- 1Department of Pulmonary Medicine, The University of Texas MD Anderson Cancer Center, Houston, TX, United States
- 2Department of Internal Medicine, The University of Texas MD Anderson Cancer Center, Houston, TX, United States
Immunotherapy has revolutionized treatments for both early and advanced cancers, and as their role evolves, their impact on sleep and circadian rhythms continues to unfold. The recognition, evaluation, and treatment of sleep and circadian rhythm disturbance leads to improved symptom management, quality of life and treatment outcomes. An intricate complex relationship exists in the microenvironment with immunity, sleep and the tumor, and these may further vary based on the cancer, addition of standard chemotherapy, and pre-existing patient factors. Sleep and circadian rhythms may offer tools to better utilize immunotherapy in the care of cancer patients, leading to better treatment outcome, reduced symptom burden, and increased quality of life.
Introduction
Immunotherapy has revolutionized treatments for both early and advanced cancers, and as their role evolves, their impact on sleep and circadian rhythms continues to unfold (1, 2). Sleep disturbance may occur at any time during the spectrum of the cancer care continuum including prior to diagnosis, during treatment and years into survivorship. Sleep disruption during cancer treatment can perpetuate and exacerbate the symptom burden for cancer patients (3–6). The recognition, evaluation, and treatment of sleep and circadian rhythm disturbance leads to improved symptom management, quality of life and treatment outcomes (7). Immune checkpoint inhibitors (ICIs) modulate the immune system to target cancer cells, and the interaction between immunity, sleep and circadian rhythm has been well described. Thus, ICIs likely contribute another dimension to the complex interactions between cancer and sleep (3, 4, 7–10). Our objectives are to review the importance of sleep and circadian rhythms in cancer care, to discuss the interplay between sleep, circadian rhythms and immunity and to highlight the interplay of ICIs with sleep, circadian rhythms, immunity and symptom burden (Figure 1).
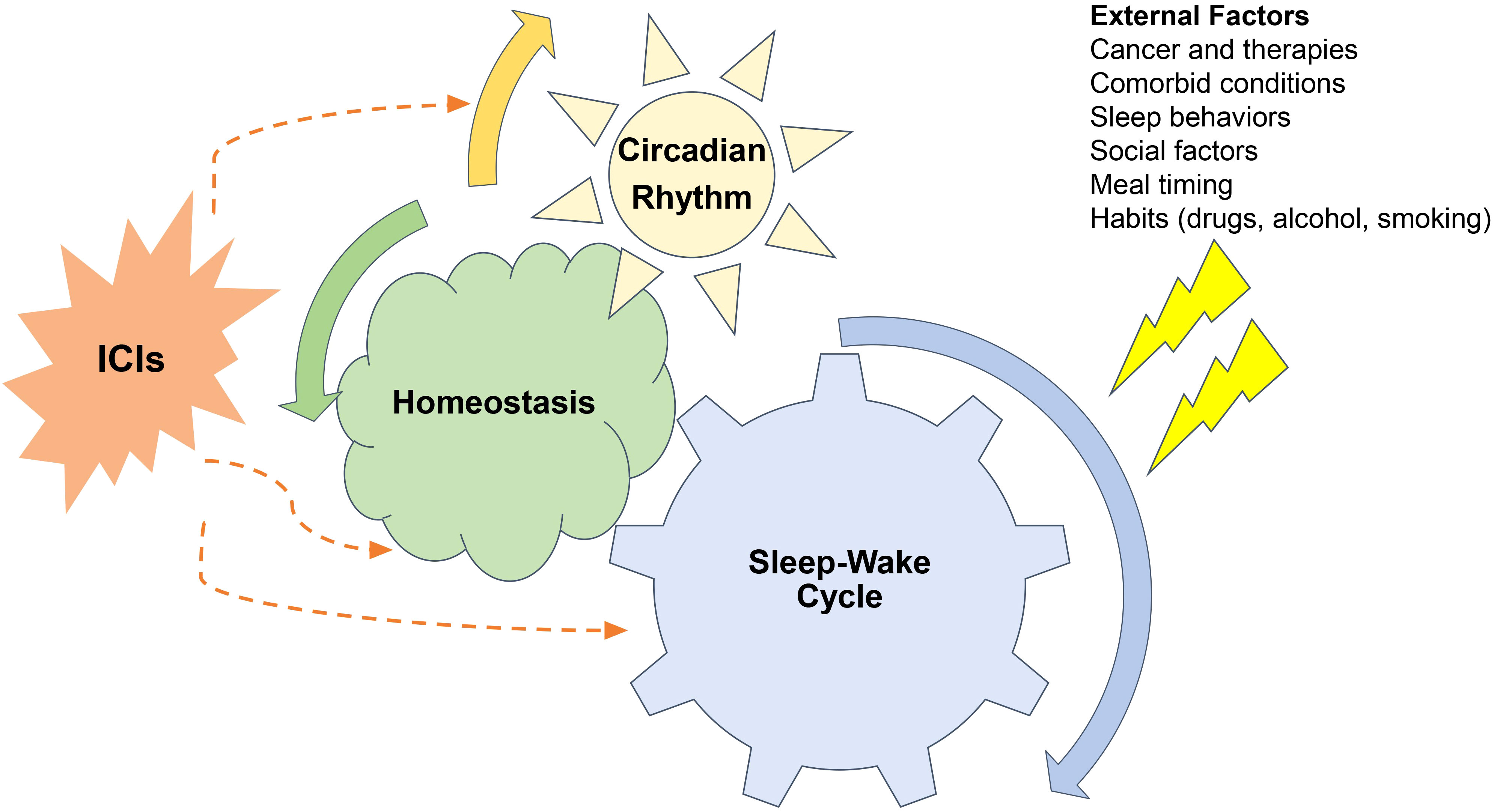
Figure 1 Relationships between sleep-wake cycles, circadian rhythms, cancer and immune checkpoint inhibitors (ICI). Sleep wake cycles are controlled by the brains neural networks and are governed by circadian rhythms. External factors related to cancer, comorbid conditions, sleep behaviors, social factors, meal timings, and other habits all can perturb both sleep-wake cycles and circadian rhythms which disturbing homeostasis. These disturbances impact the function of immune checkpoint inhibitors both on their on-target therapeutic and off- target auto-immune effects.
Immune checkpoint inhibitors
ICI’s main function is to disable T-cell regulation to amplify the impact of the T-cell mediated killing of cancer cells. Immune checkpoints are immune cell functions governed by receptor-ligand interactions which control the activation or inhibition of immune responses. Activation of the immune system is a desired outcome to achieve tumor control but can also lead to autoimmunity and toxicity (11). The discovery of monoclonal antibodies against the inhibitor immune checkpoint CTLA–4 (cytotoxic T lymphocyte antigen 4), PD-1 and PD-L11 (programmed cell death 1 and programmed cell death ligand-1, respectively) have resulted in dramatic antitumor responses by the upregulation of immune activation at various stages of the immune cycle (12).
ICI therapy has been transformational in the care of cancer patients, and they have become a pillar for cancer care including neoadjuvant, adjuvant, primary therapy and the treatment of metastatic disease of numerous cancer types (12–14). Now more than a decade after the Federal Drug Administration (FDA) approval of the first ICI, there is nascent recognition of their impact that these medications may have on sleep and circadian rhythms (15, 16). Since the approval of ipilimumab to treat metastatic melanoma in 2011, several ICIs have been approved to treat a growing list of cancers, and many regimens even include dual immunotherapy to maximize impact. The efficacy and safety have been established in a myriad of clinical trials, but immune-mediated adverse events (irAEs) resulting in organ dysfunction resembling autoimmune diseases can occur (17). The most common irAEs include rash, diarrhea and fatigue, but endocrinopathies, myocarditis, hepatitis, pneumonitis, nephritis and nervous system issues may also occur (18). Thus, ICIs, their irAEs and the underlying cancer may all impact sleep.
Fundamentals of sleep and circadian rhythms
Sleep is a fundamental and basic need for life. Sleep is formally studied by polysomnography and is divided into both non-rapid eye movement (NREM) and rapid eye movement (REM) sleep (19). NREM sleep is further divided into 3 stages including: Stage 1, light sleep or drowsiness; Stage 2 which comprises the largest portion of non-REM sleep; Stage 3, deep or slow-wave sleep. The sensitivity of the cortical response to auditory, tactile, and visual stimuli are correspondingly more depressed during stage 3 sleep compared with stage 1 sleep. REM sleep or paradoxical sleep is a metabolically active period of sleep with saccadic rapid eye movements as the hallmark of this important stage of sleep. Both NREM and REM sleep have critical restorative functions and are essential for cognition, learning, and memory (20). Sleep impacts immune function in a fundamental manner affecting both innate and adaptive immunity (21). Immune function is enhanced by both better sleep quality and optimal sleep duration (20). Perturbations of sleep quality and duration fundamentally impact health and disease and have been shown to impact cancer incidence, cancer-related symptoms, and cancer outcomes (10). Identification of these of these symptoms and disorders is enhanced by the utilization of validated subjective and objective tools to assess sleep and circadian rhythms (Table 1).
The control of sleep is determined by a defined set of hypothalamic and brainstem nuclei primarily with pathways to the thalamus and cortex and is best explained by the 2 process models of sleep regulation with both homeostatic and circadian control. Homeostatic control refers to the concept of “sleep debt” which accrues during wakefulness increases the impetus to sleep (29). Circadian, meaning about a day, rhythms are an approximately 24-hour cyclic rhythm which govern both sleep and wakefulness and are a critical input into sleep regulation and helps maintain and consolidate nocturnal sleep as the homeostatic process abates during the sleep period, as sleep debt is paid off (30). Circadian rhythms originate in the suprachiasmatic nucleus in the brain and fundamentally control biology on a behavioral, physiologic, cellular, and molecular level. Circadian rhythms also drive the visible behaviors of sleep and wakefulness (30). Disrupted circadian rhythms have also been shown to impact health and disease, including cancer incidence, cancer symptoms, and cancer outcomes (31). Furthermore, immune regulation and many aspects especially cellular immunity are also under circadian control (32). These relationships provide a basis on which to better understand the intersection between the circadian clock, cancer and immunotherapy (Figure 2).
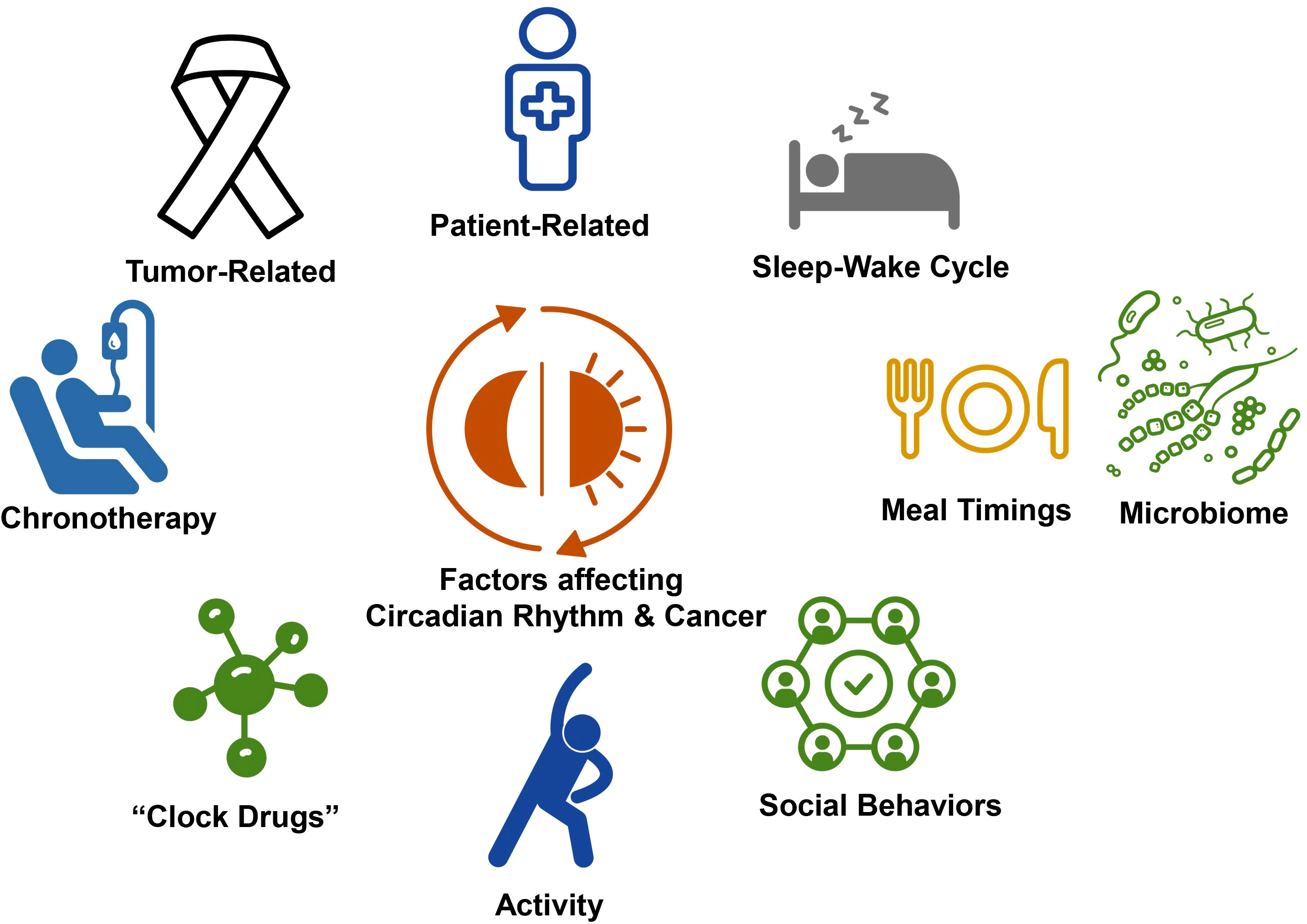
Figure 2 Factors impacting circadian rhythms and cancer. The intersection between circadian, rhythms, and cancer is governed by multiple factors, including patient related factors, such as comorbidities and habits and tumor, related factors. Activity, social behaviors, and meal timings entrain circadian rhythms and sleep wake cycles and may be targets for chronoregulation. There is increasing recognition that the microbiome is bi-directionally impacted by circadian rhythms and sleep-wake cycles. Further opportunity for intervention using circadian rhythm related strategies include chronotherapy and the development of clock drugs, the targeting clock gene expression and activity.
Sleep, circadian rhythms, and cancer
Sleep and circadian disruption are considered risk factors for cancer (33). Sleep disruption is at least 3 times as prevalent in cancer population as in the general population (34). Sleep and circadian disruption have also been shown increase with the severity of disease and the degree of disruption has prognostic value (31). Furthermore, sleep disruption clusters with other symptoms of cancer including pain, mood disturbance, and fatigue (9). Improved sleep quality in cancer patients is associated with a better prognosis and treatment response. Innominate and colleagues demonstrated an 8-month increase in overall survival in patients receiving chemotherapy for metastatic colon cancer who were shown to be objectively at rest by actigraphy during their time in bed (35). Sleep disorders such as obstructive sleep apnea has been shown to increase both cancer incidence and mortality (36, 37). Gozal posits that sleep fragmentation is oncogenic and hypothesizes several mechanisms for this relationship through sleep disruption’s impact on inflammation and immunity, the autonomic nervous system, the hypothalamic- pituitary axis, oxidative stress and hormonal pathways (33, 38).
The circadian clock is a major regulatory pathway for the cell cycle. Clock genes regulate the cell cycle and therefore modulate gene replication, gene expression, and cellular proliferation (39). In a chronic jet lag model, spontaneous hepatocellular carcinoma occurred in mice following a mechanism very similar to that observed in obese humans (40). Genetic abnormalities in clock genes have been shown to be both oncogenic as well as onco-suppressive (41–49). In studies with circadian clock gene mutant animal models, clock gene BMAL1 deficient knockout mice were shown to have greater progression of hematologic malignant disease and breast cancer cell metastasis (50, 51). Furthermore, core circadian genes Per2 and Bmal1 were shown to have cell-autonomous tumor-suppressive roles in transformation and lung tumor progression (52). Disrupted circadian rhythms in humans have consequences for health and disease and highlight the importance of maintaining robust circadian rhythms of sleep and wakefulness (20). Shift work has been classified as a carcinogen due the higher rate of cancer (53, 54). Circadian rhythm has also been shown play a role in the overall symptom burden of cancer and modify levels of fatigue, depression, and sleep disturbance. Circadian rhythm disturbance has been demonstrated as common regulating factor in the manifestation symptom clusters (35, 55–59). Cancer cells carry mutations which disassociate cells from normal circadian control of the cell cycle compared with heathy tissue, and this difference is used to amplify the therapeutic window in cancer chronotherapy (60, 61). Cancer chronotherapy has been shown to improve on-target effects while minimizing off-target adverse effects (31, 62, 63). Circadian rhythm disruption has prognostic value as cancer patients with attenuated circadian rhythms often also have higher mortality (64). These factors show that circadian rhythms are important not only for behavioral control of sleep but for the regulation and treatment of cancer (39).
The impact of sleep and circadian rhythms in cancer patients is primarily twofold. First, quality of life is often dramatically impaired due to insomnia, excessive daytime sleepiness, and fatigue. These often debilitating symptoms decrease opportunities for beneficial lifestyle habits such as exercise, social interaction, and healthy diet (65). Second, sleep and circadian rhythms are coupled to improved symptom control, better treatment outcomes, and extended survival in cancer patients. About 30 to 55% of cancer patients have impaired circadian rest-activity rhythms with attenuated rhythms correlating to more advanced and aggressive cancer. Interventions to improve both sleep disorders and circadian rhythm disorders have been shown to ameliorate cancer symptoms and may impact cancer outcomes (66–68).
Circadian rhythms regulate both immunity as well as the cycle of hormones such as cortisol which impacts immune cell function, and through this control of cortisol secretion, the circadian clock function as a gate that controls many aspects of immune function in cancer including cancer cell antigen release and presentation, activation of effector immune cells, trafficking, tumor infiltration and elimination of cancer cells. These regulatory rules in both tumor surveillance and prevention of oncogenesis highlight the circadian clock’s relevance to cancer immunotherapy (69).
Sleep, circadian rhythms, and immunity
Sleep and circadian rhythms help regulate the immune system impacting health and disease and in the context of cancer, play a role in tumor surveillance and regulation. Sleep and immunity are now thought to bi-directionally linked (20, 70). The impact of sleep and circadian rhythm on inflammatory response, leukocytes and hormones further exemplifies their potential influence with immunotherapy.
Numerous studies have shown that stimulation of the immune system by microbial challenges triggers an inflammatory response promoting sleepiness and in turn that sleep modifies the inflammatory response. Infections can increase NREM sleep through the production of cytokines such as IL-1 and TNFα (21, 71, 72). Just as sleep impacts cognitive learning and memory, it is thought that sleep also impacts the immune system’s ability to recognize learn and recall infectious stimuli modulating the adaptive immune response (20). Sleep enhancement during infection can promote host defense and increase the response to vaccination (73–76). Sleep also affects immunological memory as summarized in vaccination studies. For example, with influenza virus specific antibody titers measured 10 days after vaccination more than doubled in participants who kept their usual bedtime of 7.5 to 8 hours compared to those restricted to 4 hours of sleep. This study also showed that sleep enhances the production of Th1 effector cytokine interferonγ (77). Circadian rhythms regulate the production and function of inflammatory cytokines. For example, TNFα secretion varies in circadian fashion based on the time of an endotoxin challenge (78). Circadian rhythm and clock genes impact gene expression of key cytokines. Disruption of clock gene expression seems to be a common outcome of acute infection and it is suggested by some that the circadian clock itself is an innate immune system sensor that is disabled by infection (79).
Sleep along with circadian factors exerts a significant influence on circulating leukocyte number in the bloodstream. Studies have reported that sleep reduces the numbers of various leukocyte subsets of blood. This is thought to be due to redistribution of cells from the circulation into tissues rather than an effect on proliferation (76). Studies using sleep deprivation show an accumulation of lymphocytes in both tissue and blood. Chronic sleep deprivation which leads to the development of habitual short sleep times may cause the development of low-grade inflammation. This chronic inflammatory state is associated with an increased risk of several diseases including cancer and a decreased ability to mount an adaptive immune response (20). Clock genes and immune processes also regulate the differential maturation of leukocyte subsets as clock genes are required for the differentiation of type II lymphoid cells. Leukocyte trafficking which represents the movement of cells from the bone marrow to the bloodstream and into target organs is also under circadian control. In Bmal1 knockout mice macrophages are unable to sustain mitochondrial function, enhancing succinate dehydrogenase (SDH)-mediated mitochondrial production of reactive oxygen species as well as Hif-1α-dependent metabolic reprogramming and inflammatory damage precipitating an inflammatory and tumor- promoting cellular milieu (80). Clock genes also control neutrophil maturation (81). Furthermore specific clock genes Rev-Erbα and RORα deficient mice have negative effects on the development of and activation of dendritic cell and other antigen presenting cells (APC) critical to pathways involved with immune mediated cancer cell targeting (82). Therefore, it is increasingly clear that circadian gating is part of the core program of the immune system, and thus alteration of this regimen is likely to have widespread ramifications for disease pathogenesis (32). Furthermore, it is in slow wave sleep where high levels of growth hormone, prolactin and aldosterone and nadir levels of cortisol are present. These hormonal factors also may alter T-cell interactions. Low levels of cortisol during slow-wave sleep may allow efficient antigen-presenting cell–T-cell interactions which are important for immunomodulation and targeting cancer cells which contributes to the efficacy of cancer immune therapy (83).
Sleep, circadian rhythms, and cancer immunotherapy
Sleep and immunotherapy
There is a paucity of data on the relationship between sleep disturbance and immunotherapy. In an early study in lung cancer patients undergoing treatment with ICIs, Zarogoulis and associates recorded sleep disturbance using phone questionnaires and polysomnography. Interestingly for immunotherapy patients with a PD-L1 expression greater than 50%, disease response was rapid and associated sleep disturbances decreased rapidly. In contrast, in patients on standard chemotherapy, those with both partial response and stable disease continued to have sleep disturbances. They concluded that although ICIs did not induce sleep disturbance, treatment response may improve sleep disturbances (84). Another study by the same group showed that upon diagnosis, lung cancer patients had sleep disturbances including early morning awakenings, late sleep onset, prolonged nocturnal waking periods, daytime sleepiness, and unrefreshed sleep. These symptoms improved quickly in patients with a PDL-1 expression greater than 80% during the first 4 months of treatment due to the rapid response of the tumor to immunotherapy. There was no difference in symptom control seen between patients who received nivolumab or pembrolizumab (85).
Recent studies have demonstrated that ICIs can impact symptoms which cluster with sleep disruption such as cancer-related fatigue (CRF). These symptoms may present coincidentally as cancer-related symptoms clusters sharing common inflammatory, hormonal, autonomic nervous system, and hypothalamic-pituitary axis abnormalities. Hajjar and colleagues found that in 88 patients with advanced metastatic cancers undergoing immunotherapy, fatigue was identified in 66%. High level fatigue was found in 34%. This study is among the first to describe the microbiome in patients on immunotherapy which also has an important immune mediated impact, potentially affecting ICI effectiveness and irAEs. The study was able to show that there was a correlation of Eubacterium hallii that was negatively associated with fatigue severity scores whereas those patients with Cosenzaea sp. had higher fatigue scores (86). In a meta-analysis in subjects on ICI therapy, CTLA-4 inhibitors are associated with a higher risk of all and high-grade fatigue compared with control regimens, whereas PD-1 inhibitors are associated with a lower risk of all- and high-grade fatigue compared with control regimens. Although ICIs have revolutionized the treatment of certain cancers, many patients do not respond to ICIs and treatment outcomes vary disproportionately between cancer types (87). Therefore there is a significant interest how lifestyles, diet, and psychosocial factors including sleep quality determine the outcomes and ICI management (88). The impact of ICI therapy on sleep and related symptoms and the association with microbiome composition present opportunities to potentially augment the efficacy and tolerance of immunotherapy.
Circadian rhythm and immunotherapy
The human immune system is equipped to keep unnatural cell growth in check and aide in cancer suppression (69). Given the circadian clock’s influence on immune recognition and elimination of cancer cells, it is unsurprising that there is evolving evidence for the connection between circadian rhythms and cancer immunotherapy. In a metastatic melanoma murine model, BMAL1 is responsible for T-cell activation and the expression of CTLA-4, PD-1 and PD-L1 (89). Enrichment of clock gene pathways in animal studies increase PD-L1 expression and enhance T cell receptor signaling (90). In normal lung tissue, clock genes Per1 and Cry2 have been linked to the expression of CD4+ T cells and PD-1 expression follows a circadian rhythm (91). In clock gene RORγ knockout mice, the presence of PD-1 Type 17 cells and levels of PD-1 is decreased (92).
The circadian clock also affects the functional response of CD8 T-cells to antigen presentation by dendritic cells, the core aspect of the immune response against pathogens and cancer cells. This early T-cell receptor response was shown to impact T-cell receptor signaling such as activation, proliferation factor functions. The influence of circadian rhythms on the functional aspect of T-cell development, response to antigens, and trafficking is well recognized (69). Although there is no direct evidence that eloquently describes interplay between circadian rhythms and immunotherapy response, it is an exciting area of study and has the potential to transform immunotherapy (88).
Circadian rhythms are being considered for the prevention and management diseases including cancer (60). There are 3 broad approaches using our knowledge about circadian rhythms to impact cancer outcomes. First, lifestyle management, including sleep hygiene, diet composition and timing, and exercise timing, may be used to entrain a robust circadian rhythm. Consistent daily behavior patterns and sleep and eating may significantly reduce the risk of cancer (88). Studies in mice, utilizing rhythmic food intake have shown that these signals driven contribute to driving rhythms in liver gene expression and metabolic functions outweighing the influence of even the cell-autonomous hepatic clock (93). In addition, microbiome modification by lifestyle changes is an area of active study in patients on immunotherapy, as recently reviewed by Wargo and associates (94). Secondly, chronotherapy, or the timing of medication administration, can be used to target tumors while preventing adverse off-target effects. Nelson and Levi have shown that the time of day of infusion of both PD-1 and PD-L1 inhibitors showed clinically significant association with increased survival in patients which a variety of cancer types (95, 96). Finally, recent advancements on how to enhance our circadian clock through pharmacological targeting of circadian clock components that are already providing new preventive and therapeutic strategies for several diseases, including metabolic syndrome and cancer (97). A new class of drugs targeting circadian clock genes (“clock drugs”) act through targeting components of the clock circadian clock and have shown early promise in modulating immunotherapy (98). Clock drugs directly target the circadian clock components including, RORγ, REV–ERB’s (99). Furthermore, specific clock genes REV-ERBα and RORα deficient mice have negative effects on the development of and activation of dendritic cell and other antigen presenting cells critical to pathways involved with immune-mediated cancer cell targeting (82). In a series of experiments done by Hu and colleagues, RORγ agonists can act as monotherapy in vivo and display anti-tumor properties, including boosting the activity of TH17 cells. When treated with RORγ agonists, T-cells are more resistant to PD-L1 inhibition which is critical in suppressing anti-tumor activity. Supplementation of a RORγ agonist during ex-vivo expansion during chimeric antigen receptor (CAR)-T cell engineering, increased the antitumor activity of TH17 cells. The function of the CAR Type 17 cells is elevated when re-exposed to tumor with increase cytokine production including IL-17A and IFNγ. Moreover, mice with RORγt-primed T cells are protected after cancer cell inoculation (92, 100).
Circadian rhythms may also indicate treatment prognosis in immunotherapy with a recent study finding that in patients undergoing immunotherapy for lung adenocarcinoma, a circadian rhythm gene related signal could serve as an independent indicator for prognosis. This circadian rhythm genetic marker was found to be upregulated in cancer samples (101). These data taken together, suggest that circadian rhythms are likely to be harnessed in the future to augment the impact of immunotherapy in the treatment of cancer.
Future directions
As the role of ICIs expand, the impact of sleep, circadian rhythms, immunity, and immunotherapy requires further study. There are several unanswered and significant questions.
■ Are sleep and circadian rhythms a biomarker for prognosis in patients on immunotherapy as they have been shown in other therapies?
■ What are the interactions between sleep and circadian and other modifiable lifestyle factors such as diet?
■ Do these factors impact the microbiome and thereby affect treatment related outcomes with immunotherapy?
■ How will chronotherapy best be utilized to boost on target effects of immunotherapy?
■ Can we exploit the interconnection between the circadian clock genes and the immune system to drive more effective and safer immunotherapy approaches, minimizing auto-immunity?
It is the answers to these and other intriguing questions that lie the heart reaping of the promise of immunotherapy, that further exploration of these complex relationship hold.
Conclusion
Immunotherapy has transformed cancer treatment, and given its impact on the immune system, the role of ICI as it relates to sleep and circadian rhythm continue to unfold. Clearly an intricate complex relationship exists in the microenvironment with immunity, sleep and the tumor, and these may further vary based on the cancer, addition of standard chemotherapy, pre-existing patient factors and irAEs. Sleep and circadian rhythms may offer tools to better utilize immunotherapy in the care of cancer patients, leading to better treatment outcome, reduced symptom burden, and increased quality of life.
Author contributions
DB: Conceptualization, Formal analysis, Methodology, Project administration, Resources, Supervision, Validation, Visualization, Writing – original draft, Writing – review and editing. LB: Conceptualization, Writing – review and editing. AS: Conceptualization, Writing – review and editing. EM: Conceptualization, Writing – review and editing. SF: Conceptualization, Visualization, Writing – review and editing.
Funding
The author(s) declare financial support was received for the research, authorship, and/or publication of this article. This research is supported in part by the National Institutes of Health through MD Anderson’s Cancer Center Support Grant (CA016672).
Conflict of interest
The authors declare that the research was conducted in the absence of any commercial or financial relationships that could be construed as a potential conflict of interest.
Publisher’s note
All claims expressed in this article are solely those of the authors and do not necessarily represent those of their affiliated organizations, or those of the publisher, the editors and the reviewers. Any product that may be evaluated in this article, or claim that may be made by its manufacturer, is not guaranteed or endorsed by the publisher.
Abbreviations
ICI, immune checkpoint inhibitor; irEA, immune mediated adverse event; PD-1, programmed cell death-1; PD-L1, programmed cell death ligand-1; CTLA-4, cytotoxic T lymphocyte antigen-4; BMAL, brain and muscle ARNT–like protein 1; PER2, period 2; REV-Erbα, reverse erythroblastsosis virus alpha; RORα retinoic acid receptor–related orphan receptor alpha; Th1, T helper 1 cell; TNFα, tumor necrosis factor alpha; IL-1, interleukin-1; IFNγ, interferon gamma.
References
1. Palesh O, Peppone L, Innominato PF, Janelsins M, Jeong M, Sprod L, et al. Prevalence, putative mechanisms, and current management of sleep problems during chemotherapy for cancer. Nat Sci Sleep (2012) 4:151–62. doi: 10.2147/NSS.S18895
2. Yennurajalingam S, Balachandran D, Pedraza Cardozo SL, Berg EA, Chisholm GB, Reddy A, et al. Patient-reported sleep disturbance in advanced cancer: frequency, predictors and screening performance of the Edmonton Symptom Assessment System sleep item. BMJ Support Palliat Care (2017) 7(3):274–80. doi: 10.1136/bmjspcare-2015-000847
3. Buttner-Teleaga A, Kim YT, Osel T, Richter K. Sleep disorders in cancer-A systematic review. Int J Environ Res Public Health (2021) 18(21):1–38. doi: 10.3390/ijerph182111696
4. Dickerson SS, Connors LM, Fayad A, Dean GE. Sleep-wake disturbances in cancer patients: narrative review of literature focusing on improving quality of life outcomes. Nat Sci Sleep (2014) 6:85–100. doi: 10.2147/NSS.S34846
5. Divani A, Heidari ME, Ghavampour N, Parouhan A, Ahmadi S, Narimani Charan O, et al. Effect of cancer treatment on sleep quality in cancer patients: A systematic review and meta-analysis of Pittsburgh Sleep Quality Index. Supportive Care Cancer (2022) 30(6):4687–97. doi: 10.1007/s00520-021-06767-9
6. Ralli M, Campo F, Angeletti D, Allegra E, Minni A, Polimeni A, et al. Obstructive sleep apnoea in patients treated for head and neck cancer: A systematic review of the literature. Medicina (Kaunas) (2020) 56(8):1–9. doi: 10.3390/medicina56080399
7. Balachandran DD, Miller MA, Faiz SA, Yennurajalingam S, Innominato PF. Evaluation and management of sleep and circadian rhythm disturbance in cancer. Curr Treat Options Oncol (2021) 22(9):81. doi: 10.1007/s11864-021-00872-x
8. Olson K. Sleep-related disturbances among adolescents with cancer: a systematic review. Sleep Med (2014) 15(5):496–501. doi: 10.1016/j.sleep.2014.01.006
9. Charalambous A, Berger AM, Matthews E, Balachandran DD, Papastavrou E, Palesh O. Cancer-related fatigue and sleep deficiency in cancer care continuum: concepts, assessment, clusters, and management. Support Care Cancer (2019) 27(7):2747–53. doi: 10.1007/s00520-019-04746-9
10. Jensen LD, Oliva D, Andersson BA, Lewin F. A multidisciplinary perspective on the complex interactions between sleep, circadian, and metabolic disruption in cancer patients. Cancer Metastasis Rev (2021) 40(4):1055–71. doi: 10.1007/s10555-021-10010-6
11. Caspi RR. Immunotherapy of autoimmunity and cancer: the penalty for success. Nat Rev Immunol (2008) 8(12):970–6. doi: 10.1038/nri2438
12. Esfahani K, Roudaia L, Buhlaiga N, Del Rincon SV, Papneja N, Miller WH Jr. A review of cancer immunotherapy: from the past, to the present, to the future. Curr Oncol (2020) 27(Suppl 2):S87–97. doi: 10.3747/co.27.5223
13. Chhabra N, Kennedy J. A review of cancer immunotherapy toxicity: immune checkpoint inhibitors. J Med Toxicol (2021) 17(4):411–24. doi: 10.1007/s13181-021-00833-8
14. Miquelotti LB, Sari MHM, Ferreira LM. Immunotherapy in cancer management: A literature review of clinical efficacy of pembrolizumab in the non-small cell lung cancer treatment. Adv Pharm Bull (2023) 13(1):88–95. doi: 10.34172/apb.2023.007
15. Brunetti V, Della Marca G, Spagni G, Iorio R. Immunotherapy improves sleep and cognitive impairment in anti-IgLON5 encephalopathy. Neurol Neuroimmunol Neuroinflamm (2019) 6(4):e577. doi: 10.1212/NXI.0000000000000577
16. Vale TC, Fernandes do Prado LB, do Prado GF, Povoas Barsottini OG, Pedroso JL. Rapid eye movement sleep behavior disorder in paraneoplastic cerebellar degeneration: improvement with immunotherapy. Sleep (2016) 39(1):117–20. doi: 10.5665/sleep.5330
17. O’Cearbhaill RE, Clark L, Eskander RN, Gaillard S, Moroney J, Pereira E, et al. Immunotherapy toxicities: An SGO clinical practice statement. Gynecol Oncol (2022) 166(1):25–35. doi: 10.1016/j.ygyno.2022.05.003
18. Haanen J, Obeid M, Spain L, Carbonnel F, Wang Y, Robert C, et al. Management of toxicities from immunotherapy: ESMO Clinical Practice Guideline for diagnosis, treatment and follow-up. Ann Oncol (2022) 33(12):1217–38. doi: 10.1016/j.annonc.2022.10.001
19. Berry RB, Brooks R, Gamaldo C, Harding SM, Lloyd RM, Quan SF, et al. AASM scoring manual updates for 2017 (Version 2.4). J Clin Sleep Med (2017) 13(5):665–6. doi: 10.5664/jcsm.6576
20. Besedovsky L, Lange T, Haack M. The sleep-immune crosstalk in health and disease. Physiol Rev (2019) 99(3):1325–80. doi: 10.1152/physrev.00010.2018
21. Del Gallo F, Opp MR, Imeri L. The reciprocal link between sleep and immune responses. Arch Ital Biol (2014) 152(2-3):93–102. doi: 10.12871/000298292014234
22. Buysse DJ, Reynolds CF 3rd, Monk TH, Berman SR, Kupfer DJ. The Pittsburgh Sleep Quality Index: a new instrument for psychiatric practice and research. Psychiatry Res (1989) 28(2):193–213. doi: 10.1016/0165-1781(89)90047-4
23. Johns MW. A new method for measuring daytime sleepiness: the Epworth sleepiness scale. Sleep (1991) 14(6):540–5. doi: 10.1093/sleep/14.6.540
24. Bastien CH, Vallieres A, Morin CM. Validation of the Insomnia Severity Index as an outcome measure for insomnia research. Sleep Med (2001) 2(4):297–307. doi: 10.1016/S1389-9457(00)00065-4
25. Chung F, Yegneswaran B, Liao P, Chung SA, Vairavanathan S, Islam S, et al. STOP questionnaire: a tool to screen patients for obstructive sleep apnea. Anesthesiology (2008) 108(5):812–21. doi: 10.1097/ALN.0b013e31816d83e4
26. Ruehland WR, O’Donoghue FJ, Pierce RJ, Thornton AT, Singh P, Copland JM, et al. The 2007 AASM recommendations for EEG electrode placement in polysomnography: impact on sleep and cortical arousal scoring. Sleep (2011) 34(1):73–81. doi: 10.1093/sleep/34.1.73
27. Collop NA, Anderson WM, Boehlecke B, Claman D, Goldberg R, Gottlieb DJ, et al. Clinical guidelines for the use of unattended portable monitors in the diagnosis of obstructive sleep apnea in adult patients. Portable Monitoring Task Force of the American Academy of Sleep Medicine. J Clin Sleep Med (2007) 3(7):737–47.
28. Morgenthaler T, Alessi C, Friedman L, Owens J, Kapur V, Boehlecke B, et al. Practice parameters for the use of actigraphy in the assessment of sleep and sleep disorders: an update for 2007. Sleep (2007) 30(4):519–29. doi: 10.1093/sleep/30.4.519
29. Borbély AA, Daan S, Wirz-Justice A, Deboer T. The two-process model of sleep regulation: a reappraisal. J Sleep Res (2016) 25(2):131–43. doi: 10.1111/jsr.12371
30. Sulaman BA, Wang S, Tyan J, Eban-Rothschild A. Neuro-orchestration of sleep and wakefulness. Nat Neurosci (2023) 26(2):196–212. doi: 10.1038/s41593-022-01236-w
31. Innominato PF, Roche VP, Palesh OG, Ulusakarya A, Spiegel D, Levi FA. The circadian timing system in clinical oncology. Ann Med (2014) 46(4):191–207. doi: 10.3109/07853890.2014.916990
32. Haspel JA, Anafi R, Brown MK, Cermakian N, Depner C, Desplats P, et al. Perfect timing: circadian rhythms, sleep, and immunity - an NIH workshop summary. JCI Insight (2020) 5(1):1–4. doi: 10.1172/jci.insight.131487
33. Gozal D, Farre R, Nieto FJ. Obstructive sleep apnea and cancer: Epidemiologic links and theoretical biological constructs. Sleep Med Rev (2016) 27:43–55. doi: 10.1016/j.smrv.2015.05.006
34. Palesh OG, Roscoe JA, Mustian KM, Roth T, Savard J, Ancoli-Israel S, et al. Prevalence, demographics, and psychological associations of sleep disruption in patients with cancer: University of Rochester Cancer Center-Community Clinical Oncology Program. J Clin Oncol (2010) 28(2):292–8. doi: 10.1200/JCO.2009.22.5011
35. Innominato PF, Giacchetti S, Bjarnason GA, Focan C, Garufi C, Coudert B, et al. Prediction of overall survival through circadian rest-activity monitoring during chemotherapy for metastatic colorectal cancer. Int J Cancer (2012) 131(11):2684–92. doi: 10.1002/ijc.27574
36. Sillah A, Watson NF, Schwartz SM, Gozal D, Phipps AI. Sleep apnea and subsequent cancer incidence. Cancer causes control: CCC. (2018). doi: 10.1007/s10552-018-1073-5
37. Martinez-Garcia MA, Campos-Rodriguez F, Nagore E, Martorell A, Rodriguez-Peralto JL, Riveiro-Falkenbach E, et al. Sleep-Disordered Breathing is Independently Associated with Increased Aggressiveness of Cutaneous Melanoma. A multicentre observational study in 443 patients. Chest (2018) 154(6):1348–58. doi: 10.1016/j.chest.2018.07.015
38. Gozal D, Farre R, Nieto FJ. Putative links between sleep apnea and cancer: from hypotheses to evolving evidence. Chest (2015) 148(5):1140–7. doi: 10.1378/chest.15-0634
39. Patel SA, Kondratov RV. Clock at the core of cancer development. Biol (Basel) (2021) 10(2):1–16. doi: 10.3390/biology10020150
40. Kettner NM, Voicu H, Finegold MJ, Coarfa C, Sreekumar A, Putluri N, et al. Circadian homeostasis of liver metabolism suppresses hepatocarcinogenesis. Cancer Cell (2016) 30(6):909–24. doi: 10.1016/j.ccell.2016.10.007
41. Lin HH, Farkas ME. Altered circadian rhythms and breast cancer: from the human to the molecular level. Front Endocrinol (Lausanne) (2018) 9:219. doi: 10.3389/fendo.2018.00219
42. Villanueva MT. Cancer: Reset your circadian clock. Nat Rev Drug Discovery (2018) 17(3):166. doi: 10.1038/nrd.2018.24
43. Nirvani M, Khuu C, Utheim TP, Sand LP, Sehic A. Circadian clock and oral cancer. Mol Clin Oncol (2018) 8(2):219–26. doi: 10.3892/mco.2017.1518
44. Gu D, Li S, Ben S, Du M, Chu H, Zhang Z, et al. Circadian clock pathway genes associated with colorectal cancer risk and prognosis. Arch Toxicol (2018) 92(8):2681–9. doi: 10.1007/s00204-018-2251-7
45. Shostak A. Circadian clock, cell division, and cancer: from molecules to organism. Int J Mol Sci (2017) 18(4):1–15. doi: 10.3390/ijms18040873
46. Sun Y, Jin L, Sui YX, Han LL, Liu JH. Circadian gene CLOCK affects drug-resistant gene expression and cell proliferation in ovarian cancer SKOV3/DDP cell lines through autophagy. Cancer Biother Radiopharm (2017) 32(4):139–46. doi: 10.1089/cbr.2016.2153
47. Reszka E, Przybek M, Muurlink O, Peplonska B. Circadian gene variants and breast cancer. Cancer letters (2017) 390:137–45. doi: 10.1016/j.canlet.2017.01.012
48. Block KI. The circadian system and cancer: it’s about time! Integr Cancer Therapies (2018) 17(1):3–4. doi: 10.1177/1534735418755916
49. Robinson I, Reddy AB. Molecular mechanisms of the circadian clockwork in mammals. FEBS letters (2014) 588(15):2477–83. doi: 10.1016/j.febslet.2014.06.005
50. Lellupitiyage Don SS, Lin HH, Furtado JJ, Qraitem M, Taylor SR, Farkas ME. Circadian oscillations persist in low Malignancy breast cancer cells. Cell Cycle (2019) 18(19):2447–53. doi: 10.1080/15384101.2019.1648957
51. Taniguchi H, Fernández AF, Setién F, Ropero S, Ballestar E, Villanueva A, et al. Epigenetic inactivation of the circadian clock gene BMAL1 in hematologic Malignancies. Cancer Res (2009) 69(21):8447–54. doi: 10.1158/0008-5472.CAN-09-0551
52. Papagiannakopoulos T, Bauer MR, Davidson SM, Heimann M, Subbaraj L, Bhutkar A, et al. Circadian rhythm disruption promotes lung tumorigenesis. Cell Metab (2016) 24(2):324–31. doi: 10.1016/j.cmet.2016.07.001
53. Fritschi L, Glass DC, Heyworth JS, Aronson K, Girschik J, Boyle T, et al. Hypotheses for mechanisms linking shiftwork and cancer. Med Hypotheses (2011) 77(3):430–6. doi: 10.1016/j.mehy.2011.06.002
54. Fagundo-Rivera J, Allande-Cusso R, Ortega-Moreno M, Garcia-Iglesias JJ, Romero A, Ruiz-Frutos C, et al. Implications of lifestyle and occupational factors on the risk of breast cancer in shiftwork nurses. Healthcare (Basel) (2021) 9(6):1–18. doi: 10.3390/healthcare9060649
55. Innominato PF, Palesh O, Dhabhar FS, Levi F, Spiegel D. Regulation of circadian rhythms and hypothalamic-pituitary-adrenal axis: an overlooked interaction in cancer. Lancet Oncol (2010) 11(9):816–7. doi: 10.1016/S1470-2045(10)70171-6
56. de Rooij BH, Ramsey I, Clouth FJ, Corsini N, Heyworth JS, Lynch BM, et al. The association of circadian parameters and the clustering of fatigue, depression, and sleep problems in breast cancer survivors: a latent class analysis. J Cancer Surviv (2022). doi: 10.1007/s11764-022-01189-w
57. Innominato PF, Mormont MC, Rich TA, Waterhouse J, Levi FA, Bjarnason GA. Circadian disruption, fatigue, and anorexia clustering in advanced cancer patients: implications for innovative therapeutic approaches. Integr Cancer Therapies (2009) 8(4):361–70. doi: 10.1177/1534735409355293
58. Berger AM, Wielgus K, Hertzog M, Fischer P, Farr L. Patterns of circadian activity rhythms and their relationships with fatigue and anxiety/depression in women treated with breast cancer adjuvant chemotherapy. Supportive Care Cancer (2010) 18(1):105–14. doi: 10.1007/s00520-009-0636-0
59. Ancoli-Israel S, Liu L, Rissling M, Natarajan L, Neikrug AB, Palmer BW, et al. Sleep, fatigue, depression, and circadian activity rhythms in women with breast cancer before and after treatment: a 1-year longitudinal study. Supportive Care Cancer (2014) 22(9):2535–45. doi: 10.1007/s00520-014-2204-5
60. Truong KK, Lam MT, Grandner MA, Sassoon CS, Malhotra A. Timing matters: circadian rhythm in sepsis, obstructive lung disease, obstructive sleep apnea, and cancer. Ann Am Thorac Society (2016) 13(7):1144–54. doi: 10.1513/AnnalsATS.201602-125FR
61. Palesh O, Aldridge-Gerry A, Zeitzer JM, Koopman C, Neri E, Giese-Davis J, et al. Actigraphy-measured sleep disruption as a predictor of survival among women with advanced breast cancer. Sleep (2014) 37(5):837–42. doi: 10.5665/sleep.3642
62. Amiama-Roig A, Verdugo-Sivianes EM, Carnero A, Blanco JR. Chronotherapy: circadian rhythms and their influence in cancer therapy. Cancers (Basel) (2022) 14(20):1–21. doi: 10.3390/cancers14205071
63. Levi F, Okyar A, Dulong S, Innominato PF, Clairambault J. Circadian timing in cancer treatments. Annu Rev Pharmacol Toxicol (2010) 50:377–421. doi: 10.1146/annurev.pharmtox.48.113006.094626
64. Xu Y, Su S, Li X, Mansuri A, McCall WV, Wang X. Blunted rest-activity circadian rhythm increases the risk of all-cause, cardiovascular disease and cancer mortality in US adults. Sci Rep (2022) 12(1):20665. doi: 10.1038/s41598-022-24894-z
65. Medysky ME, Temesi J, Culos-Reed SN, Millet GY. Exercise, sleep and cancer-related fatigue: Are they related? Neurophysiologie clinique = Clin Neurophysiol (2017) 47(2):111–22. doi: 10.1016/j.neucli.2017.03.001
66. Innominato PF, Komarzynski S, Palesh OG, Dallmann R, Bjarnason GA, Giacchetti S, et al. Circadian rest-activity rhythm as an objective biomarker of patient-reported outcomes in patients with advanced cancer. Cancer Med (2018) 7(9):4396–405. doi: 10.1002/cam4.1711
67. Sephton SE, Lush E, Dedert EA, Floyd AR, Rebholz WN, Dhabhar FS, et al. Diurnal cortisol rhythm as a predictor of lung cancer survival. Brain Behavior Immunity (2013) 30 Suppl:S163–70. doi: 10.1016/j.bbi.2012.07.019
68. Mormont MC, Waterhouse J, Bleuzen P, Giacchetti S, Jami A, Bogdan A, et al. Marked 24-h rest/activity rhythms are associated with better quality of life, better response, and longer survival in patients with metastatic colorectal cancer and good performance status. Clin Cancer Res (2000) 6(8):3038–45.
69. Zhang Z, Zeng P, Gao W, Zhou Q, Feng T, Tian X. Circadian clock: a regulator of the immunity in cancer. Cell Commun Signal (2021) 19(1):37. doi: 10.1186/s12964-021-00721-2
70. Irwin MR, Opp MR. Sleep health: reciprocal regulation of sleep and innate immunity. Neuropsychopharmacology (2017) 42(1):129–55. doi: 10.1038/npp.2016.148
71. Opp MR. Sleep and psychoneuroimmunology. Immunol Allergy Clinics North America (2009) 29(2):295–307. doi: 10.1016/j.iac.2009.02.009
72. Opp MR, Imeri L. Sleep as a behavioral model of neuro-immune interactions. Acta Neurobiol Exp (Wars) (1999) 59(1):45–53.
73. Han SH, Lee SY, Cho JW, Kim JH, Moon HJ, Park HR, et al. Sleep and circadian rhythm in relation to COVID-19 and COVID-19 vaccination-national sleep survey of South Korea 2022. J Clin Med (2023) 12(4):1–10. doi: 10.3390/jcm12041518
74. Anderson MS, Chinoy ED, Harrison EM, Myers CA, Markwald RR. Sleep, immune function, and vaccinations in military personnel: challenges and future directions. Military Med (2023). doi: 10.1093/milmed/usad119
75. Opp MR. Sleep: Not getting enough diminishes vaccine responses. Curr biology: CB. (2023) 33(5):R192–R4. doi: 10.1016/j.cub.2023.02.003
76. Besedovsky L, Lange T, Born J. Sleep and immune function. Pflugers Archiv: Eur J Physiol (2012) 463(1):121–37. doi: 10.1007/s00424-011-1044-0
77. Rayatdoost E, Rahmanian M, Sanie MS, Rahmanian J, Matin S, Kalani N, et al. Sufficient sleep, time of vaccination, and vaccine efficacy: A systematic review of the current evidence and a proposal for COVID-19 vaccination. Yale J Biol Med (2022) 95(2):221–35.
78. Scheiermann C, Gibbs J, Ince L, Loudon A. Clocking in to immunity. Nat Rev Immunol (2018) 18(7):423–37. doi: 10.1038/s41577-018-0008-4
79. Silver AC, Arjona A, Walker WE, Fikrig E. The circadian clock controls toll-like receptor 9-mediated innate and adaptive immunity. Immunity (2012) 36(2):251–61. doi: 10.1016/j.immuni.2011.12.017
80. Alexander RK, Liou YH, Knudsen NH, Starost KA, Xu C, Hyde AL, et al. Bmal1 integrates mitochondrial metabolism and macrophage activation. Elife (2020) 9:1–28. doi: 10.7554/eLife.54090
81. Druzd D, Matveeva O, Ince L, Harrison U, He W, Schmal C, et al. Lymphocyte circadian clocks control lymph node trafficking and adaptive immune responses. Immunity (2017) 46(1):120–32. doi: 10.1016/j.immuni.2016.12.011
82. Amir M, Campbell S, Kamenecka TM, Solt LA. Pharmacological modulation and genetic deletion of REV-ERBalpha and REV-ERBbeta regulates dendritic cell development. Biochem Biophys Res Commun (2020) 527(4):1000–7. doi: 10.1016/j.bbrc.2020.05.012
83. Besedovsky L, Born J, Lange T. Blockade of mineralocorticoid receptors enhances naive T-helper cell counts during early sleep in humans. Brain Behavior Immunity (2012) 26(7):1116–21. doi: 10.1016/j.bbi.2012.07.016
84. Zarogoulidis P, Petridis D, Kosmidis C, Sapalidis K, Nena L, Matthaios D, et al. Immunotherapy and chemotherapy versus sleep disturbances for NSCLC patients. Curr Oncol (2023) 30(2):1999–2006. doi: 10.3390/curroncol30020155
85. Zarogoulidis P, Petridis D, Kosmidis C, Sapalidis K, Nena L, Matthaios D, et al. Non-small-cell lung cancer immunotherapy and sleep characteristics: the crossroad for optimal survival. Diseases (2023) 11(1):1–8. doi: 10.3390/diseases11010026
86. Hajjar J, Mendoza T, Zhang L, Fu S, Piha-Paul SA, Hong DS, et al. Associations between the gut microbiome and fatigue in cancer patients. Sci Rep (2021) 11(1):5847. doi: 10.1038/s41598-021-84783-9
87. Abdel-Rahman O, Helbling D, Schmidt J, Petrausch U, Giryes A, Mehrabi A, et al. Treatment-associated fatigue in cancer patients treated with immune checkpoint inhibitors; a systematic review and meta-analysis. Clin Oncol (R Coll Radiol) (2016) 28(10):e127–38. doi: 10.1016/j.clon.2016.06.008
88. Deshpande RP, Sharma S, Watabe K. The confounders of cancer immunotherapy: roles of lifestyle, metabolic disorders and sociological factors. Cancers (Basel) (2020) 12(10):1–28. doi: 10.3390/cancers12102983
89. de Assis LVM, Kinker GS, Moraes MN, Markus RP, Fernandes PA, Castrucci AML. Expression of the circadian clock gene BMAL1 positively correlates with antitumor immunity and patient survival in metastatic melanoma. Front Oncol (2018) 8:185. doi: 10.3389/fonc.2018.00185
90. Wu Y, Tao B, Zhang T, Fan Y, Mao R. Pan-cancer analysis reveals disrupted circadian clock associates with T cell exhaustion. Front Immunol (2019) 10:2451. doi: 10.3389/fimmu.2019.02451
91. Yang Y, Yuan G, Xie H, Wei T, Zhu D, Cui J, et al. Circadian clock associates with tumor microenvironment in thoracic cancers. Aging (Albany NY) (2019) 11(24):11814–28. doi: 10.18632/aging.102450
92. Hu X, Liu X, Moisan J, Wang Y, Lesch CA, Spooner C, et al. Synthetic RORgamma agonists regulate multiple pathways to enhance antitumor immunity. Oncoimmunology (2016) 5(12):e1254854. doi: 10.1080/2162402X.2016.1254854
93. Greenwell BJ, Trott AJ, Beytebiere JR, Pao S, Bosley A, Beach E, et al. Rhythmic food intake drives rhythmic gene expression more potently than the hepatic circadian clock in mice. Cell Rep (2019) 27(3):649–57.e5. doi: 10.1016/j.celrep.2019.03.064
94. Helmink BA, Khan MAW, Hermann A, Gopalakrishnan V, Wargo JA. The microbiome, cancer, and cancer therapy. Nat Med (2019) 25(3):377–88. doi: 10.1038/s41591-019-0377-7
95. Qian DC, Kleber T, Brammer B, Xu KM, Switchenko JM, Janopaul-Naylor JR, et al. Effect of immunotherapy time-of-day infusion on overall survival among patients with advanced melanoma in the USA (MEMOIR): a propensity score-matched analysis of a single-centre, longitudinal study. Lancet Oncol (2021) 22(12):1777–86. doi: 10.1016/S1470-2045(21)00546-5
96. Innominato PF, Karaboue A, Bouchahda M, Bjarnason GA, Levi FA. The future of precise cancer chronotherapeutics. Lancet Oncol (2022) 23(6):e242. doi: 10.1016/S1470-2045(22)00188-7
97. Sulli G, Manoogian ENC, Taub PR, Panda S. Training the circadian clock, clocking the drugs, and drugging the clock to prevent, manage, and treat chronic diseases. Trends Pharmacol Sci (2018) 39(9):812–27. doi: 10.1016/j.tips.2018.07.003
98. Sulli G, Lam MTY, Panda S. Interplay between circadian clock and cancer: new frontiers for cancer treatment. Trends Cancer (2019) 5(8):475–94. doi: 10.1016/j.trecan.2019.07.002
99. Sulli G, Rommel A, Wang X, Kolar MJ, Puca F, Saghatelian A, et al. Pharmacological activation of REV-ERBs is lethal in cancer and oncogene-induced senescence. Nature (2018) 553(7688):351–5. doi: 10.1038/nature25170
100. Hu X, Majchrzak K, Liu X, Wyatt MM, Spooner CJ, Moisan J, et al. In vitro priming of adoptively transferred T cells with a RORgamma agonist confers durable memory and stemness in vivo. Cancer Res (2018) 78(14):3888–98. doi: 10.1158/0008-5472.CAN-17-3973
Keywords: immunotherapy, sleep, circadian rhythm, immune checkpoint inhibitors, immunity
Citation: Balachandran DD, Bashoura L, Sheshadri A, Manzullo E and Faiz SA (2023) The Impact of Immunotherapy on Sleep and Circadian Rhythms in Patients with Cancer. Front. Oncol. 13:1295267. doi: 10.3389/fonc.2023.1295267
Received: 15 September 2023; Accepted: 06 November 2023;
Published: 27 November 2023.
Edited by:
Elizabeth Cash, University of Louisville, United StatesReviewed by:
Patrick Schwartz, University of Wisconsin-Madison, United StatesCopyright © 2023 Balachandran, Bashoura, Sheshadri, Manzullo and Faiz. This is an open-access article distributed under the terms of the Creative Commons Attribution License (CC BY). The use, distribution or reproduction in other forums is permitted, provided the original author(s) and the copyright owner(s) are credited and that the original publication in this journal is cited, in accordance with accepted academic practice. No use, distribution or reproduction is permitted which does not comply with these terms.
*Correspondence: Diwakar D. Balachandran, DBalachandran@mdanderson.org