- Department of Otolaryngology-Head and Neck Surgery, Affiliated Hospital of North Sichuan Medical College, Nanchong, China
Histones have a vital function as components of nucleosomes, which serve as the fundamental building blocks of chromatin. Histone deacetylases (HDACs), which target histones, suppress gene transcription by compacting chromatin. This implies that HDACs have a strong connection to the suppression of gene transcription. Histone deacetylase 7 (HDAC7), a member of the histone deacetylase family, may participate in multiple cellular pathophysiological processes and activate relevant signaling pathways to facilitate the progression of different tumors by exerting deacetylation. In recent years, HDAC7 has been increasingly studied in the pathogenesis of tumors. Studies that are pertinent have indicated that it has a significant impact on the growth and metastasis of tumors, the formation of the vascular microenvironment, and the emergence of resistance to drugs. Therefore, HDAC7 could potentially function as a potent predictor for tumor prognosis and a promising target for mitigating drug resistance in tumors. This review primarily concentrates on elucidating the structure and function of HDAC7, its involvement in the development of various tumors, and its interplay with relevant signaling pathways. Meanwhile, we briefly discuss the research direction and prospect of HDAC7.
1 Introduction
Histones, which are present in both eukaryotic chromatin and prokaryotic basic proteins, could associate with DNA to form nucleosomes and play a regulatory role in gene expression within the cell nucleus (1). When engaged in histone modification, HDACs catalyze lysine deacetylation to compact chromatin and suppress the transcription of associated genes (2). HDAC7, one of the 18 members in the HDACs family, has been linked to significant functions in various physiological and pathological processes. Accumulating studies have shown that HDAC7 is not only involved in critical physiological processes such as immunity (3, 4), osteogenesis (5), angiogenesis (6), and energy metabolism (7), but also in the development of various diseases including tumors.
To regulate specific signaling pathways, HDAC7, which is part of the HDAC group, causes the deacetylation of β-catenin and STAT3 (8, 9). Abnormal expression of HDAC7 is associated with the prognosis of various tumors and is involved in pathophysiological processes such as tumor cell proliferation, migration, apoptosis, and angiogenesis in the tumor microenvironment. It has a vital function in the development and metastasis of tumors. In oncology therapy, the aberrant expression of HDAC7 enables the occurrence of drug resistance in colorectal cancer (10) and breast cancer (11). Vorinostat, a pan-HDAC inhibitor targeting in HDAC7 and other HDACs, has been used for the treatment of refractory cutaneous T-cell lymphoma (12). Therefore, HDAC7 has the potential to serve as a prognostic indicator and target for tumor treatment. The study of HDAC7 in tumors is growing, but there is a scarcity of comprehensive evaluations regarding the involvement of HDAC7 in tumors. This paper will review the structure, function, and role of HDAC7 in cancer.
2 HDACs and HDAC IIa
There are 18 kinds of HDACs variations in humans, categorized into four classes (I-IV) according to their similarity in sequence with the yeast counterparts Rpd3, HadI, and Sir2 (13). In terms of structure, they have a catalytic domain at the C-terminal and a regulatory domain at the N-terminal. The catalytic domain of HDACs from classes I, II, and IV includes preserved amino acid residues that bind Zn2+ through chelation. HDACs of class III contain residues that can bind with nicotinamide adenine dinucleotide, in addition to those mentioned above. One distinguishing feature of Class IIa HDACs is the presence of a nuclear export signal (NES) in the C-terminal region, while the N-terminal region is relatively larger and less conserved (Figure 1). The N-terminal region contains a nuclear localization signal (NLS) and includes a domain that interacts with members of the myocyte enhancer factor 2 (MEF2) transcription factor family (14). Class IIa HDACs are critical regulatory factors for specific developmental and differentiation processes (15).

Figure 1 Schematic view of HDAC7, HDAC7 contains two domains which is catalytic domain and non-catalytic domain. Serine(S) residue regulate HDAC7 nuclear- cytoplasmic shuttling; Histidine(H) residue is essential for enzymatic activity; MEF2 is a bind site for members of MEF2 transcription factor family; NLS, nuclear localization signal; NES, nuclear export signal; CtBP is a binding site for с terminal binding protein.
The modulation of the interaction between HDAC7 and chaperone proteins, along with the regulation of protein stability, is significantly influenced by phosphorylation, similar to other class IIa HDACs (16). Specifically, the residues S181, S155, S358, and S486 in HDAC7 are of utmost importance in these processes (17). It is worth mentioning that CaMKI can alter the last three locations (18), whereas PKD can phosphorylate all four positions (17). Interestingly, PP2A facilitates the dephosphorylation of the four 14–3-3 binding sites in HDAC7, without showing any preference for any specific site among them (19).
3 The structure and functions of HDAC7
HDAC7 is a protein that contains 912 amino acids and is encoded by the HDAC7 gene on human chromosome 12 (20). This protein is abundant in the cardiac, placental, pancreatic, and smooth muscle tissues, and can be located within the cytoplasm or nucleus (21). HDAC4, 5, 7, and 9, which are member of the group of 11 classical HDACs that rely on zinc, function as class IIa HDAC enzymes. These enzymes play a role in controlling cellular and developmental processes using both enzymatic and non-enzymatic mechanisms (22). Similar to other traditional HDACs, class IIa HDACs have a cylindrical binding pocket for substrates, which contains the zinc cofactor at its end (23). Most classical HDACs (24) rely on a tyrosine and two histidine residues within the active site to perform their enzymatic activity. Nevertheless, in class IIa HDACs, the tyrosine is replaced by a histidine, leading to a notable reduction in the enzymatic function for removing acetyl groups from lysine on particular peptide substrates (25). Recent studies have demonstrated that certain stimuli elicit the activation of class IIa HDAC enzymes within the cytosol of cells (26). There is a possibility that a connection with HDAC3, a type I HDAC, might contribute to enabling certain enzyme-mediated functions (27). Additionally, it is conceivable that class IIa HDACs may engage in alternative post-translational modifications beyond lysine deacetylation.
Similar to other HDAC IIa proteins in terms of structure, the catalytic domain of HDAC7 is located at the C-terminal, while the N-terminal contains the highly conserved regulatory domain. Phosphorylation takes place on the serine residues of N-terminal, specifically Ser155, 178, 181, 321, 344, 446, 479 (20). This phosphorylation facilitates the binding of HDAC7 and 14-3-3 proteins, enabling their involvement in nucleocytoplasmic transmembrane transport (28, 29). Consequently, cells can swiftly and reversibly adjust to new environments as per their requirements (30). Meanwhile, the C-terminal catalytic domain can interact with various molecular chaperones, such as transcription factors USF-1 (31), to regulate multiple cellular functions. HDAC7 can deacetylate both histones and non-histones and regulate multiple biological processes through deacetylation (32), such as organismal immunity (3, 4), angiogenesis (6), embryonic development (6), energy metabolism (7) and other physiological processes. Additionally, HDAC7 plays significant roles in various tumor progression processes through different signaling pathways (Figure 2). For example, it hinders the phosphorylation of β-catenin by removing the acetyl group from β-catenin, thus activating the Wnt/β-catenin pathway and facilitating the proliferation and migration of cancer cells (33) (Figure 2A).
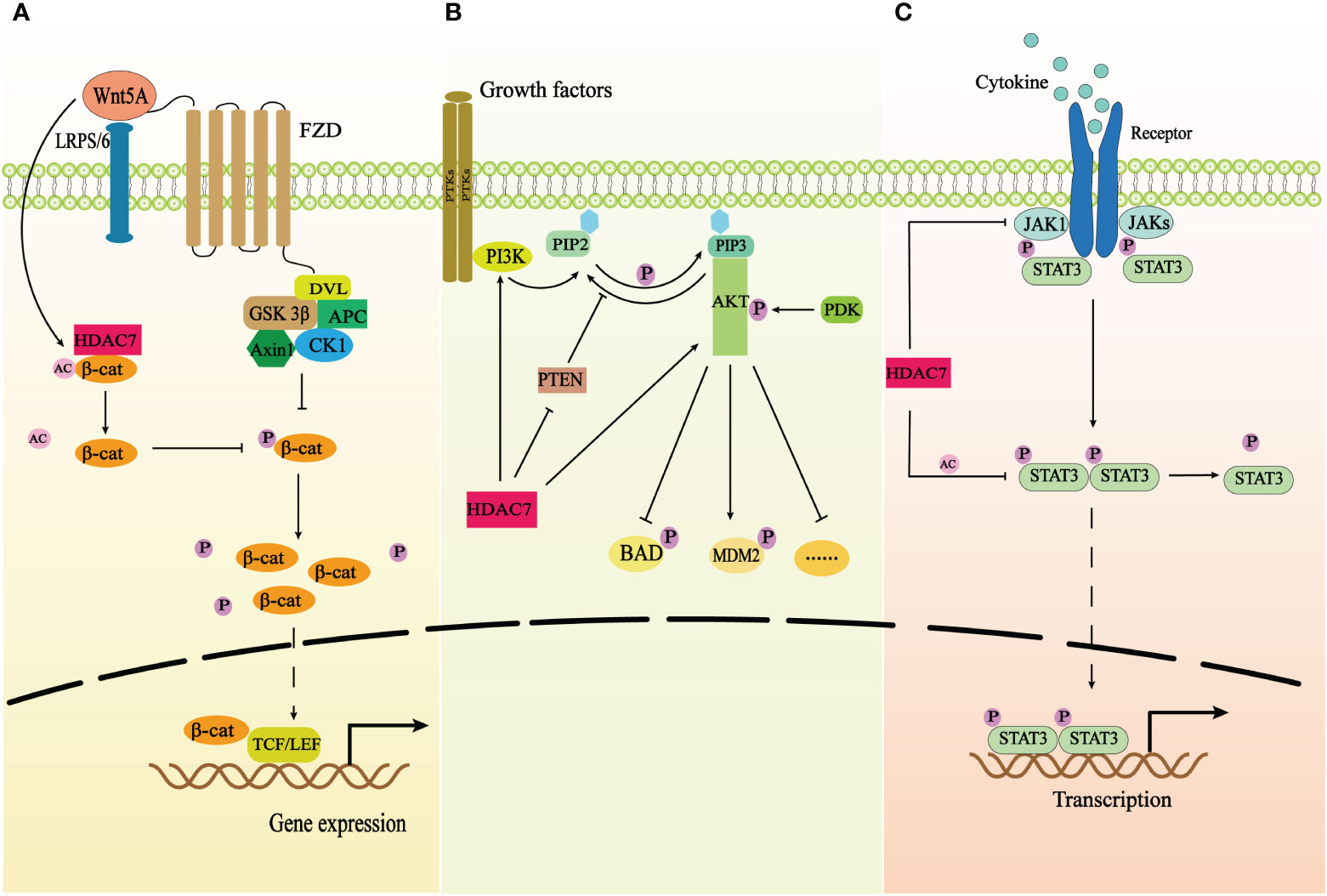
Figure 2 The correlation of HDAC7 and three signaling pathways. (A) The schematic demonstrates the correlation of HDAC7 and Wnt/β-catenin signaling pathway. HDAC7 facilitates β-catenin redistribution to the nucleus by deacetylating β-catenin and inhibiting its phosphorylation, which results in that the nuclear accumulation of β-catenin binds to TCF/LEF to activate related gene expression. Wnt5A promotes the expression of HDAC7 and exerts synergistic effects on the activation of Wnt/β-catenin pathway. (B) The schematic shows the correlation of HDAC7 and PI3K/AKT signaling pathway. HDAC7 can directly promote the expression of PI3K and AKT proteins and decrease the expression of PTEN the repressor of the PI3K/AKT signaling pathway to trigger the activation of the signaling transduction. (C) The schematic shows the correlation of HDAC7 and JAK/STAT3 signaling pathway. HDAC7 inhibits phosphorylation of STAT3 by deacetylating STAT3 and downregulating the expression of the protein kinase JAK1 to repress the activation of the signaling pathway.
4 The involvement of HDAC7 in cancer
HDAC7 was found to be aberrantly expressed in various tumors, including high expression in malignant tumors such as lung, esophageal, and gastric cancers, and low expression in acute lymphoblastic leukemia derived from B-cell progenitor cells (Table 1). The variation in HDAC7 expression was linked to clinicopathological characteristics and unfavorable prognosis in patients, impacting tumor cell proliferation, migration, and apoptosis via distinct pathological mechanisms.
4.1 Respiratory system tumors
Lung cancer is the prevailing malignant tumor within the respiratory system, with non-small cell lung cancer (NSCLC) emerging as the predominant histopathological type (41). In patients (8), it was discovered that the levels of HDAC7 protein and mRNA were elevated in lung cancer tissues, and this was associated with a poor prognosis, TNM stage, and tumor differentiation degree. In mechanistic studies, it was reported that HDAC7 promoted tumor growth by deacetylating STAT3 and inhibiting tyrosine phosphorylation in vitro, but it had no effect on the protein content of STAT3 (9). For another downstream gene, plakoglobin, the combination of HDAC7 and the promoter region of plakoglobin inhibited plakoglobin expression and promoted tumor cell growth and migration (42). OIP5-AS1, an upstream gene of HDAC7, was reported to inhibit miR-140-5p expression, thereby increasing HDAC7 expression and promoting tumor cell metastasis in NSCLC (43). Vascular endothelial progenitor cells were found to be involved in NSCLC neovascularization through HDAC7-mediated cytoskeletal regulation and angiogenic gene transcription in a study of the tumor microenvironment mechanism (44). Recently, it was reported that HDAC7 was stabilized by the deubiquitinase USP10 in NSCLC, preventing its degradation and activating the β-catenin-FGF18 pathway to promote proliferation and migration of NSCLC cells (8). The effect of HDAC7 on β-catenin is similar to that described in esophageal squamous cell carcinoma. Hence, HDAC7 might contribute to the growth and advancement of lung carcinoma via diverse mechanisms and signaling pathway, offering a conceptual foundation for the exploration of anti-cancer medications.
The pathogenesis of nasopharyngeal carcinoma (NPC) is complex. Distinct roles are played by the HDAC family members in NPC. For instance, class I HDACs demonstrate a tumor-suppressing effect (45), whereas HDAC7 shows an oncogenic effect. In NPC tissues, there was a significant overexpression of HDAC7 which led to the upregulation of EphA2. This was achieved by inhibiting the expression of miR-4465, ultimately promoting the proliferation, migration, and invasion of NPC cells in vitro (37). Given the molecular mechanism has been shown in previous studies that other members of the miRNA family inhibited HDAC7 expression by degrading HDAC7 mRNA in tumor tissues (46), while the detailed molecular mechanism that miR-4465 acts as an oncogenic factor in NPC has not been investigated, it is unclear whether miR-4465 works in the same manner as other tumors.
4.2 Digestive system tumors
The development and advancement of esophageal squamous cell carcinoma (ESCC) are linked to the accumulation of abnormal regulation of oncogenes, resembling numerous other solid tumors (47). HDAC7, one of the abnormally expressed oncogenes, was observed at higher levels in ESCC than in paraneoplastic tissue and normal esophageal mucosal tissue. The abnormal expression of HDAC7 could bind with β-catenin, leading to β-catenin Lys49 deacetylation and subsequent inhibition of β-catenin phosphorylation. Upon entering the nucleus, the β-catenin that is not phosphorylated formed a complex with the TCF4 transcription factor (33). The combination led to the generation of c-myc, which inhibits the synthesis of p21 and p27, hastening the transition from G1 to S phase (48) and ultimately promoting the proliferation of cancerous cells. This study also demonstrated that melatonin, a hormone produced by the pineal gland, could hinder the growth of ESCC tumor by reducing the activity of the HDAC7/β-catenin/c-myc positive feedback loop and suppressing the function of USP10 that is responsible for maintaining the stability of HDAC7 protein (35). An additional mechanism of ESCC has been elucidated, indicating the involvement of HDAC7 in the metastasis of tumor cells mediated by WNT5A (49). These studies reveal tumorigenesis originates from the loss of the body’s constraints on oncogenic factors. Moreover, they provide insights into how the interaction between HDAC7 and the Wnt pathway affects the growth and metastasis of ESCC. Consequently, exploring targeted drug research focused on WNT5A and HDAC7 may offer a promising therapeutic approach for ESCC.
Currently, the long-term survival rate of patients with gastric cancer following conventional treatment remains suboptimal, thereby necessitating the identification of more efficacious targeted therapeutic agents to improve the survival of such patients (50). The overexpression of HDAC7 in gastric cancer tissues compared to paraneoplastic tissues suggests that it could be a potential therapeutic target for gastric cancer. Furthermore, its abnormal expression has been associated with poor prognosis and distant metastasis in patients diagnosed with gastric cancer (39, 51). Mechanism research has shown that miR-489 is expressed in gastric cancer tissues as an upstream gene of HDAC7. Inhibition of miR-489 may enhance the levels of HDAC7 protein and mRNA expression, thereby activating the PI3K/AKT signaling pathway and facilitating the progression of gastric cancer (39). However, the detailed process which HDAC7 activates the PI3K/AKT signaling pathway has not been further investigated.
Colorectal cancer (CRC) ranks among the top three types of tumors in terms of incidence (52), manifesting diverse genetic and clinical characteristics, as well as a multifaceted pathogenesis (53). A significant increase in the HDAC7 gene expression has been revealed in recent studies of rectal biopsies from individuals with colorectal adenomas, in contrast to those without dysplasia (54). In addition, biopsies obtained from patients diagnosed with colorectal cancer (CRC) showed a comparable increase in HDAC7 expression compared to healthy colon mucosa (55). These findings strongly imply that HDAC7 holds promise as a plausible diagnostic and/or prognostic indicator for CRC. HDAC7, which was significantly upregulated in CRC tissues, could reduce histone acetylation in the promoter region of nucleoside concentrate transporter 2 (CNT2), leading to a contraction in chromatin structure and a decrease in CNT2 expression (10). Furthermore, decreased levels of histone acetylation could impede the absorption of cancerous cells. Likewise, miR-489, the upstream gene of HDAC7, was discovered to promote the proliferation and invasion of CRC cells, resembling its function in gastric cancer, throughout the malignant advancement of colorectal cancer cells (46). Moreover, HDAC7 could be regulated by the miR-140-5p upstream gene. Its overexpression increased the protein levels of PI3K and AKT, thereby inducing tumor cell invasion (38). In summary, HDAC7 exerts its oncogenic effects through different mechanisms. Additionally, it inhibits the uptake of nucleoside anticancer drugs by tumor cells, which allows the development of drug resistance in tumor therapy. Therefore, HDAC7 inhibitors can both combat tumors and reduce the development of related drug resistance.
Pancreatic carcinoma is among the most lethal types of cancer, and there is a shortage of reliable indicators to accurately differentiate between noncancerous and cancerous tumors in the pancreas. The protein and mRNA expression of HDAC7 was found to be upregulated in pancreatic cancer tissues, but the abnormal expression of HDAC7 did not exhibit a clear correlation with the clinical characteristics of patients (56). Given that this is a retrospective study, the causal relationship between the upregulation of HDAC7 and tumor progression remains uncertain. However, evidence from previous research suggests that the inhibition of HDAC7 leads to suppressed cell growth in pancreatic cancer cell lines (57), and its abnormal expression is associated with the progression of other digestive system malignancies, such as colorectal (46) and gastric cancer (39). Therefore, it is reasonable to contemplate the upregulation of HDAC7 as a possible contributing factor in the malignant advancement of cancerous tissues. Further investigation is required to establish the exact cause-and-effect connection and elucidate the intricate process by which HDAC7 promotes the advancement of pancreatic cancer.
Notably, HDAC7 exhibits significantly elevated expression levels in hepatocellular carcinoma and is correlated with poor prognosis in patients with this condition (58). However, the specific role of HDAC7 in the advancement of hepatocellular carcinoma remains unclear. It is worth mentioning that repeated liver injury induces the transformation of hepatocytes from steatosis to fibrosis. According to existing research on the pathogenesis of hepatocellular carcinoma, it has been observed that its progression ultimately culminates in the development of hepatocellular carcinoma (59). However, specific research has suggested a notable correlation between the expression of HDAC7 and collagen α-1, a fibrosis-related protein found in the extracellular matrix (60). Consequently, it is plausible to suggest that HDAC7 may potentially contribute to the pathogenic mechanisms underlying chronic liver disease and the subsequent development of hepatocellular carcinoma.
4.3 Glioma
Gliomas, originating from the epithelial cells of the central nervous system, are the prevalent tumors within the brain (61). They are highly aggressive and can directly metastasize to adjacent normal brain tissue, and standard treatment cannot achieve a favorable prognosis (62). This refers to an imbalance between histone acetylation and deacetylation regarding the mechanism of glioma development (63). HDAC7, as a histone deacetylase, had an aberrant expression that contributes to glioma development. Compared to normal brain tissue (64), gliomas exhibited an upregulation in HDAC7 mRNA levels. Silencing HDAC7 could increase STAT3 expression levels and promote STAT3 phosphorylation, inhibiting tumor angiogenesis and tumor cell growth (34). HDAC7 could inhibit the phosphorylation of STAT3 by exerting deacetylation and downregulating the expression of the protein kinase JAK1 (34). Another mechanism suggests that the zinc finger protein ZNF326 is highly expressed in gliomas and increases the transcriptional expression of HDAC7 in tumors. In the meantime, HDAC7 hinders the phosphorylation of β-catenin, enabling the entry of unphosphorylated β-catenin into the nucleus and triggering the activation of the Wnt pathway, ultimately contributing to the proliferation and invasion of glioma (33). In summary, HDAC7 promotes tumor angiogenesis and cell growth by blocking the activation of STAT3 and inhibiting β-catenin phosphorylation, which alters its cytoplasmic-nuclear redistribution and contributes to the malignant progression of glioma (62). This coincides with the highly aggressive characteristics of gliomas.
4.4 Ovarian cancer
The recurrence rate of ovarian cancer is not well controlled after first-line clinical treatment (65). The latest research indicates that the recurrence, metastasis, and occurrence of resistance to conventional chemotherapy correlates with the existence of tumor stem cells in ovarian cancer (66). HDAC7 was overexpressed in ovarian cancer stem cells and played an important role in maintaining the tumor stem cell phenotype. Therefore, the overexpression of HDAC7 in ovarian cancer stem cell lines (66) makes it a crucial therapeutic target for ovarian cancer. However, the detailed mechanisms by which HDAC7 contributes to the maintenance of the tumor stem cell phenotype have not been investigated. A discovery was reported that valproic acid has the ability to decrease the HDAC7 expression in human ovarian cancer cells when choosing therapeutic medications (67).
4.5 Breast cancer
At present, breast cancer has surpassed lung cancer as the most frequently diagnosed tumor among females (52), with the most malignant type being triple-negative breast cancer. In breast cancer tissue, the level of HDAC7 mRNA was higher compared to normal breast tissue (11). In serum samples, HDAC7 protein was significantly higher in patients with recurrent breast cancer compared to non-recurrent breast cancer (68). Furthermore, HDAC7 exhibited high expression in breast cancer stem cells (66). These findings indicate an association between HDAC7 and breast cancer. Through the deacetylation of HSP70 K246, HDAC7 could hinder autophagic cell death, thereby promoting the survival of cancer cells and the emergence of drug resistance in mechanistic investigations (11). In another research, overexpressed HDAC7 was demonstrated to maintain the breast cancer stem cell phenotype by regulating histone 3 lysine 27 acetylation and enhancing transcriptional activity of super-enhancer-associated genes in breast CSCs (32). These findings provide additional theoretical support for the research and development of HDAC7-specific inhibitors. However, two studies showed conflicting results regarding the expression of HDAC7 in triple-negative breast cancer, where it was observed to be high and low, with both expressions associated with poor prognosis (69, 70). The discrepancies in results may be attributed to differences in sample size and individual heterogeneity.
4.6 Choroidal melanoma
The most prevalent type of uveal melanoma is choroidal melanoma. Currently, therapeutic regimens for choroidal melanoma are primarily referenced to cutaneous melanoma (71), but the treatment effect is less than half that of cutaneous melanoma. Additionally, there are no effective therapeutic agents for metastatic melanoma. Therefore, it is necessary to find potential therapeutic targets for the treatment of choroidal melanoma. In vitro and in vivo experiments, HDAC7 was found to be upregulated in choroidal melanoma and demonstrated its interaction with c-myc to affect the growth and migration of tumor cells (36). Previous research indicates that β-catenin plays a crucial role in the HDAC7/c-myc signaling pathway throughout the progression of tumors. However, the relationship between HDAC7 and β-catenin is unclear and needs experiments to be demonstrated in choroidal melanoma.
4.7 Hematologic tumors
HDAC7 has two opposite expression profiles in hematologic tumors. In patients diagnosed with acute (72) and chronic (73) lymphoblastic leukemia, this gene is found to be highly expressed. However, in B lineage-derived malignancies such as diffuse large B cell lymphoma (74) and acute lymphoblastic leukemia derived from B-cell progenitors, especially in the infant t (4, 11) phenotype (40), the gene is down-regulated. Both overexpression and down-regulation expressions of HDAC7 this gene are associated with poor prognosis. However, the former lacks relevant molecular mechanism research. In contrast, for the latter, a molecular mechanism manifests that HDAC7 suppresses cell oncogenicity by downregulating the expression of c-myc oncogenes and promoting the expression of genes related to apoptosis (75). Consequently, HDAC7 can exhibit both oncogenic and anti-carcinogenic effects in different hematological malignancies.
4.8 Clear cell carcinoma
While enhanced glycolysis has been observed to facilitate tumorigenesis in numerous studies on cancer mechanisms, the expression of enzymes involved in mitochondrial metabolism displays greater heterogeneous. Renal cell carcinoma is one of the tumors with significant alterations in mitochondrial metabolism, particularly with a prominent decrease in the expression of mRNA for tricarboxylic acid cycle enzymes (76). Meanwhile, Nam et al. (7) showed that HDAC7 expression was significantly increased at mRNA and protein levels in clear renal cell carcinoma compared to normal kidneys by analyzing the TCGA database. Moreover, HDAC7 inhibits the expression of tricarboxylic acid cycle enzymes by binding to SMAD, thereby having a negative effect on mitochondrial metabolism, which was manifested in the following that induced the respiratory dysfunction of mitochondria and elevated oncogenes to promote tumorigenesis (7). The results indicate that HDAC7 has a crucial function in tumor metabolism and offers understanding into the molecular mechanism of mitochondrial metabolism changes in clear renal cell carcinoma.
5 Pharmacological strategies
Currently, the primary focus of research on HDAC7 inhibitors has been in the realm of cancer, although recent findings suggest their regulatory effects extend to the inflammatory conditions and metabolic dysfunction (77). Several potent inhibitors of HDAC7 enzymatic activity, namely TMP195 (78), CHDI-12 (79), CHDI390576 (80), CHDI00484077 (81), and JM63 (82), have been identified. Nevertheless, it is important to mention that these blockers also display inhibitory impacts on additional class IIa HDACs (HDAC4, 5, 9) and even show a certain degree of inhibition towards class I HDACs. In cellular systems, TMP195 caused alterations in the release of chemokines CCL1 and CCL2 from macrophages derived from human monocytes. Additionally, it had a lesser effect on gene expression in lymphocytes compared to Vorinostat, suggesting its potential for selectively inhibiting class IIa HDACs. Additionally, TMP195 demonstrated efficacy in reducing breast tumors and metastases in mice through the recruitment and differentiation of anti-tumor macrophages (83). Recently, the CHDI Foundation released research results on three groups of potent brain-penetrating HDAC4 (22) inhibitors, which have shown great effectiveness in treating Huntington’s Disease. Specifically, compounds CHDI: 12, 390576, and 00484077 demonstrated potent inhibition of HDAC7 as well. However, additional research is required to investigate the pharmacological approaches employed by CHDI in targeting HDAC7 for cancer treatment. JM63 is a constituent of a group of benzoyl hydroxamate inhibitors that have been identified as the most effective HDAC7 inhibitors documented thus far (82). In experiments conducted on cells, JM63 displayed an inhibitory potency that was 10 times stronger than TMP195 against class IIa HDACs. Additionally, it exhibited improved stability in cytochrome P450-rich rat liver microsomes, with a half-life of 230 minutes instead of 46 minutes (22). As a potential treatment for hepatocellular carcinoma, JM63 shows promise as a pharmaceutical agent.
6 Future direction
This paper systematically reviews the role of HDAC7 in cancer. The progress of clinical trials for different types of cancers is advancing with the development of additional pan- and selective inhibitors (84). Therefore, it might be possible to specifically focus on HDAC7 for the treatment of cancer. However, there is no doubt that there is still a long way to go.
6.1 The different forms of cancer have varying molecular mechanisms involving HDAC7
Currently, studies have shown that HDAC7 has two expression levels in cancer. For instance, it is overexpressed in gastric cancer (51), and colorectal cancer (10), among others, while it has low expression in pro-B ALL (40). Both of its aberrant expressions exert oncogenic effects in cancer. Additionally, HDAC7 can act as a mediator regulated by miRNAs, playing a role in cancer progression (11, 37, 39, 46). Considering that aberrant expression of HDAC7 correlates with the severity and progression of various cancers, it is possible for it to be a potential biomarker or target. However, making HDAC7 a targeted therapeutic site requires a more precise understanding of the molecular mechanisms that operate in different types of cancer.
6.2 The cellular functions of HDAC7 depends on its distribution in cells
Recent studies have been showed that HDAC7 promotes tumorigenesis by promoting tumor proliferation and metastasis (9, 36), inhibiting drug entry into cells to generate drug resistance (10, 85), suppressing autophagy (11, 86, 87), and many other actions. However, HDAC7 has different biological functions in different cell types, depending on its distribution within cells. For example, it ensures proper B-cell expression during B-cell development (3), p However, HDAC7 has different biological functions in different cell types, depending on its distribution within cells. For example, it ensures proper B-cell expression during B-cell development (4) and maintains the proper functioning of the TCA cycle in mitochondrial metabolism (7). Additionally, a comprehensive knockdown of HDAC7 during embryonic development can lead to embryonic lethality (6), among other effects.
6.3 Potential transcriptional targets for HDAC7
The increasing range of interacting partners suggests a diverse array of potential transcriptional targets for HDAC7.Nevertheless, most biologically significant targets for HDAC7 have thus far been found to be MEF2-dependent promoters. Therefore, it is imperative to conduct comprehensive inquiries to discover genes that are not dependent on MEF2 but could be controlled by HDAC7.
6.4 Selective inhibitors of HDAC7 for the treatment of tumors
Balancing the negative role of HDAC7 in tumors with its role in normal physiological processes is key to the future use of HDAC7 inhibitors for tumor treatment. Thorough examinations regarding the function of HDAC7 in the formation of tumors aid in the investigation of inhibitors that specifically target HDAC7. To achieve the objectives, it is crucial to utilize tissue-specific reduction and thorough mechanistic investigations on the effects of selective inhibitors of HDAC7 both in laboratory settings and within living organisms. Given that HDAC7 exerts oncogenic effects through the same signaling pathway in multiple tumors, it is possible for the same HDAC7 inhibitor to be used as a targeted therapeutic agent for numerous tumors in the future.
7 Limitations
Furthermore, we noted that this review had some limitations (1). It is also important to critically evaluate the action of HDAC7 as an epigenetic regulator and the relationships with inflammatory genes. Given the limited space available, our focus will solely be on elucidating the involvement of HDAC7 in cancer. (2) The role of HDAC7 in cancer may have been overlooked. (3) While there is a possibility of undiscovered mechanisms in the future, numerous mechanisms of HDAC7 are still unidentified in this article.
Author contributions
CL: Writing – original draft, Writing – review & editing. DZ: Validation, Writing – review & editing. XP: Validation, Writing – review & editing. SL: Conceptualization, Resources, Writing – review & editing.
Funding
The author(s) declare that no financial support was received for the research, authorship, and/or publication of this article.
Acknowledgments
Thanks to the related institutions for their help.
Conflict of interest
The authors declare that the research was conducted in the absence of any commercial or financial relationships that could be construed as a potential conflict of interest.
Publisher’s note
All claims expressed in this article are solely those of the authors and do not necessarily represent those of their affiliated organizations, or those of the publisher, the editors and the reviewers. Any product that may be evaluated in this article, or claim that may be made by its manufacturer, is not guaranteed or endorsed by the publisher.
References
1. Bruyer A, Maes K, Herviou L, Kassambara A, Seckinger A, Cartron G, et al. DNMTi/HDACi combined epigenetic targeted treatment induces reprogramming of myeloma cells in the direction of normal plasma cells. Br J Cancer. (2018) 118:1062–73. doi: 10.1038/s41416-018-0025-x
2. Haberland M, Montgomery RL, Olson EN. The many roles of histone deacetylases in development and physiology: implications for disease and therapy. Nat Rev Genet. (2009) 10:32–42. doi: 10.1038/nrg2485
3. Barneda-Zahonero B, Román-González L, Collazo O, Rafati H, Islam AB, Bussmann LH, et al. HDAC7 is a repressor of myeloid genes whose downregulation is required for transdifferentiation of pre-B cells into macrophages. PloS Genet. (2013) 9:e1003503. doi: 10.1371/journal.pgen.1003503
4. Kasler HG, Lim HW, Mottet D, Collins AM, Lee IS, Verdin E. Nuclear export of histone deacetylase 7 during thymic selection is required for immune self-tolerance. EMBO J. (2012) 31:4453–65. doi: 10.1038/emboj.2012.295
5. Jin Z, Wei W, Dechow PC, Wan Y. HDAC7 inhibits osteoclastogenesis by reversing RANKL-triggered β-catenin switch. Mol Endocrinol. (2013) 27:325–35. doi: 10.1210/me.2012-1302
6. Chang S, Young BD, Li S, Qi X, Richardson JA, Olson EN. Histone deacetylase 7 maintains vascular integrity by repressing matrix metalloproteinase 10. Cell. (2006) 126:321–34. doi: 10.1016/j.cell.2006.05.040
7. Nam H, Kundu A, Karki S, Brinkley GJ, Chandrashekar DS, Kirkman RL, et al. The TGF-β/HDAC7 axis suppresses TCA cycle metabolism in renal cancer. JCI Insight. (2021) 6:e148438. doi: 10.1172/jci.insight.148438
8. Guo K, Ma Z, Zhang Y, Han L, Shao C, Feng Y, et al. HDAC7 promotes NSCLC proliferation and metastasis via stabilization by deubiquitinase USP10 and activation of β-catenin-FGF18 pathway. J Exp Clin Cancer Res. (2022) 41:91. doi: 10.1186/s13046-022-02266-9
9. Lei Y, Liu L, Zhang S, Guo S, Li X, Wang J, et al. Hdac7 promotes lung tumorigenesis by inhibiting Stat3 activation. Mol Cancer. (2017) 16:170. doi: 10.1186/s12943-017-0736-2
10. Ye C, Han K, Lei J, Zeng K, Zeng S, Ju H, et al. Inhibition of histone deacetylase 7 reverses concentrative nucleoside transporter 2 repression in colorectal cancer by up-regulating histone acetylation state. Br J Pharmacol. (2018) 175:4209–17. doi: 10.1111/bph.14467
11. Wu M-Y, Fu J, Xiao X, Wu J, Wu R-C. MiR-34a regulates therapy resistance by targeting HDAC1 and HDAC7 in breast cancer. Cancer Lett. (2014) 354:311–9. doi: 10.1016/j.canlet.2014.08.031
12. Marks PA, Breslow R. Dimethyl sulfoxide to vorinostat: development of this histone deacetylase inhibitor as an anticancer drug. Nat Biotechnol. (2007) 25:84–90. doi: 10.1038/nbt1272
13. de Ruijter AJM, van Gennip AH, Caron HN, Kemp S, van Kuilenburg ABP. Histone deacetylases (HDACs): characterization of the classical HDAC family. Biochem J. (2003) 370:737–49. doi: 10.1042/bj20021321
14. McKinsey TA, Zhang CL, Lu J, Olson EN. Signal-dependent nuclear export of a histone deacetylase regulates muscle differentiation. Nature. (2000) 408:106–11. doi: 10.1038/35040593
15. Parra M. Class IIa HDACs - new insights into their functions in physiology and pathology. FEBS J. (2015) 282:1736–44. doi: 10.1111/febs.13061
16. Li X, Song S, Liu Y, Ko SH, Kao HY. Phosphorylation of the histone deacetylase 7 modulates its stability and association with 14-3-3 proteins. J Biol Chem. (2004) 279:34201–8. doi: 10.1074/jbc.M405179200
17. Dequiedt F, Van Lint J, Lecomte E, Van Duppen V, Seufferlein T, Vandenheede JR, et al. Phosphorylation of histone deacetylase 7 by protein kinase D mediates T cell receptor-induced Nur77 expression and apoptosis. J Exp Med. (2005) 201:793–804. doi: 10.1084/jem.20042034
18. Gao C, Li X, Lam M, Liu Y, Chakraborty S, Kao HY. CRM1 mediates nuclear export of HDAC7 independently of HDAC7 phosphorylation and association with 14-3-3s. FEBS Lett. (2006) 580:5096–104. doi: 10.1016/j.febslet.2006.08.038
19. Martin M, Potente M, Janssens V, Vertommen D, Twizere JC, Rider MH, et al. Protein phosphatase 2A controls the activity of histone deacetylase 7 during T cell apoptosis and angiogenesis. Proc Natl Acad Sci USA. (2008) 105:4727–32. doi: 10.1073/pnas.0708455105
20. Asfaha Y, Schrenk C, Alves Avelar LA, Hamacher A, Pflieger M, Kassack MU, et al. Recent advances in class IIa histone deacetylases research. Bioorg Med Chem. (2019) 27:115087. doi: 10.1016/j.bmc.2019.115087
21. Witt O, Deubzer HE, Milde T, Oehme I. HDAC family: What are the cancer relevant targets? Cancer Lett. (2009) 277:8–21. doi: 10.1016/j.canlet.2008.08.016
22. Wang Y, Abrol R, Mak JYW, Das Gupta K, Ramnath D, Karunakaran D, et al. Histone deacetylase 7: a signalling hub controlling development, inflammation, metabolism and disease. FEBS J. (2023) 290:2805–32. doi: 10.1111/febs.16437
23. Schuetz A, Min J, Allali-Hassani A, Schapira M, Shuen M, Loppnau P, et al. Human HDAC7 harbors a class IIa histone deacetylase-specific zinc binding motif and cryptic deacetylase activity. J Biol Chem. (2008) 283:11355–63. doi: 10.1074/jbc.M707362200
24. Seto E, Yoshida M. Erasers of histone acetylation: the histone deacetylase enzymes. Cold Spring Harb Perspect Biol. (2014) 6:a018713. doi: 10.1101/cshperspect.a018713
25. Lahm A, Paolini C, Pallaoro M, Nardi MC, Jones P, Neddermann P, et al. Unraveling the hidden catalytic activity of vertebrate class IIa histone deacetylases. Proc Natl Acad Sci USA. (2007) 104:17335–40. doi: 10.1073/pnas.0706487104
26. Ramnath D, Das Gupta K, Wang Y, Abrol R, Curson JEB, Lim J, et al. The histone deacetylase Hdac7 supports LPS-inducible glycolysis and Il-1β production in murine macrophages via distinct mechanisms. J Leukoc Biol. (2022) 111:327–36. doi: 10.1002/JLB.2MR1021-260R
27. Fischle W, Dequiedt F, Hendzel MJ, Guenther MG, Lazar MA, Voelter W, et al. Enzymatic activity associated with class II HDACs is dependent on a multiprotein complex containing HDAC3 and SMRT/N-CoR. Mol Cell. (2002) 9:45–57. doi: 10.1016/S1097-2765(01)00429-4
28. Martin M, Kettmann R, Dequiedt F. Class IIa histone deacetylases: regulating the regulators. Oncogene. (2007) 26:5450–67. doi: 10.1038/sj.onc.1210613
29. Sinnett-Smith J, Ni Y, Wang J, Ming M, Young SH, Rozengurt E. Protein kinase D1 mediates class IIa histone deacetylase phosphorylation and nuclear extrusion in intestinal epithelial cells: role in mitogenic signaling. Am J Physiol Cell Physiol. (2014) 306:C961–C71. doi: 10.1152/ajpcell.00048.2014
30. Di Giorgio E, Brancolini C. Regulation of class IIa HDAC activities: it is not only matter of subcellular localization. Epigenomics. (2016) 8:251–69. doi: 10.2217/epi.15.106
31. Wong RH, Chang I, Hudak CS, Hyun S, Kwan HY, Sul HS. A role of DNA-PK for the metabolic gene regulation in response to insulin. Cell. (2009) 136:1056–72. doi: 10.1016/j.cell.2008.12.040
32. Caslini C, Hong S, Ban YJ, Chen XS, Ince TA. HDAC7 regulates histone 3 lysine 27 acetylation and transcriptional activity at super-enhancer-associated genes in breast cancer stem cells. Oncogene. (2019) 38:6599–614. doi: 10.1038/s41388-019-0897-0
33. Yu X, Wang M, Wu J, Han Q, Zhang X. ZNF326 promotes Malignant phenotype of glioma by up-regulating HDAC7 expression and activating Wnt pathway. J Exp Clin Cancer Res. (2019) 38:40. doi: 10.1186/s13046-019-1031-4
34. Peixoto P, Blomme A, Costanza B, Ronca R, Rezzola S, Palacios AP, et al. HDAC7 inhibition resets STAT3 tumorigenic activity in human glioblastoma independently of EGFR and PTEN: new opportunities for selected targeted therapies. Oncogene. (2016) 35:4481–94. doi: 10.1038/onc.2015.506
35. Ma Z-Q, Feng Y-T, Guo K, Liu D, Shao C-J, Pan M-H, et al. Melatonin inhibits ESCC tumor growth by mitigating the HDAC7/β-catenin/c-Myc positive feedback loop and suppressing the USP10-maintained HDAC7 protein stability. Mil Med Res. (2022) 9:54. doi: 10.1186/s40779-022-00412-0
36. Zhang Y, Ding P, Wang Y, Shao C, Guo K, Yang H, et al. HDAC7/c-Myc signaling pathway promotes the proliferation and metastasis of choroidal melanoma cells. Cell Death Dis. (2023) 14:38. doi: 10.1038/s41419-022-05522-0
37. Li Q-G, Xiao T, Zhu W, Yu Z-Z, Huang X-P, Yi H, et al. HDAC7 promotes the oncogenicity of nasopharyngeal carcinoma cells by miR-4465-EphA2 signaling axis. Cell Death Dis. (2020) 11:322. doi: 10.1038/s41419-020-2521-1
38. Deng Q, Yang J, Chen Y, Chen Z, Li J, Fu Z. LncRNA HOXB-AS4 promotes proliferation and migration of colorectal cancer via the miR-140-5p/hdac7 axis. Biotechnol Genet Eng Rev. (2023) 1–19. doi: 10.1080/02648725.2023.2193465
39. Zhang H, Li L, Yuan C, Wang C, Gao T, Zheng Z. MiR-489 inhibited the development of gastric cancer via regulating HDAC7 and PI3K/AKT pathway. World J Surg Oncol. (2020) 18:73. doi: 10.1186/s12957-020-01846-3
40. de Barrios O, Galaras A, Trincado JL, Azagra A, Collazo O, Meler A, et al. HDAC7 is a major contributor in the pathogenesis of infant t(4;11) proB acute lymphoblastic leukemia. Leukemia. (2021) 35:2086–91. doi: 10.1038/s41375-020-01097-x
41. Bray F, Ferlay J, Soerjomataram I, Siegel RL, Torre LA, Jemal A. Global cancer statistics 2018: GLOBOCAN estimates of incidence and mortality worldwide for 36 cancers in 185 countries. CA Cancer J Clin. (2018) 68:394–424. doi: 10.3322/caac.21492
42. Sang Y, Sun L, Wu Y, Yuan W, Liu Y, Li S-W. Histone deacetylase 7 inhibits plakoglobin expression to promote lung cancer cell growth and metastasis. Int J Oncol. (2019) 54:1112–22. doi: 10.3892/ijo
43. Mei J, Liu G, Wang W, Xiao P, Yang D, Bai H, et al. OIP5-AS1 modulates epigenetic regulator HDAC7 to enhance non-small cell lung cancer metastasis via miR-140-5p. Oncol Lett. (2020) 20:7. doi: 10.3892/ol
44. Wei Y, Zhou F, Zhou H, Huang J, Yu D, Wu G. Endothelial progenitor cells contribute to neovascularization of non-small cell lung cancer via histone deacetylase 7-mediated cytoskeleton regulation and angiogenic genes transcription. Int J Cancer. (2018) 143:657–67. doi: 10.1002/ijc.31349
45. Hui KF, Cheung AKL, Choi CK, Yeung PL, Middeldorp JM, Lung ML, et al. Inhibition of class I histone deacetylases by romidepsin potently induces Epstein-Barr virus lytic cycle and mediates enhanced cell death with ganciclovir. Int J Cancer. (2016) 138:125–36. doi: 10.1002/ijc.29698
46. Gao S, Liu H, Hou S, Wu L, Yang Z, Shen J, et al. MiR-489 suppresses tumor growth and invasion by targeting HDAC7 in colorectal cancer. Clin Transl Oncol. (2018) 20:703–12. doi: 10.1007/s12094-017-1770-7
47. Tang H, Jiang L, Zhu C, Liu R, Wu Y, Yan Q, et al. Loss of cell adhesion molecule L1 like promotes tumor growth and metastasis in esophageal squamous cell carcinoma. Oncogene. (2019) 38:3119–33. doi: 10.1038/s41388-018-0648-7
48. Zhu C, Chen Q, Xie Z, Ai J, Tong L, Ding J, et al. The role of histone deacetylase 7 (HDAC7) in cancer cell proliferation: regulation on c-Myc. J Mol Med (Berl). (2011) 89:279–89. doi: 10.1007/s00109-010-0701-7
49. Feng Y, Ma Z, Pan M, Xu L, Feng J, Zhang Y, et al. WNT5A promotes the metastasis of esophageal squamous cell carcinoma by activating the HDAC7/SNAIL signaling pathway. Cell Death Dis. (2022) 13:480. doi: 10.1038/s41419-022-04901-x
50. Wadhwa R, Song S, Lee J-S, Yao Y, Wei Q, Ajani JA. Gastric cancer-molecular and clinical dimensions. Nat Rev Clin Oncol. (2013) 10:643–55. doi: 10.1038/nrclinonc.2013.170
51. Yu Y, Cao F, Yu X, Zhou P, Di Q, Lei J, et al. The expression of HDAC7 in cancerous gastric tissues is positively associated with distant metastasis and poor patient prognosis. Clin Transl Oncol. (2017) 19:1045–54. doi: 10.1007/s12094-017-1639-9
52. Sung H, Ferlay J, Siegel RL, Laversanne M, Soerjomataram I, Jemal A, et al. Global cancer statistics 2020: GLOBOCAN estimates of incidence and mortality worldwide for 36 cancers in 185 countries. CA Cancer J Clin. (2021) 71:209–49. doi: 10.3322/caac.21660
53. Sveen A, Kopetz S, Lothe RA. Biomarker-guided therapy for colorectal cancer: strength in complexity. Nat Rev Clin Oncol. (2020) 17:11–32. doi: 10.1038/s41571-019-0241-1
54. Stypula-Cyrus Y, Damania D, Kunte DP, Cruz MD, Subramanian H, Roy HK, et al. HDAC up-regulation in early colon field carcinogenesis is involved in cell tumorigenicity through regulation of chromatin structure. PloS One. (2013) 8:e64600. doi: 10.1371/journal.pone.0064600
55. Gerçeker E, Boyacıoglu SO, Kasap E, Baykan A, Yuceyar H, Yıldırım H, et al. Never in mitosis gene A-related kinase 6 and aurora kinase A: New gene biomarkers in the conversion from ulcerative colitis to colorectal cancer. Oncol Rep. (2015) 34:1905–14. doi: 10.3892/or.2015.4187
56. Ouaïssi M, Sielezneff I, Silvestre R, Sastre B, Bernard J-P, Lafontaine JS, et al. High histone deacetylase 7 (HDAC7) expression is significantly associated with adenocarcinomas of the pancreas. Ann Surg Oncol. (2008) 15:2318–28. doi: 10.1245/s10434-008-9940-z
57. Ouaïssi M, Silvy F, Loncle C, Ferraz da Silva D, Martins Abreu C, Martinez E, et al. Further characterization of HDAC and SIRT gene expression patterns in pancreatic cancer and their relation to disease outcome. PloS One. (2014) 9:e108520. doi: 10.1371/journal.pone.0108520
58. Freese K, Seitz T, Dietrich P, Lee SML, Thasler WE, Bosserhoff A, et al. Histone deacetylase expressions in hepatocellular carcinoma and functional effects of histone deacetylase inhibitors on liver cancer cells in vitro. Cancers. (2019) 11:1587. doi: 10.3390/cancers11101587
59. Anstee QM, Reeves HL, Kotsiliti E, Govaere O, Heikenwalder M. From NASH to HCC: current concepts and future challenges. Nat Rev Gastroenterol Hepatol. (2019) 16:411–28. doi: 10.1038/s41575-019-0145-7
61. Liu H-L, Fan C-H, Ting C-Y, Yeh C-K. Combining microbubbles and ultrasound for drug delivery to brain tumors: current progress and overview. Theranostics. (2014) 4:432–44. doi: 10.7150/thno.8074
62. Galli R, Binda E, Orfanelli U, Cipelletti B, Gritti A, De Vitis S, et al. Isolation and characterization of tumorigenic, stem-like neural precursors from human glioblastoma. Cancer Res. (2004) 64:7011–21. doi: 10.1158/0008-5472.CAN-04-1364
63. Eyüpoglu IY, Savaskan NE. Epigenetics in brain tumors: HDACs take center stage. Curr Neuropharmacol. (2016) 14:48–54. doi: 10.2174/1570159X13666151030162457
64. Was H, Krol SK, Rotili D, Mai A, Wojtas B, Kaminska B, et al. Histone deacetylase inhibitors exert anti-tumor effects on human adherent and stem-like glioma cells. Clin Epigenetics. (2019) 11:11. doi: 10.1186/s13148-018-0598-5
65. du Bois A, Reuss A, Pujade-Lauraine E, Harter P, Ray-Coquard I, Pfisterer J. Role of surgical outcome as prognostic factor in advanced epithelial ovarian cancer: a combined exploratory analysis of 3 prospectively randomized phase 3 multicenter trials: by the Arbeitsgemeinschaft Gynaekologische Onkologie Studiengruppe Ovarialkarzinom (AGO-OVAR) and the Groupe d'Investigateurs Nationaux Pour les Etudes des Cancers de l'Ovaire (GINECO). Cancer. (2009) 115:1234–44. doi: 10.1002/cncr.24149
66. Witt AE, Lee CW, Lee TI, Azzam DJ, Wang B, Caslini C, et al. Identification of a cancer stem cell-specific function for the histone deacetylases, HDAC1 and HDAC7, in breast and ovarian cancer. Oncogene. (2017) 36:1707–20. doi: 10.1038/onc.2016.337
67. Kwiecińska P, Wróbel A, Taubøll E, Gregoraszczuk E. Valproic acid, but not levetiracetam, selectively decreases HDAC7 and HDAC2 expression in human ovarian cancer cells. Toxicol Lett. (2014) 224:225–32. doi: 10.1016/j.toxlet.2013.10.035
68. Bera A, Russ E, Manoharan MS, Eidelman O, Eklund M, Hueman M, et al. Proteomic analysis of inflammatory biomarkers associated with breast cancer recurrence. Mil Med. (2020) 185:669–75. doi: 10.1093/milmed/usz254
69. Zhu M, Liu N, Lin J, Wang J, Lai H, Liu Y. HDAC7 inhibits cell proliferation via NudCD1/GGH axis in triple-negative breast cancer. Oncol Lett. (2023) 25:33. doi: 10.3892/ol.2022.13619
70. Uzelac B, Krivokuca A, Susnjar S, Milovanovic Z, Supic G. Histone deacetylase 7 gene overexpression is associated with poor prognosis of triple-negative breast cancer patients. Genet Test Mol Biomarkers. (2021) 25:227–35. doi: 10.1089/gtmb.2020.0138
71. Yi JH, Yi SY, Lee HR, Lee SI, Lim DH, Kim JH, et al. Dacarbazine-based chemotherapy as first-line treatment in noncutaneous metastatic melanoma: multicenter, retrospective analysis in Asia. Melanoma Res. (2011) 21:223–7. doi: 10.1097/CMR.0b013e3283457743
72. Moreno DA, Scrideli CA, Cortez MA, de Paula Queiroz R, Valera ET, da Silva Silveira V, et al. Differential expression of HDAC3, HDAC7 and HDAC9 is associated with prognosis and survival in childhood acute lymphoblastic leukaemia. Br J Haematol. (2010) 150:665–73. doi: 10.1111/j.1365-2141.2010.08301.x
73. Van Damme M, Crompot E, Meuleman N, Mineur P, Bron D, Lagneaux L, et al. HDAC isoenzyme expression is deregulated in chronic lymphocytic leukemia B-cells and has a complex prognostic significance. Epigenetics. (2012) 7:1403–12. doi: 10.4161/epi.22674
74. Lu W, Zhuang G, Guan Y, Li Y, Liu L, Xiao M. Comprehensive analysis of HDAC7 expression and its prognostic value in diffuse large B cell lymphoma: A review. Med (Baltimore). (2023) 102:e34577. doi: 10.1097/MD.0000000000034577
75. Barneda-Zahonero B, Collazo O, Azagra A, Fernández-Duran I, Serra-Musach J, Islam AB, et al. The transcriptional repressor HDAC7 promotes apoptosis and c-Myc downregulation in particular types of leukemia and lymphoma. Cell Death Dis. (2015) 6:e1635. doi: 10.1038/cddis.2014.594
76. Hu J, Locasale JW, Bielas JH, O'Sullivan J, Sheahan K, Cantley LC, et al. Heterogeneity of tumor-induced gene expression changes in the human metabolic network. Nat Biotechnol. (2013) 31:522–9. doi: 10.1038/nbt.2530
77. Liu L, Dong L, Bourguet E, Fairlie DP. Targeting class IIa HDACs: insights from phenotypes and inhibitors. Curr Med Chem. (2021) 28:8628–72. doi: 10.2174/0929867328666210629160647
78. Lobera M, Madauss KP, Pohlhaus DT, Wright QG, Trocha M, Schmidt DR, et al. Selective class IIa histone deacetylase inhibition via a nonchelating zinc-binding group. Nat Chem Biol. (2013) 9:319–25. doi: 10.1038/nchembio.1223
79. Luckhurst CA, Breccia P, Stott AJ, Aziz O, Birch HL, Bürli RW, et al. Potent, selective, and CNS-penetrant tetrasubstituted cyclopropane class IIa histone deacetylase (HDAC) inhibitors. ACS Med Chem Lett. (2016) 7:34–9. doi: 10.1021/acsmedchemlett.5b00302
80. Luckhurst CA, Aziz O, Beaumont V, Bürli RW, Breccia P, Maillard MC, et al. Development and characterization of a CNS-penetrant benzhydryl hydroxamic acid class IIa histone deacetylase inhibitor. Bioorg Med Chem Lett. (2019) 29:83–8. doi: 10.1016/j.bmcl.2018.11.009
81. Stott AJ, Maillard MC, Beaumont V, Allcock D, Aziz O, Borchers AH, et al. Evaluation of 5-(Trifluoromethyl)-1,2,4-oxadiazole-based class IIa HDAC inhibitors for huntington's disease. ACS Med Chem Lett. (2021) 12:380–8. doi: 10.1021/acsmedchemlett.0c00532
82. Mak JYW, Wu KC, Gupta PK, Barbero S, McLaughlin MG, Lucke AJ, et al. HDAC7 inhibition by phenacetyl and phenylbenzoyl hydroxamates. J Med Chem. (2021) 64:2186–204. doi: 10.1021/acs.jmedchem.0c01967
83. Guerriero JL, Sotayo A, Ponichtera HE, Castrillon JA, Pourzia AL, SChad S, et al. Class IIa HDAC inhibition reduces breast tumours and metastases through anti-tumour macrophages. Nature. (2017) 543:428–32. doi: 10.1038/nature21409
84. Bondarev AD, Attwood MM, Jonsson J, Chubarev VN, Tarasov VV, Schiöth HB. Recent developments of HDAC inhibitors: Emerging indications and novel molecules. Br J Clin Pharmacol. (2021) 87:4577–97. doi: 10.1111/bcp.14889
85. Wang Y, Zhu Q, Hu H, Zhu H, Yang B, He Q, et al. Upregulation of histone acetylation reverses organic anion transporter 2 repression and enhances 5-fluorouracil sensitivity in hepatocellular carcinoma. Biochem Pharmacol. (2021) 188:114546. doi: 10.1016/j.bcp.2021.114546
86. Zheng Z, Zhong Q, Yan X. YWHAE/14-3-3ϵ crotonylation regulates leucine deprivation-induced autophagy. Autophagy. (2023) 19:2401–2. doi: 10.1080/15548627.2023.2166276
Keywords: HDAC7, cancer, signaling pathway, molecular mechanism, inhibitors
Citation: Liu C, Zheng D, Pu X and Li S (2024) HDAC7: a promising target in cancer. Front. Oncol. 14:1327933. doi: 10.3389/fonc.2024.1327933
Received: 25 October 2023; Accepted: 14 February 2024;
Published: 28 February 2024.
Edited by:
Noritaka Yamaguchi, Chiba University, JapanReviewed by:
Fangfang Liu, Zhengzhou University, ChinaCelio Geraldo Freire-de-Lima, Federal University of Rio de Janeiro, Brazil
Copyright © 2024 Liu, Zheng, Pu and Li. This is an open-access article distributed under the terms of the Creative Commons Attribution License (CC BY). The use, distribution or reproduction in other forums is permitted, provided the original author(s) and the copyright owner(s) are credited and that the original publication in this journal is cited, in accordance with accepted academic practice. No use, distribution or reproduction is permitted which does not comply with these terms.
*Correspondence: Sijun Li, MTAwNjAzODQ3M0BxcS5jb20=