- 1Department of Epidemiology, School of Population Health, Virginia Commonwealth University, Richmond, VA, United States
- 2Department of Epidemiology and Biostatistics, Arnold School of Public Health, University of South Carolina, Columbia, SC, United States
- 3Palmetto GBA, Columbia, SC, United States
- 4Division of Epidemiology, Biostatistics, and Environmental Health, School of Public Health, University of Memphis, Memphis, TN, United States
- 5Alabama College of Osteopathic Medicine, Dothan, AL, United States
- 6Department of Biobehavioral Health and Nursing Science, College of Nursing, University of South Carolina, Columbia, SC, United States
- 7South Carolina Statewide Cancer Prevention & Control Program, University of South Carolina, Columbia, SC, United States
Introduction: Sleep disruption affects biological processes that facilitate carcinogenesis. This retrospective cohort study used de-identified data from the Veterans Administration (VA) electronic medical record system to test the hypothesis that patients with diagnosed sleep disorders had an increased risk of prostate, breast, colorectal, or other cancers (1999-2010, N=663,869). This study builds upon existing evidence by examining whether patients with more severe or longer-duration diagnoses were at a greater risk of these cancers relative to those with a less severe or shorter duration sleep disorder.
Methods: Incident cancer cases were identified in the VA Tumor Registry and sleep disorders were defined by International Classification of Sleep Disorder codes. Analyses were performed using extended Cox regression with sleep disorder diagnosis as a time-varying covariate.
Results: Sleep disorders were present among 56,055 eligible patients (8% of the study population); sleep apnea (46%) and insomnia (40%) were the most common diagnoses. There were 18,181 cancer diagnoses (41% prostate, 12% colorectal, 1% female breast, 46% other). The hazard ratio (HR) for a cancer diagnosis was 1.45 (95% confidence interval [CI]: 1.37, 1.54) among those with any sleep disorder, after adjustment for age, sex, state of residence, and marital status. Risks increased with increasing sleep disorder duration (short [<1-2 years] HR: 1.04 [CI: 1.03-1.06], medium [>2-5 years] 1.23 [1.16-1.32]; long [>5-12 years] 1.52 [1.34-1.73]). Risks also increased with increasing sleep disorder severity using cumulative sleep disorder treatments as a surrogate exposure; African Americans with more severe disorders had greater risks relative to those with fewer treatments and other race groups. Results among patients with only sleep apnea, insomnia, or another sleep disorder were similar to those for all sleep disorders combined.
Discussion: The findings are consistent with other studies indicating that sleep disruption is a cancer risk factor. Optimal sleep and appropriate sleep disorder management are modifiable risk factors that may facilitate cancer prevention.
Introduction
Sleep disorders arise due to complex circumstances that can include genetic, environmental, behavioral, and psychosocial risk factors (1, 2). Veterans comprise a vulnerable population for sleep disorder occurrence and their rates are elevated relative to the general population (3, 4). Furthermore, secular trends in sleep disorder diagnoses have been increasing, both among Veterans and within the general population (3–5). For example, a national study of sleep disorder diagnoses among Veterans from 2000 to 2010 found a ~6-fold increase in sleep disorder prevalence during that period, with the largest increases among those with combat experience or post-traumatic stress disorder (3). Because of these increasing trends, an association between sleep disorders and cancer incidence would have important public health implications.
Several pathophysiological processes may mediate the association between chronic sleep disruption and carcinogenesis, including pathological changes in immune (via inflammation- and oxidative stress-related mechanisms), endocrine, neurological, metabolic and circadian systems (6–9). For example, the intermittent hypoxia and subsequent oxidative stress that occurs with sleep apnea has been cited as a biologically plausible, experimentally supported mechanism (7, 9). Results from longitudinal studies of sleep disorders and cancer incidence tend to show increased risks although results have been inconsistent. For example, several investigators reported no association between insomnia symptoms and prostate cancer (PrCA) incidence (10–12), whereas others report higher risks for PrCA among insomniacs after ~10 or more years of follow-up (13). Insomnia has also been associated with increased risks for breast (BrCA), colorectal (CRC) or other cancer types, particularly after a decade or more of follow-up (14–16). Some studies among sleep apnics reported an elevated incidence of BrCA, PrCA, CRC (17–21) whereas other studies of patients with obstructive sleep apnea (OSA) reported lower risks (22–24). A national matched retrospective cohort among Veterans found that OSA was associated with an increased incidence of BrCA, PrCA, CRC and several other cancers after a median follow-up of 7.4 years (25). It has been suggested that cancer risk increases with OSA severity (17, 26, 27). However, few studies, if any, have examined the role of cumulative sleep-related treatments or duration of diagnosis as proxies for dose-response.
Several studies that assessed sleep either subjectively (sleep quality) or quantitatively (nightly sleep duration) reported no association with cancer incidence (28–31). However, studies among women in Singapore (32) and Ohsaki, Japan (33) both found a modest association between postmenopausal BrCA risk and long sleep duration within a 24-hour period, consistent with a USA study where long sleep (≥9 hours per night) was associated with a modest increase in BrCA risk, although short nightly sleep duration had no association (34). Among men in the Ohsaki cohort, those who reported trouble falling asleep or staying asleep had increased PrCA risks (35) whereas men who slept ≥9 hours per night had lower PrCA risks relative to those with normal sleep (33). These examples indicate that, despite decades of research among millions of patients, there are still inconsistencies and uncertainties among studies examining relationships between sleep disturbances and cancer risk. Recent systematic reviews and meta-analyses addressing this issue highlight heterogeneous sleep assessment methods as one possible explanation, and indicate a need for more studies using clinically diagnosed sleep disorders (8, 16, 17, 31, 36–38).
Racial disparities in sleep disturbances have also been described. African Americans (AAs) have shorter sleep duration, lower sleep efficiency, and unfavorable sleep stages including less slow wave sleep and worse sleep continuity relative to European Americans (EAs) (39, 40). AAs also have an elevated incidence of certain cancers relative to EAs, both in the general (colorectal, gastric, lung, renal, hepatic, prostate) and Veteran (gastric, hepatic, prostate) population (41, 42). However, few studies have examined the extent to which sleep disparities may contribute to racial cancer disparities (40). The VA’s electronic medical record (EMR) system includes data for millions of Veterans and serves as a valuable resource for examining relationships between sleep disorders and cancer incidence.
Methods
This retrospective cohort study used data from the VA EMR to test the hypothesis that Veterans in the southeastern Veterans Integrated Service Network 7 (VISN-7, includes AL, GA, SC) with a diagnosed sleep disorder had increased cancer risk relative to those without a sleep disorder. Analyses were performed to evaluate whether the duration or severity of the sleep disorder diagnosis enhanced risk, and whether cancer risk was modified by race. Following regulatory approvals, electronic medical records for patients seeking care at least once at a VISN-7 facility between January, 1999 and July, 2010 were retrieved from MedSAS Dataset and Department of Veterans Affairs (VA) Corporate Data Warehouse files. During the study period, the VISN-7 included 9 tertiary care medical centers, 14 community-based outpatient clinics, and 18 primary care clinics serving ~1.3 million Veteran patients, and it was sixth largest among VISNs nationally for percentage of patients in chronic care. Patients younger than 18 years old and those without age information were excluded. Data elements were linked via social security number by a VA data manager, and then scrambled and replaced with a unique patient identification number so that data used by the study investigators were de-identified.
Sleep disorder cases were defined as patients with at least two occurrences of a diagnosis ≥30 days apart based on American Academy of Sleep Medicine International Classification of Sleep Disorder (ICSD) categories (43). The first occurrence of the in- or out-patient diagnosis was used to define date of diagnosis for: sleep disturbances (ICD-9 780.50-59), nonorganic sleep disorders (ICD-9 307.40-49), organic insomnia (ICD-9 327.00-09), organic hypersomnia (ICD-9 327.10-19), organic sleep apnea (ICD-9 327.20-29), and circadian rhythm sleep disorders (ICD-9 327.30-39). Time since diagnosis was calculated by summing the number of months since initial sleep disorder diagnosis and was used in the statistical analyses either as a continuous variable or categorized into three groups based on tertiles defining short, medium and long duration of diagnosis (<1-2.2, 2.3-5.2, 5.3-12.4 years, respectively). Sleep disorder severity was approximated as the cumulative number of treatments by summing sleep-related prescriptions, clinical procedures and surgeries into a single continuous variable. Participants were then grouped into four categories: those without any treatment, and those with few (1 treatment/prescription), moderate (cumulative count: 2-18) or frequent (19 - 1,150) treatments.
Data from the VA Tumor Registry were accessed to identify patients with an incident, primary tumor of the: prostate (ICD-9 185), female breast (ICD-9 174), colorectum (ICD-9 153-4), lung (ICD-9 162), pancreas (ICD-9 157) kidney (ICD-9 189), brain (ICD-9 191), bladder (ICD-9 188), liver (ICD-9 155), ovary (ICD-9 183), esophagus (ICD-9 150), or stomach (ICD-9 151). Patients with a cancer diagnosis prior to the beginning of the study, and those with in situ (ICD-9 230-234) and benign (ICD-9 210-229) tumors were excluded. Patients with a rare cancer or an etiology unrelated to the study hypothesis (e.g., infectious) were excluded, which included tumors of the: small intestine (ICD-9 152), skin (ICD-9 172-173), uterus (ICD-9 179 and 182), cervix (ICD-9 180), thyroid (ICD-9 193), lips, oral cavity, pharynx (ICD-9 140-149), or a lymphoma (ICD-9 201). Demographic variables used in the analyses as covariates included: age at cohort entry (18-34 yrs, 35-44 yrs, 45-54 yrs, 55-64 yrs, ≥65 yrs), sex, marital status, and state of residence (AL, GA, SC). Prior to the creating the final analytic data set, Veteran Medicare data were merged by a VA data manager using the social security number to assign race (EA, AA, and Other/Unknown) for each patient based on VA Information Resource Center (VIReC) guidelines (44, 45).
Statistical analyses examining the relationship between sleep disorders and subsequent cancer diagnoses were conducted using extended Cox regression models (SAS 9.3, SAS Institute, Inc, Cary, NC). This method included sleep disorder diagnosis as a time-varying covariate and is applied when the proportional hazards assumption is not met (46, 47). Survival time within the cohort was defined as months from entry until cancer diagnosis or censoring. Patients with only one visit at a VISN-7 facility were censored at the time of entry into the cohort. Hazard ratios with corresponding 95% confidence intervals for each combination of the sleep disorder variable and cancer outcome were computed for both crude and adjusted (age, sex, marital status, state of residence) models with statistical significance set at alpha = 0.05. Initial analyses evaluated all sleep disorders combined and subsequent analyses were performed for the following subgroups: insomnia, sleep apnea, and all other sleep disorders. Analyses of sleep disorder duration were used to assess the latency of tumor development following a sleep disorder diagnosis, and also to address the potential for reverse causality. A time-varying model was implemented where exposure (i.e., sleep disorder diagnosis) was assessed at each failure point in the model, with the amount of time since diagnosis included as a continuous variable in the Cox extended hazard analysis (47). A sleep disorder diagnosis is not always required for a given sleep disorder treatment (i.e., some prescriptions or clinical procedures can be used to treat conditions other than a sleep disorder, or can be used for patients without a sleep disorder diagnosis). Therefore, to prevent the introduction of bias, cumulative sleep-related treatments were computed for all participants regardless of their sleep disorder status, and a Cox extended regression model was used in which the cumulative treatment was multiplied by the total time in the study to account for the lack of proportionality. Additional analyses focused on potential effect modification by race, which was assessed by including an interaction term between race and each sleep disorder variable used in the model, and results were presented as hazard ratios and 95% confidence intervals within each race stratum. In some instances, the stage of disease was missing from the tumor registry. To evaluate the potential introduction of bias, sensitivity analyses included an indicator variable (‘1’ for those without cancer stage and ‘0’ for others) with the Cox extended regression models. Those analyses produced the same conclusions as the results presented below.
Results
The final study population included 663,869 eligible patients after applying inclusion and exclusion criteria (Figure 1). At baseline, the study population was 88% male, 45% EA, 26% AA, 29% Other/Unknown, and 67% were ≥45 years old (Table 1). There were 56,055 patients with sleep disorders (8.4% of the study population); sleep apnea and insomnia were most common (46% and 40% of all sleep disorders, respectively). Sleep disorder patients were more likely to be African American (30% vs. 26%), male (92% vs. 88%) and divorced (19% vs. 8%) compared to those without a sleep disorder (Table 1). The average ( ± SD) duration of a sleep disorder diagnosis was 7.2 ± 3.1 years. Patients with a sleep disorder had an average of 66 ± 98 sleep-related treatments, whereas those without a sleep disorder had an average of 21 ± 56 treatments. The average follow-up time among all patients in the cohort was 10.8 ± 2.5 years. There were 18,181 cancer cases diagnosed during the study period (2.7% of the study population), and the average age at diagnosis was 64 ± 10 years.
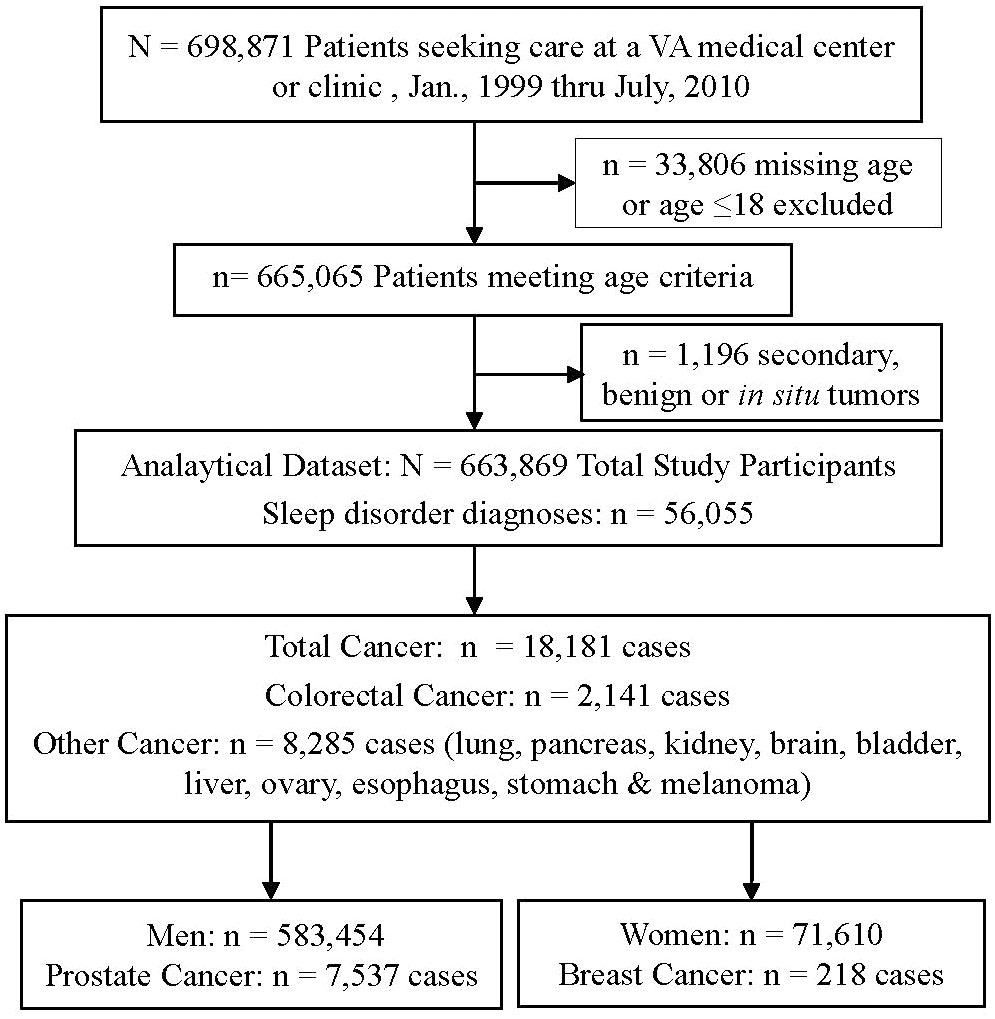
Figure 1 Flow diagram of study population after applying inclusion and exclusion criteria. Patients in the Department of Veterans Affairs (VA) Southeast United States Service Network (VISN-7). Sleep disorder and cancer diagnoses were obtained through the VA’s Corporate Data Warehouse and Tumor Registry, respectively.
Crude and adjusted HRs for cancer incidence in relation to sleep disorder diagnoses are presented in Table 2. Patients with any sleep disorder diagnosis, sleep apnea, insomnia, or other types of sleep disorders had increased risks for PrCA, all hypothesized cancers, and other selected cancers (lung, pancreatic, kidney, brain, bladder, liver, ovarian, esophageal, gastric, melanoma). Adjusted HRs for CRC were elevated among those with any sleep disorder or with sleep apnea, whereas there were no statistically significant HRs for female BrCA for any of the sleep disorders (Table 2).
When the duration of time since diagnosis was evaluated, patients with any sleep disorder had statistically significant increased HRs for all, PrCA, CRC, and for other cancers, with adjusted HRs increasing as the time after initial diagnosis increased (Table 3). The results for female BrCA did not indicate a statistically significant increase in cancer risk related to the duration of any sleep disorder (Table 3). The results were similar when the data were grouped by those with only insomnia, sleep apnea, or other sleep disorders (results not shown). When cumulative sleep-related treatments were evaluated, statistically significant increases in cancer risk were observed for each type of cancer that was assessed (including BrCA), and HRs increased with an increasing number of prescriptions and clinical procedures (Table 4).
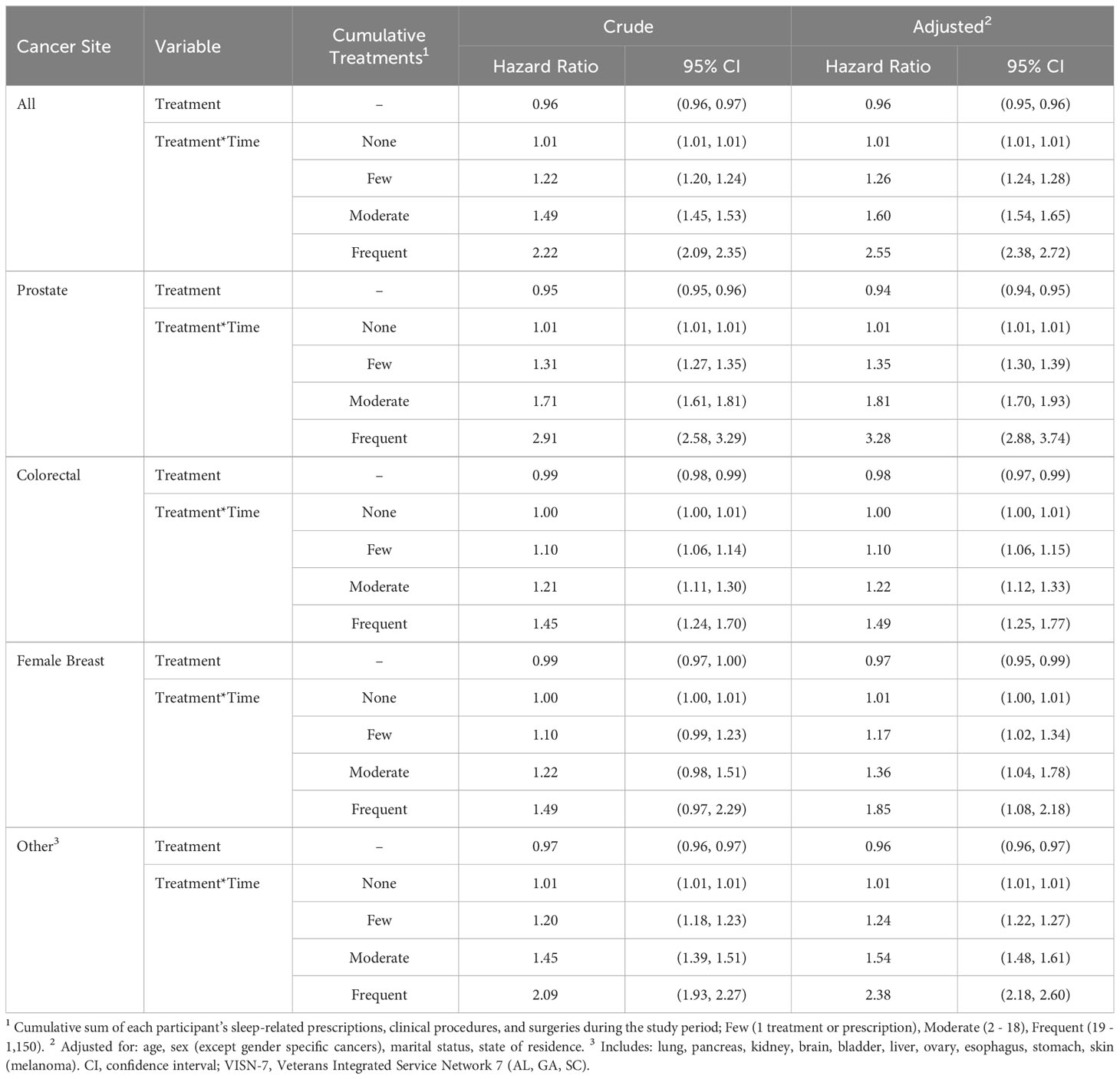
Table 4 Cumulative sleep-related treatments and cancer incidence among veterans (N=663,869, 1999-2010, VISN-7).
When effect modification by race was examined, few differences were noted among race groups for the main analyses that evaluated the risk of a sleep disorder diagnosis (Supplementary Table S1A-D), or for analyses that assessed the duration of sleep disorder diagnoses (Supplementary Table S2). However, when cumulative sleep-related treatments were stratified by race, cancer risk among AAs was elevated relative to EAs, particularly for all cancer, PrCA, CRC, and Other cancers, and among patients in the ‘Frequent’ cumulative treatment category (Supplementary Table S3). For female BrCA, the trends were similar but there were no statistically significant HRs. Results among those in the Other/Unknown race category tended to be intermediate between the AA and EA groups (Supplementary Table S3).
Discussion
In this retrospective cohort study among 663,869 Veterans in the southeastern USA, patients with a sleep disorder diagnosis had increased cancer risks over an average of 11 ± 2.5 years of follow-up. For those with any sleep disorder, the HR was greatest, though imprecise, for BrCA 1.69 (0.88, 3.24), and was lowest for CRC 1.34 (1.16, 1.54). The results are generally consistent with other studies of cancer incidence in populations with sleep disorders, including studies among patients with moderate to severe sleep apnea or insomnia (8, 16, 17, 25, 31, 36, 37). For example, a national study among Veterans with sleep apnea reported an approximate doubling of cancer risk after a median follow-up of 7.4 years (25). To the authors’ knowledge, no other study has assessed cancer risk in relation to the duration of a sleep disorder diagnosis over time, and there were incremental increases in cancer HRs with increasing sleep disorder duration for each type of cancer that was evaluated, suggesting a form of dose-response. In other studies, increased cancer risks were observed in relation to subjective or short-term quantitative sleep disruption measures, but not necessarily in those with a sleep disorder diagnosis. For example, multiple studies have evaluated nightly sleep duration in relation to cancer risk. Short duration sleep (usually defined as ≤6 hours per night) has been associated with PrCA (33), BrCA (48), CRC (49, 50), and total cancer (51), and long nightly sleep has been linked with increased risk of BrCA (34), CRC (50, 52) or other cancers relative to those with normal nightly sleep (usually 7-8 hours per night) (8, 16, 24, 36). The authors also are unaware of other research that evaluated cancer incidence in relation to cumulative sleep-related treatments as a surrogate for disease severity. In a retrospective cohort study (N=33,711), OSA severity and nocturnal hypoxemia were each associated with an increased incidence of cancer of any type (15% and 30% increased risk, respectively) over a median of seven years follow-up (26). In a recent meta-analysis, a monotonic increase in cancer incidence of any type was observed with progressive increases in OSA severity based on apnea-hypopnea indices, with pooled risk estimates ranging from 1.14 (mild), to 1.36 (moderate) and 1.59 (severe) (27). Results in the current study are similar to these observations. Incremental increases in HRs were observed for each of the cancers evaluated as cumulative sleep-related treatments increased relative to those without such treatment. This may also indicate dose-response although other interpretations are possible. A greater number of treatments may suggest an iatrogenic effect of increased cancer risk among those with extended sedative or hypnotic prescription medication use (53), or it may reflect different behaviors, such as heightened health care vigilance or an increased probability of tumor detection by a clinician.
Regardless of the interpretation, cancer risks among those with more sleep-related treatments were greatest among AAs. These results should be interpreted cautiously because evidence for effect modification by race was seen only in analyses of sleep disorder severity, and because all Veterans should have equal access to care. Nonetheless, cancer surveillance data indicate that Veteran AAs have elevated rates of gastric, hepatic, and PrCA incidence relative to EAs (41, 42), which merits further investigation. Racial cancer disparities arise from complex biological, social, and behavioral factors that can include discrimination and stress (39, 40, 54–58). AAs may differ in their endogenous circadian timing relative to EAs (59, 60), and they are more likely than EAs to have poor sleep (39, 40), to participate in shiftwork (61, 62), and to have excessive stress (allostatic overload or ‘weathering’) (54, 63). The autonomic nervous system (ANS) is coupled to sleep and other circadian processes (64–66) as well as the stress response (57, 63, 65, 67, 68). Sleep disturbances elicit stress and chronically can disrupt the ability of the ANS to modulate sympathetic nervous system activity (69, 70). A recent study reported an increased odds of metabolic syndrome, a cancer risk factor (71), among those with both poor sleep and low heart rate variability (HRV, an indicator of dysfunctional autonomic activity), relative to those with normal sleep and HRV (72). This and other evidence indicates that chronic stress leads to a pro-inflammatory state of sympathetic overactivation and ANS dysregulation (73, 74) that can promote tumorigenesis (73–77). These pathophysiological processes may underlie the etiology of racial cancer disparities (57, 58, 63, 64, 68, 74).
This study had some noteworthy strengths and limitations. Strengths include a robust sample size and the use of electronic medical records to ascertain both sleep disorders and cancer diagnoses through the VA’s Corporate Data Warehouse and Tumor Registry, which enhanced validity and provided clinically meaningful exposure and outcome data. The population was restricted to patients in the VA health care system in the southeastern US region (VISN-7), which limits generalizability. The exclusion of tumors with an infectious etiology also limits the generalizability of the results among those with ‘Other Cancers’ since immune dysfunction associated with sleep disturbances and adaptive stress may impact sensitivity to virally mediated cancer progression. Veteran patients are predominantly male (89% in this study population), whereas the distribution of sex in the general population is closer to 50%. For this reason, the statistical power for analyses of BrCA risk may have been insufficient. Veterans who did not utilize a VA medical center, migrated from the study area, or transitioned out of the VA health care system were lost to follow-up, which could have introduced a selection bias. Sleep disorders can occur in heterogeneous populations and prescription use can have multiple indications. The extent to which those factors may influence cancer risk is uncertain. Also, the presence of undiagnosed sleep disorders or other sleep disturbances in the study population may have resulted in exposure misclassification that likely resulted in a bias towards the null. A major study limitation was the inability to control for several important cancer risk factors, such as body mass index, smoking, socioeconomic status, comorbid disease, and family cancer history. Additionally, there was no information on either physical activity or diet, both of which influence carcinogenesis processes including inflammation, and are associated with cancer risk (78, 79). However, a recent study among Veterans reported only modest differences between the crude and adjusted HRs for a cancer diagnosis among those with sleep apnea after adjusting for age, sex, year of cohort entry, smoking, alcohol use, obesity, and comorbid disease (crude HR: 2.16, CI: 2.13-2.20 vs. adjusted HR: 1.97, CI: 1.94-2.00) (25). Among its strengths, this study included innovative analyses of the duration of sleep disorder diagnosis and cumulative sleep-related treatments as potential indicators of dose-response. The increasing HRs with increasing duration or severity of sleep disorder reduced the possibility of confounding but does not rule it out entirely. Similarly, the potential for reverse causality was minimized as the duration of sleep disorder diagnosis or the number of sleep-related treatments increased. The results from this study were consistent when the analyses were performed only among those with sleep apnea, insomnia, or other sleep disorder diagnoses. This suggests that sleep disturbances in general may share a common mechanism of carcinogenesis.
Sleep problems tend to be chronic and persistent (80, 81), and trends in sleep disorders have been increasing both in Veterans and in the general population (3, 4). Night work is an extreme and relevant example of how chronic sleep loss can elicit pathological effects that increase cancer risk (82, 83). The weight of evidence documenting linkages between sleep disruption and cancer incidence has continued to expand (8, 16, 17, 25, 31, 36, 37), although some inconsistencies and uncertainties remain that may be due to differences among study designs, populations studied, or other methodological considerations, particularly sleep assessment validity and representativeness, and uncertainties concerning the nature of the dose-response relationship between sleep disturbances and cancer risk. Results from the current analysis support other evidence suggesting that cancer risks associated with sleep disruption may take up to a decade or more to manifest. This has clinical implications and introduces a window of opportunity for intervention. Concerns over long term sleep medication use (53, 84) suggest a need for alternatives that are safe, effective, low-cost and sustainable. One possibility is supplemental use of melatonin, a hormone derived from the essential amino acid, tryptophan, which facilitates sleep timing and other circadian processes. Multiple studies support its use for insomnia and other sleep problems, particularly in older individuals (85, 86). Melatonin also has established antiproliferative, antioxidant, immune enhancing, and anti-inflammatory properties, and evidence for its effectiveness in treating BrCA, PrCA, CRC and other cancers is promising (87, 88). However, its role in cancer prevention has not been thoroughly studied. Another promising class of compounds are used for insomnia treatment by acting upon the orexin (hypocretin) receptor system that modulates sleep/wakefulness (89). These agents selectively induce apoptosis in several tumor types and thus have promising therapeutic potential (8). Other alternative sleep disorder treatments include interventions that act upon ANS activity, such as HRV biofeedback, which is effective at improving sleep and reducing inflammation using a nonpharmacological, mechanism-driven technique (resonance frequency breathing) that can reduce sympathetic overactivation and promote ANS homeostasis (73, 74, 90, 91). Additional research is needed to further optimize the timing, frequency and intensity of these and other innovative sleep promoting, cancer prevention strategies in order to fully characterize their effectiveness and long term benefits.
In summary, the results of this study are consistent with other data indicating a link between sleep perturbations and cancer risk. Sleep disorder diagnoses and clinical treatments were examined rather than short-term measures of nightly sleep or self-reported sleep quality. Patients with a longer duration or greater severity of sleep disorder diagnosis had the greatest risks. The findings suggest that optimal sleep and appropriate sleep disorder management may represent modifiable risk factors for facilitating cancer prevention. Carefully controlled clinical trials are needed to more rigorously evaluate these possibilities.
Data availability statement
The data analyzed in this study was obtained from USA Department of Veterans Affairs (VA) Corporate Data Warehouse and MedSAS data files. Requests to access the datasets should be directed to the VA Informatics and Computing Infrastructure (VINCI) office (VINCI@VA.GOV).
Ethics statement
The study was conducted in accordance with applicable laws and regulations governing use of secondary data in human research. Data were coded to protect the identity of study subjects. This study was reviewed and approved by the Institutional Review Boards of the Columbia Veterans Affairs Health Care System (formerly WJB Dorn Department of Veterans Affairs Medical Center) and the University of South Carolina, Columbia, SC.
Author contributions
JBB: Conceptualization, Methodology, Funding acquisition, Project administration, Investigation, Data curation, Software, Formal Analysis, Validation, Writing – original draft, Writing – review & editing. AFD: Conceptualization, Methodology, Project administration, Investigation, Data curation, Software, Formal Analysis, Validation, Writing – original draft, Writing – review & editing. HZ: Conceptualization, Methodology, Investigation, Data curation, Software, Formal Analysis, Validation, Writing – review & editing. ACM: Conceptualization, Methodology, Investigation, Data curation, Software, Formal Analysis, Validation, Writing – review & editing. MAR: Investigation, Data curation, Software, Formal Analysis, Validation, Writing – review & editing. AM: Writing – original draft, Writing – review & editing. SAA: Conceptualization, Methodology, Writing – review & editing. JRH: Conceptualization, Methodology, Writing – review & editing.
Funding
The author(s) declare financial support was received for the research, authorship, and/or publication of this article. This research was supported by a Department of Veterans Affairs, Office of Research and Development, Health Services Research and Development grant (PPO 09-246, PI: Burch). JB was supported by grants from the National Cancer Institute (R01CA231321) and National Institute for Justice (2019-R2-CX-0021). JH was supported by a grant from the National Cancer Institute (U01 CA272977-01).
Acknowledgments
This work was performed at the Columbia Veterans Affairs Health Care System (formerly WJB Dorn Department of Veterans Affairs Medical Center) and the University of South Carolina, Columbia, SC. The authors gratefully acknowledge input from Daniel Kripke, MD, on the use of hypnotics and other prescription pharmaceuticals for sleep disorder treatment.
Conflict of interest
Author AD is currently employed by Palmetto GBA, LLC. When the study was conducted, she was employed by the University of South Carolina.
The remaining authors declare that the research was conducted in the absence of any commercial or financial relationships that could be construed as a potential conflict of interest.
Publisher’s note
All claims expressed in this article are solely those of the authors and do not necessarily represent those of their affiliated organizations, or those of the publisher, the editors and the reviewers. Any product that may be evaluated in this article, or claim that may be made by its manufacturer, is not guaranteed or endorsed by the publisher.
Author disclaimer
The views expressed in this article do not necessarily reflect those of the Palmetto GBA or the Center for Medicaid and Medicare Services.
Supplementary material
The Supplementary Material for this article can be found online at: https://www.frontiersin.org/articles/10.3389/fonc.2024.1336487/full#supplementary-material
Supplementary Table 1a | All Sleep Disorder Diagnoses and Cancer Incidence Stratified by Race, Veterans in the Southeast USA (1999-2010, VISN-7).
Supplementary Table 1b | Insomnia Diagnoses and Cancer Incidence Stratified by Race, Veterans in the Southeast USA (1999-2010, VISN-7).
Supplementary Table 1c | Sleep Apnea Diagnosis and Cancer Incidence stratified by Race, Veterans in the Southeast USA (1999-2010, VISN-7).
Supplementary Table 1d | Other Sleep Disorder Diagnoses and Cancer Incidence stratified by Race, Veterans in the Southeast USA (1999-2010, VISN-7).
Supplementary Table 2 | Duration of Sleep Disorder Diagnosis and Cancer Incidence Stratified by Race, Veterans in the SE USA (VISN-7, 1999-2010).
Supplementary Table 3 | Cumulative Sleep-Related Treatments and Cancer Incidence Among Veterans, Stratified by Race (VISN-7, 1999-2010).
References
1. Estes S, Tice JR. Understanding and addressing the unique challenges and conditions of the veteran: improving sleep and well-being. Nurs Clin North Am (2021) 56(2):219–27. doi: 10.1016/j.cnur.2021.03.002
2. Pavlova MK, Latreille V. Sleep disorders. Am J Med (2019) 132(3):292–9. doi: 10.1016/j.amjmed.2018.09.021
3. Alexander M, Ray MA, Hebert JR, Youngstedt SD, Zhang H, Steck SE, et al. The national veteran sleep disorder study: descriptive epidemiology and secular trends, 2000-2010. Sleep (2016) 39(7):1399–410. doi: 10.5665/sleep.5972
4. Folmer RL, Smith CJ, Boudreau EA, Hickok AW, Totten AM, Kaul B, et al. Prevalence and management of sleep disorders in the Veterans Health Administration. Sleep Med Rev (2020) 54:101358. doi: 10.1016/j.smrv.2020.101358
5. Ford ES, Wheaton AG, Cunningham TJ, Giles WH, Chapman DP, Croft JB. Trends in outpatient visits for insomnia, sleep apnea, and prescriptions for sleep medications among US adults: findings from the national ambulatory medical care survey 1999-2010. Sleep (2014) 37(8):1283–93. doi: 10.5665/sleep.3914
6. Jagielo AD, Benedict C, Spiegel D. Circadian, hormonal, and sleep rhythms: effects on cancer progression implications for treatment. Front Oncol (2023) 13:1269378. doi: 10.3389/fonc.2023.1269378
7. Almendros I, Wang Y, Becker L, Lennon FE, Zheng J, Coats BR, et al. Intermittent hypoxia-induced changes in tumor-associated macrophages and tumor Malignancy in a mouse model of sleep apnea. Am J Respir Crit Care Med (2014) 189(5):593–601. doi: 10.1164/rccm.201310-1830OC
8. Mogavero MP, DelRosso LM, Fanfulla F, Bruni O, Ferri R. Sleep disorders and cancer: State of the art and future perspectives. Sleep Med Rev (2021) 56:101409. doi: 10.1016/j.smrv.2020.101409
9. Gozal D, Farre R, Nieto FJ. Putative links between sleep apnea and cancer: from hypotheses to evolving evidence. Chest (2015) 148(5):1140–7. doi: 10.1378/chest.15-0634
10. Markt SC, Grotta A, Nyren O, Adami HO, Mucci LA, Valdimarsdottir UA, et al. Insufficient sleep and risk of prostate cancer in a large swedish cohort. Sleep (2015) 38(9):1405–10. doi: 10.5665/sleep.4978
11. Markt SC, Flynn-Evans EE, Valdimarsdottir UA, Sigurdardottir LG, Tamimi RM, Batista JL, et al. Sleep duration and disruption and prostate cancer risk: a 23-year prospective study. Cancer Epidemiol Biomarkers Prev (2016) 25(2):302–8. doi: 10.1158/1055-9965.EPI-14-1274
12. Tan X, Cedernaes J, Forsberg LA, Schioth HB, Benedict C. Self-reported sleep disturbances and prostate cancer morbidity and mortality in Swedish men: A longitudinal study over 40 years. J Sleep Res (2018) 27(6):e12708. doi: 10.1111/jsr.12708
13. Lv X, Li Y, Li R, Guan X, Li L, Li J, et al. Relationships of sleep traits with prostate cancer risk: A prospective study of 213,999 UK Biobank participants. Prostate (2022) 82(9):984–92. doi: 10.1002/pros.24345
14. Sen A, Opdahl S, Strand LB, Vatten LJ, Laugsand LE, Janszky I. Insomnia and the risk of breast cancer: the HUNT study. Psychosom Med (2017) 79(4):461–8. doi: 10.1097/PSY.0000000000000417
15. Lin CL, Liu TC, Wang YN, Chung CH, Chien WC. The association between sleep disorders and the risk of colorectal cancer in patients: A population-based nested case-control study. In Vivo (2019) 33(2):573–9. doi: 10.21873/invivo.11513
16. Shi T, Min M, Sun C, Zhang Y, Liang M, Sun Y. Does insomnia predict a high risk of cancer? A systematic review and meta-analysis of cohort studies. J Sleep Res (2020) 29(1):e12876. doi: 10.1111/jsr.12876
17. Sillah A, Watson NF, Schwartz SM, Gozal D, Phipps AI. Sleep apnea and subsequent cancer incidence. Cancer Causes Control (2018) 29(10):987–94. doi: 10.1007/s10552-018-1073-5
18. Choi JH, Lee JY, Han KD, Lim YC, Cho JH. Association between obstructive sleep apnoea and breast cancer: The Korean National Health Insurance Service Data 2007-2014. Sci Rep (2019) 9(1):19044. doi: 10.1038/s41598-019-55551-7
19. Gao XL, Jia ZM, Zhao FF, An DD, Wang B, Cheng EJ, et al. Obstructive sleep apnea syndrome and causal relationship with female breast cancer: a mendelian randomization study. Aging (Albany NY) (2020) 12(5):4082–92. doi: 10.18632/aging.102725
20. Qin Y, Zhou Y, Zhang X, Wei X, He J. Sleep duration and breast cancer risk: a meta-analysis of observational studies. Int J Cancer (2014) 134(5):1166–73. doi: 10.1002/ijc.28452
21. Palamaner Subash Shantha G, Kumar AA, Cheskin LJ, Pancholy SB. Association between sleep-disordered breathing, obstructive sleep apnea, and cancer incidence: a systematic review and meta-analysis. Sleep Med (2015) 16(10):1289–94. doi: 10.1016/j.sleep.2015.04.014
22. Gozal D, Ham SA, Mokhlesi B. Sleep apnea and cancer: analysis of a nationwide population sample. Sleep (2016) 39:1493–500. doi: 10.5665/sleep.6004
23. Sillah A, Watson NF, Gozal D, Phipps AI. Obstructive sleep apnea severity and subsequent risk for cancer incidence. Prev Med Rep (2019) 15:100886. doi: 10.1016/j.pmedr.2019.100886
24. Cao Y, Ning P, Li Q, Wu S. Cancer and obstructive sleep apnea An updated meta-analysis. Medicine (2022) 101(10):1–8. doi: 10.1097/MD.0000000000028930
25. Jara SM, Phipps AI, Maynard C, Weaver EM. The association of sleep apnea and cancer in veterans. Otolaryng Head Neck (2020) 162(4):581–8. doi: 10.1177/0194599819900487
26. Kendzerska T, Povitz M, Leung RS, Boulos MI, McIsaac DI, Murray BJ, et al. Obstructive sleep apnea and incident cancer: A large retrospective multicenter clinical cohort study. Cancer Epidemiol Biomarkers Prev (2021) 30(2):295–304. doi: 10.1158/1055-9965.EPI-20-0975
27. Cheng HR, Li DC. Investigation into the association between obstructive sleep apnea and incidence of all-type cancers: a systematic review and meta-analysis. Sleep Med (2021) 88:274–81. doi: 10.1016/j.sleep.2021.05.031
28. Vogtmann E, Levitan EB, Hale L, Shikany JM, Shah NA, Endeshaw Y, et al. Association between sleep and breast cancer incidence among postmenopausal women in the women’s health initiative. Sleep (2013) 36(10):1437–44. doi: 10.5665/sleep.3032
29. Girschik J, Fritschi L, Erren TC, Heyworth J. Quantitative exposure metrics for sleep disturbance and their association with breast cancer risk. Cancer Causes Control (2013) 24(5):919–28. doi: 10.1007/s10552-013-0168-2
30. Girschik J, Heyworth J, Fritschi L. Self-reported sleep duration, sleep quality, and breast cancer risk in a population-based case-control study. Am J Epidemiol (2013) 177(4):316–27. doi: 10.1093/aje/kws422
31. Wong ATY, Heath AK, Tong TYN, Reeves GK, Floud S, Beral V, et al. Sleep duration and breast cancer incidence: results from the Million Women Study and meta-analysis of published prospective studies. Sleep (2021) 44(2). doi: 10.1093/sleep/zsaa166
32. Wu AH, Wang R, Koh WP, Stanczyk FZ, Lee HP, Yu MC. Sleep duration, melatonin and breast cancer among Chinese women in Singapore. Carcinogenesis (2008) 29(6):1244–8. doi: 10.1093/carcin/bgn100
33. Kakizaki M, Inoue K, Kuriyama S, Sone T, Matsuda-Ohmori K, Nakaya N, et al. Sleep duration and the risk of prostate cancer: the Ohsaki Cohort Study. Br J Cancer (2008) 99(1):176–8. doi: 10.1038/sj.bjc.6604425
34. McElroy J, Newcomb P, Titus-Ernstoff L, Trentham-Dietz A, Hampton J, Egan K. Duration of sleep and breast cancer risk in a large population based case-control study. J Sleep Res (2006) 15:241–9. doi: 10.1111/j.1365-2869.2006.00523.x
35. Sigurdardottir LG, Valdimarsdottir UA, Mucci LA, Fall K, Rider JR, Schernhammer E, et al. Sleep disruption among older men and risk of prostate cancer. Cancer Epidemiol Biomarkers Prev (2013) 22(5):872–9. doi: 10.1158/1055-9965.EPI-12-1227-T
36. Li X, Huang D, Liu F, Li X, Lv J, Wu Q, et al. Sleep characteristics and cancer-related outcomes: an umbrella review of systematic reviews and meta-analyses of observational studies. J Clin Med (2022) 11(24):7289. doi: 10.3390/jcm11247289
37. Teo YH, Tan BKJ, Tan NKW, Yap DWT, Chai YX, Teo YN, et al. Obstructive sleep apnea and the incidence and mortality of gastrointestinal cancers: a systematic review and meta-analysis of 5,120,837 participants. J Gastrointest Oncol (2022) 13(6):2789–98. doi: 10.21037/jgo-22-153
38. Liu RL, Wu SR, Zhang BL, Guo MY, Zhang Y. The association between sleep duration and prostate cancer A systematic review and meta-analysis. Medicine (2020) 99(28):1–8. doi: 10.1097/MD.0000000000021180
39. Ahn S, Lobo JM, Logan JG, Kang H, Kwon Y, Sohn MW. A scoping review of racial/ethnic disparities in sleep. Sleep Med (2021) 81:169–79. doi: 10.1016/j.sleep.2021.02.027
40. Chattu VK, Chattu SK, Spence DW, Manzar MD, Burman D, Pandi-Perumal SR. Do disparities in sleep duration among racial and ethnic minorities contribute to differences in disease prevalence? J Racial Ethn Health Disparities (2019) 6(6):1053–61. doi: 10.1007/s40615-019-00607-7
41. Siegel RL, Miller KD, Wagle NS, Jemal A. Cancer statistics, 2023. CA Cancer J Clin (2023) 73(1):17–48. doi: 10.3322/caac.21763
42. Zullig LL, Sims KJ, McNeil R, Williams CD, Jackson GL, Provenzale D, et al. Cancer incidence among patients of the U.S. Veterans affairs health care system: 2010 update. Mil Med (2017) 182(7):e1883–e91. doi: 10.7205/MILMED-D-16-00371
43. Thorpy MJ. Classification of sleep disorders. Neurotherapeutics (2012) 9(4):687–701. doi: 10.1007/s13311-012-0145-6
44. Stroupe KT, Tarlov E, Zhang Q, Haywood T, Owens A, Hynes DM. Use of Medicare and DOD data for improving VA race data quality. J Rehabil Res Dev (2010) 47(8):781–96. doi: 10.1682/JRRD.2009.08.0122
45. Va Information Resource Center (Virec) Data Quatlity Update: Race. (2009). Available online at: www.virec.reesearch.va.gov.
46. Kleinbaum DGaK M. Statistics for biology and health: survival analysi. In: A Self Learning Text, 2nd ed. New York, NY: Springer Science + Business Media, LLC (2005).
47. Therneau TM, Grambsch PM. Modeling Survival Data: Extending the Cox Model New York, NY: Springer (2000).
48. Kakizaki M, Kuriyama S, Sone T, Ohmori-Matsuda K, Hozawa A, Nakaya N, et al. Sleep duration and the risk of breast cancer: the Ohsaki Cohort Study. Br J Cancer (2008) 99(9):1502–5. doi: 10.1038/sj.bjc.6604684
49. Thompson CL, Larkin EK, Patel S, Berger NA, Redline S, Li L. Short duration of sleep increases risk of colorectal adenoma. Cancer (2011) 117(4):841–7. doi: 10.1002/cncr.25507
50. Jiao L, Duan Z, Sangi-Haghpeykar H, Hale L, White DL, El-Serag HB. Sleep duration and incidence of colorectal cancer in postmenopausal women. Br J Cancer (2013) 108(1):213–21. doi: bjc2012561
51. Ruesten A, Weikert C, Fietze I, Boeing H. Association of sleep duration with chronic diseases in the european prospective investigation into cancer and nutrition (EPIC)-potsdam study. PloS One (2012) 7(1):e30972. doi: 10.1371/journal.pone.0030972
52. Zhang X, Giovannucci EL, Wu K, Gao X, Hu F, Ogino S, et al. Associations of self-reported sleep duration and snoring with colorectal cancer risk in men and women. Sleep (2013) 36(5):681–8. doi: 10.5665/sleep.2626
53. Peng TR, Yang LJ, Wu TW, Chao YC. Hypnotics and risk of cancer: A meta-analysis of observational studies. Medicina (Kaunas) (2020) 56(10):1–16. doi: 10.3390/medicina56100513
54. Geronimus AT, Hicken M, Keene D, Bound J. “Weathering” and age patterns of allostatic load scores among blacks and whites in the United States. Am J Public Health (2006) 96(5):826–33. doi: 10.2105/AJPH.2004.060749
55. Hebert JR, Braun KL, Kaholokula JK, Armstead CA, Burch JB, Thompson B. Considering the role of stress in populations of high-risk, underserved community networks program centers. Prog Community Health Partnersh (2015) 9 Suppl:71–82. doi: 10.1353/cpr.2015.0028
56. Zavala VA, Bracci PM, Carethers JM, Carvajal-Carmona L, Coggins NB, Cruz-Correa MR, et al. Cancer health disparities in racial/ethnic minorities in the United States. Br J Cancer (2021) 124(2):315–32. doi: 10.1038/s41416-020-01038-6
57. Lucas T, Wegner R, Pierce J, Lumley MA, Laurent HK, Granger DA. Perceived discrimination, racial identity, and multisystem stress response to social evaluative threat among African American men and women. Psychosom Med (2017) 79(3):293–305. doi: 10.1097/PSY.0000000000000406
58. Stepanikova I, Bateman LB, Oates GR. Systemic inflammation in midlife: race, socioeconomic status, and perceived discrimination. Am J Prev Med (2017) 52(1S1):S63–76. doi: 10.1016/j.amepre.2016.09.026
59. Smith MR, Burgess HJ, Fogg LF, Eastman CI. Racial differences in the human endogenous circadian period. PloS One (2009) 4(6):e6014. doi: 10.1371/journal.pone.0006014
60. Eastman CI, Molina TA, Dziepak ME, Smith MR. Blacks (African Americans) have shorter free-running circadian periods than whites (Caucasian Americans). Chronobiology Int (2012) 29(8):1072–7. doi: 10.3109/07420528.2012.700670
61. Durrence HH, Lichstein KL. The sleep of African Americans: a comparative review. Behav Sleep Med (2006) 4(1):29–44. doi: 10.1207/s15402010bsm0401_3
62. McMenamin TM. A time to work: recent trends in shift work and flexible schedules. Monthly Labor Rev (2007) 130(12):3–15.
63. Simons RL, Lei MK, Beach SRH, Barr AB, Simons LG, Gibbons FX, et al. Discrimination, segregation, and chronic inflammation: Testing the weathering explanation for the poor health of Black Americans. Dev Psychol (2018) 54(10):1993–2006. doi: 10.1037/dev0000511
64. Tobaldini E, Nobili L, Strada S, Casali KR, Braghiroli A, Montano N. Heart rate variability in normal and pathological sleep. Front Physiol (2013) 4:294. doi: 10.3389/fphys.2013.00294
65. Smith R, Thayer JF, Khalsa SS, Lane RD. The hierarchical basis of neurovisceral integration. Neurosci Biobehav Rev (2017) 75:274–96. doi: 10.1016/j.neubiorev.2017.02.003
66. Stein PK, Pu Y. Heart rate variability, sleep and sleep disorders. Sleep Med Rev (2012) 16(1):47–66. doi: 10.1016/j.smrv.2011.02.005
67. Porges SW. The polyvagal theory: new insights into adaptive reactions of the autonomic nervous system. Cleve Clin J Med (2009) 76 Suppl 2:86. doi: 10.3949/ccjm.76.s2.17
68. Hill LK, Hoggard LS, Richmond AS, Gray DL, Williams DP, Thayer JF. Examining the association between perceived discrimination and heart rate variability in African Americans. Cultur Divers Ethnic Minor Psychol (2017) 23(1):5–14. doi: 10.1037/cdp0000076
69. Goldstein-Piekarski AN, Greer SM, Saletin JM, Walker MP. Sleep deprivation impairs the human central and peripheral nervous system discrimination of social threat. J Neurosci (2015) 35(28):10135–45. doi: 10.1523/JNEUROSCI.5254-14.2015
70. Zhong X, Hilton HJ, Gates GJ, Jelic S, Stern Y, Bartels MN, et al. Increased sympathetic and decreased parasympathetic cardiovascular modulation in normal humans with acute sleep deprivation. J Appl Physiol (1985) (2005) 98(6):2024–32. doi: 10.1152/japplphysiol.00620.2004
71. Esposito K, Chiodini P, Colao A, Lenzi A, Giugliano D. Metabolic syndrome and risk of cancer: a systematic review and meta-analysis. Diabetes Care (2012) 35(11):2402–11. doi: 10.2337/dc12-0336
72. Nevels TL, Wirth MD, Ginsberg JP, McLain AC, Burch JB. The role of sleep and heart rate variability in metabolic syndrome: evidence from the Midlife in the United States study. Sleep (2023) 46(5):1–11. doi: 10.1093/sleep/zsad013
73. De Couck M, Gidron Y. Norms of vagal nerve activity, indexed by Heart Rate Variability, in cancer patients. Cancer Epidemiol (2013) 37(5):737–41. doi: 10.1016/j.canep.2013.04.016
74. Cooper TM, McKinley PS, Seeman TE, Choo TH, Lee S, Sloan RP. Heart rate variability predicts levels of inflammatory markers: evidence for the vagal anti-inflammatory pathway. Brain Behav Immun (2015) 49:94–100. doi: 10.1016/j.bbi.2014.12.017
75. Chang A, Kim-Fuchs C, Le CP, Hollande F, Sloan EK. Neural regulation of pancreatic cancer: A novel target for intervention. Cancers (Basel) (2015) 7(3):1292–312. doi: 10.3390/cancers7030838
76. Taylor MR, Knight JM, Rosenberg AR. The biology of stress in cancer: Applying the biobehavioral framework to adolescent and young adult oncology research. Brain Behav Immun Health (2021) 17:100321. doi: 10.1016/j.bbih.2021.100321
77. Zygulska AL, Furgala A, Krzemieniecki K, Wlodarczyk B, Thor P. Autonomic dysregulation in colon cancer patients. Cancer Invest (2018) 36:255–63. doi: 10.1080/07357907.2018.1474893
78. Li ZY, Wang K, Shivappa N, Hébert JR, Chen H, Liu H, et al. Inflammatory potential of diet and colorectal carcinogenesis: a prospective longitudinal cohort. Br J Cancer (2022) 126(12):1735–43. doi: 10.1038/s41416-022-01731-8
79. Park YMM, Shivappa N, Petimar J, Hodgson ME, Nichols HB, Steck SE, et al. Dietary inflammatory potential, oxidative balance score, and risk of breast cancer: Findings from the Sister Study. Int J Cancer (2021) 149(3):615–26. doi: 10.1002/ijc.33581
80. Green MJ, Espie CA, Hunt K, Benzeval M. The longitudinal course of insomnia symptoms: inequalities by sex and occupational class among two different age cohorts followed for 20 years in the west of Scotland. Sleep (2012) 35(6):815–23. doi: 10.5665/sleep.1882
81. McMahon DM, Burch JB, Wirth MD, Youngstedt SD, Hardin JW, Hurley TG, et al. Persistence of social jetlag and sleep disruption in healthy young adults. Chronobiol Int (2018) 35(3):312–28. doi: 10.1080/07420528.2017.1405014
82. Burch JB, Wirth M, Yang X. Disruption of circadian rhythms and sleep: role in carcinogenesis. In: Kushida CA, editor. The Encyclopedia of Sleep, vol. 3 . Academic Press, Waltham, MA (2013). p. 150–5.
83. National Toxicology Program Cancer Hazard Assessment Report on Night Shift Work and Light at Night. Research Triangle Park, NC: U.S. Department of Health and Human Services (2021).
84. Kripke DF. Do hypnotic drugs cause cancer, like cigarettes? Sleep Med (2015) 16(12):1550–1. doi: 10.1016/j.sleep.2015.05.011
85. Poza JJ, Pujol M, Ortega-Albas JJ, Romero O. Insomnia Study Group of the Spanish Sleep S. Melatonin in sleep disorders. Neurologia (Engl Ed) (2022) 37(7):575–85. doi: 10.1016/j.nrl.2018.08.002
86. Cruz-Sanabria F, Carmassi C, Bruno S, Bazzani A, Carli M, Scarselli M, et al. Melatonin as a chronobiotic with sleep-promoting properties. Curr Neuropharmacol (2023) 21(4):951–87. doi: 10.2174/1570159X20666220217152617
87. Talib WH, Alsayed AR, Abuawad A, Daoud S, Mahmod AI. Melatonin in cancer treatment: current knowledge and future opportunities. Molecules (2021) 26(9):1–46. doi: 10.3390/molecules26092506
88. Reiter RJ, Rosales-Corral SA, Tan DX, Acuna-Castroviejo D, Qin LL, Yang SF, et al. Melatonin, a full service anti-cancer agent: inhibition of initiation, progression and metastasis. Int J Mol Sci (2017) 18(4):1–47. doi: 10.3390/ijms18040843
89. Mogavero MP, Silvani A, Lanza GSP, DelRosso LM, Ferini-Strambi L, Ferri R. Targeting orexin receptors for the treatment of insomnia: from physiological mechanisms to current clinical evidence and recommendations. Nat Sci Sleep (2023) 15:17–38. doi: 10.2147/NSS.S201994
90. Lehrer P, Kaur K, Sharma A, Shah KB, Huseby R, Bhavsar J, et al. Heart rate variability biofeedback improves emotional and physical health and performance: A systematic review and meta analysis. Appl Psychophys Biof (2020) 45(3):109–29. doi: 10.1007/s10484-020-09466-z
Keywords: apnea, cancer, insomnia, risk, sleep, veteran
Citation: Burch JB, Delage AF, Zhang H, McLain AC, Ray MA, Miller A, Adams SA and Hébert JR (2024) Sleep disorders and cancer incidence: examining duration and severity of diagnosis among veterans. Front. Oncol. 14:1336487. doi: 10.3389/fonc.2024.1336487
Received: 13 November 2023; Accepted: 29 January 2024;
Published: 26 February 2024.
Edited by:
Zhengming Chen, Cornell University, United StatesReviewed by:
Mario Cioce, Campus Bio-Medico University, ItalyMasoud Sadeghi, Islamic Azad University, Iran
Antonino Maniaci, Kore University of Enna, Italy
Anna Brzecka, Wroclaw Medical University, Poland
Copyright © 2024 Burch, Delage, Zhang, McLain, Ray, Miller, Adams and Hébert. This is an open-access article distributed under the terms of the Creative Commons Attribution License (CC BY). The use, distribution or reproduction in other forums is permitted, provided the original author(s) and the copyright owner(s) are credited and that the original publication in this journal is cited, in accordance with accepted academic practice. No use, distribution or reproduction is permitted which does not comply with these terms.
*Correspondence: James B. Burch, amFtZXMuYi5idXJjaEB2Y3VoZWFsdGgub3Jn