- Department of Pediatric Surgery, Sichuan Provincial People’s Hospital, School of Medicine, University of Electronic Science and Technology of China, Chengdu, China
Infantile hemangioma (IH) is the most common benign vascular tumor characterized by three phases — proliferation, early involution and late involution. Mast cells (MCs) play an important role in allergic reactions and numerous diseases, including tumors. While the mechanisms underlying MCs migration, activation and function in the life cycle of IH remain unclear, previous studies suggested that MCs circulate through the vasculature and migrate into IH, and subsequently mature and get activated. Estradiol (E2) emerges as a potential attractant for MC migration into IH and their subsequent activation. In various stages of IH, activated MCs secrete both proangiogenic and anti-angiogenic modulators, absorbed by various cells adjacent to them. Imbalances in these modulators may contribute to IH proliferation and involution.
1 Introduction
Infantile hemangioma (IH) is the most common benign vascular tumor among infants, impacting nearly 10% of all infants worldwide (1, 2). IH is more prevalent in females, premature births, Caucasian descent, and low birth weight infants (1, 2). Despite its prevalence, the exact cause of IH remains elusive. IH comprises stem cells capable of proliferating and differentiating into various cell types, including neuroglial stem cells, endothelial progenitor cells, hematopoietic stem cells, and mesenchymal stem cells (3). IH exhibits three overlapping phases: proliferation (proliferating) phase, early involution (involuting) phase, and late involution (involuting) phase (4). Histologically, the proliferation phase is characterized by a lot of rapidly dividing endothelial cells (ECs), plump pericytes, mast cells (MCs), and interstitial dendritic cells. The early involution phase exhibits increased MCs, apoptotic bodies, and decreased mitotic figures, along with a reduction in lesional capillaries and ECs. As involution continues into the late phase, ECs, MCs, and vascular channels gradually diminish, replaced by loose fibrous or fibrofatty tissues (4). This spontaneous regression is the most important biological feature of IH. Although approximately 90% of IH cases resolve spontaneously, about 69% are left with varying degrees of sequelae, such as hyperpigmentation, telangiectasia, fibrofatty tissue accumulation, and scarring (5).
MCs, recognized for their involvement in allergic reactions and various physiological processes, including angiogenesis, vasodilation, wound healing, fibrosis, and immune regulation, have also garnered attention in the context of diseases, including tumors (6). Over a century ago, Ehrlich and Westphal reported the presence of MCs in human tumors for the first time (7). Extensive evidence suggests that MCs accumulating around tumors can either promote or inhibit tumor growth, contingent on local stromal conditions and the stage of tumorigenesis (8). However, their association with IH remains somewhat contentious. Some researchers have reported that MCs promote the proliferation of ECs in IH by secreting multiple modulators (9), while others have contended that MCs inhibit IH ECs proliferation through the release of anti-angiogenic factors (10). In this review, we delve into recent findings regarding MCs origin, migration, activation, and reactivity within the context of IH.
2 Origin and localization of mast cells
MCs were initially thought to originate from blood basophils or local histiocytic progenitors (11). Paul Ehrlich made the pioneering discovery of MCs and documented their presence in perivascular tissues in 1877 (12). Lombardo’s study in 1909 revealed that MCs first appear in human embryos during the eighth week of gestation (13).
Subsequently, various cells, including ECs, mesenchymal cells, thymocytes, pericytes, and fibroblasts, have been identified as precursors to MCs (14, 15). Unlike T and B lymphocytes, monocytes, and neutrophils that circulate in the bloodstream, MCs are tissue-resident guard cells (16). They are distributed throughout nearly all tissues, with a particularly prominent presence in the dermis, respiratory mucosa, digestive and urogenital systems, blood and lymph vessels, and fibroblasts. This distribution allows them to detect changes in their local microenvironment (17). The presence of MCs within vessel walls underscores their role in the vasculature, notably in vasodilation and tissue-specific responses to circulating agents (18).
3 Mast cells migrate into IH
As previously mentioned, MCs originate from progenitors in the bone marrow and enter the peripheral blood, but they are not yet fully mature at this stage. Circulating MC precursors migrate into various vascularized peripheral tissues via adhesion contacts to a network formed by integrins (19). Subsequently, they take up residence within these tissues, completing their maturation and function under the influence of locally produced factors (20, 21). Studies have indicated that the number of MCs fluctuates during the different pathological phases of IH (22, 23). Indeed, the count of MCs is highest during the early involuting phase, followed by the late involuting and proliferating phases, respectively (24). Furthermore, the percentage of proliferating MCs is highest in the proliferating phase and lowest in the late involuting phase (23). Nevertheless, the precise mechanisms underlying the presence and fluctuations in MC numbers within IH remain unclear.
A recent observation regarding the presence of Estradiol (E2) on MCs suggests that E2 might play a role in attracting MCs to migrate into IH tissue (24). This is likely because MCs primarily migrate to their target locations in response to locally produced chemokines (21, 25, 26), and E2 could potentially modulate the expression of chemokine receptors on the surface of MCs (20). Upon migrating into IH tissue, MCs undergo maturation and activation. A previous study reported an abundance of immature MCs during the IH proliferation phase, while the involution phase sees a rich infiltration of mature MCs (4).
4 Activated MCs in IH
MCs may be activated through various mechanisms, leading to the secretion of a wide array of mediators, including proteases (such as tryptase, chymase, carboxypeptidase, MCP-1, MCP-2), proteoglycans (like heparin and chondroitin sulfate), proteins (including CRH, osteopontin, thymic stromal lymphopoietin), biogenic amines (histamine and serotonin), growth factors (PDGF, VEGF, FGF2, bFGF, NGF, PAF), and cytokines (such as TNFα, lymphotactin, IL-1β, IL-3, IL-4, IL-5, IL-6, IL-9, IL-10, IL-12, IL-13, IL-16, IL-17A, IL-18, IL-23, IFN-α, IFN-β, IFNγ) (6, 20, 25, 27).
MCs degranulation is essential for the release of these mediators. This complex process involves membrane fusion and various proteins, depending on whether allergic or non-allergic reactions trigger it (28). Recent advances have elucidated three primary pathways of degranulation activation in non-allergic reactions (27) (Figure 1):
1. Activation through Mas-related G-protein-coupled-ligand receptor member X2 (MRGPRX2) binding, which triggers Inositol Phosphate-Phospholipase C (IP-PLC) and leads to the formation of Inositol trisphosphate (IP3). This results in the opening of Ca2+ channels, elevating intracellular Ca2+ levels and initiating degranulation.
2. Activation by binding of Benzoyl ATP activates ion channels, causing a rapid influx of nonselective ions, including Ca2+, and the opening of large plasma membrane pores, ultimately resulting in degranulation.
3. Activation of Extracellular signal-regulated kinase 1/2 (ERK1/2) due to physical contact with activated T-cell membranes and released microvesicles, leading to the expression of cytokines, chemokines, adenosine, and growth factors.
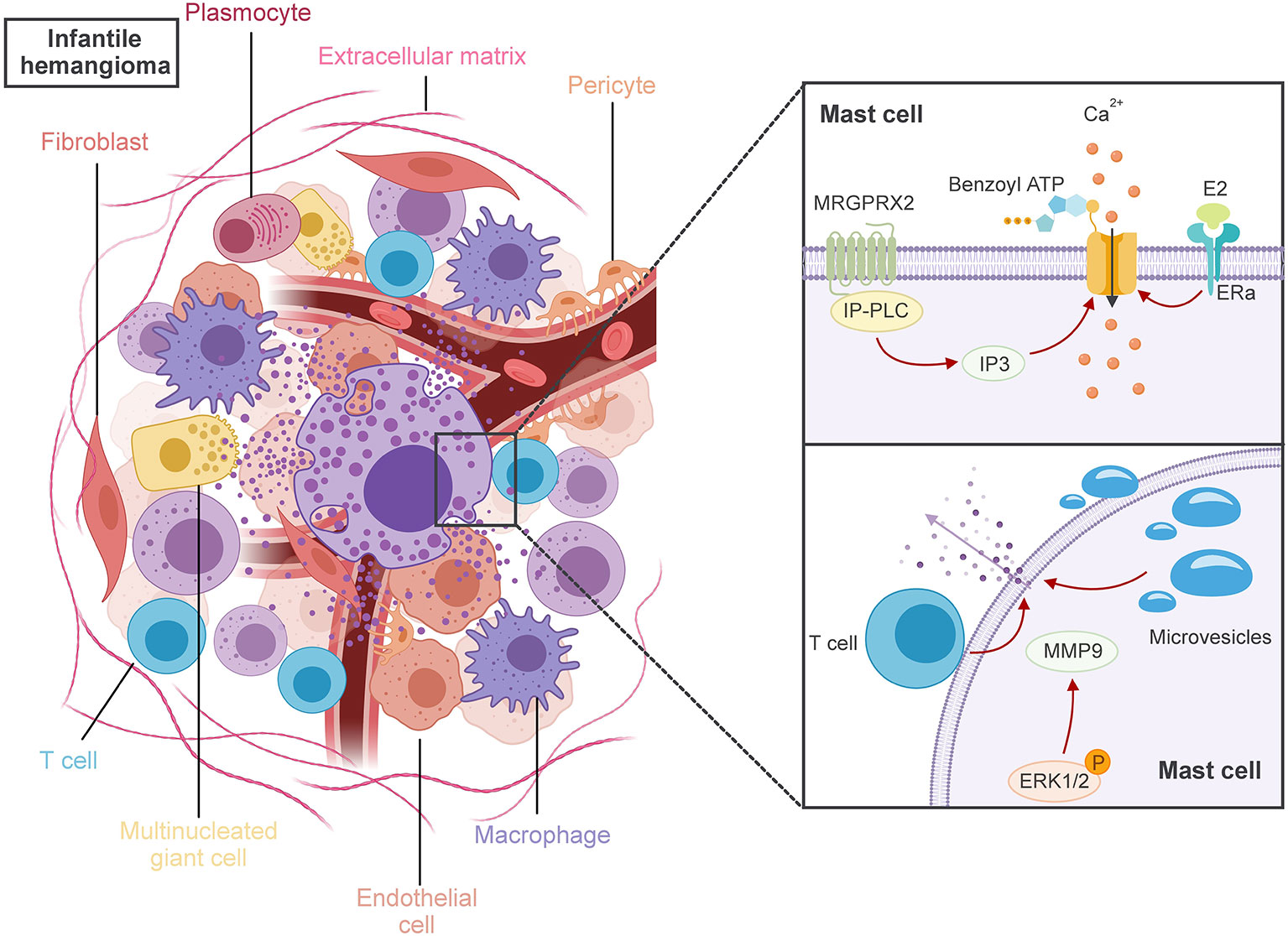
Figure 1 MCs have crucial roles to play in the pathogenesis of IH. MRGPRX2 binding triggers IP-PLC and leads to the formation of IP3. The binding of benzoyl ATP activates ion channels, and E2 binding to ER-a on MCs, all of which could induce the inflow of extracellular Ca2+, ultimately leading to the activation of MCs degranulation. Physical contact with activated T-cell membranes and released microvesicles triggers the degranulation of MCs, which in turn stimulates the activation of ERK1/2. These actions ultimately lead MCs to release either angiogenesis or anti-angiogenesis key factors during various IH phases, which are taken up by adjacent cells like fibroblasts, macrophages, multinucleated giant cells, and plasma cells.
Despite these insights, the precise mechanisms behind MCs activation and the number of activated MCs in IH remain elusive. Some literature suggests that local elevated levels of E2 may influence MCs activation (29). It has been reported that E2 binding to estrogen receptor-a (ERa) on MCs may trigger a rapid onset and progressive influx of extracellular Ca2+, leading to MCs activation (30). In this context, a previous report suggests that E2-positive MCs might be involved in the population of activated MCs in IH (24).
Upon activation, MCs can release a diverse group of pivotal factors that play crucial roles in either angiogenesis or anti-angiogenesis during the various phases of IH (31). Those factors will be uptake by the adjacent cells, such as fibroblasts, macrophages, multinucleated giant cells, and plasma cells within IH (32). However, the specific angiogenesis or anti-angiogenesis factors exchanged between these cells remain unclear. As a general rule of thumb, MCs release mediators based on the ligands that activate their receptors and the surrounding microenvironment (18).
5 Mast cells are involved in IH proliferation
The notion that MCs may promote tumor growth has long been suggested (33) and has been observed to induce the proliferation of human microvascular ECs (34). Activated MCs release multiple modulators, including histamine, tryptase, chymase, type VIII collagen, vascular endothelial growth factor (VEGF), and fibroblast growth factor (FGF)-2, which have been implicated as angiogenic factors in IH (9).
Type VIII collagen and FGF-2 are believed to play significant roles in IH ECs proliferation, with their expression detected on MCs only during the proliferation phase of IH. These findings align with recent in vitro research showing that propranolol can inhibit IH proliferation by reducing the expression of VEGF-A, bFGF, MMP2, MMP9, and tryptase in MCs (35).
There are three types of specific proteases present in MCs secretory granules: chymase, tryptase and carboxypeptidase A (31). Chymase indirectly induces angiogenesis by activating MMP-9 or converting Angiotensin I to Angiotensin II. Tryptase serves as a significant factor in angiogenesis. It can alter the composition of the extracellular matrix by stimulating its components, leading to neovascularization (36). In IH, the percentage of MCs positive for both tryptase and chymase reaches highest during the proliferation phase, and decreases in early and late involution phases, indicating a proangiogenic role for chymase in IH (36). Histamine, a vasoactive amine synthesized and stored in MCs’ cytoplasmic granules, contributes to vasodilation, arteriolar constriction, angiogenesis, and vascular permeability (18). Despite the above knowledge, confirming the proangiogenic role of tryptase and histamine in IH remains a great challenge. MCs express histamine and tryptase throughout all phases of IH (31). The total number of MCs positive for tryptase peaks during the involution phase of IH (24, 31).
In addition to secreting mediators upon activation, human skin MCs can spontaneously and constitutively release pro-angiogenic factors (37). Meanwhile, IH arise most commonly in or under the skin.
6 Mast cells are involved in IH involution
Activated MCs also release multiple anti-angiogenic modulators, including interferon (IFN)-α, IFN-β, IFN-γ, and transforming growth factor (TGF)-β. FGF-2 and VEGF are recognized as significant angiogenic factors in proliferating IH. IFN-α and IFN-β can down-regulate FGF-2 (38, 39), while IFN-γ inhibits the mitogenic effect of VEGF (40). TGF-β can inhibit ECs proliferation, and a lack of TGF-β may lead to increased ECs proliferation (10, 31). In IH, the expression of TGF-β in the early involution phase was significantly higher than the late involution and proliferation phases (41). Furthermore, TGF-β1 can stimulate the proliferation of fibroblasts (42), As fibrosis occurs in the IH involution phase, cytoplasmic bridges between MCs and fibroblasts have been observed by ultrastructural studies (31).
Moreover, the number of MCs positive for clusterin/apolipoprotein J (an apoptotic protein) is highest during the early involuting phase and absent in the proliferating phase of IH (23). Recent studies have observed that IH in the involuting phases contains more E2-positive MCs compared to proliferating phase specimens (24). These findings suggest that certain MCs, activated by E2, probably play a role in IH regression.
Propranolol, a non-specific beta-blocker, has gained recognition as the first-line treatment for IH, resulting in rapid lesion regression and reduced fibrofatty residuum. While the molecular mechanisms underlying propranolol’s action on IH are not fully understood, some research suggests that MCs, through secreted proteases, may contribute to accelerated microvascular maturation, enhanced tissue remodeling, and reduced fibrofatty residuum observed with propranolol treatment (43). Prey S et al. (44) studied the expression of beta-adrenergic receptors (ADRB1, 2 and 3) to identify potential propranolol targets cells. They found that ADRB2 was highly and uniformly expressed on MCs of IH. MCs, due to their high ADRB2 expression, may be potential targets for propranolol and may play an indirect anti-angiogenic role in IH (45).
Furthermore, the significant increase in MCs numbers following steroid treatment of IH suggests that MCs may possess antiangiogenic properties and expedite IH involution (46), although propranolol’s proven efficacy and safety in treating IH have reduced the use of steroids (47).
7 Conclusions
Throughout the years, researchers have been captivated by the biology and the role of MCs in IH. Several significant discoveries have shed light on the function of this unique cell in IH. Nevertheless, numerous questions remain unanswered. E2, a female sex hormone, has long been suggested to influence IH and MCs functionality (24, 33). Moreover, IH, like many MC-related diseases, exhibits a higher prevalence in females than in males (26, 48). However, the well-documented connections among E2, MCs, and IH continue to elude us. Recent robust data indicate that imbalanced levels of angiogenic and anti-angiogenic factors secreted by activated MCs may contribute to IH proliferation and involution. Therefore, the coming years hold the potential for rapid advancements in MCs research, offering insights not only into their relationship with E2 but also a deeper understanding of their role in IH.
Author contributions
MX: Writing – original draft. WL: Writing – review & editing. FH: Writing – review & editing.
Funding
The author(s) declare financial support was received for the research, authorship, and/or publication of this article. This work was supported by the National Natural Science Foundation of China (Grant No. 81300238), Key research Project of Sichuan Provincial Science and Technology Department (Grant No. 2021YFS0381) and Specialized Research Foundation of Sichuan Provincial People’s Hospital (Grant No.2021ZX03).
Conflict of interest
The authors declare that the research was conducted in the absence of any commercial or financial relationships that could be construed as a potential conflict of interest.
Publisher’s note
All claims expressed in this article are solely those of the authors and do not necessarily represent those of their affiliated organizations, or those of the publisher, the editors and the reviewers. Any product that may be evaluated in this article, or claim that may be made by its manufacturer, is not guaranteed or endorsed by the publisher.
References
1. Léauté-Labrèze C, Harper JI, Hoeger PH. Infantile haemangioma. Lancet. (2017) 1:85–94. doi: 10.1016/S0140-6736(16)00645-0
2. Munden A, Butschek R, Tom WL, Marshall JS, Poeltler DM, Krohne SE, et al. Prospective study of infantile haemangiomas: incidence, clinical characteristics and association with placental anomalies. Br J Dermatol (2014) 170(4):907–13. doi: 10.1111/bjd.12804
3. Bandera AIR, M.D. FACD, Sebaratnam DF, M.D. FACD, Wargon O, Wong LCF. Infantile hemangioma. Part 1: Epidemiology, pathogenesis, clinical presentation and assessment. J Am Acad Dermatol (2021) 85(6):1379–92. doi: 10.1016/j.jaad.2021.08.019
4. Mulliken JB, Bischoff J. Pathogenesis of infantile hemangioma. In: Mulliken JB, Burrows PE, Fishman SJ, editors. Vascular anomalies: hemangiomas and malformations, 2nd. New York: Oxford University Press (2013). p. 43–67.
5. Bauland CG, Lüning TH, Smit JM, Zeebregts CJ, Spauwen PHM. Untreated hemangiomas: growth pattern and residual lesions. Plast Reconstr Surg (2011) 127(4):1643—8. doi: 10.1097/PRS.0b013e318208d2ac.
6. Kim HS, Kawakami Y, Kasakura K, Kawakami T. Recent advances in mast cell activation and regulation. F1000Res. (2020) 9:F1000 Faculty Rev–196. doi: 10.12688/f1000research.22037.1
7. Floroni E, Ceauşu AR, Cosoroabă RM, Niculescu Talpoş IC, Popovici RA, Gaje NP, et al. Mast cell density in the primary tumor predicts lymph node metastases in patients with breast cancer. Rom J Morphol Embryol. (2022) 63(1):129–35. doi: 10.47162/RJME.63.1.13
8. Theoharides TC, Conti P. Mast cells: the JEKYLL and HYDE of tumor growth. Trends Immunol (2004) 25(5):235–41. doi: 10.1016/j.it.2004.02.013
9. Park M, Jung HL, Shim YJ, Kim HS, Yoon HS, Park SK, et al. Serum cytokine profiles in infants with infantile hemagimomas on oral propranolol treatment: VEGF-A and bFGF, potential biomarkers predicting clinical outcomes. Pediatr Res (2020) 88:749–55. doi: 10.1038/s41390-020-0862-1
10. Jonker L. TGF-β & BMP receptors endoglin and ALK1: overview of their functional role and status as antiangiogenic targets. Microcirculation.21 (2014) 2):93–103. doi: 10.1111/micc.12099
11. Ribatti D, d’Amati A. Hematopoiesis and mast cell development. Int J Mol Sci (2023) 24(13):10679. doi: 10.3390/ijms241310679
12. Ehrlich P. Beitraege zur kenntniss der anilinfaerbungen und ihrer verwendung in der mikroskopischen technik. Arch mikr anat u entwcklngsmech. (1877) 13:263–77. doi: 10.1007/BF02933937
14. Bloom GD. Chapter 10—Structural and biochemical characteristics of mast cells. In: Zweifach BW, Grant L, McCluskey RT, editors. The inflammatory process, 2nd. Cambridge, MA, USA: Academic Press (1974), ISBN: 978-0-12-783401-6. p. 545–99.
15. Ginsburg H. The in vitro differentiation and culture of normal mast cells from the mouse thymus. Ann N Y. Acad Sci (1963) 103:20–39. doi: 10.1111/j.1749-6632.1963.tb53690.x
16. Krystel-Whittemore M, Dileepan KN, Wood JG. Mast cell: a multi-functional master cell. Front Immunol (2015) 6:620. doi: 10.3389/fimmu.2015.00620
17. Maurer M, Theoharides T, Granstein RD, Bischoff SC, Bienenstock J, Henz B, et al. What is the physiological function of mast cells? Exp Dermatol (2003) 12:886. doi: 10.1111/j.0906-6705.2003.0109a.x
18. Dileepan KN, Raveendran VV, Sharma R, Abraham H, Barua R, Singh V, et al. Mast cell-mediated immune regulation in health and disease. Front Med (2023) 10:1213320. doi: 10.3389/fmed.2023.1213320
19. Kaltenbach L, Martzloff P, Bambach SK, Aizarani N, Mihlan M, Gavrilov A, et al. Slow integrin-dependent migration organizes networks of tissue-resident mast cells. Nat Immunol (2023) 24:915–24. doi: 10.1038/s41590-023-01493-2
20. Jensen F, Woudwyk M, Teles A, Woidacki K, Taran F, Costa S, et al. Estradiol and progesterone regulate the migration of mast cells from the periphery to the uterus and induce their maturation and degranulation. PloS One (2010) 5(12):e14409. doi: 10.1371/journal.pone.0014409
21. Dahlin JS, Maurer M, Metcalfe DD, Pejler G, Sagi-Eisenberg R, Nilsson G. The ingenious mast cell: Contemporary insights into mast cell behavior and function. Allergy. (2022) 77(1):83–99. doi: 10.1111/all.14881
22. Itinteang T, Tan ST, Jia J, Steel R, Laing EL, Brasch HD, et al. Mast cells in infantile haemangioma possess a primitive myeloid phenotype. J Clin Pathol (2013) 66(7):597–600. doi: 10.1136/jclinpath-2012-201096
23. Hasan Q, Ruger BM, Tan ST, Gush J, Davis PF. Clusterin/apoJ expression during the development of hemangioma. Hum Pathol (2000) 31:691–97. doi: 10.1053/hupa.2000.7638
24. Hou F, Dai Y, Fan CY, Suen JY, Richter GT. Estrogen is involved in hemangioma regression associated with mast cells. Orphanet J Rare Dis (2018) 13(1):181. doi: 10.1186/s13023-018-0928-x
25. Krystel-Whittemore M, Dileepan KN, Wood JG. Mast cell: A multi-functional master cell. Front Immunol (2016) 6:620. doi: 10.3389/fimmu.2015.00620
26. Brightling CE, Bradding P, Pavord ID, Wardlaw AJ. New insights into the role of the mast cell in asthma. Clin Exp Allergy (2003) 33(5):550–6. doi: 10.1046/j.1365-2222.2003.01636.x
27. Gutowski Ł., Kanikowski S, Formanowicz D. Mast cell involvement in the pathogenesis of selected musculoskeletal diseases. Life (2023) 13(8):1690. doi: 10.3390/life13081690
28. Singh J, Shah R, Singh D. Targeting mast cells: uncovering prolific therapeutic role in myriad diseases. Int Immunopharmacol. (2016) 40:362–84. doi: 10.1016/j.intimp.2016.09.019
29. Zhu TH, Ding SJ, Li TT, Zhu LB, Huang XF, Zhang XM. Estrogen is an important mediator of mast cell activation in ovarian endometriomas. Reproduction. (2018) 155(1):73–83. doi: 10.1530/REP-17-0457
30. Zaitsu M, Narita S, Lambert KC, Grady JJ, Estes DM, Curran EM, et al. Estradiol activates mast cells via a non-genomic estrogen receptor-alpha and calcium influx. Mol Immunol.44 (2007) 8):1977–85. doi: 10.1016/j.molimm.2006.09.030
31. Tan ST, Wallis RA, He Y, Davis PF. Mast cells and he27mangioma. Plast Reconstr Surg (2004) 113(3):999–1011. doi: 10.1097/01.prs.0000105683.10752.a6
32. Dethlefsen SM, Mulliken JB, Glowacki J. An ultrastructural study of mast cell interactions in hemangiomas. Ultrastruct Pathol (1986) 10:175–83. doi: 10.3109/01913128609014593
33. Majorini MT, Cancila V, Rigoni A, Botti L, Dugo M, Triulzi T, et al. Infiltrating mast cell-mediated stimulation of estrogen receptor activity in breast cancer cells promotes the luminal phenotype. Cancer Res (2020) 80(11):2311–24. doi: 10.1158/0008-5472.CAN-19-3596
34. Marks RM, Roche WR, Czerniecki M, Penny R, Nelson DS. Mast cell granules cause proliferation of human microvascular endothelial cells. Lab Investig (1986) 55(3):289–94.
35. Ye Y, Zhong H, Dou L, Song W, Dong C, Lu W, et al. Propranolol inhibits the angiogenic capacity of hemangioma endothelia via blocking β-adrenoceptor in mast cell. Pediatr Res (2021) 92(2):424–9. doi: 10.1038/s41390-021-01683-4
36. de Souza Junior DA, Santana AC, da Silva EZM, Oliver C, Jamur MC. The role of mast cell specific chymases and tryptases in tumor angiogenesis. BioMed Res Int (2015) 13:142359. doi: 10.1155/2015/142359
37. McHale C, Mohammed Z, Gomez G. Human skin-derived mast cells spontaneously secrete several angiogenesis-related factors. Front Immunol (2019) 10:1445. doi: 10.3389/fimmu.2019.01445
38. Lee BS, Stewart EA, Sahakian M, Nowak RA. Interferon-alpha is a potent inhibitor of basic fibroblast growth factor-stimulated cell proliferation in human uterine cells. Am J Reprod Immunol (1998) 40:19–25. doi: 10.1111/j.1600-0897.1998.tb00383.x
39. Akl MR, Nagpal P, Ayoub NM, Tai B, Prabhu SA, Capac CM, et al. Molecular and clinical significance of fibroblast growth factor 2 (FGF2 /bFGF) in Malignancies of solid and hematological cancers for personalized therapies. Oncotarget. (2016) 7(28):44735–62. doi: 10.18632/oncotarget.8203
40. Qin Y, Zhang C. The regulatory role of IFN-gamma on the proliferation and differentiation of hematopoietic stem and progenitor cells. Stem Cell Rev (2017) 13(6):705–12. doi: 10.1007/s1201501797611
41. Yuan WL, Qin XJ, Wang XK. Expression and correlation of mast cell, Clusterin/apoJ and transforming growth factor-beta in the different stages of human dermal hemangioma. West China J Stomatology (2009) 27(4):361–5.
42. Chen H, Xu Y, Yang G, Zhang Q, Huang X, Yu L, et al. Mast cell chymase promotes hypertrophic scar fibroblast proliferation and collagen synthesis by activating TGF-β1/Smads signaling pathway. Exp Ther Med (2017) 14(5):4438–42. doi: 10.3892/etm.2017.5082
43. Steel R, Day D. Increased apoptosis and secretion of tryptase by mast cells in infantile haemangioma treated with propranolol. Pathology (2014) 46(6):496–500. doi: 10.1097/PAT.0000000000000143
44. Prey S, Leaute-Labreze C, Pain C, Moisan F, Vergnes P, Loot M, et al. Mast cells as possible targets of propranolol therapy: an immunohistological study of beta-adrenergic receptors in infantile haemangiomas. Histopathology (2014) 65(3):436–9. doi: 10.1111/his.12421
45. Baker JG. The selectivity of beta-adrenoceptor antagonists at the human beta1, beta2 and beta3 adrenoceptors. Br J Pharmacol (2005) 144:317–22. doi: 10.1038/sj.bjp.0706048
46. Sun ZJ, Zhao YF, Zhao JH. Mast cells in hemangioma: A double-edged sword. Med Hypotheses (2007) 68(4):805–7. doi: 10.1016/j.mehy.2006.09.012
47. Pahl KS, McLean TW. Infantile hemangioma: A current review. J Pediatr Hematol Oncol (2021) 44(2):31–9. doi: 10.1097/MPH.0000000000002384
Keywords: infantile hemangioma, mast cell, estradiol, proangiogenesis, anti-angiogenesis
Citation: Xia M, Liu W and Hou F (2024) Mast cell in infantile hemangioma. Front. Oncol. 14:1304478. doi: 10.3389/fonc.2024.1304478
Received: 29 September 2023; Accepted: 05 January 2024;
Published: 19 January 2024.
Edited by:
Kaiying Yang, Guangzhou Women and Children’s Medical Center, ChinaReviewed by:
Shinji Kagami, Kanto Central Hospital of the Mutual Aid Association of Public School Teachers, JapanAlessandro Boscarelli, Institute for Maternal and Child Health Burlo Garofolo (IRCCS), Italy
Copyright © 2024 Xia, Liu and Hou. This is an open-access article distributed under the terms of the Creative Commons Attribution License (CC BY). The use, distribution or reproduction in other forums is permitted, provided the original author(s) and the copyright owner(s) are credited and that the original publication in this journal is cited, in accordance with accepted academic practice. No use, distribution or reproduction is permitted which does not comply with these terms.
*Correspondence: Fang Hou, SG91ZmFuZ2Rpb0Bob3RtYWlsLmNvbQ==