- 1Department of Otolaryngology-Head and Neck Surgery, the Affiliated Hospital of Southwest Medical University, Southwest Medical University, Luzhou, Sichuan, China
- 2Department of Otolaryngology-Head and Neck Surgery, Chengdu Second People’s Hospital, Chengdu, Sichuan, China
- 3Department of Otolaryngology-Head and Neck Surgery, Bishan hospital of Chongqing Medical University, Bishan Hospital of Chongqing, Bishan, Chongqing, China
Nasopharyngeal carcinoma (NPC) is a highly prevalent head and neck malignancy in southern China frequently diagnosed at advanced stages owing to subtle early symptoms and associated metastasis. Angiogenesis emerges as a pivotal factor in NPC progression, with numerous angiogenesis-related factors showing aberrant expression and contributing to increased neovascularization within NPC tumors. These abnormal vessels not only nourish tumor growth but also facilitate metastasis, culminating in unfavorable patient outcomes. Multiple studies have demonstrated the applicability of various imaging techniques for assessing angiogenesis in NPC tumors, thus serving as a foundation for personalized treatment strategies and prognostic assessments. Anti-angiogenic therapies have exhibited significant potential for inhibiting NPC angiogenesis and exerting anti-tumor effects. To enhance efficacy, anti-angiogenic drugs are frequently combined with other treatment modalities to synergistically enhance anti-tumor effects while mitigating the side effects associated with single-agent therapies, consequently improving patient prognosis. Identifying the potential mechanisms and key targets underlying NPC angiogenesis and exploring more effective detection and treatment approaches holds promise for shaping the future of NPC diagnosis, treatment, and prognosis, thereby offering new avenues and perspectives for research and clinical practice.
1 Introduction
Nasopharyngeal carcinoma (NPC) is a prevalent head and neck cancer in Southeast Asia, particularly in southern China, and often presents challenges due to its hidden location and nonspecific early symptoms (1, 2). As an epithelial carcinoma originating from the nasopharyngeal mucosa, NPC exhibits high sensitivity to radiotherapy (3). However, its propensity for local recurrence and distant metastasis often leads to treatment failure and poor prognosis (4–6). The precise mechanism underlying distant metastasis of NPC remains elusive; nevertheless, there is a consensus that angiogenesis significantly contributes to this process.
NPC represents a pathological ecosystem wherein a close relationship exists between the tumor and the host, with the vascular system serving as an integral component (7). Angiogenesis is an essential step in tumor progression, which not only facilitates the supply of nutrients crucial for tumor growth but also serves as a conduit for eliminating the metabolic waste generated by cells. In contrast, tumor cells release pro-angiogenic factors that stimulate the proliferation and migration of vascular endothelial cells, ultimately leading to the formation of new blood vessels. This intricate process accelerates tumor growth and facilitates metastasis (8). Moreover, newly formed blood vessels within the tumor exhibit immaturity and high permeability, resulting in inadequate perfusion of the tumor and the creation of a hypoxic microenvironment (9). This further promotes tumor invasion and metastasis while inhibiting the effectiveness of immune cells and reducing the diffusion and efficacy of chemotherapy drugs. Clinically, these factors contribute to poor prognosis (10).
Currently, radiotherapy is widely considered the primary treatment for early-stage non-metastatic NPC. However, when early distant metastasis occurs, radiotherapy combined with chemotherapy is more effective in improving the prognosis (1, 2, 11). However, resistance to chemoradiotherapy results in unsatisfactory therapeutic effects (4–6). NPC angiogenesis plays a crucial role in tumor progression (12), significantly affecting both patient outcomes and prognosis, highlighting its considerable clinical value and prospects for anti-angiogenic therapy. Therefore, gaining a comprehensive understanding of the research advancements and applications of angiogenesis-related NPC is of tremendous importance for treating advanced or metastatic NPC.
2 Transcriptional regulation of angiogenesis in NPC
2.1 VEGF
As a highly specific mitogen of endothelial cells, vascular endothelial growth factor (VEGF) can strongly stimulate endothelial cell proliferation to induce angiogenesis and lymphangiogenesis (13). When VEGF expression levels increase in the tumor tissues or serum of NPC patients, it typically closely correlates with TNM staging, leads to a higher risk of distant metastasis, and lowers long-term survival rates; therefore, it could be a potential marker for distant metastasis and prognosis in NPC (14–18). VEGF serves as a prognostic indicator for NPC patients and is positively associated with an unfavorable prognosis (15, 17). Serum VEGF detection proves beneficial in predicting tumor metastasis and the clinical outcome of the patients (14, 16). VEGF expression is significantly correlated with microvessel density (MVD), which is a potent indicator of vasculogenesis (19). VEGF and MVD in NPC show a positive correlation with tumor progression and stage (20). Secreted by NPC cells VEGF promotes tumor angiogenesis, cell invasion, and migration (21). Exosomes released by tumor cells can stimulate VEGF-related pathways, fostering angiogenesis (22, 23). EBV-encoded proteins can contribute to promoting tumor growth and progression by interacting with VEGF (24, 25). VEGF is subject to regulation by various factors. For instance, the pro-angiogenic effect of chemotaxis-related factors is mediated through the regulation of VEGF expression (26). In the hypoxic conditions resulting from extensive tumor growth, activated HIF-1α stimulates VEGF transcription (27). In the angiogenesis mechanism, VEGF can activate the Ras/MAPK and PI3K/AKT pathways by influencing matrix metalloproteinases and cyclooxygenase-2 in NPC (21, 28). Conversely, when VEGF function is disrupted, angiogenesis is prevented in NPC (29). Clinically, quercetin, a plant extract, along with several tyrosine kinase inhibitors, inhibits angiogenesis by targeting VEGF or its receptors (29–32). Gene polymorphisms, specifically VEGF-460T/C and VEGF-2578C/A, can influence the risk and invasiveness of NPC. These variations are significant in guiding the assessment of clinical outcomes in patients, potentially associated with angiogenesis (33, 34).
2.2 ANG
Angiogenin (ANG) is a secreted ribonuclease with proangiogenic properties. Angiogenin-2 (ANG-2) plays a beneficial role in promoting angiogenesis in NPC (35). Particularly, ANG-2 has been reported to exert an anti-vascular effect in NPC, which is highly dependent on VEGF expression levels. When endogenous VEGF is absent, ANG-2 overexpression decreases the tumor microvascular density, thereby playing an antitumor role (36). Furthermore, angiotensin- (1–7) [ANG- (1–7)] can against the tumor angiogenic process by reducing the expression of VEGF and hypoxia-inducible factor-1α (HIF-1α) (37).
2.3 HIF-1α
HIF-1α is an important regulatory factor in the adaptation of tumor cells to anoxic environmental conditions (27). It can serve as an indicator of hypoxia within tumors and is intricately linked to processes such as angiogenesis, invasion, metastasis, energy metabolism, and resistance to tumor radiotherapy. During the NPC angiogenic process, HIF-1α is regulated by many factors. To achieve Epstein–Barr virus (EBV)-induced vasculogenic mimicry (VM), EBV stimulates the expression of HIF-1α, which is also activated by latent membrane protein 2A (LMP2A) (38). Additionally, EBV-miR-BART1-5P (39), NPC-extracellular vesicle (EV) -derived miR-144 (40), chemokine (C-C motif) ligand (CCL5) (41), EBV- Epstein-Barr nuclear antigen 1 (EBNA1) (25), forkhead box M1 (42), BART10-5p and miR-18a (43) can increase HIF-1α synthesis to bring about tumor angiogenesis and progression. NPC tissue growth leads to the formation of a hypoxic microenvironment. In this hypoxic condition, HIF-1α expression can be inhibited by α-momorcharin, causing the NPC vessel formation suppressed (44).
2.4 MMPs
Matrix metalloproteinases (MMPs) are a group of endogenous peptidases that degrade extracellular matrix (45). Exosomal MMP-13 plays a pro-angiogenic role in NPC (46). MMPs also act as mediators of other factors that enhance angiogenesis in NPC. MMP-9 cooperates with the protease-activated receptor 2 and EBV-encoded latent membrane protein 1 (LMP1) to induce vasculogenesis in NPC (47). Ras-like estrogen-regulated growth inhibitor (RERG) can suppress MMPs’ expression and influence tumor vessel formation (48). In another study, RBMS3 (RNA binding motif, single-stranded interacting protein 3) indirectly inhibits the angiogenic effects of MMP-9 and MMP-2 (49). ADAMTS9, a member of the ADAMTS (a disintegrin-like and metalloproteinase (reprolysin type) with thrombospondin type 1 motif) metalloproteinase family, downregulates MMP-9 and VEGF-A expression to inhibit NPC growth and vessel formation (50).
2.5 NF-кB
Nuclear transcription factor кB (NF-кB), a family of transcription factors, is involved in the regulation of tumor angiogenesis and growth. According to some studies, NF-κB can restrain the ability to promote NPC neovascularization by tumor suppressor genes, such as transforming growth factor-β binding protein 2 (51), RERG (48), cylindromatosis lysine 63 deubiquitinase (52) and NFKB inhibitor beta (53). Additionally, it has been reported that upregulated receptor-interacting serine/threonine kinase 4 (RIPK4) (54), LMP1 (55, 56), and pregnancy upregulated nonubiquitous calmodulin (CaM) kinase (PNCK) (57) are related to the activation of NF-кB signaling pathway, which results in tumor vessel formation and disease progression.
3 EBV-associated NPC angiogenesis factors
In addition to host genetics, EBV infection may be the most common causal agent of NPC (2) (Figure 1A). The positive correlation between plasma EBV DNA load and NPC risk not only makes it a valuable tool for NPC screening (58) but also underscores its close association with NPC neovascularization. EBV could activate stromal interaction molecule 1 (STIM1) -dependent Ca2+ signaling to promote vasculogenesis in NPC (59) and increased expression of the angiogenic factor CCL5, promoting NPC vessel formation through modulation of the PI3K/AKT and HIF-1α pathways (41). In NPC, EBV could promote VM by activating the LMP2A-mediated PI3K/AKT/mTOR/HIF-1α signaling pathway (38).
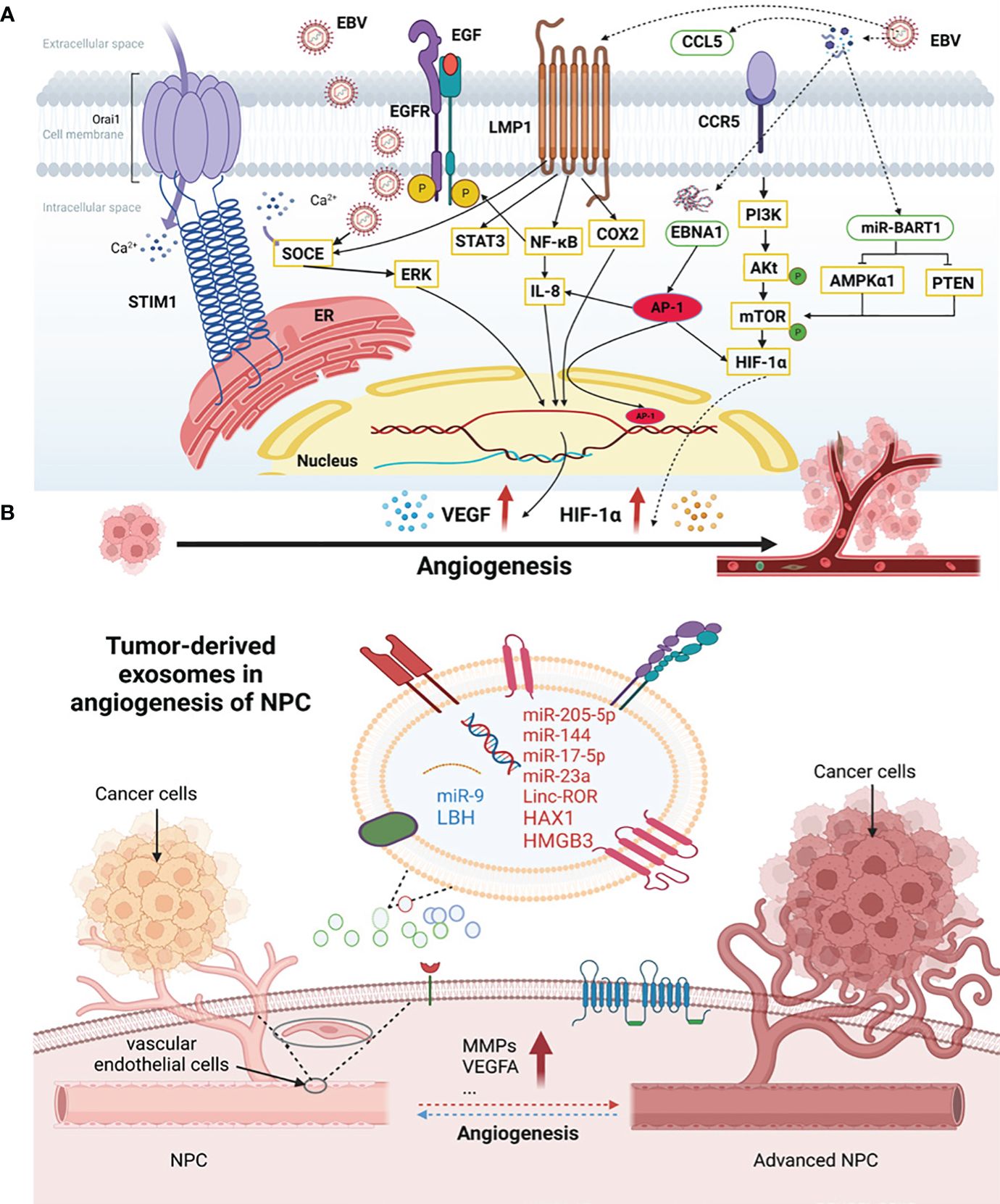
Figure 1 The association of EBV infection and exosomes with angiogenesis in NPC. (A) EBV-associated NPC angiogenesis factors. EBV and some genes encoded by EBV could activate the angiogenesis in NPC. EGF, epidermal growth factors; EGFR, epidermal growth factor receptors; SOCE, store-operated Ca2+ entry; STIM1, stromal interaction molecule 1; LMP1, latent membrane protein 1; STAT3, signal transducer and activator of transcription 3; IL-8, interleukin-8; COX-2, cyclooxygenase-2; EBNA1, Epstein-Barr nuclear antigen 1; AMPKα1, AMP-activated protein kinase; PTEN, tensin homolog deleted on chromosome ten; CCL5, chemokine (C-C motif) ligand; CCR5, C-C chemokine receptor type 5; ERK, extracellular regulated kinase; PI3K, phosphoinositide 3-kinase; mTOR, mammalian target of the rapamycin; VEGF, vascular endothelial growth factor; HIF-1α, hypoxia-inducible factor-1α; ER, endoplasmic reticulum. (B) Tumor-derived exosomes in angiogenesis of NPC. NPC cells secreted exosomes which can be internalized and absorbed by endothelial cells and tumor cells themselves, promoting the angiogenic process. HAX1, HS1-related protein X-1; HMGB3, high-mobility group box 3; LBH, limb-bud and heart; VEGF, vascular endothelial growth factor; MMPs, matrix metalloproteinases.
Moreover, some genes encoded by EBV actively participate in NPC neovascularization (Figure 1A). LMP1, a proto-oncogene encoded by EBV, induces angiogenesis through several signaling pathways (60). It promotes the expression of epidermal growth factor receptors (EGFR) in NPC cells by activating the NF-κB pathway and signal transducer and activator of transcription 3 (STAT3), which affects angiogenesis and enhances EBV infection in NPC cells (55). LMP1 also promotes VM through the VEGF/VEGFR1 signaling pathway (61). Under the influence of extracellular epidermal growth factors (EGF), LMP1 enhances VEGF-mediated angiogenesis by facilitating store-operated Ca2+ entry (SOCE) (24). Additionally, LMP1 can induce VEGF expression through the JAK/STAT and MAPK/ERK pathways to upregulate tumor vasculogenesis (62). In the NPC cell line NPC-KT, LMP1 induces interleukin-8 (IL-8) expression to regulate angiogenesis by activating the NF-κB pathway (56). Research shows that LMP1-induced cyclooxygenase-2 (COX-2) may play a role in NPC angiogenesis (63).
Moreover, EBV-encoded EBNA1 stimulates the expression of transcription factor AP-1, which can promote NPC neovasculogenesis by targeting IL-8, VEGF, and HIF-1α (25). EBV-encoded RNAs promote angiogenesis by stimulating vascular cell adhesion molecule-1 expression (64). EBV-miR-Bart1-5p, a key miRNA encoded by EBV, can directly target AMP-activated protein kinase (AMPKα1) and activate the AMPK/mTOR/HIF-1 pathway, inducing abnormal glycolysis and angiogenesis in NPC cells (39).
4 Exosome-associated NPC angiogenesis factors
Exosomes, a subtype of EVs, are crucial mediators of intercellular communication, being vesicular structures secreted by cells and containing proteins, nucleic acids, and other bioactive molecules that facilitate this process. Exosomes secreted by tumor cells can be internalized and absorbed by endothelial cells and tumor cells themselves, promoting angiogenesis and a premetastatic niche, ultimately leading to tumor progression (65) (Figure 1B). Similarly, exosomes in NPC have been reported to be associated with pathological angiogenesis, distant metastasis, resistance to chemoradiotherapy, and immunosuppression, and their influence extends to the tumor microenvironment (66).
lncRNAs, miRNAs, and other non-coding RNAs are important components of exosomes derived from tumor cells and participate in NPC neovascularization. Linc-ROR, a long-stranded exosomal non-coding RNA of nasopharyngeal origin, promotes NPC proliferation, migration, and angiogenesis via the p-AKT/p-VEGFR2 pathway (67). Exosome miR-205-5p plays a vasostimulatory role in NPC by activating the EGFR/ERK signaling pathway and MMPs expression by targeting desmocollin-2 (68). MiR-144 in EVs derived from NPC plays a role in promoting tumor progression through the FBXW7/HIF-1a/VEGF-A axis, which promotes angiogenesis (40). MiR-17-5p, which is highly expressed in NPC, targets bone morphogenetic protein and activin membrane-bound inhibitor and regulates the AKT/VEGF-A signaling pathway to increase angiogenesis (22). MiR-23a plays an important role in NPC neovasculogenesis by acting on TSGA10 (69). However, exosomal miR-9 secreted by NPC cells inhibits vascular formation and metastasis by targeting midkine and regulating PDK/AKT signaling (70).
Other factors derived from NPC exosomes have been reported. HS1-related protein X-1 (HAX1) in EVs enhances the expression level of integrin β6 and regulates the FAK pathway to promote tumor angiogenesis (71, 72). High-mobility group box 3 (HMGB3) of nuclear exosome origin accelerates pathological vasculogenesis in NPC (73). NPC exosomes containing limb-bud and heart (LBH) inhibit epithelial-mesenchymal transformation and angiogenesis by regulating VEGF-A (74). Additionally, PFKFB-3 (Enzymes 6-phosphofructo-2-kinase/fructose-2, 6-bisphosphatase-3) (75), MMP13 (46), intercellular adhesion molecule-1 (ICAM-1), CD44 variant isoform 5 and platelet response protein-1 (76) in exosomes are involved in the regulation of NPC angiogenesis.
5 Other NPC angiogenesis factors
There are many studies on NPC angiogenesis. For instance, the proangiogenic lncRNA LINC00240 functions by inhibiting the expression of miR-26a-5p (77). The enhancer of zeste homolog 2 (EZH2), which is highly expressed in NPC, can activate NPC neovasculogenesis via the EZH2-miR-1-ET-1 axis and promote the proliferation, migration, and VM of NPC cells (78). Additionally, kinesin family member 2A (79), vimentin (80), tripartite motif-containing 24 (81), CD93 (82), TWIST (12), and annexin A2 (83) exert angiogenic effects on NPC. Instead, it is reported that in NPC human METCAM/MUC18 (84), inhibitor of growth 4 (ING4), PTEN (phosphatase and tensin homolog deleted on chromosome ten) (85), IkappaB kinase alpha (86), PTPRG (protein tyrosine phosphatase, receptor type G) (87), fibulin-2 (88) and latent TGF-β binding protein 2 (89) plays an anti-angiogenic role. In addition to the aforementioned factors, hypoxia can trigger angiogenesis of NPC (90).
6 Angiogenic application in NPC
6.1 Angiogenesis-related imaging studies in NPC
Abnormal microangiogenesis can aggravate hypoxia, which is an important factor affecting treatment resistance and poor prognosis of NPC. Dynamic contrast-enhanced magnetic resonance imaging (DCE-MRI) is a noninvasive technique that reflects capillary permeability, angiogenic activity, tumor angiogenesis, blood perfusion, and hypoxic status of NPC tissues. Remarkably, some parameters in DCE-MRI are associated with positive expression of HIF-1α in NPC, which can provide the basis for the formulation of individualized treatment for NPC patients (91). Due to significant changes in dynamic parameters during radiotherapy, DCE-MRI can monitor NPC angiogenesis during treatment and quantitatively evaluate the effects of tumor treatment (60). Multiple studies have shown that magnetic resonance perfusion-weighted imaging (MR-PWI) (92) and diffusion-weighted imaging (DWI) also reflect NPC angiogenesis and radiotherapy sensitivity. Common parameters in DWI assessments include pure molecular diffusion (D) and perfusion-related diffusion (D*). Research has indicated that elevated D* values correlate with increased angiogenesis and parenchymal perfusion in NPC (93, 94). The D value was significantly decreased in primary NPC; however, elevated D and D* values indicated radiosensitivity of the cancer, suggesting a favorable prognosis for the tumor.
Contrast-enhanced ultrasound (CEUS) is an imaging technique that assesses tumor vasculogenesis by examining the diffusion patterns of contrast agents within a tumor. It has been claimed that MVD in NPC grafts can be reflected by CEUS parameters in a nude mouse model (95). Additionally, dynamic contrast-enhanced ultrasonography (DCE-US) can be used to evaluate the efficacy of anti-angiogenic therapy in NPC (96).
6.2 Angiogenesis-related therapy in NPC
In recent years, various new treatment schemes have been developed, among which anti-angiogenesis therapy has emerged and has been used in clinical practice to inhibit the malignant progression of tumors by blocking or inhibiting the related regulatory pathways (Table 1) (Supplementary Table 1).
Bevacizumab, a monoclonal antibody targeting VEGF, effectively inhibits VEGF activity, thereby achieving the objectives of anti-tumor angiogenesis and metastasis suppression in NPC (97). This therapy can be used for the treatment of locally advanced and metastatic NPC (98–100). Recent studies have demonstrated that Endostar, a recombinant human endostatin, is a targeted drug with antiangiogenic and antitumor effects, and when combined with other antitumor therapies, it significantly enhances the overall anticancer effect (101, 102). Endostar significantly enhances radiosensitivity in NPC, reducing the side effects of radiotherapy. Moreover, it can be combined with chemotherapy or chemoradiotherapy to improve the prognosis of patients with metastatic NPC (101, 103, 104). The effect of endostar is related to the downregulation of VEGF expression (105) and could correct the pathological angiogenesis process to disrupt the hypoxic environment in tumor tissues (106).
Many kinase inhibitors can also be employed as anti-angiogenic drugs for NPC treatment. Apatinib, famitinib, sunitinib, and lenvatinib are tyrosine kinase inhibitors that inhibit MVD in patients with NPC. The mechanism of tumor angiogenesis inhibition by apatinib is related to blocking the binding of VEGF and VEGFR. Studies have confirmed that apatinib can achieve good efficacy in the treatment of NPC patients with lung metastasis and advanced VEGFR-2-negative NPC while ensuring safety (107). Apatinib can be used not only as a monotherapy but also in combination with radiotherapy or chemotherapy to treat patients with NPC, effectively enhancing its anti-vascular effects (29, 108). Famitinib combined with radiotherapy increased the radiosensitivity of NPC cells by inhibiting angiogenesis (109). As a single agent, sunitinib significantly inhibited tumor growth and angiogenesis in NPC xenografts. These effects can be enhanced when combined with chemotherapy (32). For anti-angiogenic drug-resistant NPC, lenvatinib has been shown to effectively reverse the resistance of NPC with high FGF-2 expression (31). Additionally, the angiokinase inhibitor BIBF 1120 has an antitumor angiogenic effect and can be used in combination with cisplatin to treat NPC (115).
Many traditional Chinese medicines and their extracts have antitumor effects in the antivascular treatment of NPC. Bioinformatics analysis showed that Rhizoma Curcumae could inhibit angiogenesis in NPC (112). After treatment with quercetin, VEGF expression and NF-κB activity were decreased, and endothelial cell tube formation was inhibited (30). Li Yanwei et al. found that NPC01, an ancient recipe from the Song Dynasty of China, may play an anti-angiogenic role in cancer by inhibiting the effects of pro-angiogenic factors HIF-1α and VEGF (113). Triptolide, a traditional Chinese medicinal extract combined with radiotherapy, inhibits the growth and angiogenesis of NPC (114).
Furthermore, some drugs have unexpected effects in anti-angiogenic therapy for NPC, in addition to their traditional effects. Low doses of the opioid analgesic morphine in NPC led to chemoresistance, which was surprisingly associated with reduced tumor neovascularization, whereas high doses had the opposite effect (110). Angiotensin II receptor blockers (ARBs), which are commonly used in cardiovascular diseases, not only have traditional antihypertensive effects but also have anti-angiogenic effects in NPC by promoting cell apoptosis (111).
7 Conclusions
Angiogenesis is an essential process for the distant metastasis and local recurrence of NPC; however, the underlying mechanism is intricate and ambiguous. Based on the current state of researches, key factors such as VEGF, ANG, HIF-1α, MMPs, and NF-κB play a pivotal role in the angiogenesis of NPC (Supplementary Table 2). Recent findings have highlighted the regulatory roles of EBV infection and exosomes in angiogenesis. In clinical practice, DCE-MRI and CEUS have proven to be effective imaging techniques for angiogenesis detection. Furthermore, the use of antiangiogenic drugs, such as bevacizumab and Endostar, either alone or in combination with other chemotherapy drugs, has demonstrated significant potential for enhancing the prognosis of patients with NPC.
The ongoing advancements in angiogenesis research within NPC offer a novel avenue for the identification of biomarkers. The miRNAs within exosomes and the distinctive molecules linked to EBV imply a potential correlation with the angiogenesis and metastasis of NPC. Subsequent investigations are warranted to identify more precise and clinically significant markers related to exosomes and EBV. These may offer valuable insights for clinical diagnosis and prognosis of NPC.
Multi-targeted anti-angiogenic approach may emerge as a more efficacious strategy for NPC treatment. However, the reported clinical anti-angiogenesis drugs are limited, and their targets exhibit relative simplicity. EBV participates in NPC angiogenesis through diverse pathways. Exploring crucial targets and developing drugs targeting Epstein-Barr virus-mediated angiogenesis could represent a novel avenue for anti-angiogenic interventions. Combining such approaches with anti-angiogenic drugs may enhance the efficacy of NPC treatment in the future.
In summary, a better understanding of angiogenesis provides new insights into the mechanism of NPC. Advancements in key factors, EBV infection, and exosomes hold promise for enhancing NPC diagnosis, treatment, and prognosis, paving the way for future research and clinical application of NPC.
Author contributions
CX: Conceptualization, Writing – original draft, Writing – review & editing. JZ: Conceptualization, Investigation, Writing – original draft. YH: Methodology, Writing – original draft. HM: Methodology, Writing – original draft. FZ: Funding acquisition, Supervision, Writing – review & editing.
Funding
The author(s) declare financial support was received for the research, authorship, and/or publication of this article. This work was supported by the National Natural Science Foundation of China under Grant (81602570); the Health Commission of Sichuan Province under Grant (17ZB0465); and the Education Department of Sichuan Province under Grant (17PJ532).
Acknowledgments
We thank Elsevier Webshop language services for language help and BioRender.com for Figure drafting.
Conflict of interest
The authors declare that the research was conducted in the absence of any commercial or financial relationships that could be construed as a potential conflict of interest.
Publisher’s note
All claims expressed in this article are solely those of the authors and do not necessarily represent those of their affiliated organizations, or those of the publisher, the editors and the reviewers. Any product that may be evaluated in this article, or claim that may be made by its manufacturer, is not guaranteed or endorsed by the publisher.
Supplementary material
The Supplementary Material for this article can be found online at: https://www.frontiersin.org/articles/10.3389/fonc.2024.1331064/full#supplementary-material
References
1. Lee AW, Ma BB, Ng WT, Chan AT. Management of nasopharyngeal carcinoma: current practice and future perspective. J Clin Oncol. (2015) 33:3356–64. doi: 10.1200/JCO.2015.60.9347
2. Chen YP, Chan ATC, Le QT, Blanchard P, Sun Y, Ma J. Nasopharyngeal carcinoma. Lancet (London England). (2019) 394:64–80. doi: 10.1016/S0140-6736(19)30956-0
3. Jicman Stan D, Niculet E, Lungu M, Onisor C, Rebegea L, Vesa D, et al. Nasopharyngeal carcinoma: A new synthesis of literature data (Review). Exp Ther Med. (2022) 23:136. doi: 10.3892/etm.2021.11059
4. Chen S, Lv L, Zhan Z, Wang X, You Z, Luo X, et al. Silencing of long noncoding RNA SRRM2-AS exerts suppressive effects on angiogenesis in nasopharyngeal carcinoma via activating MYLK-mediated cGMP-PKG signaling pathway. J Cell Physiol. (2020) 235:7757–68. doi: 10.1002/jcp.29382
5. Xiao WW, Huang SM, Han F, Wu SX, Lu LX, Lin CG, et al. Local control, survival, and late toxicities of locally advanced nasopharyngeal carcinoma treated by simultaneous modulated accelerated radiotherapy combined with cisplatin concurrent chemotherapy: long-term results of a phase 2 study. Cancer. (2011) 117:1874–83. doi: 10.1002/cncr.25754
6. Liu Z, Chen Y, Su Y, Hu X, Peng X. Nasopharyngeal carcinoma: clinical achievements and considerations among treatment options. Front Oncol. (2021) 11:635737. doi: 10.3389/fonc.2021.635737
7. Luo W. Nasopharyngeal carcinoma ecology theory: cancer as multidimensional spatiotemporal "unity of ecology and evolution" pathological ecosystem. Theranostics. (2023) 13:1607–31. doi: 10.7150/thno.82690
8. Bergers G, Benjamin LE. Tumorigenesis and the angiogenic switch. Nat Rev Cancer. (2003) 3:401–10. doi: 10.1038/nrc1093
9. Jiang X, Wang J, Deng X, Xiong F, Zhang S, Gong Z, et al. The role of microenvironment in tumor angiogenesis. J Exp Clin Cancer Res CR. (2020) 39:204. doi: 10.1186/s13046-020-01709-5
10. Carmeliet P, Jain RK. Molecular mechanisms and clinical applications of angiogenesis. Nature. (2011) 473:298–307. doi: 10.1038/nature10144
11. Zhang MX, Li J, Shen GP, Zou X, Xu JJ, Jiang R, et al. Intensity-modulated radiotherapy prolongs the survival of patients with nasopharyngeal carcinoma compared with conventional two-dimensional radiotherapy: A 10-year experience with a large cohort and long follow-up. Eur J Cancer (Oxford Engl 1990). (2015) 51:2587–95. doi: 10.1016/j.ejca.2015.08.006
12. Zhuo X, Chang A, Huang C, Yang L, Xiang Z, Zhou Y. Expression and clinical significance of microvessel density and its association with TWIST in nasopharyngeal carcinoma. Int J Clin Exp Med. (2015) 8:1265–70.
13. Apte RS, Chen DS, Ferrara N. VEGF in signaling and disease: beyond discovery and development. Cell. (2019) 176:1248–64. doi: 10.1016/j.cell.2019.01.021
14. Lv X, Xiang YQ, Cao SM, Qian CN, Li NW, Guo L, et al. Prospective validation of the prognostic value of elevated serum vascular endothelial growth factor in patients with nasopharyngeal carcinoma: more distant metastases and shorter overall survival after treatment. Head Neck. (2011) 33:780–5. doi: 10.1002/hed.21541
15. Pan J, Tang T, Xu L, Lu JJ, Lin S, Qiu S, et al. Prognostic significance of expression of cyclooxygenase-2, vascular endothelial growth factor, and epidermal growth factor receptor in nasopharyngeal carcinoma. Head Neck. (2013) 35:1238–47. doi: 10.1002/hed.23116
16. Qian CN, Zhang CQ, Guo X, Hong MH, Cao SM, Mai WY, et al. Elevation of serum vascular endothelial growth factor in male patients with metastatic nasopharyngeal carcinoma. Cancer. (2000) 88:255–61. doi: 10.1002/(SICI)1097-0142(20000115)88:2<255::AID-CNCR2>3.3.CO;2-E
17. Wang F, Peng L, Wang Y, Liu X. A meta-analysis of vascular endothelial growth factor for nasopharyngeal cancer prognosis. Front Oncol. (2018) 8:486. doi: 10.3389/fonc.2018.00486
18. Li YH, Hu CF, Shao Q, Huang MY, Hou JH, Xie D, et al. Elevated expressions of survivin and VEGF protein are strong independent predictors of survival in advanced nasopharyngeal carcinoma. J Trans Med. (2008) 6:1. doi: 10.1186/1479-5876-6-1
19. Zhang Q, Wu J, Bai X, Liang T. Evaluation of intra-tumoral vascularization in hepatocellular carcinomas. Front Med. (2020) 7:584250. doi: 10.3389/fmed.2020.584250
20. Guang-Wu H, Sunagawa M, Jie-En L, Shimada S, Gang Z, Tokeshi Y, et al. The relationship between microvessel density, the expression of vascular endothelial growth factor (VEGF), and the extension of nasopharyngeal carcinoma. Laryngoscope. (2000) 110:2066–9. doi: 10.1097/00005537-200012000-00017
21. Chen L, Lin G, Chen K, Liang R, Wan F, Zhang C, et al. VEGF promotes migration and invasion by regulating EMT and MMPs in nasopharyngeal carcinoma. J Cancer. (2020) 11:7291–301. doi: 10.7150/jca.46429
22. Duan B, Shi S, Yue H, You B, Shan Y, Zhu Z, et al. Exosomal miR-17-5p promotes angiogenesis in nasopharyngeal carcinoma via targeting BAMBI. J Cancer. (2019) 10:6681–92. doi: 10.7150/jca.30757
23. Zhou T, Zhao S, Tang S, Wang Y, Wu R, Zeng X, et al. Guggulsterone promotes nasopharyngeal carcinoma cells exosomal circfip1L1 to mediate miR-125a-5p/VEGFA affecting tumor angiogenesis. Curr Mol Pharmacol. (2023) 16:870–80. doi: 10.2174/1874467216666230111112116
24. Ye J, Huang J, He Q, Zhao W, Zhou X, Zhang Z, et al. Blockage of store-operated Ca(2+) entry antagonizes Epstein-Barr virus-promoted angiogenesis by inhibiting Ca(2+) signaling-regulated VEGF production in nasopharyngeal carcinoma. Cancer Manage Res. (2018) 10:1115–24. doi: 10.2147/CMAR
25. O'Neil JD, Owen TJ, Wood VHJ, Date KL, Valentine R, Chukwuma MB, et al. Epstein-Barr virus-encoded EBNA1 modulates the AP-1 transcription factor pathway in nasopharyngeal carcinoma cells and enhances angiogenesis in vitro. J Gen Virol. (2008) 89:2833–42. doi: 10.1099/vir.0.2008/003392-0
26. Segawa Y, Oda Y, Yamamoto H, Shiratsuchi H, Hirakawa N, Komune S, et al. Close correlation between CXCR4 and VEGF expression and their prognostic implications in nasopharyngeal carcinoma. Oncol Rep. (2009) 21:1197–202. doi: 10.3892/or
27. Shi D, Guo W, Chen W, Fu L, Wang J, Tian Y, et al. Nicotine promotes proliferation of human nasopharyngeal carcinoma cells by regulating α7AChR, ERK, HIF-1α and VEGF/PEDF signaling. PloS One. (2012) 7:e43898. doi: 10.1371/journal.pone.0043898
28. Chen Z, Xu XH. Combining antiangiogenic therapy and radiation in nasopharyngeal carcinoma. Saudi Med J. (2015) 36:659–64. doi: 10.15537/smj.2015.6.11460
29. Liu S, Wu F, Zhang Y, Qin R, Zhu N, Li Y, et al. Apatinib combined with radiotherapy enhances antitumor effects in an in vivo nasopharyngeal carcinoma model. Cancer control J Moffitt Cancer Center. (2020) 27:1073274820922553. doi: 10.1177/1073274820922553
30. Huang DY, Dai ZR, Li WM, Wang RG, Yang SM. Inhibition of EGF expression and NF-κB activity by treatment with quercetin leads to suppression of angiogenesis in nasopharyngeal carcinoma. Saudi J Biol Sci. (2018) 25:826–31. doi: 10.1016/j.sjbs.2016.11.011
31. Sun Q, Wang Y, Ji H, Sun X, Xie S, Chen L, et al. Lenvatinib for effectively treating antiangiogenic drug-resistant nasopharyngeal carcinoma. Cell Death disease. (2022) 13:724. doi: 10.1038/s41419-022-05171-3
32. Hui EP, Lui VW, Wong CS, Ma BB, Lau CP, Cheung CS, et al. Preclinical evaluation of sunitinib as single agent or in combination with chemotherapy in nasopharyngeal carcinoma. Investigational New Drugs. (2011) 29:1123–31. doi: 10.1007/s10637-010-9451-1
33. Tan J, Jiang L, Cheng X, Wang C, Chen J, Huang X, et al. Association between VEGF-460T/C gene polymorphism and clinical outcomes of nasopharyngeal carcinoma treated with intensity-modulated radiation therapy. OncoTargets Ther. (2017) 10:909–18. doi: 10.2147/OTT
34. Wang T, Hu K, Ren J, Zhu Q, Wu G, Peng G. Polymorphism of VEGF-2578C/A associated with the risk and aggressiveness of nasopharyngeal carcinoma in a Chinese population. Mol Biol Rep. (2010) 37:59–65. doi: 10.1007/s11033-009-9526-2
35. Fu WM, Lu YF, Hu BG, Liang WC, Zhu X, Yang HD, et al. Long noncoding RNA Hotair mediated angiogenesis in nasopharyngeal carcinoma by direct and indirect signaling pathways. Oncotarget. (2016) 7:4712–23. doi: 10.18632/oncotarget.v7i4
36. Chen HH, Weng BQ, Cheng KJ, Liu HY, Wang SQ, Lu YY. Effect of the vascular endothelial growth factor expression level on angiopoietin-2-mediated nasopharyngeal carcinoma growth. Vasc Cell. (2014) 6:4. doi: 10.1186/2045-824X-6-4
37. Pei N, Wan R, Chen X, Li A, Zhang Y, Li J, et al. Angiotensin-(1-7) decreases cell growth and angiogenesis of human nasopharyngeal carcinoma xenografts. Mol Cancer Ther. (2016) 15:37–47. doi: 10.1158/1535-7163.MCT-14-0981
38. Xiang T, Lin YX, Ma W, Zhang HJ, Chen KM, He GP, et al. Vasculogenic mimicry formation in EBV-associated epithelial Malignancies. Nat Commun. (2018) 9:5009. doi: 10.1038/s41467-018-07308-5
39. Lyu X, Wang J, Guo X, Wu G, Jiao Y, Faleti OD, et al. EBV-miR-BART1-5P activates AMPK/mTOR/HIF1 pathway via a PTEN independent manner to promote glycolysis and angiogenesis in nasopharyngeal carcinoma. PLoS Pathog. (2018) 14:e1007484. doi: 10.1371/journal.ppat.1007484
40. Tian X, Liu Y, Wang Z, Wu S. miR-144 delivered by nasopharyngeal carcinoma-derived EVs stimulates angiogenesis through the FBXW7/HIF-1α/VEGF-A axis. Mol Ther Nucleic Acids. (2021) 24:1000–11. doi: 10.1016/j.omtn.2021.03.016
41. Ma W, Feng L, Zhang S, Zhang H, Zhang X, Qi X, et al. Induction of chemokine (C-C motif) ligand 5 by Epstein-Barr virus infection enhances tumor angiogenesis in nasopharyngeal carcinoma. Cancer Sci. (2018) 109:1710–22. doi: 10.1111/cas.13584
42. Jiang L, Wang P, Chen L, Chen H. Down-regulation of FoxM1 by thiostrepton or small interfering RNA inhibits proliferation, transformation ability and angiogenesis, and induces apoptosis of nasopharyngeal carcinoma cells. Int J Clin Exp Pathol. (2014) 7:5450–60.
43. Wang J, Jiang Q, Faleti OD, Tsang CM, Zhao M, Wu G, et al. Exosomal delivery of antagomiRs targeting viral and cellular microRNAs synergistically inhibits cancer angiogenesis. Mol Ther Nucleic Acids. (2020) 22:153–65. doi: 10.1016/j.omtn.2020.08.017
44. Pan WL, Wong JH, Fang EF, Chan YS, Ng TB, Cheung RC. Preferential cytotoxicity of the type I ribosome inactivating protein alpha-momorcharin on human nasopharyngeal carcinoma cells under normoxia and hypoxia. Biochem Pharmacol. (2014) 89:329–39. doi: 10.1016/j.bcp.2014.03.004
45. Cabral-Pacheco GA, Garza-Veloz I, Castruita-De la Rosa C, Ramirez-Acuña JM, Perez-Romero BA, Guerrero-Rodriguez JF, et al. The roles of matrix metalloproteinases and their inhibitors in human diseases. Int J Mol Sci. (2020) 21(24):9739. doi: 10.3390/ijms21249739
46. You Y, Shan Y, Chen J, Yue H, You B, Shi S, et al. Matrix metalloproteinase 13-containing exosomes promote nasopharyngeal carcinoma metastasis. Cancer Sci. (2015) 106:1669–77. doi: 10.1111/cas.12818
47. Li Z, Bian LJ, Li Y, Liang YJ, Liang HZ. Expression of protease-activated receptor-2 (PAR-2) in patients with nasopharyngeal carcinoma: correlation with clinicopathological features and prognosis. Pathol Res Pract. (2009) 205:542–50. doi: 10.1016/j.prp.2009.01.015
48. Zhao W, Ma N, Wang S, Mo Y, Zhang Z, Huang G, et al. RERG suppresses cell proliferation, migration and angiogenesis through ERK/NF-κB signaling pathway in nasopharyngeal carcinoma. J Exp Clin Cancer Res CR. (2017) 36:88. doi: 10.1186/s13046-017-0565-6
49. Chen J, Kwong DL, Zhu CL, Chen LL, Dong SS, Zhang LY, et al. RBMS3 at 3p24 inhibits nasopharyngeal carcinoma development via inhibiting cell proliferation, angiogenesis, and inducing apoptosis. PloS One. (2012) 7:e44636. doi: 10.1371/journal.pone.0044636
50. Lo PH, Lung HL, Cheung AK, Apte SS, Chan KW, Kwong FM, et al. Extracellular protease ADAMTS9 suppresses esophageal and nasopharyngeal carcinoma tumor formation by inhibiting angiogenesis. Cancer Res. (2010) 70:5567–76. doi: 10.1158/0008-5472.CAN-09-4510
51. Kan R, Shuen WH, Lung HL, Cheung AK, Dai W, Kwong DL, et al. NF-κB p65 subunit is modulated by latent transforming growth factor-β Binding protein 2 (LTBP2) in nasopharyngeal carcinoma HONE1 and HK1 cells. PloS One. (2015) 10:e0127239. doi: 10.1371/journal.pone.0127239
52. Deng M, Dai W, Yu VZ, Tao L, Lung ML. Cylindromatosis lysine 63 deubiquitinase (CYLD) regulates NF-kB signaling pathway and modulates fibroblast and endothelial cells recruitment in nasopharyngeal carcinoma. Cancers. (2020) 12(7):1924. doi: 10.3390/cancers12071924
53. Phoon YP, Cheung AK, Cheung FM, Chan KF, Wong S, Wong BW, et al. IKBB tumor suppressive role in nasopharyngeal carcinoma via NF-κB-mediated signalling. Int J Cancer. (2016) 138:160–70. doi: 10.1002/ijc.29702
54. Gong Y, Luo X, Yang J, Jiang Q, Liu Z. RIPK4 promoted the tumorigenicity of nasopharyngeal carcinoma cells. Biomed Pharmacother = Biomedecine pharmacotherapie. (2018) 108:1–6. doi: 10.1016/j.biopha.2018.08.147
55. Peng X, Zhou Y, Tao Y, Liu S. Nasopharyngeal carcinoma: the role of the EGFR in epstein-barr virus infection. Pathogens (Basel Switzerland). (2021) 10(9):1113. doi: 10.3390/pathogens10091113
56. Ren Q, Sato H, Murono S, Furukawa M, Yoshizaki T. Epstein-Barr virus (EBV) latent membrane protein 1 induces interleukin-8 through the nuclear factor-kappa B signaling pathway in EBV-infected nasopharyngeal carcinoma cell line. Laryngoscope. (2004) 114:855–9. doi: 10.1097/00005537-200405000-00012
57. Chen X, Weng Y, Li Y, Fu W, Huang Z, Pan Y, et al. Upregulation of PNCK promotes metastasis and angiogenesis via activating NF-κB/VEGF pathway in nasopharyngeal carcinoma. J Oncol. (2022) 2022:8541582. doi: 10.1155/2022/8541582
58. Chen WJ, Xu WN, Wang HY, Chen XX, Li XQ, Xie SH, et al. Plasma Epstein-Barr virus DNA and risk of nasopharyngeal carcinoma in a prospective seropositive population. BMC cancer. (2021) 21:651. doi: 10.1186/s12885-021-08408-0
59. Ye J, Wei J, Luo Y, Deng Y, Que T, Zhang X, et al. Epstein-barr virus promotes tumor angiogenesis by activating STIM1-dependent Ca(2+) signaling in nasopharyngeal carcinoma. Pathogens (Basel Switzerland). (2021) 10(10):1275. doi: 10.3390/pathogens10101275
60. Liao WH, Yang LF, Liu XY, Zhou GF, Jiang WZ, Hou BL, et al. DCE-MRI assessment of the effect of Epstein-Barr virus-encoded latent membrane protein-1 targeted DNAzyme on tumor vasculature in patients with nasopharyngeal carcinomas. BMC Cancer. (2014) 14:835. doi: 10.1186/1471-2407-14-835
61. Xu S, Bai J, Zhuan Z, Li B, Zhang Z, Wu X, et al. EBV−LMP1 is involved in vasculogenic mimicry formation via VEGFA/VEGFR1 signaling in nasopharyngeal carcinoma. Oncol Rep. (2018) 40:377–84. doi: 10.3892/or
62. Wang Z, Luo F, Li L, Yang L, Hu D, Ma X, et al. STAT3 activation induced by Epstein-Barr virus latent membrane protein1 causes vascular endothelial growth factor expression and cellular invasiveness via JAK3 And ERK signaling. Eur J Cancer (Oxford Engl 1990). (2010) 46:2996–3006. doi: 10.1016/j.ejca.2010.07.008
63. Murono S, Inoue H, Tanabe T, Joab I, Yoshizaki T, Furukawa M, et al. Induction of cyclooxygenase-2 by Epstein-Barr virus latent membrane protein 1 is involved in vascular endothelial growth factor production in nasopharyngeal carcinoma cells. Proc Natl Acad Sci USA. (2001) 98:6905–10. doi: 10.1073/pnas.121016998
64. Cheng S, Li Z, He J, Fu S, Duan Y, Zhou Q, et al. Epstein-Barr virus noncoding RNAs from the extracellular vesicles of nasopharyngeal carcinoma (NPC) cells promote angiogenesis via TLR3/RIG-I-mediated VCAM-1 expression. Biochim Biophys Acta Mol Basis Dis. (2019) 1865:1201–13. doi: 10.1016/j.bbadis.2019.01.015
65. Kalluri R, LeBleu VS. The biology, function, and biomedical applications of exosomes. Science (New York NY). (2020) 367(6478):eaau6977. doi: 10.1126/science.aau6977
66. Liu Y, Wen J, Huang W. Exosomes in nasopharyngeal carcinoma. Clinica chimica acta; Int J Clin Chem. (2021) 523:355–64. doi: 10.1016/j.cca.2021.10.013
67. Zhang S, Cai J, Ji Y, Zhou S, Miao M, Zhu R, et al. Tumor-derived exosomal lincRNA ROR promotes angiogenesis in nasopharyngeal carcinoma. Mol Cell Probes. (2022) 66:101868. doi: 10.1016/j.mcp.2022.101868
68. Yang W, Tan S, Yang L, Chen X, Yang R, Oyang L, et al. Exosomal miR-205-5p enhances angiogenesis and nasopharyngeal carcinoma metastasis by targeting desmocollin-2. Mol Ther Oncolytics. (2022) 24:612–23. doi: 10.1016/j.omto.2022.02.008
69. Bao L, You B, Shi S, Shan Y, Zhang Q, Yue H, et al. Metastasis-associated miR-23a from nasopharyngeal carcinoma-derived exosomes mediates angiogenesis by repressing a novel target gene TSGA10. Oncogene. (2018) 37:2873–89. doi: 10.1038/s41388-018-0183-6
70. Lu J, Liu QH, Wang F, Tan JJ, Deng YQ, Peng XH, et al. Exosomal miR-9 inhibits angiogenesis by targeting MDK and regulating PDK/AKT pathway in nasopharyngeal carcinoma. J Exp Clin Cancer Res CR. (2018) 37:147. doi: 10.1186/s13046-018-0814-3
71. You B, Pan S, Gu M, Zhang K, Xia T, Zhang S, et al. Extracellular vesicles rich in HAX1 promote angiogenesis by modulating ITGB6 translation. J Extracell Vesicles. (2022) 11:e12221. doi: 10.1002/jev2.12221
72. You B, Cao X, Shao X, Ni H, Shi S, Shan Y, et al. Clinical and biological significance of HAX-1 overexpression in nasopharyngeal carcinoma. Oncotarget. (2016) 7:12505–24. doi: 10.18632/oncotarget.v7i11
73. Zhang K, Liu D, Zhao J, Shi S, He X, Da P, et al. Nuclear exosome HMGB3 secreted by nasopharyngeal carcinoma cells promotes tumour metastasis by inducing angiogenesis. Cell Death Dis. (2021) 12:554. doi: 10.1038/s41419-021-03845-y
74. Wu A, Luo N, Xu Y, Du N, Li L, Liu Q. Exosomal LBH inhibits epithelial-mesenchymal transition and angiogenesis in nasopharyngeal carcinoma via downregulating VEGFA signaling. Int J Biol Sci. (2022) 18:242–60. doi: 10.7150/ijbs.66506
75. Gu M, Li L, Zhang Z, Chen J, Zhang W, Zhang J, et al. PFKFB3 promotes proliferation, migration and angiogenesis in nasopharyngeal carcinoma. J Cancer. (2017) 8:3887–96. doi: 10.7150/jca.19112
76. Chan YK, Zhang H, Liu P, Tsao SW, Lung ML, Mak NK, et al. Proteomic analysis of exosomes from nasopharyngeal carcinoma cell identifies intercellular transfer of angiogenic proteins. Int J Cancer. (2015) 137:1830–41. doi: 10.1002/ijc.29562
77. Chen X, Wu G, Qing J, Li C, Chen X, Shen J. LINC00240 knockdown inhibits nasopharyngeal carcinoma progress by targeting miR-26a-5p. J Clin Lab analysis. (2022) 36:e24424. doi: 10.1002/jcla.24424
78. Lu J, Zhao FP, Peng Z, Zhang MW, Lin SX, Liang BJ, et al. EZH2 promotes angiogenesis through inhibition of miR-1/Endothelin-1 axis in nasopharyngeal carcinoma. Oncotarget. (2014) 5:11319–32. doi: 10.18632/oncotarget.v5i22
79. Zhang Q, Lu D, Liu W, Ye S, Guo H, Liao T, et al. Effects of KIF2A on the prognosis of nasopharyngeal carcinoma and nasopharyngeal carcinoma cells. Oncol Lett. (2019) 18:2718–23. doi: 10.3892/ol
80. Wang W, Yi M, Zhang R, Li J, Chen S, Cai J, et al. Vimentin is a crucial target for anti-metastasis therapy of nasopharyngeal carcinoma. Mol Cell Biochem. (2018) 438:47–57. doi: 10.1007/s11010-017-3112-z
81. Wang P, Shen N, Liu D, Ning X, Wu D, Huang X. TRIM24 siRNA induced cell apoptosis and reduced cell viability in human nasopharyngeal carcinoma cells. Mol Med Rep. (2018) 18:369–76. doi: 10.3892/mmr
82. Bao L, Tang M, Zhang Q, You B, Shan Y, Shi S, et al. Elevated expression of CD93 promotes angiogenesis and tumor growth in nasopharyngeal carcinoma. Biochem Biophys Res Commun. (2016) 476:467–74. doi: 10.1016/j.bbrc.2016.05.146
83. Chen CY, Lin YS, Chen CL, Chao PZ, Chiou JF, Kuo CC, et al. Targeting annexin A2 reduces tumorigenesis and therapeutic resistance of nasopharyngeal carcinoma. Oncotarget. (2015) 6:26946–59. doi: 10.18632/oncotarget.v6i29
84. Liu YC, Chen YJ, Wu GJ. METCAM/MUC18 plays a tumor suppressor role in the development of nasopharyngeal carcinoma type I. Int J Mol Sci. (2022) 23(21):13389. doi: 10.3390/ijms232113389
85. Wang Y, Yang J, Sheng W, Xie Y, Liu J. Adenovirus-mediated ING4/PTEN double tumor suppressor gene co-transfer modified by RGD enhances antitumor activity in human nasopharyngeal carcinoma cells. Int J Oncol. (2015) 46:1295–303. doi: 10.3892/ijo.2015.2822
86. Deng L, Li Y, Ai P, Xie Y, Zhu H, Chen N. Increase in IkappaB kinase alpha expression suppresses the tumor progression and improves the prognosis for nasopharyngeal carcinoma. Mol Carcinogenesis. (2015) 54:156–65. doi: 10.1002/mc.v54.2
87. Cheung AK, Ip JC, Chu AC, Cheng Y, Leong MM, Ko JM, et al. PTPRG suppresses tumor growth and invasion via inhibition of Akt signaling in nasopharyngeal carcinoma. Oncotarget. (2015) 6:13434–47. doi: 10.18632/oncotarget.v6i15
88. Law EW, Cheung AK, Kashuba VI, Pavlova TV, Zabarovsky ER, Lung HL, et al. Anti-angiogenic and tumor-suppressive roles of candidate tumor-suppressor gene, Fibulin-2, in nasopharyngeal carcinoma. Oncogene. (2012) 31:728–38. doi: 10.1038/onc.2011.272
89. Chen H, Ko JM, Wong VC, Hyytiainen M, Keski-Oja J, Chua D, et al. LTBP-2 confers pleiotropic suppression and promotes dormancy in a growth factor permissive microenvironment in nasopharyngeal carcinoma. Cancer Lett. (2012) 325:89–98. doi: 10.1016/j.canlet.2012.06.005
90. Hong B, Lui VW, Hashiguchi M, Hui EP, Chan AT. Targeting tumor hypoxia in nasopharyngeal carcinoma. Head Neck. (2013) 35:133–45. doi: 10.1002/hed.21877
91. Liu L, Hu L, Zeng Q, Peng D, Chen Z, Huang C, et al. Dynamic contrast-enhanced MRI of nasopharyngeal carcinoma: correlation of quantitative dynamic contrast-enhanced magnetic resonance imaging (DCE-MRI) parameters with hypoxia-inducible factor 1α expression and tumor grade/stage. Ann palliative Med. (2021) 10:2238–53. doi: 10.21037/apm
92. Hu Y, E H, Yu X, Li F, Zeng L, Lu Q, et al. Correlation of quantitative parameters of magnetic resonance perfusion-weighted imaging with vascular endothelial growth factor, microvessel density and hypoxia-inducible factor-1α in nasopharyngeal carcinoma: Evaluation on radiosensitivity study. Clin Otolaryngol. (2018) 43:425–33. doi: 10.1111/coa.12982
93. Zhang SX, Jia QJ, Zhang ZP, Liang CH, Chen WB, Qiu QH, et al. Intravoxel incoherent motion MRI: emerging applications for nasopharyngeal carcinoma at the primary site. Eur radiology. (2014) 24:1998–2004. doi: 10.1007/s00330-014-3203-0
94. Chen WB, Zhang B, Liang L, Dong YH, Cai GH, Liang CH, et al. To predict the radiosensitivity of nasopharyngeal carcinoma using intravoxel incoherent motion MRI at 3.0 T. Oncotarget. (2017) 8:53740–50. doi: 10.18632/oncotarget.v8i32
95. Liang S, Gao Y, Liu Y, Qiu C, Chen Y, Zhu S. Contrast-enhanced Ultrasound in evaluating of angiogenesis and tumor staging of nasopharyngeal carcinoma in nude mice. PloS One. (2019) 14:e0221638. doi: 10.1371/journal.pone.0221638
96. Yang Z, Kang M, Zhu S, Huang J, Li X, Wang R. Clinical evaluation of vascular normalization induced by recombinant human endostatin in nasopharyngeal carcinoma via dynamic contrast-enhanced ultrasonography. OncoTargets Ther. (2018) 11:7909–17. doi: 10.2147/OTT
97. Chong WQ, Lim CM, Sinha AK, Tan CS, Chan GHJ, Huang Y, et al. Integration of antiangiogenic therapy with cisplatin and gemcitabine chemotherapy in patients with nasopharyngeal carcinoma. Clin Cancer Res. (2020) 26:5320–8. doi: 10.1158/1078-0432.CCR-20-1727
98. Zhang HJ, Yuan GL, Liang QL, Peng XX, Cheng SA, Jiang L. Addition of bevacizumab to systemic therapy for locally advanced and metastatic nasopharyngeal carcinoma. Oncol Lett. (2018) 15:7799–805. doi: 10.3892/ol
99. Lee NY, Harris J, Kim J, Garden A, Mechalakos J, Pfister DG, et al. Long-term outcomes of bevacizumab and chemoradiation for locoregionally advanced nasopharyngeal carcinoma: A nonrandomized controlled trial. JAMA Netw Open. (2023) 6:e2316094. doi: 10.1001/jamanetworkopen.2023.16094
100. Zhou T, Yang Y, Ma S, Lin L, Zhou T, Zhang C, et al. Bevacizumab versus placebo in combination with paclitaxel and carboplatin as first-line treatment for recurrent or metastatic nasopharyngeal carcinoma: a multicentre, randomised, open-label, phase II trial. ESMO Open. (2021) 6:100313. doi: 10.1016/j.esmoop.2021.100313
101. Jin T, Li B, Chen XZ. A phase II trial of Endostar combined with gemcitabine and cisplatin chemotherapy in patients with metastatic nasopharyngeal carcinoma (NCT01612286). Oncol Res. (2013) 21:317–23. doi: 10.3727/096504014X13983417587401
102. Gong X, Wang L, Wu W, Li Y, Long J, Chen X, et al. The treatment combining antiangiogenesis with chemoradiotherapy impinges on the peripheral circulation vascular endothelial cells and therapeutic effect in the patients with locally advanced nasopharyngeal carcinoma. BioMed Res Int. (2022) 2022:1787854. doi: 10.1155/2022/1787854
103. Li K, Shi M, Qin S. Current status and study progress of recombinant human endostatin in cancer treatment. Oncol Ther. (2018) 6:21–43. doi: 10.1007/s40487-017-0055-1
104. Xu L, Li D, Ji J, Chen Z, Tang X, Chen D, et al. Recombinant human endostatin injection (Endostar) combined with PF chemotherapy and sequential intensity-modulated radiotherapy is tolerable and improves prognosis of locally advanced nasopharyngeal carcinoma: a randomized, open, multicenter phase II clinical study. Am J Cancer Res. (2022) 12:4622–36.
105. Zhou J, Wang L, Xu X, Tu Y, Qin S, Yin Y. Antitumor activity of Endostar combined with radiation against human nasopharyngeal carcinoma in mouse xenograft models. Oncol Lett. (2012) 4:976–80. doi: 10.3892/ol.2012.856
106. Peng F, Xu Z, Wang J, Chen Y, Li Q, Zuo Y, et al. Recombinant human endostatin normalizes tumor vasculature and enhances radiation response in xenografted human nasopharyngeal carcinoma models. PloS One. (2012) 7:e34646. doi: 10.1371/journal.pone.0034646
107. Jin J, Du J, Wu Y. Apatinib monotherapy for advanced VEGFR-2-negative nasopharyngeal carcinoma: A case report. Medicine. (2019) 98:e13491. doi: 10.1097/MD.0000000000013491
108. Peng QX, Han YW, Zhang YL, Hu J, Fan J, Fu SZ, et al. Apatinib inhibits VEGFR-2 and angiogenesis in an in vivo murine model of nasopharyngeal carcinoma. Oncotarget. (2017) 8:52813–22. doi: 10.18632/oncotarget.v8i32
109. Mu X, Ma J, Zhang Z, Zhou H, Xu S, Qin Y, et al. Famitinib enhances nasopharyngeal cancer cell radiosensitivity by attenuating radiation-induced phosphorylation of platelet-derived growth factor receptor and c-kit and inhibiting microvessel formation. Int J Radiat Biol. (2015) 91:771–6. doi: 10.3109/09553002.2015.1062574
110. Cao LH, Li HT, Lin WQ, Tan HY, Xie L, Zhong ZJ, et al. Morphine, a potential antagonist of cisplatin cytotoxicity, inhibits cisplatin-induced apoptosis and suppression of tumor growth in nasopharyngeal carcinoma xenografts. Sci Rep. (2016) 6:18706. doi: 10.1038/srep18706
111. Lin YT, Wang HC, Tsai MH, Su YY, Yang MY, Chien CY. Angiotensin II receptor blockers valsartan and losartan improve survival rate clinically and suppress tumor growth via apoptosis related to PI3K/AKT signaling in nasopharyngeal carcinoma. Cancer. (2021) 127:1606–19. doi: 10.1002/cncr.33391
112. Zhai S, Huang Q, Liao X, Yin S. Study on the drug targets and molecular mechanisms of rhizoma curcumae in the treatment of nasopharyngeal carcinoma based on network pharmacology. Evid Based Complement Alternat Med eCAM. (2020) 2020:2606402. doi: 10.1155/2020/2606402
113. Yanwei L, Yinli Y, Pan Z. Traditional herbal formula NPC01 exerts antiangiogenic effects through inhibiting the PI3K/akt/mTOR signaling pathway in nasopharyngeal carcinoma cells. Evid Based Complement Alternat Med eCAM. (2018) 2018:5291517. doi: 10.1155/2018/5291517
114. Zhang W, Kang M, Zhang T, Li B, Liao X, Wang R. Triptolide combined with radiotherapy for the treatment of nasopharyngeal carcinoma via NF-κB-related mechanism. Int J Mol Sci. (2016) 17(12):2139. doi: 10.3390/ijms17122139
Keywords: nasopharyngeal carcinoma, angiogenesis, Epstein–Barr virus, exosomes, anti-angiogenic therapy
Citation: Xia C, Zhao J, Huang Y, Miao H and Zhao F (2024) Angiogenesis in nasopharyngeal carcinoma: insights, imaging, and therapeutic strategies. Front. Oncol. 14:1331064. doi: 10.3389/fonc.2024.1331064
Received: 31 October 2023; Accepted: 09 May 2024;
Published: 28 May 2024.
Edited by:
Sharon R. Pine, University of Colorado, United StatesReviewed by:
Weiren Luo, The Second Affiliated hospital of Southern University of Science and Technology, ChinaWenjun Liao, Sichuan Cancer Hospital, China
Copyright © 2024 Xia, Zhao, Huang, Miao and Zhao. This is an open-access article distributed under the terms of the Creative Commons Attribution License (CC BY). The use, distribution or reproduction in other forums is permitted, provided the original author(s) and the copyright owner(s) are credited and that the original publication in this journal is cited, in accordance with accepted academic practice. No use, distribution or reproduction is permitted which does not comply with these terms.
*Correspondence: Feipeng Zhao, emhhb2ZlaXBlbmcwMDhAc3dtdS5lZHUuY24=
†These authors have contributed equally to this work