suggested that System Xc- overexpression in human hepatocytes could significantly inhibit ferroptosis in HCC cells. Decreased GSH synthesis and inhibition of GPX4 activity for various reasons can promote ferroptosis in HCC cells. For example, ATF3, ATF4, BACH1, PRC1, BAP1, and BECN1 can inhibit the expression of SLC7A11 (19–23); Knockdown of metallothionein (MT-1G) accelerated GSH consumption (24). The above factors and compounds can weaken the antioxidant capacity in vivo through the GSH/GPX4 pathway, thereby improving the sensitivity of HCC cells to ferroptosis.
3.3.2 The NAD(P)H/FSP1/CoQ10 pathway
This pathway is another antioxidant pathway independent of the classical ferroptosis pathway. Ferroptosis suppressor protein 1 (FSP1) is an oxidoreductase of Coenzyme Q10 (CoQ10), which is an important factor in the regulatory mechanism of oxidative phosphorylation in vivo. It exerts antioxidant effects mainly by reducing NAD(P)H-dependent ubiquinone (CoQ) to panthenol with antioxidant activity (37), In the case of GPX4 deficiency, FSP1 can protect cells from ferroptosis by regenerating CoQ10 using NADH. On the other hand, FSP1-deficient cancer cells were found to be sensitive to ferroptosis activator-induced cell death, and intracellular administration of CoQ10 failed to reverse FSP1-deficient mediated ferroptosis. In contrast, FSP1 activates the transporter required endosomal sorting complex (ESCRT)-III-dependent membrane repair pathway. This mechanism is driven by impaired FSP1-dependent membrane repair machinery, but not by FSP1-dependent panthenol production. This suggests that FSP1 directly inhibits ferroptosis independent of panthenol production in HCC (38). Therefore, ferroptosis of HCC cells can be regulated by modulating the expression of the NAD(P)H/FSP1/CoQ10 pathway. For example, high-density lipoprotein binding protein (HDLBP) is significantly increased in HCC, which can bind and stabilize the long non-coding (lnc)RNA lncFAL (ferroptosis-related lncRNA). RNA lncFAL can directly bind to FSP1 and competitively eliminate TRIM69-dependent FSP1 polyubiquitination degradation to increase ferroptosis susceptibility in HCC (25).
3.3.3 The P62-Keap1-NRF2 pathway
The P62-kelch-like ECH-associated protein 1 (Keap1)-transcription factor nuclear factor erythroid-derived 2 (NRF2) signaling pathway protects the body from oxidative stress by inducing cells to produce protective factors. However, NRF2 plays a major role as an antioxidant. NRF2 is normally tightly bound to Keap1, and NRF2 is maintained at a low dissociation level in vivo. When the body is in a state of oxidative stress, NRF2 will immediately dissociate from Keap1. At the same time, NRF2 will be rapidly transported to the nucleus to activate the expression of target genes with antioxidant activity in the promoter region of the nucleus, and resist the oxidative stress by transcription to avoid cell damage caused by ferroptosis induced by cells under strong oxidative stress for a long time (39). P62 is an autophagy adaptor protein, which can promote the activation of NRF2 and the antioxidant effect by directly inhibiting Keap1. In addition, NRF2 can also promote the expression of GPX4, and the target mechanism of action is to regulate the expression of SystemXc-. NRF2, also induces the expression of MT-1G through the cystathionine pathway and inhibits ferroptosis. Therefore, it has been shown that inhibition of MT-1G expression can enhance the anti-cancer activity of sorafenib in the treatment of HCC (40). This provides a promising strategy for improving sorafenib resistance. NRF2 activation can further mediate HCC cells against ferroptosis by regulating iron-related genes such as HO-1, FTH, and FTL, preventing iron accumulation and further inhibiting the production of ROS (41).
3.3.4 Inhibition of DHODH pathway induction
Dihydroorotate dehydrogenase (DHODH) is a newly discovered antioxidant pathway that can regulate ferroptosis, but it mainly plays a role in regulating ferroptosis in mitochondria. DHODH, an enzyme widely expressed in the inner mitochondrial membrane, is the fourth catalytic rate-limiting enzyme for de novo pyrimidine synthesis and is involved in the regulation of de novo pyrimidine nucleotide synthesis. DHODH inhibits ferroptosis in the inner mitochondrial membrane by oxidizing dihydroorotic acid to orotic acid and reducing ubiquinone (CoQ) to CoQH2 with antioxidant activity (42). Therefore, the addition of a DHODH inhibitor such as breqinar increases sensitivity to ferroptosis in the inner mitochondrial membrane, and the inactivation of DHODH induces widespread ferroptosis in cancer cells with low GPX4 expression (42). As shown in recent studies, MnCl2 can promote the expression of type I interferon by enhancing the phosphorylation levels of STING, TBK1 and IRF3, and then inhibit the expression of DHODH in tumor cells, leading to an increase in mitochondrial ROS and lipid peroxidation, thereby increasing the sensitivity of cells to ferroptosis (26). Li et al. found that in HCC, inhibition of DHODH expression could enhance the sensitivity of HCC cells to sorafenib-induced ferroptosis (43). This study provides a new idea for the future treatment of HCC by targeting ferroptosis.
3.3.5 Blockade of GCH1/BH4 pathway induced ferroptosis
GTP Cyclohydrolase 1 (GCH1) is the first rate-limiting enzyme for de novo synthesis of tetrahydrobipterin (BH4), an antioxidant that protects cells from lipid peroxidation. On the other hand, BH4 is also a key enzyme in the synthesis of CoQ precursors, which plays a dual role in antioxidant activity (44). Epigenetic silencing of GCH1 promotes HCC cell growth by inhibiting the de novo synthesis of BH4 and activating the superoxide anion mediated ASK1/p38 signaling pathway, suggesting that targeting the GCH1/BH4 pathway may be a promising therapeutic strategy against HCC (45). Furthermore, overexpression of GCH1 in mouse fibroblasts significantly inhibited GPX4 induced ferroptosis. These results indicated that GCH1 overexpression could selectively inhibit the peroxidation of some unsaturated fatty acids and thus down-regulate ferroptosis (46). Therefore, blocking the pathway and inhibiting the expression of CPX4 can play a synergistic role in inducing ferroptosis, which could provide us with a new method for the future treatment of HCC: CPX4 inhibitors can be combined with GCH1/BH4 pathway inhibitors, and both play a role in inducing ferroptosis in HCC cells to fight tumor proliferation.
3.4 Other molecular pathways: the p53 signaling pathway
P53 is a tumor suppressor gene. It has been found that p53 can regulate ferroptosis of HCC cells through multiple pathways as follows (1): The expression of SLC7A11 is inhibited by transcription, the uptake of cystine is inhibited, the substrate of GSH is reduced, synthesis is insufficient, and the content of GSH is decreased, thus the activity of GPX4 in cells is inhibited, the antioxidant capacity in vivo is weakened, oxidation is dominant, and ferroptosis of HCC cells is promoted (47). (2) Regulation of induced spermidine/spermine N1-acetyltransferase (SAT1) gene expression, thereby promoting arachidonate-15-lipoxygenase (ALOX15), an enzyme that promotes lipid peroxidation, leading to ferroptosis (48). (3) Overexpression of Glutaminase 2 (GLS2), which is a key enzyme in the conversion of glutamine to glutamate, one of the three synthesis substrates of GSH, can inhibit the ferroptosis of HCC cells by increasing GSH synthesis (49). (4) Iron metabolism is regulated by ferredoxin reductase (FDXR). FDXR regulates iron metabolism through the transfer of electrons to cytochrome P450 by ferredoxin in mitochondria, and p53 can induce the transcriptional expression of FDXR. At the same time, FDXR promoted the translation of p53mRNA through intracellular iron regulatory protein 2, thereby increasing the level of p53 protein. Thus, FDXR and p53 are mutually regulated and can promote each other, and this FDXR-p53 loop has been shown to regulate ferroptosis in HCC cells by maintaining mitochondrial iron homeostasis (50). As mentioned above, p53 can regulate HCC cell development through a variety of mechanisms. New guidelines for the diagnosis and treatment of HCC can be provided using these multiple regulatory ideas.
4 Treatment of HCC based on ferroptosis
The induction of ferroptosis in HCC cells provides a new therapeutic option for HCC treatment. In the current treatment of HCC, some drugs have been found to exert anti-tumor effects by inducing cell ferroptosis (Table 2).
4.1 Sorafenib
Sorafenib is the first targeted drug approved for clinical application in the treatment of patients with advanced liver cancer worldwide. Previously, as a multikinase inhibitor, sorafenib was considered to play an anti-cancer effect mainly through an enzymatic reaction. However, in recent years, the anti-cancer effect of sorafenib has been confirmed to be mainly due to the induction of ferroptosis in HCC cells. There are two known ways sorafenib induces ferroptosis: (1) silencing the retinoblastoma (Rb) gene in HCC cells by transfection of short hairpin RNA. Silencing of Rb protein can increase the sensitivity of HCC cells to ferroptosis, thereby enhancing its anti-cancer effect (51). (2) By inhibiting the production of System Xc-, the antioxidant capacity of HCC cells is weakened, oxidation is dominant, the production of lipid peroxides is increased, and HCC cells are more susceptible to ferroptosis (51). At the same time, resistance mechanisms related to sorafenib are also involved in the regulation of ferroptosis: Some studies have found that following sorafenib treatment, p62 protein can inactivate Keap1 and inhibit the degradation of NRF2, resulting in an increase of NRF2 content and the inhibition of iron accumulation by activating iron-related genes, thereby reducing the generation of ROS and inhibiting the occurrence of ferroptosis. In addition, NRF2 activation increased MT-1G expression in HCC cells and inhibited lipid peroxidation by blocking GSH consumption. This suggests that inhibition of NRF2 expression may enhance the efficacy of sorafenib in the treatment of HCC. For example: A. Quiescin Q6 Sulfhydryl Oxidase 1 (QSOX1) is a disulfide catalyst that inhibits epidermal growth factor (EGF)-induced EGF receptor (EGFR) activation by promoting ubiquitin-mediated EGFR degradation and accelerating its intracellular endosomal trafficking, thereby inhibiting NRF2 activity. Furthermore, it promotes sorafenib-induced ferroptosis in HCC cells (52). B. Gao et al. found that the YAP/TAZ and ATF4 genes in the nucleus were highly expressed in HCC cells with sorafenib resistance, and the expression of SLC7A11 in these cells was increased, which reduced the expression level of ROS and the production of lipid peroxides, and inhibited the ferroptosis of HCC cells (53). C. Inhibition of phosphoseryl-tRNA kinase (PSTK) increased the sensitivity of HCC cells to chemotherapy. PSTK has been implicated in the inhibition of chemotherapy-induced ferroptosis in HCC cells, and depletion of PSTK leads to GPX4 inactivation and disruption of GSH metabolism due to inhibition of selenocysteine and cysteine synthesis, thereby enhancing ferroptosis induction after targeted therapy. Punicalin, a drug used to treat hepatitis B virus, was identified as a possible PSTK inhibitor of peroxide accumulation and negatively regulated ferroptosis in HCC cells. Inhibition of MT-1G expression enhanced the anti-cancer activity of sorafenib by inducing ferroptosis in vitro and in vivo. This finding indicates that MT-1G is a novel regulator of ferroptosis in HCC and demonstrates a novel molecular mechanism of sorafenib resistance (37). It can be seen that NRF2 status has an important role in influencing the therapeutic efficacy of ferroptosis in HCC treatment, showing synergistic efficacy when applied together with sorafenib for HCC treatment in vitro and in vivo (54).
4.2 Dihydroartemisinin
Arteannuin (ART) is a malaria drug discovered by the Chinese scientist Tu Youyou (55, 56). Dihydroartemisinin (DHA) is one of the many derivatives of ART. Studies have shown that DHA can not only be used for the treatment of malaria, but also has anti-tumor effects. ART is considered to be one of the many inducers of ferroptosis, which can induce ferroptosis in a variety of malignant tumor cells and plays an anti-tumor role. It has been found that DHA induces ferroptosis in cancer cells mainly through the following mechanisms: (1) by increasing the degradation of ferritin by transferrin, lysosomes and autophagosomes, and directly increasing the concentration of Fe in the labile iron pool, thereby promoting the accumulation of ROS and inducing ferroptosis (57). (2) By inhibiting the synthesis of GSH and thus the activity of GPX4 is then inhibited, the antioxidant effect is lost, and oxidation is enhanced, which is conducive to the occurrence of ferroptosis. (3) Activation of the ER stress response, PERK-ATF4-HSPA5 pathway and ATF4-CHOP-CHAC1 pathway affect Fe content to regulate ferroptosis. (4) By affecting the electron transport chain in mitochondria, it promotes the generation of ROS and increases the sensitivity of cells to ferroptosis (58). (5) Mammalian 15-lipoxygenase/phosphoethanolamine binding protein-1 (15-LO) oxidizes free PUFA with the help of PEBP1 to form the PEBP1/15-LO complex. DHA inhibits the ubiquitin-proteasome pathway of PEBP1 to increase its protein level and bind to 15-LO. Furthermore, DHA promoted ferroptosis induced by lipid peroxidation of HCC cell membranes, thereby exerting the anti-HCC activity of DHA (17). In conclusion, DHA induces ferroptosis through multiple pathways, which fully illustrates the potential role of artemisinin in the treatment of tumors. At present, DHA has been approved for clinical trials in cancer, and clinical trials of artemisinin in the treatment of HCC are under investigation (59). It is believed that artemisinin and its derivatives will be used in the treatment of clinical tumors in the near future.
4.3 Others
(1) Polyphylla saponins, are Chinese herbal medicine components (60). Polyphyllin can increase the iron content of HCC cells by increasing ferritin phagocytosis, thereby inducing ferroptosis of HCC cells. (2) Haloperidol is an antipsychotic drug, but its combination can promote sorafenib-induced ferroptosis in HCC cells. As a sigma receptor 1 antagonist, haloperidol mainly promotes the accumulation of intracellular ROS, thereby promoting lipid peroxidation and inducing ferroptosis. Haloperidol combined with sorafenib has been shown to enhance sorafenib-induced ferroptosis in the treatment of HCC patients (61). (3) The combination of auranofin and buthionine sulfoxide (BSO) or the treatment combination of sub-toxic concentrations of erastin and BSO, both inhibited GPX4 production and affected HCC cell ferroptosis (62). (4) Lenvatinib is another targeted drug that can be used for the treatment of advanced HCC. In 2018, lenvatinib was officially approved by the US Food and Drug Administration for the first-line treatment of patients with advanced HCC. The mechanism of ferroptosis induction mainly involves inhibiting fibroblast growth factor receptor-4 (FGFR4) to inhibit System Xc- expression and increase ROS accumulation, thereby inducing ferroptosis in HCC cells (63). (5) Donafinil, a deuterated derivative of sorafenib, is an approved standard treatment for patients with advanced HCC. Preclinical studies using small-molecule inhibitors and drug-induced CRISPR libraries have shown that donafinil acts synergistically with GSK-J4 to promote HMOX1 expression and increase intracellular Fe2+ levels, ultimately leading to ferroptosis in HCC (64, 65). (6) Histone deacetylase inhibitors (HDACi) can inhibit SLC7A11, induce ROS accumulation and induce ferroptosis in tumor cells (66). Of these, sodium butyrate (NaB) has been shown to inhibit the proliferation of HCC cells as a class I HDACi (67). (7) DSF/Cu can disrupt mitochondrial homeostasis, increase the free iron pool, enhance lipid peroxidation, and eventually lead to iron-induced cell death. Mechanistically, DSF/Cu can enhance the cytotoxicity of sorafenib and inhibit tumor growth in vitro and in vivo by inhibiting NRF2 (68).
4.4 Radiotherapy
Radiotherapy is considered beneficial for patients with unresectable or advanced HCC. It is an important part of the treatment of these patients. Studies have demonstrated that radiotherapy can trigger ferroptosis in cancer cells (69). Radiotherapy induces ROS production and ACSL4 expression, leading to lipid peroxidation and ferroptosis. As an adaptation, radiotherapy also induces overexpression of death suppressor genes such as SLC7A11 and GPX4. Tumor cells overexpressing SLC7A11 and GPX4 show marked resistance to radiotherapy. Sensitization of radioresistant cancer cells to radiotherapy can be achieved through inactivation of SLC7A11 or GPX4 with ferroptosis inducers (70). For instance, suppressor of cytokine signaling 2 (SOCS2) is a potential target for radiosensitization in HCC (71), the sensitivity of HCC cells to radiotherapy can be increased by overexpressing SOCS2, which promotes the degradation of SLC7A11. The protein complex regulated by nuclear respiratory factor 1/Ras-associated nuclear protein/dihydrolipoamide dehydrogenase can be targeted for radiation to enhance the radiosensitivity of HCC cells by inducing ferroptosis (72). Ionizing radiation reduces the expression of Copper metabolism MURR1 domain 10 (COMMD10), inhibits the ubiquitin degradation of HIF1α, and impairs its binding to HIF1α. While inhibiting ferroptosis in HCC, it promotes nuclear translocation of HIF1α and transcription of CP and SLC7A11. Additionally, elevated CP promotes HIF1α expression by reducing iron, forming a positive feedback loop. In conclusion, these results indicate that COMMD10 enhances ferroptosis and radiosensitivity in HCC by disrupting Cu-Fe homeostasis and inhibiting HIF1α/CP loop function (73). These findings indicate that gene sensitizing therapy can enhance the effectiveness of radiotherapy, decrease the dosage and adverse effects of radiotherapy, and improve the survival rate of HCC patients when combined with COMMD10 overexpression.
4.5 Immune microenvironment and related immunotherapy
In recent years, many studies have shown that tumor cell ferroptosis can reshape the tumor immune microenvironment. Immune cells can also promote tumor cell ferroptosis, thereby exerting anti-tumor effects (74).
CD8+ T cells are the main executors of adaptive immunity. In addition to maintaining the survival and expansion of CD4+ and CD8+ T cells, GPX4 acts as a regulator of ferroptosis to protect activated regulatory T cells from ferroptosis and plays a major role in the inhibition of anti-tumor immunity (75). CD8+ T cells are the major players in adaptive immunity. In addition to maintaining the survival and expansion of CD4+ and CD8+ T cells, GPX4 acts as a regulator of ferroptosis to protect activated regulatory T cells from ferroptosis and plays an important role in inhibiting anti-tumor immunity (76). IFN-γ, released by CD8+ T cells, alters the lipid composition of tumor cells via ACSL4 and induces an immunogenic ferroptosis of the tumor cells (77). A number of studies of targeted ferroptosis in combination with immune checkpoint blockade therapy have also shown that induction of ferroptosis in tumor cells in combination with anti-programmed death 1 (PD-1) antibody therapy has a strong anti-tumor effect (78). Treg cells are an immunosuppressive subset of CD4+ T cells that play an important role in maintaining self-tolerance and immune homeostasis. The abundance of Treg cells in the peripheral blood of patients with HCC was higher than that of patients without HCC (79). GPX4 is essential for Treg cells to maintain immune tolerance, and GPX4 deficiency causes excessive accumulation of lipid peroxides in Treg cells and triggers ferroptosis. In addition, GPX4 deficiency in Treg cells increased mitochondrial superoxide production and promoted IL-1β production. GPX4-specific knockdown of Treg cells suppressed tumor growth and prevented tumor immune escape without inducing significant autoimmunity (75).
Tumor-associated macrophages (TAMs) play an important role in recycling iron produced during red blood cell clearance. They are also an important component of host defense, protecting the host from the invasion of pathogens (80). M1 macrophages were more resistant to ferroptosis induced by GPX4 deletion. The M2 isoform is more susceptible to ferroptosis induced by GPX4 inhibition due to its lack of inducible nitric oxide synthase expression and nitric oxide production (81). APOC1 is overexpressed in TAMs of HCC tissues in comparison to normal tissues, and inhibition of APOC1 reverses the transition from M2 phenotype to M1 phenotype via the ferroptosis pathway, thereby enhancing the efficacy of anti-PD1 immunotherapy for HCC (82).
Pathologically activated neutrophil-myeloid-derived suppressor cells (PMN-MDSCs), downregulation of GPX4 promotes ferroptosis of PMN-MDSCs. Tumor PMN-MDSCs were more sensitive to ferroptosis and could mediate immunosuppression after ferroptosis compared to PMN-MDSCs isolated from bone marrow and spleen (83). Thus, ferroptosis represents a unique and targeted immunosuppressive mechanism of PMN-MDSCs in TEMs that can be pharmacologically modulated to limit the progression of tumors (84). Thus, ferroptosis is a unique and targeted PMN-MDSC immunosuppressive mechanism in TEMs that can be pharmacologically modulated to limit tumor progression.
Natural killer (NK) cell dysfunction leads to increased tumor incidence and growth rate. Dysfunctional NK cells in the TME were found to have increased expression of proteins associated with lipid peroxidation and oxidative damage, and cell morphology was similar to that of ferroptosis cells. Oxidative stress associated with lipid peroxidation may inhibit NK cell glucose metabolism, leading to NK cell dysfunction in the TME (85). The liver contains large numbers of hyporeactive NK cells, which are an important source of hepatic tolerance that can expose the body to enteric exogenous antigens on a daily basis without causing inflammation. We do not know whether NK cells themselves are subject to ferroptosis, but in a study of sepsis, researchers reported that many ferroptosis-related genes were dysregulated in models of liver failure and promoted liver failure by interacting with NK cells (86).
In conclusion, ferroptosis is expected to provide new ideas and targets to improve the efficacy of immunotherapy in HCC patients. Through a strategy of combining ferroptosis with immunotherapy, it brings new hope for the treatment of HCC. Nanotechnology has had remarkable success in cancer therapy. Studies have shown that in SRF@Hb-Ce6 nanoparticles, constructed with hemoglobin (Hb) linked to the photosensitiser chlorin e6 (Ce6) and loaded with sorafenib (SRF), Hb uses its own oxygen-bound iron to provide oxygen for photodynamic therapy (PDT) and iron for ferroptosis. PDT enhanced ferroptosis by inducing IFNγ secretion from immune cells (87). Carbon quantum dots (CQDs) are a class of zero-dimensional carbon nanomaterials with significant fluorescence capability. Yao et al. reported a CQD-based biocompatible nanoenzyme prepared from chlorogenic acid (ChA), a major bioactive natural product in coffee. ChACQDs induced ferroptosis through a GSH depletion-dependent ROS production mechanism, while attracting a large number of tumor-expanding immune cells, including T cells and NK cells, and activating systemic anti-tumor immune responses (88). Nanoparticles may therefore be an effective anti-tumor strategy. They induce ferroptosis and mobilize an immune response against HCC cells.
4.6 Combination therapy
Ferroptosis plays an important role in anti-tumor therapy, but it is often difficult to achieve the desired effect with a single treatment method. Therefore, the treatment of tumors is usually a combination of several treatment modalities, especially in advanced patients. Many studies have shown great potential for synergistic therapy by inducing ferroptosis in cancer cells in combination with other existing therapies. A. The combination of immunotherapy and targeted molecular therapy has shown promising therapeutic effects in HCC. For example, phosphoglycerate mutase 1 (PGAM1) has been identified as a novel immunometabolism target. Inhibition of PGAM1 exerts anti-tumor effects by promoting ferroptosis and CD8+ T-cell infiltration, and can significantly enhance the efficacy of anti-PD-1 immunotherapy in HCC through synergy with anti-PD-1 immunotherapy in HCC, providing preclinical evidence for the use of this combination therapy (89). Other studies have reported that combining ferroptosis induction with MDSC blockade sensitises primary and metastatic tumors in the liver to immune checkpoint blockade and can be used to treat HCC and liver metastases (90). B. In radiotherapy and molecular targeted therapy, SOCS2 acts as a bridge to transfer the bound ubiquitin to SLC7A11, promoting polyubiquitination and degradation of K48-associated SLC7A11, ultimately leading to ferroptosis and radiosensitivity of HCC. These results suggest that SOCS2-targeting drugs may be combined with radiotherapy to improve patient prognosis (71). C. Sorafenib plays a role by inducing ferroptosis in HCC cells, but sorafenib resistance has also been reported to be associated with ferroptosis in several studies. Upregulation of fatty acid synthase (FASN) antagonized SLC7A11-mediated ferroptosis, thereby promoting sorafenib resistance. The FASN inhibitor orlistat combined with sorafenib showed significant synergistic anti-tumor effects and reversed sorafenib resistance in vitro and in vivo. The combination of the two has excellent synergistic anti-tumor effects in sorafenib-resistant HCC cells (91). D. DHA loaded Fe-MnO2 nanosheets are used in ferroptosis and immunotherapy. DHA not only acts as an ferroptosis inducer, but also acts as an immunomodulator to inhibit Tregs to exert systemic anti-tumor effects. Fe-MnO2/DHA is a multimodal treatment for HCC driven by ferroptosis, apoptosis, and immune activation, which significantly improves the efficacy of synergistic cancer treatment (92).
5 Prospects
Research progress in ferroptosis has been a hot topic in recent years. Ferroptosis plays an important role in combating the occurrence and development of many diseases. This article mainly reviews the regulation of ferroptosis in HCC. As our insight into the regulation of ferroptosis in liver cancer continues to deepen, based on the study of ferroptosis, we have new ideas for the diagnosis and treatment of HCC, such as early diagnosis, new tumor classification, improvement in drug resistance, development of new drugs, and evaluation of disease prognosis. By reviewing the relevant references, we have confirmed that there are potential biomarkers of ferroptosis that can help in the early diagnosis and monitoring of HCC. Based on these biomarkers, researchers have conducted relevant in-depth research on the treatment of HCC. For example, the study by Deng et al. showed that coiledcoil containing domain containing 25 (CCDC25) may be a potential diagnostic and prognostic marker for HCC associated with immune infiltration and ferroptosis. In-depth studies have shown that downregulation of CCDC25 increases the sensitivity of HCC patients to a variety of drugs, including sorafenib (93). Yan et al. found that BAP1-mutant HCCS had a reduced ability to promote ferroptosis, suggesting that high BAP1 expression is associated with ferroptosis. They identified BAP1 as a potential diagnostic and prognostic marker for HCC and as a marker for ferroptosis and immune checkpoint blockade therapies (94). However, there are still a lot of questions that need to be answered, such as: A. How are the three main regulatory pathways of ferroptosis related to each other? B. The current ferroptosis-based drugs for HCC treatment are in the early stages of development, and many of them have not been used in the clinic. C. The direct targets of ferroptosis in HCC have not been fully defined. D. Induction of ferroptosis has a dual effect. When ferroptosis is induced in HCC cells, it also induces ferroptosis in other normal cells, which damages normal cells. How to better induce ferroptosis in HCC cells and avoid ferroptosis in other cells? Induction of ferroptosis has a dual effect. While it induces ferroptosis in HCC cells, it also induces ferroptosis in normal cells and damages normal cells. Therefore, how to effectively induce ferroptosis in HCC cells without affecting normal cells is an important consideration. We believe that molecular targeted therapy is the important part to solve this problem. Based on the differential expression of ferroptosis-related regulatory factors in HCC, the targeted drugs that regulate related factors can specifically induce ferroptosis in HCC cells without damaging or minimally damaging normal cells, and exert an effective anti-tumor effect. For example, Li et al. identified N6F11 as a ferroptosis inducer and found that N6F11 must bind to a specific domain of the ubiquitin E3 ligase (TRIM25) to trigger the ubiquitination degradation of GPX4. Since TRIM25 is mainly expressed in pancreatic cancer tumor cells and not in immune cells, this small molecule can selectively induce GPX4 protein degradation in pancreatic cancer tumor cells and induce T cell-mediated anti-tumor immunity without inducing ferroptosis in immune cells (95). Further research is needed to address these issues, particularly in the treatment of HCC, especially for patients who are not candidates for surgical resection. New drugs and solutions to drug resistance are also needed.
Author contributions
SJ: Writing – original draft, Writing – review & editing. GZ: Writing – review & editing. YM: Supervision, Writing – review & editing. DW: Writing – review & editing. DX: Writing – review & editing. SZ: Writing – review & editing. XJ: Funding acquisition, Resources, Supervision, Writing – review & editing.
Funding
The author(s) declare financial support was received for the research, authorship, and/or publication of this article. This study was supported by the National Natural Science Foundation of China (No.81960446).
Conflict of interest
The authors declare that the research was conducted in the absence of any commercial or financial relationships that could be construed as a potential conflict of interest.
Publisher’s note
All claims expressed in this article are solely those of the authors and do not necessarily represent those of their affiliated organizations, or those of the publisher, the editors and the reviewers. Any product that may be evaluated in this article, or claim that may be made by its manufacturer, is not guaranteed or endorsed by the publisher.
References
1. Sung H, Ferlay J, Siegel RL, Laversanne M, Soerjomataram I, Jemal A, et al. Global cancer statistics 2020: GLOBOCAN estimates of incidence and mortality worldwide for 36 cancers in 185 countries. CA Cancer J Clin. (2021) 71:209–49. doi: 10.3322/caac.21660
2. Wang W, Wei C. Advances in the early diagnosis of hepatocellular carcinoma. Genes Dis. (2020) 7:308–19. doi: 10.1016/j.gendis.2020.01.014
3. Harris PS, Hansen RM, Gray ME, Massoud OI, McGuire BM, Shoreibah MG. Hepatocellular carcinoma surveillance: An evidence-based approach. World J Gastroenterol. (2019) 25:1550–59. doi: 10.3748/wjg.v25.i13.1550
4. Choi J, Kim GA, Han S, Lee W, Chun S, Lim YS. Longitudinal assessment of three serum biomarkers to detect very early-stage hepatocellular carcinoma. Hepatology. (2019) 69:1983–94. doi: 10.1002/hep.30233
5. Chen J, Li X, Ge C, Min J, Wang F. The multifaceted role of ferroptosis in liver disease. Cell Death Differ. (2022) 29:467–80. doi: 10.1038/s41418-022-00941-0
6. Dixon SJ, Lemberg KM, Lamprecht MR, Skouta R, Zaitsev EM, Gleason CE, et al. Ferroptosis: an iron-dependent form of nonapoptotic cell death. Cell. (2012) 149:1060–72. doi: 10.1016/j.cell.2012.03.042
7. Harris IS, DeNicola GM. The complex interplay between antioxidants and ROS in cancer. Trends Cell Biol. (2020) 30:440–51. doi: 10.1016/j.tcb.2020.03.002
8. Lu T, Li C, Xiang C, Gong Y, Peng W, Chen C. Overexpression of CISD1 predicts worse survival in hepatocarcinoma patients. BioMed Res Int. (2022) 2022:7823191. doi: 10.1155/2022/7823191
9. Shang Y, Luo M, Yao F, Wang S, Yuan Z, Yang Y. Ceruloplasmin suppresses ferroptosis by regulating iron homeostasis in hepatocellular carcinoma cells. Cell Signal. (2020) 72:109633. doi: 10.1016/j.cellsig.2020.109633
10. Werth EG, Rajbhandari P, Stockwell BR, Brown LM. Time course of changes in sorafenib-treated hepatocellular carcinoma cells suggests involvement of phospho-regulated signaling in ferroptosis induction. Proteomics. (2020) 20:e2000006. doi: 10.1002/pmic.202000006
11. Zhang J, Zhang X, Li J, Song Z. Systematic analysis of the ABC transporter family in hepatocellular carcinoma reveals the importance of ABCB6 in regulating ferroptosis. Life Sci. (2020) 257:118131. doi: 10.1016/j.lfs.2020.118131
12. Zhu G, Murshed A, Li H, Ma J, Zhen N, Ding M, et al. O-GlcNAcylation enhances sensitivity to RSL3-induced ferroptosis via the YAP/TFRC pathway in liver cancer. Cell Death Discovery. (2021) 7:83. doi: 10.1038/s41420-021-00468-2
13. Ren X, Wang X, Yan Y, Chen X, Cai Y, Liang Q, et al. Integrative bioinformatics and experimental analysis revealed TEAD as novel prognostic target for hepatocellular carcinoma and its roles in ferroptosis regulation. Aging (Albany NY). (2022) 14:961–74. doi: 10.18632/aging.203853
14. Qu L, He X, Tang Q, Fan X, Liu J, Lin A. Iron metabolism, ferroptosis, and lncRNA in cancer: knowns and unknowns. J Zhejiang Univ Sci B. (2022) 23:844–62. doi: 10.1631/jzus.B2200194
15. Turcu AL, Versini A, Khene N, Gaillet C, Caneque T, Muller S, et al. DMT1 inhibitors kill cancer stem cells by blocking lysosomal iron translocation. Chemistry. (2020) 26:7369–73. doi: 10.1002/chem.202000159
16. Jiang X, Stockwell BR, Conrad M. Ferroptosis: mechanisms, biology and role in disease. Nat Rev Mol Cell Biol. (2021) 22:266–82. doi: 10.1038/s41580-020-00324-8
17. Su Y, Zhao D, Jin C, Li Z, Sun S, Xia S, et al. Dihydroartemisinin induces ferroptosis in HCC by promoting the formation of PEBP1/15-LO. Oxid Med Cell Longev. (2021) 2021:3456725. doi: 10.1155/2021/3456725
18. Liu J, Kang R, Tang D. Signaling pathways and defense mechanisms of ferroptosis. FEBS J. (2022) 289:7038–50. doi: 10.1111/febs.16059
19. Li Y, Yan J, Zhao Q, Zhang Y, Zhang Y. ATF3 promotes ferroptosis in sorafenib-induced cardiotoxicity by suppressing Slc7a11 expression. Front Pharmacol. (2022) 13:904314. doi: 10.3389/fphar.2022.904314
20. He F, Zhang P, Liu J, Wang R, Kaufman RJ, Yaden BC, et al. ATF4 suppresses hepatocarcinogenesis by inducing SLC7A11 (xCT) to block stress-related ferroptosis. J Hepatol. (2023) 79(2):362–77. doi: 10.1016/j.jhep.2023.03.016
21. Nishizawa H, Matsumoto M, Shindo T, Saigusa D, Kato H, Suzuki K, et al. Ferroptosis is controlled by the coordinated transcriptional regulation of glutathione and labile iron metabolism by the transcription factor BACH1. J Biol Chem. (2020) 295:69–82. doi: 10.1074/jbc.RA119.009548
22. Zhang Y, Koppula P, Gan B. Regulation of H2A ubiquitination and SLC7A11 expression by BAP1 and PRC1. Cell Cycle. (2019) 18:773–83. doi: 10.1080/15384101.2019.1597506
23. Kang R, Zhu S, Zeh HJ, Klionsky DJ, Tang D. BECN1 is a new driver of ferroptosis. Autophagy. (2018) 14(12):2173–75. doi: 10.1080/15548627.2018.1513758
24. Sun X, Niu X, Chen R, He W, Chen D, Kang R, et al. Metallothionein-1G facilitates sorafenib resistance through inhibition of ferroptosis. Hepatology. (2016) 64:488–500. doi: 10.1002/hep.28574
25. Yuan J, Lv T, Yang J, Wu Z, Yan L, Yang J, et al. HDLBP-stabilized lncFAL inhibits ferroptosis vulnerability by diminishing Trim69-dependent FSP1 degradation in hepatocellular carcinoma. Redox Biol. (2022) 58:102546. doi: 10.1016/j.redox.2022.102546
26. Zhang S, Kang L, Dai X, Chen J, Chen Z, Wang M, et al. Manganese induces tumor cell ferroptosis through type-I IFN dependent inhibition of mitochondrial dihydroorotate dehydrogenase. Free Radic Biol Med. (2022) 193:202–12. doi: 10.1016/j.freeradbiomed.2022.10.004
27. Jiao L, Li X, Luo Y, Wei J, Ding X, Xiong H, et al. Iron metabolism mediates microglia susceptibility in ferroptosis. Front Cell Neurosci. (2022) 16:995084. doi: 10.3389/fncel.2022.995084
28. Ye CL, Du Y, Yu X, Chen ZY, Wang L, Zheng YF, et al. STEAP3 affects ferroptosis and progression of renal cell carcinoma through the p53/xCT pathway. Technol Cancer Res Treat. (2022) 21:15330338221078728. doi: 10.1177/15330338221078728
29. Magtanong L, Ko PJ, To M, Cao JY, Forcina GC, Tarangelo A, et al. Exogenous monounsaturated fatty acids promote a ferroptosis-resistant cell state. Cell Chem Biol. (2019) 26:420–32 e9. doi: 10.1016/j.chembiol.2018.11.016
30. Zhang HL, Hu BX, Li ZL, Du T, Shan JL, Ye ZP, et al. PKCbetaII phosphorylates ACSL4 to amplify lipid peroxidation to induce ferroptosis. Nat Cell Biol. (2022) 24:88–98. doi: 10.1038/s41556-021-00818-3
31. Lee JY, Nam M, Son HY, Hyun K, Jang SY, Kim JW, et al. Polyunsaturated fatty acid biosynthesis pathway determines ferroptosis sensitivity in gastric cancer. Proc Natl Acad Sci U.S.A. (2020) 117:32433–42. doi: 10.1073/pnas.2006828117
32. Yamane D, Hayashi Y, Matsumoto M, Nakanishi H, Imagawa H, Kohara M, et al. FADS2-dependent fatty acid desaturation dictates cellular sensitivity to ferroptosis and permissiveness for hepatitis C virus replication. Cell Chem Biol. (2022) 29:799–810 e4. doi: 10.1016/j.chembiol.2021.07.022
33. Lv Y, Wu M, Wang Z, Wang J. Ferroptosis: From regulation of lipid peroxidation to the treatment of diseases. Cell Biol Toxicol. (2023) 39(3):827–51. doi: 10.1007/s10565-022-09778-2
34. Guerriero E, Capone F, Accardo M, Sorice A, Costantini M, Colonna G, et al. GPX4 and GPX7 over-expression in human hepatocellular carcinoma tissues. Eur J Histochem. (2015) 59:2540. doi: 10.4081/ejh.2015.2540
35. Li C, Zhou S, Chen C, Zhu L, Li S, Song Z, et al. DDTC-Cu(I) based metal-organic framework (MOF) for targeted melanoma therapy by inducing SLC7A11/GPX4-mediated ferroptosis. Colloids Surf B Biointerf. (2023) 225:113253. doi: 10.1016/j.colsurfb.2023.113253
36. Lee J, Roh JL. SLC7A11 as a gateway of metabolic perturbation and ferroptosis vulnerability in cancer. Antioxidants (Basel). (2022) 11(12):2444. doi: 10.3390/antiox11122444
37. Koppula P, Lei G, Zhang Y, Yan Y, Mao C, Kondiparthi L, et al. A targetable CoQ-FSP1 axis drives ferroptosis- and radiation-resistance in KEAP1 inactive lung cancers. Nat Commun. (2022) 13:2206. doi: 10.1038/s41467-022-29905-1
38. Dai E, Zhang W, Cong D, Kang R, Wang J, Tang D. AIFM2 blocks ferroptosis independent of ubiquinol metabolism. Biochem Biophys Res Commun. (2020) 523:966–71. doi: 10.1016/j.bbrc.2020.01.066
39. Lu J, Zhao Y, Liu M, Lu J, Guan S. Toward improved human health: Nrf2 plays a critical role in regulating ferroptosis. Food Funct. (2021) 12:9583–606. doi: 10.1039/D1FO01036K
40. Anandhan A, Dodson M, Schmidlin CJ, Liu P, Zhang DD. Breakdown of an ironclad defense system: the critical role of NRF2 in mediating ferroptosis. Cell Chem Biol. (2020) 27:436–47. doi: 10.1016/j.chembiol.2020.03.011
41. Li X, Chen J, Yuan S, Zhuang X, Qiao T. Activation of the P62-keap1-NRF2 pathway protects against ferroptosis in radiation-induced lung injury. Oxid Med Cell Longev. (2022) 2022:8973509. doi: 10.1155/2022/8973509
42. Amos A, Amos A, Wu L, Xia H. The Warburg effect modulates DHODH role in ferroptosis: a review. Cell Commun Signal. (2023) 21:100. doi: 10.1186/s12964-022-01025-9
43. Li X, Yu Q, Zhao R, Guo X, Liu C, Zhang K, et al. Designer exosomes for targeted delivery of a novel therapeutic cargo to enhance sorafenib-mediated ferroptosis in hepatocellular carcinoma. Front Oncol. (2022) 12:898156. doi: 10.3389/fonc.2022.898156
44. Vasquez-Vivar J, Shi Z, Tan S. Tetrahydrobiopterin in cell function and death mechanisms. Antioxid Redox Signal. (2022) 37:171–83. doi: 10.1089/ars.2021.0136
45. Liu M, Kong XY, Yao Y, Wang XA, Yang W, Wu H, et al. The critical role and molecular mechanisms of ferroptosis in antioxidant systems: a narrative review. Ann Transl Med. (2022) 10:368. doi: 10.21037/atm-21-6942
46. Kraft VAN, Bezjian CT, Pfeiffer S, Ringelstetter L, Muller C, Zandkarimi F, et al. GTP cyclohydrolase 1/tetrahydrobiopterin counteract ferroptosis through lipid remodeling. ACS Cent Sci. (2020) 6:41–53. doi: 10.1021/acscentsci.9b01063
47. Jiang L, Kon N, Li T, Wang SJ, Su T, Hibshoosh H, et al. Ferroptosis as a p53-mediated activity during tumour suppression. Nature. (2015) 520:57–62. doi: 10.1038/nature14344
48. Ou Y, Wang SJ, Li D, Chu B, Gu W. Activation of SAT1 engages polyamine metabolism with p53-mediated ferroptotic responses. Proc Natl Acad Sci U.S.A. (2016) 113:E6806–E12. doi: 10.1073/pnas.1607152113
49. Liu J, Zhang C, Lin M, Zhu W, Liang Y, Hong X, et al. Glutaminase 2 negatively regulates the PI3K/AKT signaling and shows tumor suppression activity in human hepatocellular carcinoma. Oncotarget. (2014) 5:2635–47. doi: 10.18632/oncotarget.1862
50. Zhang Y, Qian Y, Zhang J, Yan W, Jung YS, Chen M, et al. Ferredoxin reductase is critical for p53-dependent tumor suppression via iron regulatory protein 2. Genes Dev. (2017) 31:1243–56. doi: 10.1101/gad.299388.117
51. Louandre C, Marcq I, Bouhlal H, Lachaier E, Godin C, Saidak Z, et al. The retinoblastoma (Rb) protein regulates ferroptosis induced by sorafenib in human hepatocellular carcinoma cells. Cancer Lett. (2015) 356:971–7. doi: 10.1016/j.canlet.2014.11.014
52. Sun J, Zhou C, Zhao Y, Zhang X, Chen W, Zhou Q, et al. Quiescin sulfhydryl oxidase 1 promotes sorafenib-induced ferroptosis in hepatocellular carcinoma by driving EGFR endosomal trafficking and inhibiting NRF2 activation. Redox Biol. (2021) 41:101942. doi: 10.1016/j.redox.2021.101942
53. Gao R, Kalathur RKR, Coto-Llerena M, Ercan C, Buechel D, Shuang S, et al. YAP/TAZ and ATF4 drive resistance to Sorafenib in hepatocellular carcinoma by preventing ferroptosis. EMBO Mol Med. (2021) 13:e14351. doi: 10.15252/emmm.202114351
54. Chen Y, Li L, Lan J, Cui Y, Rao X, Zhao J, et al. CRISPR screens uncover protective effect of PSTK as a regulator of chemotherapy-induced ferroptosis in hepatocellular carcinoma. Mol Cancer. (2022) 21:11. doi: 10.1186/s12943-021-01466-9
55. Yuan D, Yang X, Guo JC. A great honor and a huge challenge for China: You-you TU getting the Nobel Prize in Physiology or Medicine. J Zhejiang Univ Sci B. (2016) 17:405–8. doi: 10.1631/jzus.B1600094
56. Tu Y. Artemisinin-A gift from traditional chinese medicine to the world (Nobel lecture). Angew Chem Int Ed Engl. (2016) 55:10210–26. doi: 10.1002/anie.201601967
57. Zhu S, Yu Q, Huo C, Li Y, He L, Ran B, et al. Ferroptosis: A novel mechanism of artemisinin and its derivatives in cancer therapy. Curr Med Chem. (2021) 28:329–45. doi: 10.2174/0929867327666200121124404
58. Hu Y, Guo N, Yang T, Yan J, Wang W, Li X. The potential mechanisms by which artemisinin and its derivatives induce ferroptosis in the treatment of cancer. Oxid Med Cell Longev. (2022) 2022:1458143. doi: 10.1155/2022/1458143
59. Deeken JF, Wang H, Hartley M, Cheema AK, Smaglo B, Hwang JJ, et al. A phase I study of intravenous artesunate in patients with advanced solid tumor Malignancies. Cancer Chemother Pharmacol. (2018) 81:587–96. doi: 10.1007/s00280-018-3533-8
60. Lin PL, Tang HH, Wu SY, Shaw NS, Su CL. Saponin formosanin C-induced ferritinophagy and ferroptosis in human hepatocellular carcinoma cells. Antioxidants (Basel). (2020) 9(8):682. doi: 10.3390/antiox9080682
61. Hirata Y, Oka K, Yamamoto S, Watanabe H, Oh-Hashi K, Hirayama T, et al. Haloperidol prevents oxytosis/ferroptosis by targeting lysosomal ferrous ions in a manner independent of dopamine D2 and sigma-1 receptors. ACS Chem Neurosci. (2022) 13:2719–27. doi: 10.1021/acschemneuro.2c00398
62. Lippmann J, Petri K, Fulda S, Liese J. Redox modulation and induction of ferroptosis as a new therapeutic strategy in hepatocellular carcinoma. Transl Oncol. (2020) 13:100785. doi: 10.1016/j.tranon.2020.100785
63. Iseda N, Itoh S, Toshida K, Tomiyama T, Morinaga A, Shimokawa M, et al. Ferroptosis is induced by lenvatinib through fibroblast growth factor receptor-4 inhibition in hepatocellular carcinoma. Cancer Sci. (2022) 113:2272–87. doi: 10.1111/cas.15378
64. Zheng C, Zhang B, Li Y, Liu K, Wei W, Liang S, et al. Donafenib and GSK-J4 synergistically induce ferroptosis in liver cancer by upregulating HMOX1 expression. Adv Sci (Weinh). (2023) 10:e2206798. doi: 10.1002/advs.202206798
65. Qin S, Bi F, Gu S, Bai Y, Chen Z, Wang Z, et al. Donafenib versus sorafenib in first-line treatment of unresectable or metastatic hepatocellular carcinoma: A randomized, open-label, parallel-controlled phase II-III trial. J Clin Oncol. (2021) 39:3002–11. doi: 10.1200/JCO.21.00163
66. Bian Z, Sun X, Liu L, Qin Y, Zhang Q, Liu H, et al. Sodium butyrate induces CRC cell ferroptosis via the CD44/SLC7A11 pathway and exhibits a synergistic therapeutic effect with erastin. Cancers (Basel). (2023) 15(2):423. doi: 10.3390/cancers15020423
67. Tsilimigras DI, Ntanasis-Stathopoulos I, Moris D, Spartalis E, Pawlik TM. Histone deacetylase inhibitors in hepatocellular carcinoma: A therapeutic perspective. Surg Oncol. (2018) 27:611–18. doi: 10.1016/j.suronc.2018.07.015
68. Ren X, Li Y, Zhou Y, Hu W, Yang C, Jing Q, et al. Overcoming the compensatory elevation of NRF2 renders hepatocellular carcinoma cells more vulnerable to disulfiram/copper-induced ferroptosis. Redox Biol. (2021) 46:102122. doi: 10.1016/j.redox.2021.102122
69. Ye LF, Chaudhary KR, Zandkarimi F, Harken AD, Kinslow CJ, Upadhyayula PS, et al. Radiation-induced lipid peroxidation triggers ferroptosis and synergizes with ferroptosis inducers. ACS Chem Biol. (2020) 15:469–84. doi: 10.1021/acschembio.9b00939
70. Lei G, Zhang Y, Koppula P, Liu X, Zhang J, Lin SH, et al. The role of ferroptosis in ionizing radiation-induced cell death and tumor suppression. Cell Res. (2020) 30:146–62. doi: 10.1038/s41422-019-0263-3
71. Chen Q, Zheng W, Guan J, Liu H, Dan Y, Zhu L, et al. SOCS2-enhanced ubiquitination of SLC7A11 promotes ferroptosis and radiosensitization in hepatocellular carcinoma. Cell Death Differ. (2023) 30:137–51. doi: 10.1038/s41418-022-01051-7
72. Yuan Y, Cao W, Zhou H, Qian H, Wang H CLTRN. Regulated by NRF1/RAN/DLD protein complex, enhances radiation sensitivity of hepatocellular carcinoma cells through ferroptosis pathway. Int J Radiat Oncol Biol Phys. (2021) 110:859–71. doi: 10.1016/j.ijrobp.2020.12.062
73. Yang M, Wu X, Hu J, Wang Y, Wang Y, Zhang L, et al. COMMD10 inhibits HIF1alpha/CP loop to enhance ferroptosis and radiosensitivity by disrupting Cu-Fe balance in hepatocellular carcinoma. J Hepatol. (2022) 76:1138–50. doi: 10.1016/j.jhep.2022.01.009
74. Shi L, Liu Y, Li M, Luo Z. Emerging roles of ferroptosis in the tumor immune landscape: from danger signals to anti-tumor immunity. FEBS J. (2022) 289:3655–65. doi: 10.1111/febs.16034
75. Xu C, Sun S, Johnson T, Qi R, Zhang S, Zhang J, et al. The glutathione peroxidase Gpx4 prevents lipid peroxidation and ferroptosis to sustain Treg cell activation and suppression of antitumor immunity. Cell Rep. (2021) 35:109235. doi: 10.1016/j.celrep.2021.109235
76. Wang W, Green M, Choi JE, Gijon M, Kennedy PD, Johnson JK, et al. CD8(+) T cells regulate tumour ferroptosis during cancer immunotherapy. Nature. (2019) 569:270–74. doi: 10.1038/s41586-019-1170-y
77. Liao P, Wang W, Wang W, Kryczek I, Li X, Bian Y, et al. CD8(+) T cells and fatty acids orchestrate tumor ferroptosis and immunity via ACSL4. Cancer Cell. (2022) 40:365–78. doi: 10.1016/j.ccell.2022.02.003
78. Jiang Z, Lim SO, Yan M, Hsu JL, Yao J, Wei Y, et al. TYRO3 induces anti-PD-1/PD-L1 therapy resistance by limiting innate immunity and tumoral ferroptosis. J Clin Invest. (2021) 131(8):e139434. doi: 10.1172/JCI139434
79. Kalathil S, Lugade AA, Miller A, Iyer R, Thanavala Y. Higher frequencies of GARP(+)CTLA-4(+)Foxp3(+) T regulatory cells and myeloid-derived suppressor cells in hepatocellular carcinoma patients are associated with impaired T-cell functionality. Cancer Res. (2013) 73:2435–44. doi: 10.1158/0008-5472.CAN-12-3381
80. Yang Y, Wang Y, Guo L, Gao W, Tang TL, Yan M. Interaction between macrophages and ferroptosis. Cell Death Dis. (2022) 13:355. doi: 10.1038/s41419-022-04775-z
81. Kapralov AA, Yang Q, Dar HH, Tyurina YY, Anthonymuthu TS, Kim R, et al. Redox lipid reprogramming commands susceptibility of macrophages and microglia to ferroptotic death. Nat Chem Biol. (2020) 16:278–90. doi: 10.1038/s41589-019-0462-8
82. Hao X, Zheng Z, Liu H, Zhang Y, Kang J, Kong X, et al. Inhibition of APOC1 promotes the transformation of M2 into M1 macrophages via the ferroptosis pathway and enhances anti-PD1 immunotherapy in hepatocellular carcinoma based on single-cell RNA sequencing. Redox Biol. (2022) 56:102463. doi: 10.1016/j.redox.2022.102463
83. Kim R, Hashimoto A, Markosyan N, Tyurin VA, Tyurina YY, Kar G, et al. Ferroptosis of tumour neutrophils causes immune suppression in cancer. Nature. (2022) 612:338–46. doi: 10.1038/s41586-022-05443-0
84. Zhu H, Klement JD, Lu C, Redd PS, Yang D, Smith AD, et al. Asah2 represses the p53-hmox1 axis to protect myeloid-derived suppressor cells from ferroptosis. J Immunol. (2021) 206:1395–404. doi: 10.4049/jimmunol.2000500
85. Poznanski SM, Singh K, Ritchie TM, Aguiar JA, Fan IY, Portillo AL, et al. Metabolic flexibility determines human NK cell functional fate in the tumor microenvironment. Cell Metab. (2021) 33:1205–20 e5. doi: 10.1016/j.cmet.2021.03.023
86. Chen Q, Liu L, Ni S. Screening of ferroptosis-related genes in sepsis-induced liver failure and analysis of immune correlation. PeerJ. (2022) 10:e13757. doi: 10.7717/peerj.13757
87. Xu T, Ma Y, Yuan Q, Hu H, Hu X, Qian Z, et al. Enhanced ferroptosis by oxygen-boosted phototherapy based on a 2-in-1 nanoplatform of ferrous hemoglobin for tumor synergistic therapy. ACS Nano. (2020) 14:3414–25. doi: 10.1021/acsnano.9b09426
88. Yao L, Zhao MM, Luo QW, Zhang YC, Liu TT, Yang Z, et al. Carbon quantum dots-based nanozyme from coffee induces cancer cell ferroptosis to activate antitumor immunity. ACS Nano. (2022) 16:9228–39. doi: 10.1021/acsnano.2c01619
89. Zheng Y, Wang Y, Lu Z, Wan J, Jiang L, Song D, et al. PGAM1 inhibition promotes HCC ferroptosis and synergizes with anti-PD-1 immunotherapy. Adv Sci (Weinh). (2023) 10:e2301928. doi: 10.1002/advs.202301928
90. Conche C, Finkelmeier F, Pesic M, Nicolas AM, Bottger TW, Kennel KB, et al. Combining ferroptosis induction with MDSC blockade renders primary tumours and metastases in liver sensitive to immune checkpoint blockade. Gut. (2023) 72:1774–82. doi: 10.1136/gutjnl-2022-327909
91. Li Y, Yang W, Zheng Y, Dai W, Ji J, Wu L, et al. Targeting fatty acid synthase modulates sensitivity of hepatocellular carcinoma to sorafenib via ferroptosis. J Exp Clin Cancer Res. (2023) 42:6. doi: 10.1186/s13046-022-02567-z
92. Huang D, Xu D, Chen W, Wu R, Wen Y, Liu A, et al. Fe-MnO(2) nanosheets loading dihydroartemisinin for ferroptosis and immunotherapy. BioMed Pharmacother. (2023) 16:1114431. doi: 10.1016/j.biopha.2023.114431
93. Deng H, Zhang J, Zheng Y, Li J, Xiao Q, Wei F, et al. Corrigendum: CCDC25 may be a potential diagnostic and prognostic marker of hepatocellular carcinoma: Results from microarray analysis. Front Surg. (2023) 10:1175721. doi: 10.3389/fsurg.2023.1175721
94. Yan YC, Meng GX, Ding ZN, Liu YF, Chen ZQ, Yan LJ, et al. Somatic mutation and expression of BAP1 in hepatocellular carcinoma: an indicator for ferroptosis and immune checkpoint inhibitor therapies. J Cancer. (2022) 13:88–101. doi: 10.7150/jca.65574
Keywords: ferroptosis, hepatocellular carcinoma, lipid peroxidation, drugs, radiotherapy, immunotherapy
Citation: Jiang S, Zhang G, Ma Y, Wu D, Xie D, Zhou S and Jiang X (2024) Ferroptosis in hepatocellular carcinoma, from mechanism to effect. Front. Oncol. 14:1350011. doi: 10.3389/fonc.2024.1350011
Received: 09 January 2024; Accepted: 21 February 2024;
Published: 05 March 2024.
Edited by:
Haiwei Mou, Wistar Institute, United StatesReviewed by:
Zilong Zhao, University of Texas MD Anderson Cancer Center, United StatesHaiyin Li, Wistar Institute, United States
Jinghui Liang, Wistar Institute, United States
Xueqin Xie, Memorial Sloan Kettering Cancer Center, United States
Copyright © 2024 Jiang, Zhang, Ma, Wu, Xie, Zhou and Jiang. This is an open-access article distributed under the terms of the Creative Commons Attribution License (CC BY). The use, distribution or reproduction in other forums is permitted, provided the original author(s) and the copyright owner(s) are credited and that the original publication in this journal is cited, in accordance with accepted academic practice. No use, distribution or reproduction is permitted which does not comply with these terms.
*Correspondence: Xuemei Jiang, jiangxuemei@hainmc.edu.cn