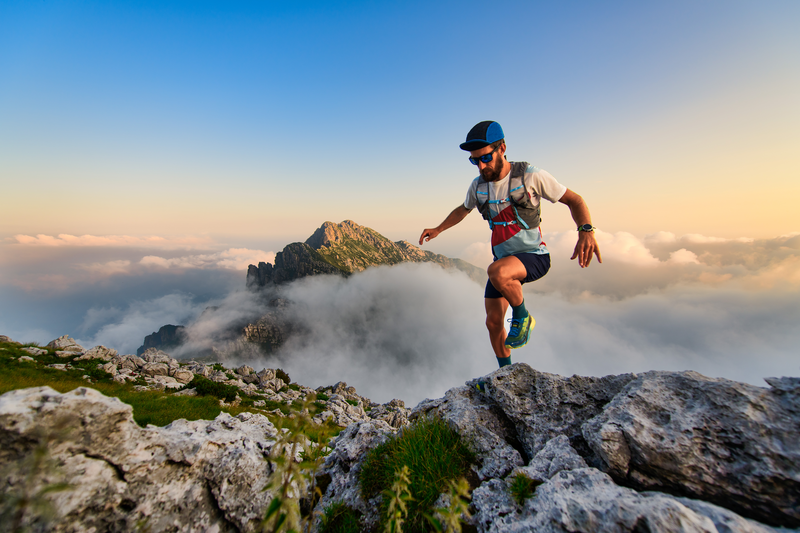
95% of researchers rate our articles as excellent or good
Learn more about the work of our research integrity team to safeguard the quality of each article we publish.
Find out more
SYSTEMATIC REVIEW article
Front. Oncol. , 10 January 2024
Sec. Neuro-Oncology and Neurosurgical Oncology
Volume 13 - 2023 | https://doi.org/10.3389/fonc.2023.1346951
This article is part of the Research Topic Recent Advances in Diagnosis and Treatment of Brain Tumors: From Pediatrics to Adults View all 23 articles
Purpose: The purpose of our meta-analysis and systematic review was to evaluate and compare the diagnostic effectiveness of [18F]FET PET and [18F]FDOPA PET in detecting glioma recurrence.
Methods: Sensitivities and specificities were assessed using the DerSimonian and Laird methodology, and subsequently transformed using the Freeman-Tukey double inverse sine transformation. Confidence intervals were computed employing the Jackson method, while heterogeneity within and between groups was evaluated through the Cochrane Q and I² statistics. If substantial heterogeneity among the studies was observed (P < 0.10 or I² > 50%), we conducted meta-regression and sensitivity analyses. Publication bias was assessed through the test of a funnel plot and the application of Egger’s test. For all statistical tests, except for assessing heterogeneity (P < 0.10), statistical significance was determined when the two-tailed P value fell below 0.05.
Results: Initially, 579 publications were identified, and ultimately, 22 studies, involving 1514 patients(1226 patients for [18F]FET PET and 288 patients for [18F]FDOPA PET), were included in the analysis. The sensitivity and specificity of [18F]FET PET were 0.84 (95% CI, 0.75-0.90) and 0.86 (95% CI, 0.80-0.91), respectively, while for [18F]FDOPA PET, the values were 0.95 (95% CI, 0.86-1.00) for sensitivity and 0.90 (95% CI, 0.77-0.98) for specificity. A statistically significant difference in sensitivity existed between these two radiotracers (P=0.04), while no significant difference was observed in specificity (P=0.58).
Conclusion: It seems that [18F]FDOPA PET demonstrates superior sensitivity and similar specificity to [18F] FET PET. Nevertheless, it’s crucial to emphasize that [18F]FDOPA PET results were obtained from studies with limited sample sizes. Further larger prospective studies, especially head-to-head comparisons, are needed in this issue.
Systematic Review Registration: identifier CRD42023463476
Glioma, a primary tumor of the central nervous system, represents a formidable challenge in the realm of oncology due to its infiltrative nature and variable biological behavior (1, 2).Nevertheless, a few months into treatment, numerous patients experience pseudoprogression or radiation necrosis, conditions frequently indistinguishable from tumor recurrence (3). Given the potential aggressiveness of glioma recurrence, early detection is paramount in facilitating interventions that can potentially extend patient survival and improve their quality of life (4).
Historically, conventional imaging modalities such as computed tomography (CT) and magnetic resonance imaging (MRI) have played a pivotal role in glioma diagnosis and monitoring (5).While these methods have provided essential insights into tumor structure and volume, they have shown limitations in distinguishing between active tumor tissue and post-treatment changes, often leading to equivocal results (6). CT scans utilize X-rays to create detailed cross-sectional images of the brain, allowing clinicians to visualize the tumor’s location, size, and its impact on surrounding structures. However, CT scans are limited in their capacity to differentiate different types of brain tissue with precision. This lack of specificity can lead to difficulties in distinguishing active tumor tissue from non-cancerous changes, such as post-treatment radiation effects or edema, which can yield false-positive results. MRI, a non-invasive imaging technique, offers superior soft tissue contrast and is especially valuable in delineating tumor boundaries and identifying associated brain edema (4). However, similar to CT, MRI also faces challenges when it comes to distinguishing between recurrent tumor and radiation-induced changes (7). Glioma recurrence can present with subtle changes that may overlap with post-treatment effects, causing diagnostic ambiguity (2, 8). These limitations have spurred the exploration of advanced imaging techniques that can offer improved specificity and sensitivity in detecting glioma recurrence (9, 10).
A significant development in this pursuit is the application of positron emission tomography (PET) imaging using radiolabeled amino acids like [18F]FET (O-(2-[18F]fluoroethyl)-L-tyrosine) and [18F]FDOPA (6-[18F]fluoro-L-DOPA). These radiotracers have shown promise in glioma recurrence diagnosis by capitalizing on the increased metabolic activity of tumor cells. [18F]FET is an amino acid analog that is actively transported into tumor cells, reflecting increased amino acid metabolism associated with malignancy (11, 12), known for its minimal uptake in normal brain tissue and rapid clearance from non-tumor cells, displays a distribution pattern predominantly focused within the tumor, enhancing the contrast between malignant and healthy tissues (13). Conversely, [18F]FDOPA PET relies on the radiotracer 6-[18F]fluoro-L-DOPA, which is a precursor of dopamine and is actively transported into cells (14). Like [18F]FET PET, [18F]FDOPA PET can detect regions of heightened metabolic activity, but it does so by targeting amino acid metabolism differently. [18F]FDOPA, on the other hand, shows a somewhat different biodistribution, characterized by a higher basal level of uptake in normal brain tissue but still demonstrates a significant increase in uptake in tumor cells (15). This distinction in biodistribution between [18F]FET and [18F]FDOPA is pivotal in their application for glioma recurrence detection and forms a basis for ongoing comparative studies. Some studies suggest that [18F]FET PET may offer superior diagnostic accuracy due to its specificity for amino acid transport, while others argue that [18F]FDOPA PET’s ability to probe different aspects of amino acid metabolism makes it a preferable choice (12, 14).
In light of the ongoing debate surrounding the diagnostic accuracy of [18F]FET PET and [18F]FDOPA PET in glioma recurrence, this systematic review and meta-analysis seek to provide a rigorous and evidence-based comparison of these imaging techniques. Our primary objective is to assess the diagnostic performance of [18F]FET PET and [18F]FDOPA PET in detecting glioma recurrence, including their sensitivity and specificity.
Our review has been registered with PROSPERO, the international prospective register of systematic reviews, under the identifier CRD42023463476.
A comprehensive search was conducted of the PubMed and Embase databases for all available literatures through September 10, 2023 based on the following combination of terms:(1)Positron-Emission Tomography OR PET OR Positron-Emission Tomography; (2)Regeneration OR Recurrence OR pseudoprogression OR recurrent OR relapse OR Recrudescence OR radionecrosis;(3) Glioma OR Glioma OR Glial Cell Tumor OR Mixed Glioma OR Malignant Glioma;(4) fluoroethyltyrosine OR FET OR fluorodopa F-18 OR FDOPA OR fluorodopa OR 18F-dopa. Studies that were potentially related were also enclosed from the reference lists.
Only studies that met all of the following condition were included: (1) Articles evaluating the diagnostic efficiency of [18F]FET PET or [18F]FDOPA PET in detecting glioma recurrence; (2) Patients under suspicion of recurrent glioma, without any limitations related to age, gender, race, or geographical origin; (3) A prerequisite for inclusion is a minimum of 10 patients or lesions.; (3) The reference standard included histopathological confirmation or imaging follow-up, a requirement that should be explicitly stated in the article; (4) True positive (TP), false positive (FP), true negative (TN), false negative (FN) data could be extracted. The exclusion condition were: (1) Irrelevant topic; (2) Duplicated articles; (3) Cell or animal experiments; (4) Non-English articles; (5) Abstract, editorial comments, letters, case reports, review and meta-analyses. After reviewing the titles and abstracts of the articles based on the incorporation and exclusion criteria, we evaluated the full-text variants of the selected articles to confirm their adherence to the inclusion criteria. Any disagreements between scholars were solved by consensus.
Using the Quality Assessment of Diagnostic Performance Studies (QUADAS-2) methodology (16), two independent researchers assessed the quality of the included studies. They evaluated each study’s risk of bias and applicability, rating them as either high, low, or unclear in these aspects. In case of any disputes, a third reviewer was consulted for resolution. The analysis was conducted using RevMan (version 5.4).
Data extraction for all incorporated papers was carried out separately by two researchers(Table 1). The data that were extracted included: (1) The author, year of publication; (2) Study characteristics including country, study design, analysis, duration, reference standard; (3) Patient characteristics including variety of patients, mean/median age; (4) Technical characteristics including types of tracers, parameter, TP, FP, FN, TN. Data were manually accessed from the literature, tables, and figures when not clearly stated. If the article lacked sufficient information, we will contact the corresponding authors by email and request further data or interpretation. Any disagreements between the two researchers were consequently resolved by consensus.
The sensitivities and specificities were evaluated using the DerSimonian and Laird method and transformed with the Freeman-Tukey double inverse sine transformation. The confidence intervals were calculated using the Jackson method. The Cochrane Q and I² statistics were used to assess the heterogeneity within and between groups. If the heterogeneity between the studies differed significantly (P < 0.10 or I² > 50%), meta-regression analysis and sensitivity analysis were performed by reassessing the sensitivities or specificities following the omission of articles one by one. This was done to evaluate the robustness of the overall sensitivities or specificities and to identify single studies that may contribute to heterogeneity.
Publication bias was assessed through a funnel plot and Egger’s test. Except for heterogeneity (P < 0.10), a two-tailed p-value below 0.05 was considered statistically significant for all statistical tests. Statistical analyses were performed using the R software for statistical computing and graphics version 4.3.1.
The initial search yielded a total of 579 publications. After eliminating 112 duplicated studies, we identified 467 unique studies. Upon reviewing the titles and abstracts, 437 studies were excluded. Among the remaining results, 4 lacked available data, 2 had fewer than 10 patients, and 2 utilized different radiotracers. Finally, 22 studies assessing the diagnostic accuracy of glioma recurrence diagnosis, involving 1514 patients, were included in the analysis. This encompassed 17 articles specifically focusing on [18F]FET PET (11, 17–32) and an additional 5 articles centered on [18F]FDOPA PET (33–37). The PRISMA flow diagram of the study selection process was shown in Figure 1.
Table 1 presents the study characteristics and technical details derived from the 22 selected studies, encompassing a total of 1514 patients. Additionally, we conducted an assessment of the study quality, utilizing the Quality Assessment of Diagnostic Accuracy Studies (QUADAS-2) tool (16). The quality evaluation graph elucidated that the primary area of high-risk bias concerns was centered around patient selection (Figure 2), primarily due to the fact that many of the studies did not involve consecutive patient recruitment. In general, the risk of bias in the articles was deemed acceptable.
The pooled sensitivity for glioma recurrence was 0.84 (95% CI, 0.75-0.90) for [18F]FET PET and 0.95 (95% CI, 0.86-1.00) for [18F]FDOPA PET (Figure 3). Likewise, the pooled specificity for [18F]FET PET was 0.86 (95% CI, 0.80-0.91), while for [18F]FDOPA PET, it was 0.90 (95% CI, 0.77-0.98)(Figure 4). A statistically significant difference in sensitivity existed between these two radiotracers (P=0.04), while no significant difference was observed in specificity (P=0.58).
Regarding the sensitivity of [18F]FET PET and [18F]FDOPA PET for glioma recurrence, the I2 was 82%, 75%, respectively. In terms of the specificity of [18F]FET PET and [18F]FDOPA PET, the I2 were 57% and 63%. For [18F] FET PET, we did not find the reason for its sensitivity heterogeneity through sensitivity analysis and meta-regression analysis (Figure 5) (Table 2). This may be related to significant differences in the study duration of different studies and many of the studies did not involve consecutive patient recruitment. The meta-regression analysis showed that the reference standard (P=0.01 for specificity) may account for the heterogeneity (Table 2). Sensitivity analysis, excluding data from Kebir et al. (25) and Maurer et al. (24),resulted in a combined specificity of 0.83 (95% CI: 0.78-0.87) and 0.83 (95% CI: 0.81-0.92) with low heterogeneity (I2 = 31% and I2 = 47%), respectively (Figure 6). For [18F]FDOPA PET, sensitivity analysis by excluding data from Rozenblum et al. (33) reported a combined specificity of 0.96(95% CI: 0.89-1.00), with an acceptable heterogeneity (I2 = 0%) (Figure 7). Sensitivity analysis, after the exclusion of data from Rozenblum et al. (33), yielded a combined sensitivity of 0.98 (95% CI: 0.92-1.00) with minimal heterogeneity (I2 = 33%) (Figure 8).
Figure 5 Sensitivity analysis evaluating heterogeneity in [18F]FET PET sensitivity for glioma recurrence diagnosis.
Figure 6 Sensitivity analysis evaluating heterogeneity in [18F]FET PET specificity for glioma recurrence diagnosis.
Figure 7 Sensitivity analysis evaluating heterogeneity in [18F]FDOPA PET sensitivity for glioma recurrence diagnosis.
Figure 8 Sensitivity analysis evaluating heterogeneity in [18F]FDOPA PET specificity for glioma recurrence diagnosis.
The funnel plot asymmetry test revealed a significant publication bias regarding the sensitivity of [18F]FET PET, as indicated by Egger’s test (P=0.01), no significant publication bias was found in relation to the specificity of [18F]FET PET (P=0.06). No notable publication bias was observed in sensitivity and specificity for [18F]FDOPA PET (P=0.25, 0.86).
The detection of recurrent signs during post-treatment follow-up for glioma patients portends an unfavorable prognosis. Several studies indicate that patients experiencing their first recurrence have a median survival time of only 9 to 10 months (38). The central question that has spurred extensive debate within the neuro-oncology community revolves around the optimal choice between [18F]FET PET and [18F]FDOPA PET for the diagnosis of glioma recurrence (14, 39–41).
It seems that [18F]FDOPA PET demonstrates superior sensitivity and similar specificity to [18F] FET PET. [18F]FET PET exhibits a pooled sensitivity of 0.84 (95% CI, 0.75-0.90) and specificity of 0.86 (95% CI, 0.80-0.91), while [18F]FDOPA PET demonstrates a pooled sensitivity of 0.95 (95% CI, 0.86-1.00) and specificity of 0.90 (95%CI, 0.77-0.98).These results underscore that both radiotracers are valuable tools in clinical practice (14). It seems that [18F]FDOPA PET demonstrates superior sensitivity in detecting glioma recurrence when contrasted with [18F]FET PET. This may be due to their slightly different mechanisms of action. [18F]FET, an amino acid analog, is transported into tumor cells via amino acid transporters. It capitalizes on the increased amino acid metabolism observed in malignant tissue (42). Conversely, [18F]FDOPA, a precursor of dopamine, is actively transported into cells and reflects increased amino acid metabolism as well. Its advantage lies in targeting different aspects of amino acid metabolism, which may contribute to its diagnostic sensitivity in distinguishing between recurrent tumor and treatment-related changes (43).
Despite numerous published studies, the selection of the ideal radiotracer for discriminating between authentic glioma recurrence and spurious progression remains undetermined. Previously, two meta-analyses regarding [18F]FET PET or [18F]FDOPA PET for glioma recurrence have been conducted and published. According to a meta-analysis by Yu et al. (14), the findings suggest that [18F]FDOPA PET exhibited superior diagnostic performance in patients with glioma recurrence. In summary, within the glioma subgroup, [18F]FDOPA PET demonstrated superior ability across all outcomes compared to [18F]FET PET: sensitivity (0.94 vs. 0.78) and specificity (0.89 vs. 0.75). However, in this article, all data pertaining to the diagnosis of glioma recurrence using [18F]FDOPA PET were sourced from a compilation of three studies (comprising 10 studies) conducted by the same research institution and authored by Karunanithi et al. (37, 43, 44) This circumstance could potentially undermine the reliability of the research data, consequently impacting the outcomes of subgroup analysis. In 2023, Tian et al (12). conducted a systematic review and meta-analysis of the diagnostic performance of different PET imaging agents for glioma recurrence. They included 15 articles that met the inclusion criteria, and ultimately showed that [18F]FET had the highest SUCRA values (diagnostic performance) in sensitivity, specificity, positive predictive value, and accuracy, followed by 18F-FDOPA. Indicating that [18F]FET is one of the most popular imaging agents for glioma recurrence. However due to the limitations of network meta-analysis, articles that only evaluate individual [18F]FET PET or [18F]FDOPA PET were not included, resulting in many available articles being excluded, further affecting the credibility of their articles. While prior meta-analyses have explored this topic to varying degrees, several factors differentiate our study and make it a valuable addition to the existing body of literature. One of the critical strengths of our analysis is the incorporation of the most recent and up-to-date studies (9, 17–22, 33–35). This inclusion ensures that our findings are aligned with the latest research developments, providing the most relevant insights for clinical practice.
Heterogeneity is an inherent challenge in meta-analyses, and it was indeed observed in our study, there was high heterogeneity in [18F]FET PET (sensitivity and specificity) and [18F]FDOPA PET (sensitivity and specificity). In order to find out the source of the heterogeneity and improve the reliability of our research results, we have adopted several strategies such as meta-regression and sensitivity analysis. For [18F] FET PET, we were unable to identify the cause of sensitivity heterogeneity through sensitivity analysis and meta-regression. One possible source of heterogeneity is the significant difference in research duration between included studies. Some studies encompassed longer follow-up periods, while others had relatively shorter intervals. This temporal variability can introduce heterogeneity in the assessment of glioma recurrence due to changes in disease progression and treatment response over time. Another contributing factor is the observation that many studies did not involve consecutive recruitment of patients. This non-consecutive recruitment approach can introduce selection bias, as patients with differing disease characteristics or treatment histories may be included, affecting the overall diagnostic accuracy. Meta-regression analysis showed that reference standard was the possible cause of specificity heterogeneity. Sensitivity analysis by excluding data from Kebir et al. (25) and Maurer et al. (24) demonstrated a combined specificity of 0.83 and 0.83 with low heterogeneity (I² = 31%, I² = 47%). This variance could be attributed to the distinct impact of various chemotherapy regimens on the frequency and characteristics of glioma pseudoprogression. For [18F]FDOPA PET, sensitivity analysis by excluding data from Rozenblum et al. (33) showed a combined specificity of 0.96, with an satisfactory heterogeneity (I² = 0%), sensitivity analysis by excluding data from Rozenblum et al. (33) yielded a combined sensitivity of 0.98, with low heterogeneity (I² = 33%), which could be explained by different cut-off thresholds. However, difference in imaging protocols, such as radiotracer dosage, imaging timing, and scanner technology, can also contribute to heterogeneity.
When assessing the advantages and disadvantages of [18F]FET PET and [18F]FDOPA PET for glioma recurrence diagnosis, it is essential to consider not only diagnostic accuracy but also practical aspects such as cost and accessibility. [18F]FDOPA PET exhibits commendable sensitivity in detecting glioma recurrence, making it a valuable tool for identifying subtle disease progression. Its ability to probe various aspects of amino acid metabolism allows [18F]FDOPA PET to effectively differentiate between active tumor tissue and treatment-related changes, enhancing diagnostic accuracy (45). But [18F]FDOPA PET can be cost-prohibitive for some healthcare systems and may be less accessible in certain regions, limiting its widespread use. While [18F]FDOPA PET has shown promise, it is relatively newer compared to [18F]FET PET, which has a longer history in clinical practice (46, 47). [18F]FET PET, while effective, may exhibit slightly lower sensitivity compared to [18F]FDOPA PET in specific cases. Because the dependence of [18F] FET on amino acid transporters may be affected by the integrity of the blood-brain barrier, in some cases affecting its accuracy (41). However, [18F] FET PET is more widespread and cheaper than [18F] FDOPA PET, making it a practical choice for many clinical environments (46). The choice between these two imaging agents should be guided by careful consideration of patient-specific factors, clinical context, cost, and accessibility. Further larger studies that focus on cost-effective comparison were needed.
[18F] FDOPA PET and [18F] FET PET are specialized forms of positron emission tomography that utilize specific radiotracers to target and visualize brain tumors. MRI, on the other hand, uses magnetic fields and radio waves to create detailed images of the brain (48).The effectiveness of [18F] FDOPA PET and [18F] FET PET lies in their ability to detect changes at a molecular level, often before these changes are visible on MRI. One study by Xiaoxue T et al. conducted a Bayesian network meta-analysis to evaluate the diagnostic accuracy of six different imaging modalities, including [18F] FDOPA PET and [18F] FET PET, for differentiating glioma recurrence from post-radiotherapy changes. The study revealed that [18F] FDOPA PET has the highest sensitivity (0.84) among the evaluated modalities, indicating its effectiveness in correctly identifying true positive cases of recurrent glioma. For [18F] FET PET, the sensitivity is 0.73, which is also relatively high, though slightly lower than [18F] FDOPA PET. MRI had the highest specificity (0.81), demonstrating its superior accuracy in correctly identifying non-recurrent cases (12). This suggests that in clinical practice, combining these imaging techniques could offer a more balanced and comprehensive diagnostic approach, utilizing the high sensitivity of PET tracers and the high specificity of MRI.
It is imperative to acknowledge the limitations of this systematic review and meta-analysis. First, only five studies provided adequate data to assess the diagnostic performance of [18F]FDOPA PET in glioma recurrence detection, resulting in a limited sample size (33–37). Second, the heterogeneity observed in our study remains a challenge that impacts the generalizability of our findings. While our sensitivity analysis and meta-regression provided valuable insights, some degree of unexplained heterogeneity still exist. Third, the diagnostic performance of [18F]FET PET and [18F]FDOPA PET may be influenced by various factors not accounted for in our analysis, such as the specific tracer dosage, timing of imaging, and variations in scanner technology. Standardization of these aspects in future research would contribute to a more comprehensive understanding of these imaging modalities.
In light of the findings mentioned earlier, it seems that [18F]FDOPA PET demonstrates superior sensitivity and similar specificity to [18F] FET PET. Nevertheless, it’s crucial to emphasize that [18F]FDOPA PET results were obtained from studies with limited sample sizes. Further larger prospective studies, especially head-to-head comparisons, are need in this issue.
The original contributions presented in the study are included in the article/Supplementary Material. Further inquiries can be directed to the corresponding author.
PY: Data curation, Formal Analysis, Methodology, Software, Writing – original draft. YW: Data curation, Software, Writing – original draft. FS: Formal Analysis, Methodology, Writing – original draft. YC: Conceptualization, Supervision, Validation, Visualization, Writing – review & editing.
The author(s) declare that no financial support was received for the research, authorship, and/or publication of this article.
The authors declare that the research was conducted in the absence of any commercial or financial relationships that could be construed as a potential conflict of interest.
All claims expressed in this article are solely those of the authors and do not necessarily represent those of their affiliated organizations, or those of the publisher, the editors and the reviewers. Any product that may be evaluated in this article, or claim that may be made by its manufacturer, is not guaranteed or endorsed by the publisher.
The Supplementary Material for this article can be found online at: https://www.frontiersin.org/articles/10.3389/fonc.2023.1346951/full#supplementary-material
1. Ostrom QT, Patil N, Cioffi G, Waite K, Kruchko C, Barnholtz-Sloan JS. CBTRUS statistical report: primary brain and other central nervous system tumors diagnosed in the United States in 2013-2017. Neuro Oncol (2020) 22(12 Suppl 2):iv1–iv96. doi: 10.1093/neuonc/noaa200
2. Brandsma D, van den Bent MJ. Pseudoprogression and pseudoresponse in the treatment of gliomas. Curr Opin Neurol (2009) 22(6):633–8. doi: 10.1097/WCO.0b013e328332363e
3. Parvez K, Parvez A, Zadeh G. The diagnosis and treatment of pseudoprogression, radiation necrosis and brain tumor recurrence. Int J Mol Sci (2014) 15(7):11832–46. doi: 10.3390/ijms150711832
4. Verma N, Cowperthwaite MC, Burnett MG, Markey MK. Differentiating tumor recurrence from treatment necrosis: a review of neuro-oncologic imaging strategies. Neuro Oncol (2013) 15(5):515–34. doi: 10.1093/neuonc/nos307
5. Wu Y, Den Z, Lin Y. Accuracy of susceptibility-weighted imaging and dynamic susceptibility contrast magnetic resonance imaging for differentiating high-grade glioma from primary central nervous system lymphomas: meta-analysis. World Neurosurg (2018) 112:e617–e23. doi: 10.1016/j.wneu.2018.01.098
6. Chao ST, Ahluwalia MS, Barnett GH, Stevens GH, Murphy ES, Stockham AL, et al. Challenges with the diagnosis and treatment of cerebral radiation necrosis. Int J Radiat Oncol Biol Phys (2013) 87(3):449–57. doi: 10.1016/j.ijrobp.2013.05.015
7. Zikou A, Sioka C, Alexiou GA, Fotopoulos A, Voulgaris S, Argyropoulou MI. Radiation necrosis, pseudoprogression, pseudoresponse, and tumor recurrence: imaging challenges for the evaluation of treated gliomas. Contrast Media Mol Imaging (2018) 2018:6828396. doi: 10.1155/2018/6828396
8. la Fougère C, Suchorska B, Bartenstein P, Kreth FW, Tonn JC. Molecular imaging of gliomas with PET: opportunities and limitations. Neuro Oncol (2011) 13(8):806–19. doi: 10.1093/neuonc/nor054
9. Page MJ, McKenzie JE, Bossuyt PM, Boutron I, Hoffmann TC, Mulrow CD, et al. The PRISMA 2020 statement: an updated guideline for reporting systematic reviews. Bmj (2021) 372:n71. doi: 10.1136/bmj.n71
10. Zuniga RM, Torcuator R, Jain R, Anderson J, Doyle T, Ellika S, et al. Efficacy, safety and patterns of response and recurrence in patients with recurrent high-grade gliomas treated with bevacizumab plus irinotecan. J Neurooncol (2009) 91(3):329–36. doi: 10.1007/s11060-008-9718-y
11. Bashir A, Mathilde Jacobsen S, Mølby Henriksen O, Broholm H, Urup T, Grunnet K, et al. Recurrent glioblastoma versus late posttreatment changes: diagnostic accuracy of O-(2-[18F]fluoroethyl)-L-tyrosine positron emission tomography (18F-FET PET). Neuro Oncol (2019) 21(12):1595–606. doi: 10.1093/neuonc/noz166
12. Xiaoxue T, Yinzhong W, Meng Q, Lu X, Lei J. Diagnostic value of PET with different radiotracers and MRI for recurrent glioma: a Bayesian network meta-analysis. BMJ Open (2023) 13(3):e062555. doi: 10.1136/bmjopen-2022-062555
13. Calabria FF, Chiaravalloti A, Jaffrain-Rea ML, Zinzi M, Sannino P, Minniti G, et al. 18F-DOPA PET/CT physiological distribution and pitfalls: experience in 215 patients. Clin Nucl Med (2016) 41(10):753–60. doi: 10.1097/RLU.0000000000001318
14. Yu J, Zheng J, Xu W, Weng J, Gao L, Tao L, et al. Accuracy of (18)F-FDOPA positron emission tomography and (18)F-FET positron emission tomography for differentiating radiation necrosis from brain tumor recurrence. World Neurosurg (2018) 114:e1211–e24. doi: 10.1016/j.wneu.2018.03.179
15. Fuenfgeld B, Mächler P, Fischer DR, Esposito G, Rushing EJ, Kaufmann PA, et al. Reference values of physiological 18F-FET uptake: Implications for brain tumor discrimination. PloS One (2020) 15(4):e0230618. doi: 10.1371/journal.pone.0230618
16. Whiting PF, Rutjes AW, Westwood ME, Mallett S, Deeks JJ, Reitsma JB, et al. QUADAS-2: a revised tool for the quality assessment of diagnostic accuracy studies. Ann Intern Med (2011) 155(8):529–36. doi: 10.7326/0003-4819-155-8-201110180-00009
17. Paprottka KJ, Kleiner S, Preibisch C, Kofler F, Schmidt-Graf F, Delbridge C, et al. Fully automated analysis combining [(18)F]-FET-PET and multiparametric MRI including DSC perfusion and APTw imaging: a promising tool for objective evaluation of glioma progression. Eur J Nucl Med Mol Imaging (2021) 48(13):4445–55. doi: 10.1007/s00259-021-05427-8
18. Skoblar Vidmar M, Doma A, Smrdel U, Zevnik K, Studen A. The value of FET PET/CT in recurrent glioma with a different IDH mutation status: the relationship between imaging and molecular biomarkers. Int J Mol Sci (2022) 23(12):6787. doi: 10.3390/ijms23126787
19. Müller M, Winz O, Gutsche R, Leijenaar RTH, Kocher M, Lerche C, et al. Static FET PET radiomics for the differentiation of treatment-related changes from glioma progression. J Neurooncol (2022) 159(3):519–29. doi: 10.1007/s11060-022-04089-2
20. Puranik AD, Rangarajan V, Dev ID, Jain Y, Purandare NC, Sahu A, et al. Brain FET PET tumor-to-white mater ratio to differentiate recurrence from post-treatment changes in high-grade gliomas. J Neuroimaging (2021) 31(6):1211–8. doi: 10.1111/jon.12914
21. Steidl E, Langen KJ, Hmeidan SA, Polomac N, Filss CP, Galldiks N, et al. Sequential implementation of DSC-MR perfusion and dynamic [(18)F]FET PET allows efficient differentiation of glioma progression from treatment-related changes. Eur J Nucl Med Mol Imaging (2021) 48(6):1956–65. doi: 10.1007/s00259-020-05114-0
22. Werner JM, Weller J, Ceccon G, Schaub C, Tscherpel C, Lohmann P, et al. Diagnosis of pseudoprogression following lomustine-temozolomide chemoradiation in newly diagnosed glioblastoma patients using FET-PET. Clin Cancer Res (2021) 27(13):3704–13. doi: 10.1158/1078-0432.CCR-21-0471
23. Lohmann P, Elahmadawy MA, Gutsche R, Werner JM, Bauer EK, Ceccon G, et al. FET PET radiomics for differentiating pseudoprogression from early tumor progression in glioma patients post-chemoradiation. Cancers (Basel) (2020) 12(12):3835. doi: 10.3390/cancers12123835
24. Maurer GD, Brucker DP, Stoffels G, Filipski K, Filss CP, Mottaghy FM, et al. (18)F-FET PET imaging in differentiating glioma progression from treatment-related changes: A single-center experience. J Nucl Med (2020) 61(4):505–11. doi: 10.2967/jnumed.119.234757
25. Kebir S, Schmidt T, Weber M, Lazaridis L, Galldiks N, Langen KJ, et al. A preliminary study on machine learning-based evaluation of static and dynamic FET-PET for the detection of pseudoprogression in patients with IDH-wildtype glioblastoma. Cancers (Basel) (2020) 12(11):3080. doi: 10.3390/cancers12113080
26. Kertels O, Mihovilovic MI, Linsenmann T, Kessler AF, Tran-Gia J, Kircher M, et al. Clinical utility of different approaches for detection of late pseudoprogression in glioblastoma with O-(2-[18F]Fluoroethyl)-L-tyrosine PET. Clin Nucl Med (2019) 44(9):695–701. doi: 10.1097/RLU.0000000000002652
27. Pyka T, Hiob D, Preibisch C, Gempt J, Wiestler B, Schlegel J, et al. Diagnosis of glioma recurrence using multiparametric dynamic 18F-fluoroethyl-tyrosine PET-MRI. Eur J Radiol (2018) 103:32–7. doi: 10.1016/j.ejrad.2018.04.003
28. Dunkl V, Cleff C, Stoffels G, Judov N, Sarikaya-Seiwert S, Law I, et al. The usefulness of dynamic O-(2-18F-fluoroethyl)-L-tyrosine PET in the clinical evaluation of brain tumors in children and adolescents. J Nucl Med (2015) 56(1):88–92. doi: 10.2967/jnumed.114.148734
29. Galldiks N, Dunkl V, Stoffels G, Hutterer M, Rapp M, Sabel M, et al. Diagnosis of pseudoprogression in patients with glioblastoma using O-(2-[18F]fluoroethyl)-L-tyrosine PET. Eur J Nucl Med Mol Imaging (2015) 42(5):685–95. doi: 10.1007/s00259-014-2959-4
30. Herrmann K, Czernin J, Cloughesy T, Lai A, Pomykala KL, Benz MR, et al. Comparison of visual and semiquantitative analysis of 18F-FDOPA-PET/CT for recurrence detection in glioblastoma patients. Neuro Oncol (2014) 16(4):603–9. doi: 10.1093/neuonc/not166
31. Jeong SY, Lee TH, Rhee CH, Cho AR, Il Kim B, Cheon GJ, et al. 3'-deoxy-3'-[(18)F]fluorothymidine and O-(2-[(18)F]fluoroethyl)-L-tyrosine PET in patients with suspicious recurrence of glioma after multimodal treatment: initial results of a retrospective comparative study. Nucl Med Mol Imaging (2010) 44(1):45–54. doi: 10.1007/s13139-009-0007-2
32. Rachinger W, Goetz C, Pöpperl G, Gildehaus FJ, Kreth FW, Holtmannspötter M, et al. Positron emission tomography with O-(2-[18F]fluoroethyl)-l-tyrosine versus magnetic resonance imaging in the diagnosis of recurrent gliomas. Neurosurgery. (2005) 57(3):505–11. doi: 10.1227/01.NEU.0000171642.49553.B0
33. Rozenblum L, Zaragori T, Tran S, Morales-Martinez A, Taillandier L, Blonski M, et al. Differentiating high-grade glioma progression from treatment-related changes with dynamic [(18)F]FDOPA PET: a multicentric study. Eur Radiol (2023) 33(4):2548–60. doi: 10.1007/s00330-022-09221-4
34. Li C, Yi C, Chen Y, Xi S, Guo C, Yang Q, et al. Identify glioma recurrence and treatment effects with triple-tracer PET/CT. BMC Med Imaging (2021) 21(1):92. doi: 10.1186/s12880-021-00624-1
35. Pellerin A, Khalifé M, Sanson M, Rozenblum-Beddok L, Bertaux M, Soret M, et al. Simultaneously acquired PET and ASL imaging biomarkers may be helpful in differentiating progression from pseudo-progression in treated gliomas. Eur Radiol (2021) 31(10):7395–405. doi: 10.1007/s00330-021-07732-0
36. Zaragori T, Ginet M, Marie PY, Roch V, Grignon R, Gauchotte G, et al. Use of static and dynamic [(18)F]-F-DOPA PET parameters for detecting patients with glioma recurrence or progression. EJNMMI Res (2020) 10(1):56. doi: 10.1186/s13550-020-00645-x
37. Karunanithi S, Bandopadhyaya GP, Sharma P, Kumar A, Singla S, Malhotra A, et al. Prospective comparison of (99m)Tc-GH SPECT/CT and (18)F-FDOPA PET/CT for detection of recurrent glioma: a pilot study. Clin Nucl Med (2014) 39(2):e121–8. doi: 10.1097/RLU.0b013e318279bcd8
38. Xu T, Chen J, Lu Y, Wolff JE. Effects of bevacizumab plus irinotecan on response and survival in patients with recurrent Malignant glioma: a systematic review and survival-gain analysis. BMC Canc (2010) 10:252. doi: 10.1186/1471-2407-10-252
39. Ginet M, Zaragori T, Marie PY, Roch V, Gauchotte G, Rech F, et al. Integration of dynamic parameters in the analysis of (18)F-FDopa PET imaging improves the prediction of molecular features of gliomas. Eur J Nucl Med Mol Imaging (2020) 47(6):1381–90. doi: 10.1007/s00259-019-04509-y
40. Galldiks N, Niyazi M, Grosu AL, Kocher M, Langen KJ, Law I, et al. Contribution of PET imaging to radiotherapy planning and monitoring in glioma patients - a report of the PET/RANO group. Neuro Oncol (2021) 23(6):881–93. doi: 10.1093/neuonc/noab013
41. Evangelista L, Cuppari L, Bellu L, Bertin D, Caccese M, Reccia P, et al. Comparison between 18F-dopa and 18F-fet PET/CT in patients with suspicious recurrent high grade glioma: A literature review and our experience. Curr Radiopharm (2019) 12(3):220–8. doi: 10.2174/1874471012666190115124536
42. Laverman P, Boerman OC, Corstens FH, Oyen WJ. Fluorinated amino acids for tumour imaging with positron emission tomography. Eur J Nucl Med Mol Imaging (2002) 29(5):681–90. doi: 10.1007/s00259-001-0716-y
43. Karunanithi S, Sharma P, Kumar A, Khangembam BC, Bandopadhyaya GP, Kumar R, et al. Comparative diagnostic accuracy of contrast-enhanced MRI and (18)F-FDOPA PET-CT in recurrent glioma. Eur Radiol (2013) 23(9):2628–35. doi: 10.1007/s00330-013-2838-6
44. Karunanithi S, Sharma P, Kumar A, Khangembam BC, Bandopadhyaya GP, Kumar R, et al. 18F-FDOPA PET/CT for detection of recurrence in patients with glioma: prospective comparison with 18F-FDG PET/CT. Eur J Nucl Med Mol Imaging (2013) 40(7):1025–35. doi: 10.1007/s00259-013-2384-0
45. Galldiks N, Stoffels G, Filss C, Rapp M, Blau T, Tscherpel C, et al. The use of dynamic O-(2-18F-fluoroethyl)-l-tyrosine PET in the diagnosis of patients with progressive and recurrent glioma. Neuro Oncol (2015) 17(9):1293–300. doi: 10.1093/neuonc/nov088
46. Langen KJ, Heinzel A, Lohmann P, Mottaghy FM, Galldiks N. Advantages and limitations of amino acid PET for tracking therapy response in glioma patients. Expert Rev Neurother (2020) 20(2):137–46. doi: 10.1080/14737175.2020.1704256
47. Jager PL, Vaalburg W, Pruim J, de Vries EG, Langen KJ, Piers DA. Radiolabeled amino acids: basic aspects and clinical applications in oncology. J Nucl Med (2001) 42(3):432–45.
Keywords: glioma, [18F]FET PET, [18F]FDOPA PET, recurrence, meta-analysis
Citation: Yu P, Wang Y, Su F and Chen Y (2024) Comparing [18F]FET PET and [18F]FDOPA PET for glioma recurrence diagnosis: a systematic review and meta-analysis. Front. Oncol. 13:1346951. doi: 10.3389/fonc.2023.1346951
Received: 30 November 2023; Accepted: 20 December 2023;
Published: 10 January 2024.
Edited by:
Cesare Zoia, San Matteo Hospital Foundation (IRCCS), ItalyReviewed by:
Christian F. Freyschlag, Innsbruck Medical University, AustriaCopyright © 2024 Yu, Wang, Su and Chen. This is an open-access article distributed under the terms of the Creative Commons Attribution License (CC BY). The use, distribution or reproduction in other forums is permitted, provided the original author(s) and the copyright owner(s) are credited and that the original publication in this journal is cited, in accordance with accepted academic practice. No use, distribution or reproduction is permitted which does not comply with these terms.
*Correspondence: Yan Chen, ZHJjaGVueWFuQGpsdS5lZHUuY24=
Disclaimer: All claims expressed in this article are solely those of the authors and do not necessarily represent those of their affiliated organizations, or those of the publisher, the editors and the reviewers. Any product that may be evaluated in this article or claim that may be made by its manufacturer is not guaranteed or endorsed by the publisher.
Research integrity at Frontiers
Learn more about the work of our research integrity team to safeguard the quality of each article we publish.