- 1Department of Radiology and Nuclear Medicine, University Medical Centre Mannheim, Medical Faculty Mannheim, University of Heidelberg, Mannheim, Germany
- 2Department of Biomedical Imaging and Image-Guided Therapy, Vienna General Hospital, Medical University of Vienna, Vienna, Austria
Background: Economic evaluations have become an accepted methodology for decision makers to allocate resources in healthcare systems. Particularly in screening, where short-term costs are associated with long-term benefits, and adverse effects of screening intermingle, cost-effectiveness analyses provide a means to estimate the economic value of screening.
Purpose: To introduce the methodology of economic evaluations and to review the existing evidence on cost-effectiveness of MR-based breast cancer screening.
Materials and methods: The various concepts and techniques of economic evaluations critical to the interpretation of cost-effectiveness analyses are briefly introduced. In a systematic review of the literature, economic evaluations from the years 2000-2022 are reviewed.
Results: Despite a considerable heterogeneity in the reported input variables, outcome categories and methodological approaches, cost-effectiveness analyses report favorably on the economic value of breast MRI screening for different risk groups, including both short- and long-term costs and outcomes.
Conclusion: Economic evaluations indicate a strongly favorable economic value of breast MRI screening for women at high risk and for women with dense breast tissue.
Introduction
Breast cancer is the most common cancer and the leading cause of cancer-related death in women worldwide with an estimated 2.3 million incident cases and 685,000 deaths in 2020, despite significant advances in therapeutic options and widespread screening programs (1, 2). Diagnosed at an early stage, localized breast cancer, much like colorectal cancer, is associated with excellent 5-year survival rates of approximately 99% (3). Due to the lack of symptoms in an early stage, screening for breast cancer is particularly promising and relevant.
For conventional screening programs, reductions in breast cancer mortality have been demonstrated (4–6), even though the positive results have been a matter of scientific discussion: some authors critically remark the high number of false positive cases (7, 8) and the imperfect sensitivity of mammography. Other authors derive benefits in survival predominantly from advances in breast cancer therapy and an effect of overdiagnosis (9). On top, the risk of radiation-induced cancers must be considered (10).
Among the various modalities applied in breast imaging, breast MRI is accepted to have the highest sensitivity in detecting breast cancer independent from breast density (11). Concerns on specificity and high costs, among other reasons, have averted breast MRI from taking a prominent role in screening.
The most recent multi-center studies have demonstrated that breast MRI does not suffer from reduced specificity compared to conventional mammography (12–14). However, reader experience, quality assurance and continuous monitoring are considered prerequisites for optimizing the diagnostic performance of breast MRI.
While evidence on the superior diagnostic performance of breast MRI in screening women at high risk has been available for several years (15–17), prospective multi-centric data for women with dense breasts have become available only recently and have confirmed superior sensitivity of 95.2% - 95.7% and reduced interval cancer rates of MRI-based screening compared to conventional approaches (18–20). Specificity increased in subsequent screening rounds (incidence rounds) as compared to the first screening round (prevalence round). In general, MRI-detected cancers were smaller than tumors detected by conventional mammography (21), and biologically aggressive cancers are more likely to be detected by MRI (22).
Besides requirements of efficacy, safety, and acceptance of screening, costs and potential benefits of screening programs need to be economically balanced (23). Innovative screening programs and expensive diagnostic tests are required to not only provide superior efficacy but also favorable economic effects (24). As a consequence, both short- and long-term costs and outcomes of screening are increasingly assessed by economic evaluations in order to capture their economic potential and to direct healthcare resource allocation accordingly. Cost-effectiveness analyses have evolved as an established framework for estimating economic value of innovative screening measures based on economic modeling and represent a prerequisite to establish funding by health insurance funds in various healthcare systems (25).
There are various methodological approaches with different outcome categories reported, hampering comparability of the findings and misleading economically inexperienced readers (26, 27).
However, for the various diagnostic modalities in breast imaging, each with different diagnostic potential and financial burden, cost-effectiveness analyses are particularly valuable and may help identify the most efficient medical care for each risk group.
Firstly, we introduce various methodologies of economic evaluations, explain the different approaches of outcome measurement and aim at developing a conceptual understanding of economic evaluations. Secondly, in the systematic review of the literature, the latest available evidence on cost-effectiveness of MRI-based breast cancer screening is discussed and evaluated.
A brief guide to economic evaluations
In health economics, evaluations are conducted to systematically compare different diagnostic or therapeutic strategies, e.g. the standard of care versus an innovative technique. Not only the costs of medical interventions can be considered but a certain “value” can be assigned to the outcomes. Capturing the value of diagnostic radiology can be challenging since diagnostic techniques only indirectly affect health care outcomes (28).
Empirical studies on the economic value of long-term patient journeys and health services administration are often not feasible due to associated costs and time constraints, and controlled experiments may be difficult to implement due to ethical and medical concerns. To overcome these limitations, the contemporary methodology is based on economic modeling and theoretical decision analysis that are applied to simulate the alternating diagnostic or therapeutic pathways, including all relevant medical costs and associated outcomes (24).
Measurement of costs
Various perspectives can be assumed to estimate costs, e.g. the perspective of the healthcare system, society or healthcare provider (29). Depending on the perspective, different costs have to be considered, e.g. direct medical costs including costs of treatment and personnel, indirect medical costs including transportation costs and intangible costs including non-monetary factors such as quality of life. For example, absence from work due to disease may result in productivity losses on the level of the economy that can be expressed in monetary terms.
Measurement of outcomes
There are various outcome categories applicable in economic modeling: Outcomes can be measured in monetary terms, in natural units such as mmHg blood pressure reduction, or life years gained. However, the heterogeneity of outcomes intrinsically limits comparability and transferability of the consecutive results.
Considering changes in life expectancy (life years gained) allows comparisons across various conditions. However, differences in quality of life (QoL) are neglected, e.g. due to side effects of therapies. Therefore, quality-adjusted life years (QALYs) have evolved as a reference standard as well as generic means of outcome measurement. QALYs include both the quantity as well as quality of life time, obtainable by multiplying life time with quality of life (29). This way, generic outcomes can be compared between different diseases and therapies. Hence, QALYs have become the gold standard in measuring health care outcomes in cost-effectiveness- or cost-utility analyses.
When estimating outcomes in cost-effectiveness modeling, a practical concern is the availability of input variables to construct valid economic models. Data on quality of life are still scarce for many conditions, and the methodological variability of valuing utilities may limit their validity (30). It may be difficult to quantify the quality of life of any health state. Literature on quality of life is growing, and an increasing number of prospective study designs include QoL-measurements as well.
Types of economic evaluations
There are various types of economic evaluations (29): In cost-benefit analyses, outcomes are expressed in monetary terms. Cost-effectiveness analyses try to relate costs to natural outcomes such as reduction of cholesterol levels or life years gained. Cost-utility analyses use quality-adjusted life years as generic outcomes and are considered a reference standard as they enable comparisons across conditions based on a common denominator, i.e. QALYs. In the literature, the terms cost-effectiveness analysis and cost-utility analysis are often used interchangeably.
Decision analysis and Markov-Models
For an economic evaluation based on modeling, a decision tree is necessary including the therapeutic or diagnostic strategies and representing all possible outcomes. Each branch of the decision tree is assigned a predefined probability.
For each branch of the decision tree, a Markov-Model is used as a state-transition model to simulate costs and effects over a predefined time horizon (31). The Markov states are mutually exclusive and collectively exhaustive which means they represent all possible and necessary disease states. Patients may freely transition from one Markov state to another after each cycle, as they receive treatments or experience changes in health states. A Markov model is “memoryless”, which means that the transition probabilities assigned to each Markov state do not depend on the history of prior Markov states but only on the current disease state. A fixed cycle length is chosen depending on the modeled disease entity. Associated costs and outcomes are assigned to each Markov state.
Economic modeling of breast cancer screening incorporates not only the costs and outcomes of screening tests and follow-ups, but also stage-dependent costs of therapeutic pathways and consecutive reductions in quality of life. True positive and true negative results are included as well as false negative and false positive findings.
An exemplary decision tree and Markov model to simulate breast cancer screening is depicted in Figure 1. All possible diagnostic outcomes are included: True positive, false negative, true negative and false positive findings. The set of Markov states needs to be differentiated enough to represent the variety of all possible disease states. However, it also needs to be simple enough to be based on valid estimates of input variables, in order to prevent from getting lost in assumptions on subgroups and pre-conditions. From a practical point of view, identification of valid point estimates for the input variables is crucial and depends on the quality of the underlying evidence.
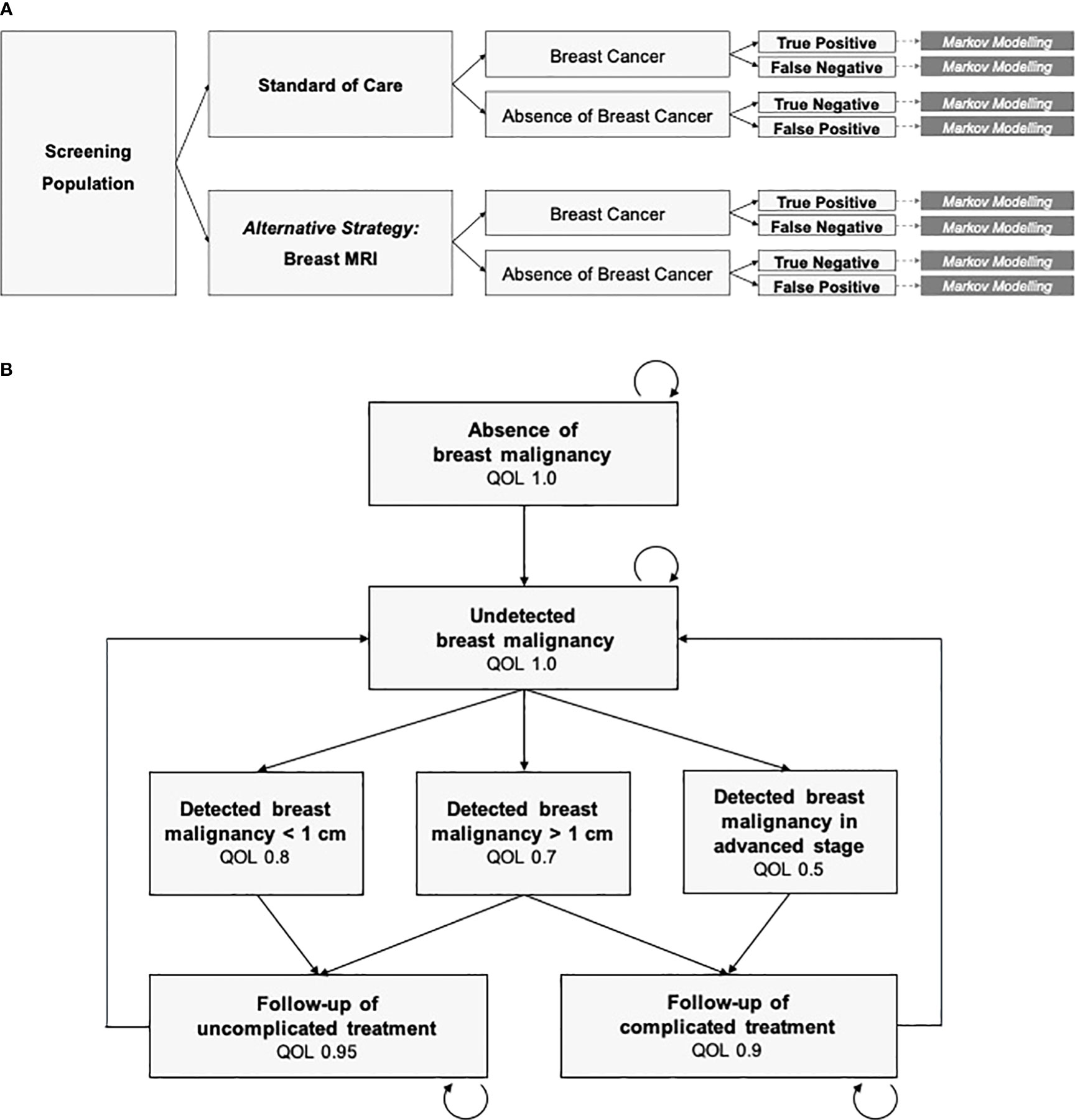
Figure 1 Decision analysis and economic modeling. (A) Decision tree including the diagnostic strategies (standard of care vs. breast MRI), ground truth (breast cancer vs. absence of breast cancer) and the diagnostic outcomes. Markov Modeling is conducted for each branch of the decision tree. (B) The Markov Model is defined by the mutually exclusive and collectively exhaustive Markov states, cycle length, transition probabilities, and the costs and quality of life (QOL) assigned to each state. Mortality is included in any state.
Microsimulation models
While Markov models offer a means to simulate cohorts of patients based on cohort averages, and the simulation is memoryless by its classical definition (“Markov assumption”), microsimulation models represent an alternative technique.
Microsimulation is used to model individual patients’ histories that are characterized by predefined variables and sets of rules (32), which is computationally more demanding. Due to their higher complexity, microsimulation models usually require a more thorough design, detailed and epidemiological input data as well as model validation. For instance, Microsimulation Screening Analysis (MISCAN) models have been designed to examine various cancer entities (33).
Incremental cost-effectiveness ratios and willingness to pay
When comparing alternative health care strategies, e.g. an innovative technique versus the established standard of care, additional costs per certain outcome are calculated and expressed as the ICER:
The ICER as a measure of cost-effectiveness can be used by decision makers to direct resource allocation in healthcare systems. The adoption of a new medical procedure is favored when the ICER falls below the WTP-threshold. There is a substantial global heterogeneity in the value of health and the resulting WTP for medical services. Thresholds differ between countries, healthcare systems and individual contexts, and depend on various factors such as reimbursement schemes, availability of services and resources, individual preferences and cultural factors. For instance, developing countries may not be comparable to developed countries, and there is substantial heterogeneity even within Western industrialized countries.
In the United States, a WTP-threshold between US-dollar (USD) 50,000 and USD 200,000 per QALY gained has been discussed, whereas a threshold of £ 20,000 - 30,000 has been adopted for the United Kingdom (34, 35). The world health organization has proposed to use the gross domestic product (GDP) per capita as a threshold that indicates high cost-effectiveness and 3 x the GDP indicating cost-effectiveness (36).
In a cost-effectiveness plane, incremental costs and effects of various strategies are displayed. In case a strategy achieves superior outcomes at reduced costs, the strategy is preferred (dominant strategy). In case a strategy achieves superior outcomes, but is associated with increased costs, the ICER is reflected by the slope of the line (Figure 2).
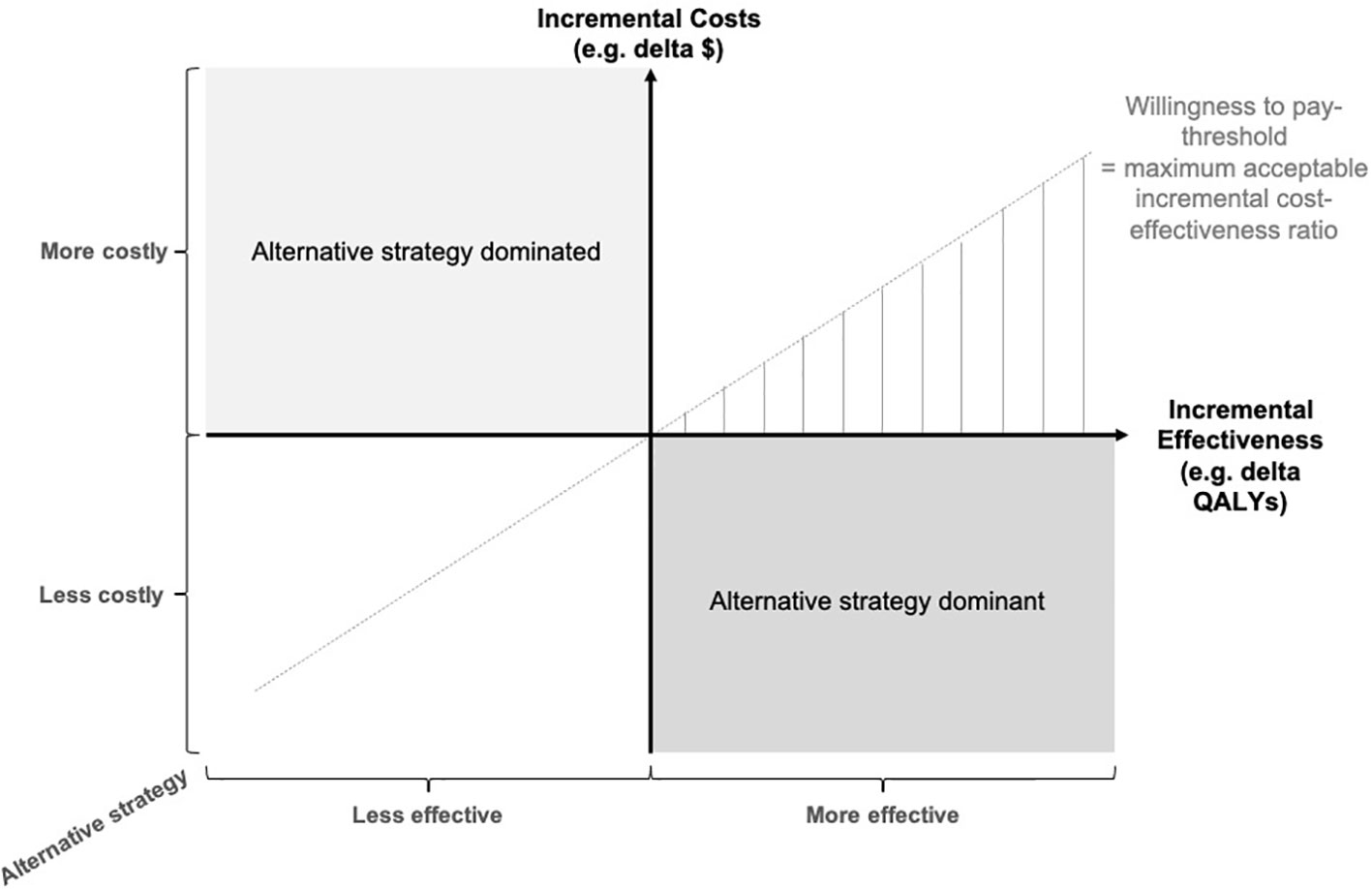
Figure 2 Cost-effectiveness plane. The incremental costs (e.g. in $) and incremental effects (e.g. in quality-adjusted life years, QALYs) of the alternative strategy, e.g. breast MRI, are computed to locate the strategy on the cost-effectiveness plane. In case it is more costly and less effective than the standard of care, the alternative strategy is dominated. In case of smaller costs and additional effectiveness, the alternative strategy is dominant. In the case of more effectiveness but more costs, the incremental cost-effectiveness ratio (ICER) has to be smaller than the willingness to pay (WTP) - threshold to be economically preferable.
Sensitivity analyses
Sensitivity analyses are conducted in order to address variability of the input parameters and uncertainty in the model design, and to estimate robustness of model outcomes (37). Input variables are point estimates and often represent the population average. In a deterministic sensitivity analysis, an input variable is varied within a predefined range and the model outcomes are computed. For instance, a range of costs per breast MRI has been reported for different health care systems and providers, and depending on reader experience, sensitivity and specificity of breast MRI may vary. These uncertainties can be addressed by simulating outcomes for a range of possible input values.
However, most variables can not only be expressed by a population average, but follow a probability distribution in the respective population. Therefore, a Monte Carlo simulation is conducted by randomly assuming values from the probability distribution of every variable in the model simultaneously. In a probabilistic sensitivity analysis, the resulting cost-effectiveness is simulated for a significant number of iterations, e.g. 30,000 iterations.
Quality assurance and checklists
In order to maintain a high standard of quality, extensive recommendations on the methodological conduct of cost-effectiveness analyses have been defined (38). Checklists are available to evaluate adherence to these recommendations. For instance, the Consolidated Health Economic Evaluation Reporting Standards (CHEERS) Statement (39) is widely applied to ensure appropriate reporting (Supplementary Table S1).
Materials and methods: systematic review
In a systematic review of economic evaluations on breast MRI screening, the PubMed database was scanned for literature between January 1, 2000 and November 25th, 2022 (Figure 3). Key-words included “breast MR*”, “breast cancer screening”, “MR-mammography”, “magnetic resonance imaging screening”, and “cost effective”, “cost-benefit”, “economic evaluation”, “cost-utility”, or “cost”. Economic evaluations including cost-effectiveness, cost-utility and cost-benefit analyses on breast cancer screening that applied screening MRI were included into analysis.
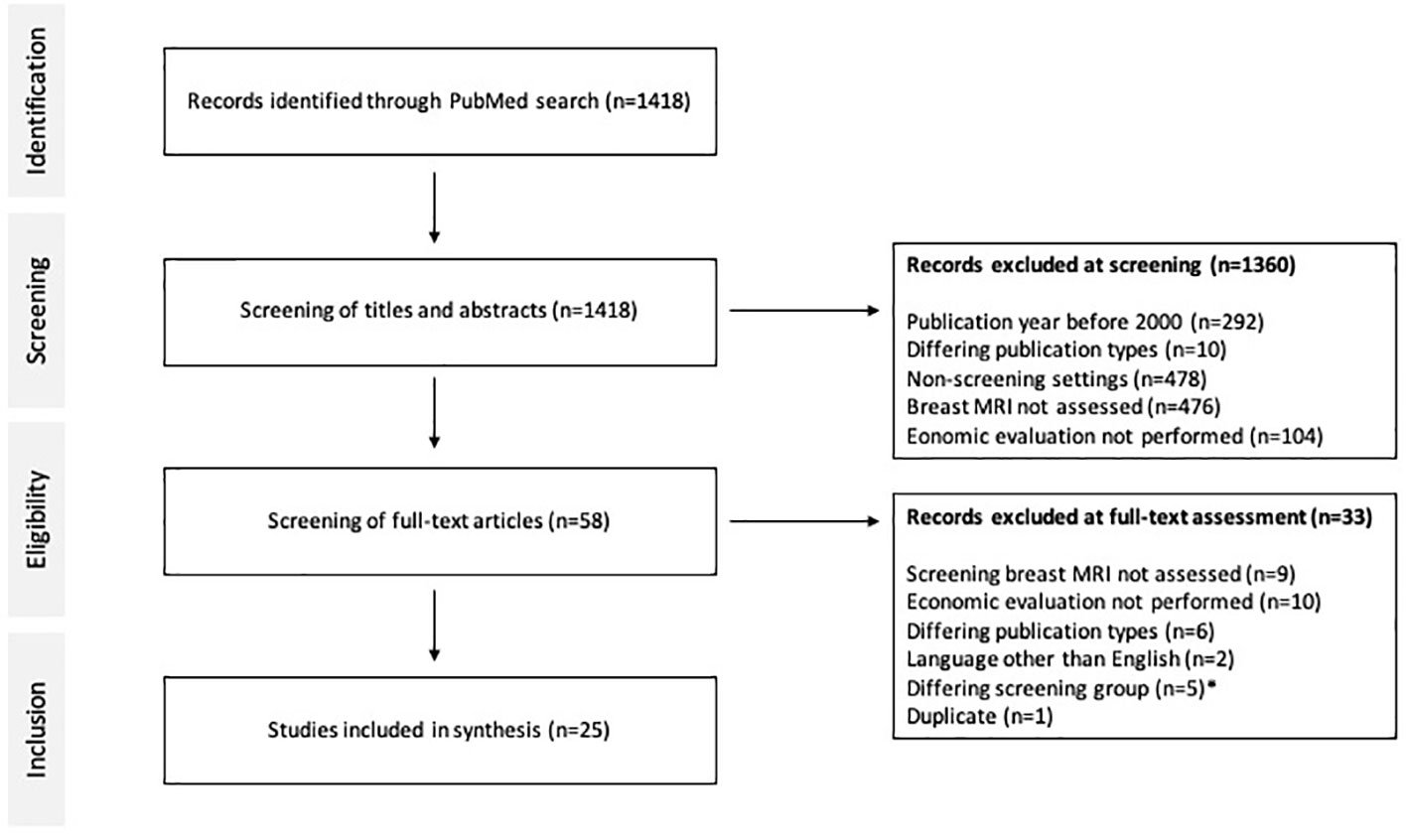
Figure 3 PRISMA diagram and literature search. Economic evaluations between 2000 and 2022 on breast MRI in screening of breast cancer were included. * Exceptional patient subgroups such as dialysis patients and childhood survivors of cancer or lymphoma were excluded.
Results
In total, 1418 studies were identified on PubMed, 1360 were excluded during screening of abstracts and 33 were excluded during full-text analysis (Figure 3). Finally, 25 articles on economics of breast MRI screening of different risk groups were included into further analysis. The CHEERS checklist was used to assess the quality of the included studies (Supplementary Table S2). Various indications of MRI-based screening have been established in the past years (Table 1).
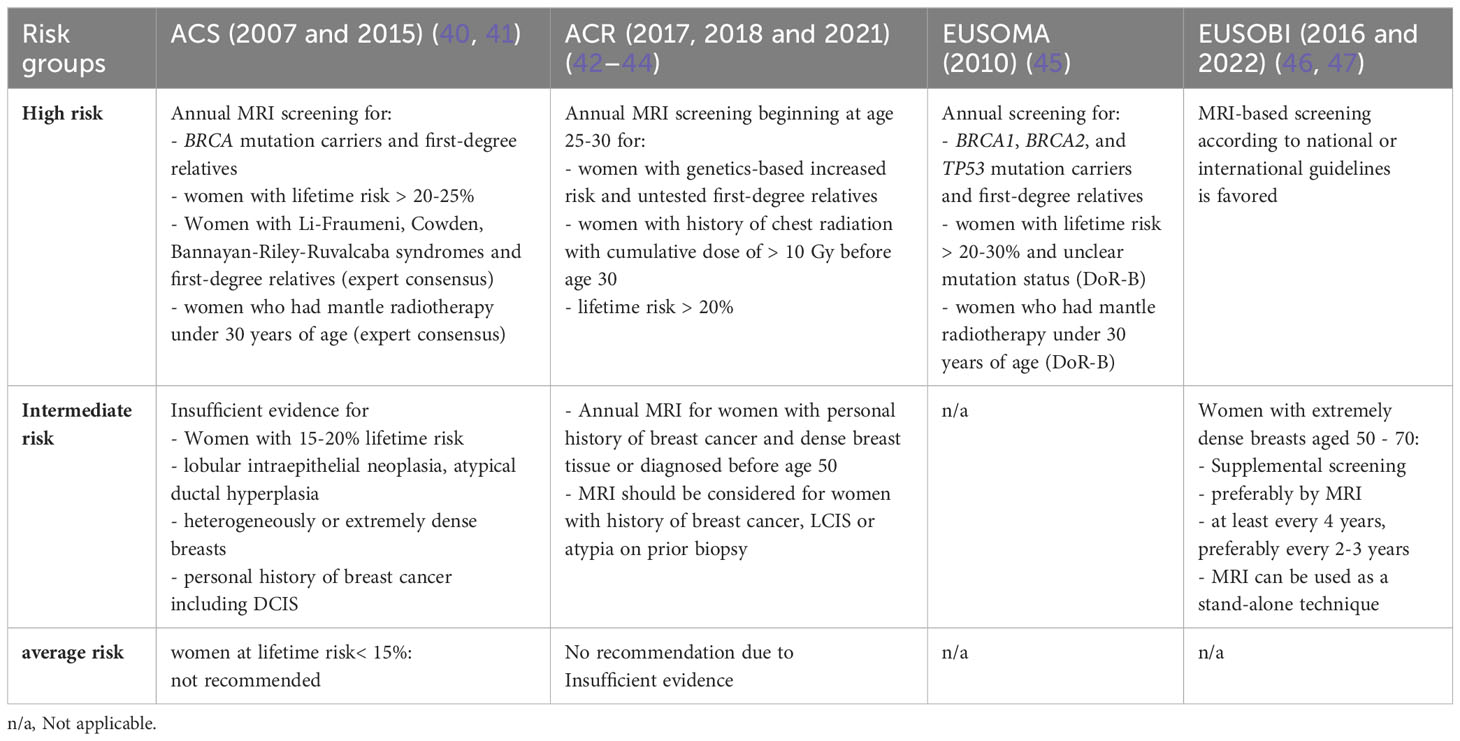
Table 1 International recommendations for breast MRI in breast cancer screening stratified for risk groups.
Cost-effectiveness of breast MRI in high-risk screening
The superior diagnostic performance of breast MRI in women at high risk of breast cancer has repeatedly been demonstrated over the past three decades. The most recent prospective multi-center trials confirm a superior sensitivity of 90 - 93% and a specificity of 89 - 98% in women at high risk of breast cancer, whereas mammography achieved a sensitivity of 33 - 50% and a specificity of 97 - 99% (15–17).
Based on the broad evidence available for several years, a number of economic evaluations have assessed the cost-effectiveness of breast MRI in the high-risk group (Table 2) (42–44, 48–60). The associated cost per breast MRI examination decreased over the previous years. For instance, in 2006 Plevritis et al. assumed a cost of USD 1,038, whereas contemporary analyses assume costs of USD 314 (48). They demonstrated that screening with mammography and MRI could be cost-effective especially for middle-aged breast cancer gene 1 (BRCA 1) mutation carriers vs. BRCA 2 mutation carriers with dense breast tissue at an ICER of USD 55,420 vs. USD 98,454 per QALY, respectively (48). In Canadian women with mutations in the BRCA 1 or 2 gene, alternating screening with conventional mammography and breast MRI every six months compared to annual mammography alone was cost-effective with an ICER of Canadian dollars (CAD) 50,900 per QALY gained (54).
Addressing the impact of specificity on cost-effectiveness of high-risk screening, Kaiser et al. have simulated the ICER for varying levels of specificity in women with high risk of breast cancer based on annual screening intervals (60). Compared to conventional mammography, breast MRI remained cost-effective at a WTP-threshold of USD 100,000 per QALY as long as the specificity did not drop below 86.7%.
Simulating various screening intervals and combinations of breast MRI and conventional mammography for the Dutch healthcare system, Geuzinge et al. found breast MRI in 18-month intervals between the ages of 35 and 60 years to be most cost-effective at an ICER of € 21,380 per QALY gained (59).
In a recent review including economic evaluations from 2006-2019, Li et al. proposed precision screening strategies tailored to age and individual risk from an economical perspective (61). Mammography and additional breast MRI were predominantly cost-effective for BRCA1 mutation carriers in middle-age groups, whereas additional breast MRI was not cost-effective for BRCA 2 mutation carriers.
Cost-effectiveness of breast MRI in intermediate-risk screening
MRI screening in women with dense breast tissue, i.e. intermediate risk for breast cancer, has recently demonstrated excellent outcomes. In the DENSE trial, women with extremely dense breast tissue were offered supplemental MRI screening in the Netherlands. The cancer detection rate dropped from 16.5 per 1000 examinations in the first round to 5.8 per 1000 in the second screening round (18, 19). At the same time the false positive rate decreased from 79.8 to 26.3 per 1000 examinations.
Kaiser et al. have first demonstrated the favorable economic value of breast MRI as a screening technique in women with extremely dense breast tissue (62) based on the findings from the first round of the DENSE study (Table 3). Compared to conventional mammography, they calculated an ICER of USD 8,798 per QALY gained for biennial screening with breast MRI.
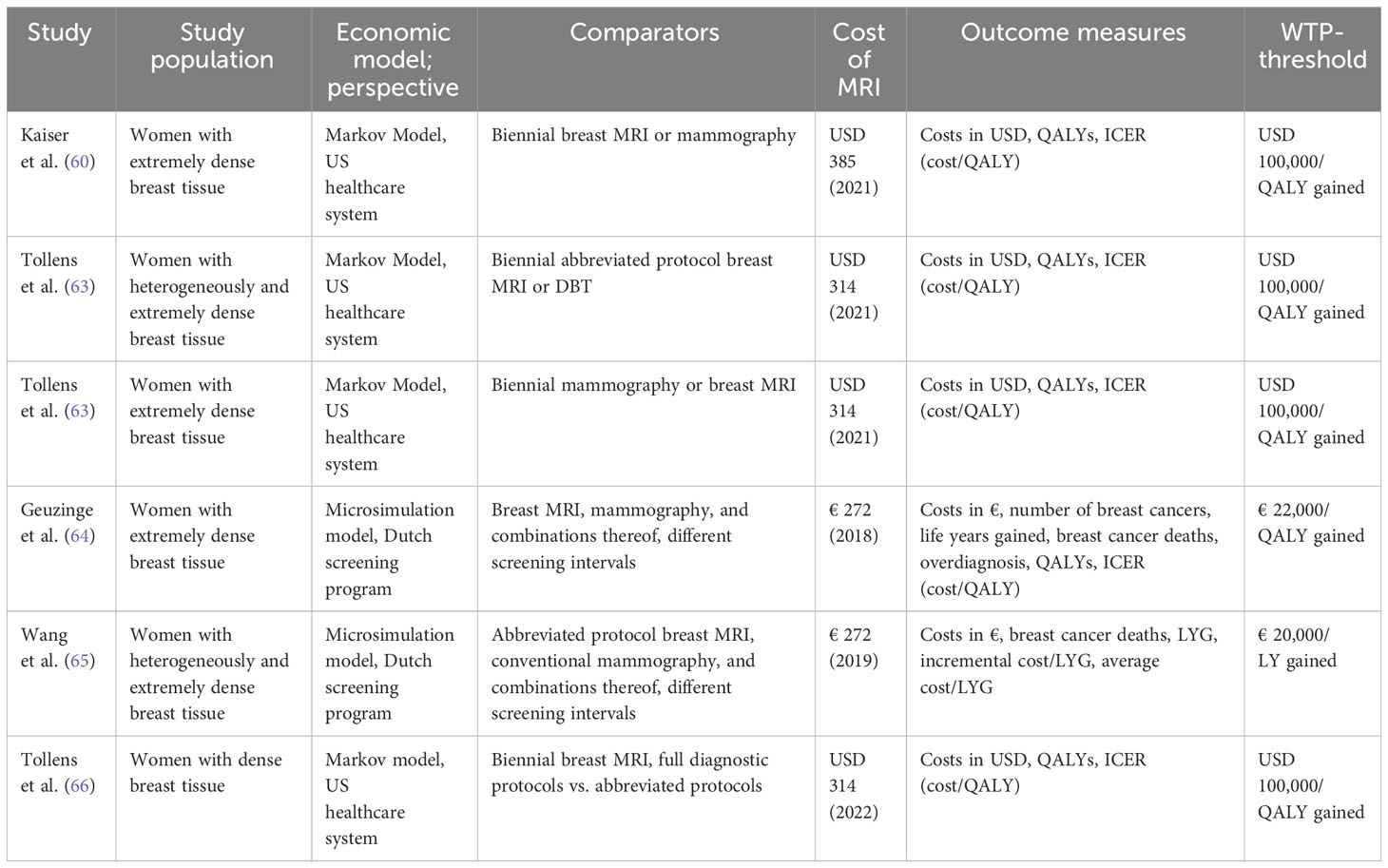
Table 3 Economic evaluations on MRI-based breast cancer screening of women at intermediate risk due to elevated breast tissue density.
Considering the shift in diagnostic performance of breast MRI in the second screening round, i.e. increased specificity of breast MRI reported by the DENSE study group, as well as the reduced cancer detection rate of the second screening round (incidence round) compared to the first round (prevalence round), Tollens et al. confirmed the cost-effectiveness of breast MRI in this patient collective with a further refined Markov-Model (63). When the reduced false positive rate and cancer detection rate from the second screening round are projected on subsequent screening rounds, the reported ICER dropped from USD 38,849 to USD 13,493 per QALY. The authors concluded that the reduced false positive findings and reduced associated follow-up costs outweighed the reduced cancer detection rate from an economic perspective.
Long-term outcomes were also simulated by microsimulation modeling (MISCAN) based on the DENSE trial data and estimated cost-effectiveness of screening women with extremely dense breasts (64). Comparing biennial MRI to biennial mammography would save 8.6 additional lives per 1,000 women invited and cost € 22,500 per QALY gained. In this simulation, MRI screening alone every 4 years saved 7.6 additional lives per 1,000 women at a cost of € 11,500 per QALY gained.
Examining the economic potential of abbreviating MRI protocols for breast cancer screening patients of intermediate risk, evidence on diagnostic performance is scarce. Comparing abbreviated breast MRI to digital breast tomosynthesis (DBT) in women with dense breasts and extremely dense breasts, the EA1411 ECOG-ACRIN study determined a cancer detection rate of 11.8 per 1000 examinations for abbreviated breast-MRI (AB-MRI) and 4.8 per 1000 for DBT (20). No interval cancers were observed. Comstock et al. reported similar levels of sensitivity, yet reduced levels of specificity of AB-MRI.
A simulation of long-term costs and outcomes by Tollens et al. (66) based on the data of Comstock et al. confirmed the cost-effectiveness of AB-MRI in screening women of intermediate risk for breast cancer, including increased false positive findings of abbreviated examinations. As long as the cost of AB-MRI did not exceed 82% of the cost of a full protocol examination, AB-MRI should be considered the cost-effective alternative.
In women with heterogeneously and extremely dense breasts, MRI screening with abbreviated protocols was cost-effective across a wide range of plausible costs per examination when compared to DBT (67). When varying the assumed cost per examination, abbreviated breast MRI was cost-effective below USD 593 and cost-saving below USD 241 compared to DBT.
Wang et al. used the SiMRiSc microsimulation model to compare different screening scenarios including conventional mammography and abbreviated breast MRI in screening women with dense breasts in the Netherlands (65). Costs associated with implementation of a screening program, the involution of breast tissue over time, and radiation-induced tumors were incorporated as well. Biennial MRI screening from 50 - 65 years plus mammography from 66 - 74 years for women with extremely dense breasts was identified as the optimal strategy at an ICER of € 18,201 per life year gained (LYG). Other screening scenarios applying more extensive MRI screening, e.g. biennial MRI from 50 - 74 years, achieved even more LYG and smaller interval cancer rates, yet at an ICER above the predefined WTP-threshold of € 20,000 per LYG.
Cost-effectiveness of breast MRI in average-risk screening
As of today, data on the diagnostic performance of breast MRI in average-risk collectives is limited.
Screening women with average risk of breast cancer with supplemental breast MRI, including women with dense breast tissue, Kuhl et al. found a supplemental cancer detection rate of 15.5 per 1000 cases, with a median size of MRI-detected tumors of 8 mm and no interval cancers in the collective of 2120 women with an observation period of 7007 women-years (68).
Based on these data, a recent cost-benefit analysis (Table 4) simulating screening costs only has indicated that despite higher costs in the short run, triennial MRI screening of women at average risk could be cost-saving compared to annual mammography after 6 years, assuming costs per MRI of USD 400 (69).
Discussion
Indications of MRI-based screening have gradually been extended over the last 20 years (Table 1) along with increasing evidence on improved cancer detection rates of breast MRI in different risk groups (40, 41, 45–47, 70–72).
With evidence on a high specificity of breast MRI in expert hands (12–14), as well as evidence against adverse effects when using repetitive macrocyclic contrast media-enhanced breast MRI for screening (73), financial concerns represent the main obstacle to an increased application of breast MRI in screening women beyond the subgroup of women at high risk. Along with increasing evidence on the safety and efficacy of MRI-based breast cancer screening in women at intermediate risk, the technique has demonstrated to be cost-effective in a variety of indications and conditions that have not yet been implemented in population screening programs. Randomized controlled studies on MRI-based breast cancer screening in women at average risk are unavailable so far.
Major determinants of cost-effectiveness in screening of various risk groups using breast MRI have been identified, with examination costs being identified as the most potent driver of cost-effectiveness. Diagnostic performance, incidence and prevalence rates could be identified as major determinants as well. However, due to heterogeneity of the modeling approaches, they often cannot be quantitatively compared.
Impact of diagnostic performance
While early studies on the diagnostic performance of breast MRI in high-risk screening have indicated lower levels of sensitivity and specificity of 46 - 77% and 81 - 95%, respectively (74–76), the most recent prospective multi-center trials confirmed a largely superior sensitivity of 90 - 93% and a specificity of 89 - 98% (15–17). These shifts in diagnostic performance may be attributable to premature technique as well as initially limited experience with the new technique. At the same time, examination costs have gradually declined over the previous years. Therefore, initial economic evaluations need to be interpreted in the light of their input parameters and assumptions.
While excellent sensitivity is considered a prerequisite for effective screening, generally accomplished by breast MRI (15–17), specificity has been identified as a major determinant for the economic success of MRI screening. This has particular importance for breast cancer screening as positive findings often result in invasive procedures such as biopsies and surgeries, often associated with psychological burden and significant costs.
Quality assurance, benchmarking and performance metrics should be monitored when designing future cost-effective screening programs, since optimal specificity relies on high-quality imaging and image interpretation (77, 78). Multicentric evaluation has shown that different decision algorithms, such as the Kaiser score, can substantially help to improve the specificity of breast MRI (79–81) and compensate for reader experience to some degree (82).
Facets of economic evaluations
From an economical point of view, the costs of setting up a screening program and performing the first screening rounds (prevalence rounds) are initially higher, but decrease over time as initial expenses include training, quality assurance and supervision. The prevalence rounds are known to yield more false positive findings, i.e. more recommendations for biopsies as the stability of equivocal lesions cannot be determined without prior imaging.
In subsequent screening rounds, specificity therefore increases and less false positives are observed (15, 19). As a consequence, the costs of MRI-based screening are higher for women entering screening programs. To capture all economic effects of screening, the stage and nodal status of MRI-detected cancers need to be considered in economic modeling as well as reduced costs for treatment and long-term follow-up. Comprehensive economic evaluations need to account for these short- and long-term effects in order to yield valid conclusions.
Other factors that improve cost-effectiveness of breast MRI are high prevalence and incidence, i.e. higher risk of breast cancer, that can be influenced by further refining the screening population by more sophisticated risk models.
Overdiagnosis
Overdiagnosis refers to the detection of clinically insignificant breast cancer that does not have an impact on a woman’s life expectancy. Concerns on overdiagnosis have been raised after the incidence of invasive breast cancer and ductal carcinoma in situ (DCIS) increased particularly in early mammography screening rounds (9, 83). As the significance of cancer currently cannot be distinguished with any reliability by histology and cannot be identified on an individual level, a number of women potentially receive unnecessary work-up and therapy. However, when adjusting for breast cancer risk and lead time, most plausible estimates of overdiagnosis due to screening mammography range between 1% to 10% (84, 85).
At the same time, underdiagnosis represents a major challenge and many women are underserved by conventional screening as evidenced by breast cancer morbidity and mortality statistics. Underdiagnosis hereby is defined as not detecting a present cancer, i.e. false-negative finding. Notably, MRI preferentially detects more aggressive tumors (22) and may be used to predict the course of disease (86), thereby providing an angle for exploring strategies to escalate or de-escalate treatment.
Modeling overdiagnosis remains a challenge in economic evaluations as evidence on breast MRI screening is limited and accurate numbers are scarce. Several microsimulation studies have incorporated estimates of overdiagnosis (59, 64).
Extrapolation from real-world data
Model-based economic evaluations provide valuable insights by simulating long-term costs and outcomes and by modeling different screening strategies for the purpose of decision analysis. However, the more economic models rely on extrapolations from real-world data, the more the validity of the findings may be limited. The results should therefore be interpreted with caution.
For instance, when various screening intervals are simulated in economic modeling, the outcomes are not directly based on empirical evidence. Economic models should be designed to rely on real-world scientific evidence as much as possible, in order for the results to not represent artificial interrelations depending on the modeling approach. For instance, the longer the screening interval, the lower the costs of screening. If increased interval cancer rates and advanced disease stages of belated diagnoses are not properly accounted for, the resulting ICER may be artificially low for prolonged screening intervals. This could potentially result in an endorsement of longer screening intervals that is not directly based on empirical outcomes, which is why a cautious interpretation is advised (87). Further, prolonged screening intervals may affect attendance rates of screening and women’s’ psychological comfort, which might result in unforeseen but relevant economic effects. Therefore, recommendations on the length of screening intervals should not be derived from economic simulations alone.
Along with the development of breast MRI as a screening technique, the methodology of economic evaluations has evolved as well. While early cost-effectiveness analyses applied various techniques such as Monte Carlo simulations based on spreadsheet programs and statistics software, dedicated software for economic modeling has become state of the art for contemporary cost-effectiveness analyses. Markov Modeling has been established as a robust economic approach in contrast to Microsimulation models (e.g. MISCAN) that have a strength in accurately modeling epidemiological contexts.
Note on abbreviation
Examination costs depend on various healthcare policy factors, including reimbursement schemes and organization as well as the funding of a screening program. Since small cost reductions have a significant impact on the cost-effectiveness of a technique, there have been many attempts to streamline workflows, reduce non-value added time, and to reduce acquisition and image reading times of breast MRI.
Abbreviated breast MRI, i.e. restricting the number of sequences in breast MRI to an essential “abbreviated” limit, has been proposed as a means to reduce the costs of MR-based screening. Although initially defined as solely pre- and post-contrast sequences with subtracted and maximum-intensity projection images (71, 72), a variety of abbreviated protocols have recently been proposed in clinical studies (73–76) that reported varying levels of specificity. So far, however, a standard definition of abbreviated protocols has not been achieved, resulting in a heterogeneous diagnostic landscape, highly individual abbreviation approaches (17, 77) and - consecutively - in varying results regarding economic potential and implications.
While, likely due to small study and patient selection bias, a similar diagnostic performance compared to full diagnostic protocols was reported in the majority of retrospective studies (88), abbreviated breast MRI suffered from reduced specificity in the most recent prospective multi-center trial (20). High-level evidence on the diagnostic performance of different degrees of protocol abbreviation remains scarce. This is why caution is advised when implementing abbreviated protocols.
So far, the cost of abbreviated breast MRI has not been assigned a fixed reimbursement. First experiences of implementing abbreviated breast MRI as a self-played, supplemental screening tool in the U.S. have shown that three examinations per hour may be considered feasible instead of one full diagnostic protocol, with a scan time of less than 10 minutes at USD 250 per examination (89).
At the same time, innovative techniques such as parallel imaging and deep learning-based reconstruction algorithms (90, 91) have reduced examination times of full diagnostic protocols that effectively overlap with the definition of AB-MRI without a detrimental effect on diagnostic performance. For example, a full diagnostic protocol at our institution including T2w imaging, DWI and dynamic contrast enhanced sequences with pre- and 5 post-injection series is acquired in less than 10 minutes (Magnetom Sola, 16 channel coil, Siemens Healthineers), which meets the most common requirements of an abbreviated protocol in terms of acquisition time, yet offers access to the full diagnostic accuracy of breast MRI.
Limitations of economic modeling
Economic evaluations are afflicted with well-known methodological constraints. The technique is based on a utilitarian approach (92). Large effects and benefits are valued more than smaller effects regardless of the affected patients and the actual needs of those patients. For instance, treatment-related health outcomes in young patients with mild chronic conditions may be substantially larger than health outcomes of oncologic patients in end-of-life conditions which may raise ethical concerns on equity and fairness.
Model-based analyses rely on a simplification of complex clinical pathways and heterogenous patient groups that have to be translated into economical models. In reality, adherence to screening recommendations varies and women enter screening programs at different points in time, skip or prolong screening intervals and deviate from therapeutic and diagnostic pathways projected in economic models.
The validity of the modeled costs and effects depends on the quality of input data. However, as high-quality data on costs and outcomes are scarce, applicability to different contexts is limited. Many cost-effectiveness analyses lack calibration of input parameters and external validation and are therefore prone to bias (93).
Conclusion
With increasing evidence on the efficacy and safety of MRI-based breast cancer screening, available cost-effectiveness analyses indicate a strongly favorable economic value compared to conventional screening for a variety of risk groups.
MRI screening is expected to be extended from women with high risk of breast cancer towards women with dense breast tissue. Cost-effectiveness of breast MRI screening in women with dense breast tissue could be demonstrated based on the most recent evidence from prospective multi-center trials. However, further studies are necessary to evaluate the outcomes and cost-effectiveness of screening women at average risk.
Author contributions
FT: Conceptualization, Investigation, Methodology, Visualization, Writing – original draft, Writing – review & editing. PB: Supervision, Writing – review & editing. MF: Methodology, Supervision, Writing – review & editing. CK: Conceptualization, Investigation, Supervision, Validation, Writing – original draft, Writing – review & editing.
Funding
The author(s) declare that no financial support was received for the research, authorship, and/or publication of this article.
Conflict of interest
The authors declare that the research was conducted in the absence of any commercial or financial relationships that could be construed as a potential conflict of interest.
Publisher’s note
All claims expressed in this article are solely those of the authors and do not necessarily represent those of their affiliated organizations, or those of the publisher, the editors and the reviewers. Any product that may be evaluated in this article, or claim that may be made by its manufacturer, is not guaranteed or endorsed by the publisher.
Supplementary material
The Supplementary Material for this article can be found online at: https://www.frontiersin.org/articles/10.3389/fonc.2023.1292268/full#supplementary-material
Abbreviations
AB-MRI, Abbreviated breast MRI; BRCA (gene), Breast cancer (gene); CAD, Canadian dollar; CHEERS, Consolidated Health Economic Evaluation Reporting Standards; DBT, Digital breast tomosynthesis; DCIS, Ductal carcinoma in situ; GDP, Gross domestic product; ICER, Incremental cost-effectiveness ratio; LYG, Life years gained; MISCAN, Microsimulation Screening Analysis; QALY, Quality-adjusted life year; QoL, Quality of life; USD, US-dollar; WTP, Willingness to pay.
References
1. Sung H, Ferlay J, Siegel RL, Laversanne M, Soerjomataram I, Jemal A, et al. Global cancer statistics 2020: GLOBOCAN estimates of incidence and mortality worldwide for 36 cancers in 185 countries. CA Cancer J Clin Mai (2021) 71(3):209–49. doi: 10.3322/caac.21660
2. Torre LA, Siegel RL, Ward EM, Jemal A. Global cancer incidence and mortality rates and trends–an update. Cancer Epidemiol biomark Prev Publ Am Assoc Cancer Res Cosponsored Am Soc Prev Oncol (2016) 25(1):16–27. doi: 10.1158/1055-9965.EPI-15-0578
3. Surveillance, Epidemiology, and End Results (SEER) Program. SEER*Stat Database: Incidence - SEER Research Data, 9 Registries, Nov 2020 Sub (1975-2018) - Linked To County Attributes - Time Dependent (1990-2018) Income/Rurality, 1969-2019 Counties, National Cancer Institute, DCCPS, Surveillance Research Program (2021). Available at: www.seer.cancer.gov.
4. Tabár L, Dean PB, Chen THH, Yen AMF, Chen SLS, Fann JCY, et al. The incidence of fatal breast cancer measures the increased effectiveness of therapy in women participating in mammography screening. Cancer (2019) 125(4):515–23. doi: 10.1002/cncr.31840
5. Puliti D, Zappa M. Breast cancer screening: are we seeing the benefit? BMC Med (2012) 10:106. doi: 10.1186/1741-7015-10-106
6. Coldman A, Phillips N, Wilson C, Decker K, Chiarelli AM, Brisson J, et al. Pan-Canadian study of mammography screening and mortality from breast cancer. J Natl Cancer Inst (2014) 106(11):dju261. doi: 10.1093/jnci/dju261
7. Paci E, EUROSCREEN Working Group. Summary of the evidence of breast cancer service screening outcomes in Europe and first estimate of the benefit and harm balance sheet. J Med Screen (2012) 19 Suppl 1:5–13. doi: 10.1258/jms.2012.012077
8. Autier P, Boniol M, Koechlin A, Pizot C, Boniol M. Effectiveness of and overdiagnosis from mammography screening in the Netherlands: population based study. BMJ (2017) 359:j5224. doi: 10.1136/bmj.j5224
9. Welch HG, Prorok PC, O’Malley AJ, Kramer BS. Breast-cancer tumor size, overdiagnosis, and mammography screening effectiveness. N Engl J Med (2016) 375(15):1438–47. doi: 10.1056/NEJMoa1600249
10. M.Ali RMK, England A, McEntee MF, Mercer CE, Tootell A, Hogg P. Effective lifetime radiation risk for a number of national mammography screening programmes. Radiography (2018) 24(3):240–6. doi: 10.1016/j.radi.2018.02.001
11. Mann RM, Cho N, Moy L. Breast MRI: state of the art. Radiol (2019) 292(3):520–36. doi: 10.1148/radiol.2019182947
12. Benndorf M, Baltzer PAT, Vag T, Gajda M, Runnebaum IB, Kaiser WA. Breast MRI as an adjunct to mammography: Does it really suffer from low specificity? A retrospective analysis stratified by mammographic BI-RADS classes. Acta Radiol Stockh Swed 1987 (2010) 51(7):715–21. doi: 10.3109/02841851.2010.497164
13. Bennani-Baiti B, Bennani-Baiti N, Baltzer PA. Diagnostic performance of breast magnetic resonance imaging in non-calcified equivocal breast findings: results from a systematic review and meta-analysis. PloS One (2016) 11(8):e0160346. doi: 10.1371/journal.pone.0160346
14. Bennani-Baiti B, Baltzer PA. MR imaging for diagnosis of Malignancy in mammographic microcalcifications: A systematic review and meta-analysis. Radiology (2017) 283(3):692–701. doi: 10.1148/radiol.2016161106
15. Riedl CC, Luft N, Bernhart C, Weber M, Bernathova M, Tea MKM, et al. Triple-modality screening trial for familial breast cancer underlines the importance of magnetic resonance imaging and questions the role of mammography and ultrasound regardless of patient mutation status, age, and breast density. J Clin Oncol Off J Am Soc Clin Oncol (2015) 33(10):1128–35. doi: 10.1200/JCO.2014.56.8626
16. Sardanelli F, Podo F, Santoro F, Manoukian S, Bergonzi S, Trecate G, et al. Multicenter surveillance of women at high genetic breast cancer risk using mammography, ultrasonography, and contrast-enhanced magnetic resonance imaging (the high breast cancer risk italian 1 study): final results. Invest Radiol (2011) 46(2):94–105. doi: 10.1097/RLI.0b013e3181f3fcdf
17. Kuhl C, Weigel S, Schrading S, Arand B, Bieling H, König R, et al. Prospective multicenter cohort study to refine management recommendations for women at elevated familial risk of breast cancer: the EVA trial. J Clin Oncol (2010) 28(9):1450–7. doi: 10.1200/JCO.2009.23.0839
18. Bakker MF, de Lange SV, Pijnappel RM, Mann RM, Peeters PHM, Monninkhof EM, et al. Supplemental MRI screening for women with extremely dense breast tissue. N Engl J Med (2019) 381(22):2091–102. doi: 10.1056/NEJMoa1903986
19. Veenhuizen SGA, de Lange SV, Bakker MF, Pijnappel RM, Mann RM, Monninkhof EM, et al. Supplemental breast MRI for women with extremely dense breasts: results of the second screening round of the DENSE trial. Radiology (2021) 299(2):278–86. doi: 10.1148/radiol.2021203633
20. Comstock CE, Gatsonis C, Newstead GM, Snyder BS, Gareen IF, Bergin JT, et al. Comparison of abbreviated breast MRI vs digital breast tomosynthesis for breast cancer detection among women with dense breasts undergoing screening. JAMA (2020) 323(8):746. doi: 10.1001/jama.2020.0572
21. Warner E, Hill K, Causer P, Plewes D, Jong R, Yaffe M, et al. Prospective study of breast cancer incidence in women with a BRCA1 or BRCA2 mutation under surveillance with and without magnetic resonance imaging. J Clin Oncol Off J Am Soc Clin Oncol (2011) 29(13):1664–9. doi: 10.1200/JCO.2009.27.0835
22. Sung JS, Stamler S, Brooks J, Kaplan J, Huang T, Dershaw DD, et al. Breast Cancers Detected at Screening MR Imaging and Mammography in Patients at High Risk: Method of Detection Reflects Tumor Histopathologic Results. Radiology (2016) 280(3):716–22. doi: 10.1148/radiol.2016151419
23. Wilson JMG, Jungner G. Principles and practice of screening for disease. Public health papers 34. Geneva: World Health Organization (1968). Available at: https://apps.who.int/iris/handle/10665/37650.
24. Kadom N, Itri JN, Trofimova A, Otero HJ, Horný M. Cost-effectiveness analysis: an overview of key concepts, recommendations, controversies, and pitfalls. Acad Radiol (2019) 26(4):534–41. doi: 10.1016/j.acra.2018.10.014
25. Iragorri N, Spackman E. Assessing the value of screening tools: reviewing the challenges and opportunities of cost-effectiveness analysis. Health Rev (2018) 39:17. doi: 10.1186/s40985-018-0093-8
26. Schiller-Frühwirth IC, Jahn B, Arvandi M, Siebert U. Cost-effectiveness models in breast cancer screening in the general population: A systematic review. Appl Health Econ Health Policy (2017) 15(3):333–51. doi: 10.1007/s40258-017-0312-3
27. Koleva-Kolarova RG, Zhan Z, Greuter MJW, Feenstra TL, De Bock GH. Simulation models in population breast cancer screening: A systematic review. Breast Edinb Scotl (2015) 24(4):354–63. doi: 10.1016/j.breast.2015.03.013
28. Duong PAT, Bresnahan B, Pastel DA, Sadigh G, Ballard D, Sullivan JC, et al. Value of imaging part I: perspectives for the academic radiologist. Acad Radiol (2016) 23(1):18–22. doi: 10.1016/j.acra.2015.10.006
29. Drummond MF, Sculpher MJ, Claxton K, Stoddart GL, Torrance GW. Methods for the economic evaluation of health care programmes. (Oxford, United Kingdom: Oxford university press) (2015).
30. Bromley HL, Petrie D, Mann GB, Nickson C, Rea D, Roberts TE. Valuing the health states associated with breast cancer screening programmes: A systematic review of economic measures. Soc Sci Med 1982 (2019) 228:142–54. doi: 10.1016/j.socscimed.2019.03.028
31. Briggs A, Sculpher M. An introduction to Markov modelling for economic evaluation. PharmacoEconomics (1998) 13(4):397–409. doi: 10.2165/00019053-199813040-00003
32. Rutter CM, Zaslavsky AM, Feuer EJ. Dynamic microsimulation models for health outcomes: a review. Med Decis Mak Int J Soc Med Decis Mak (2011) 31(1):10–8. doi: 10.1177/0272989X10369005
33. Habbema JD, van Oortmarssen GJ, Lubbe JT, van der Maas PJ. The MISCAN simulation program for the evaluation of screening for disease. Comput Methods Programs Biomed (1985) 20(1):79–93. doi: 10.1016/0169-2607(85)90048-3
34. Cameron D, Ubels J, Norström F. On what basis are medical cost-effectiveness thresholds set? Clashing opinions and an absence of data: a systematic review. Glob Health Action (2018) 11(1):1447828. doi: 10.1080/16549716.2018.1447828
35. McDougall JA, Furnback WE, Wang BCM, Mahlich J. Understanding the global measurement of willingness to pay in health. J Mark Access Health Policy (2020) 8(1):1717030. doi: 10.1080/20016689.2020.1717030
36. Tan-Torres Edejer T, Baltussen R, Adam T, Hutubessy R, Acharya A, Evans DB, et al. Making choices in health: WHO guide to cost-effectiveness analysis. (Geneva: World Health Organization) (2003).
37. Claxton K. Exploring uncertainty in cost-effectiveness analysis. PharmacoEconomics (2008) 26(9):781–98. doi: 10.2165/00019053-200826090-00008
38. Sanders GD, Neumann PJ, Basu A, Brock DW, Feeny D, Krahn M, et al. Recommendations for conduct, methodological practices, and reporting of cost-effectiveness analyses: second panel on cost-effectiveness in health and medicine. JAMA (2016) 316(10):1093–103. doi: 10.1001/jama.2016.12195
39. Husereau D, Drummond M, Augustovski F, de Bekker-Grob E, Briggs AH, Carswell C, et al. Consolidated health economic evaluation reporting standards 2022 (CHEERS 2022) statement: updated reporting guidance for health economic evaluations. Value Health J Int Soc Pharmacoeconomics Outcomes Res (2022) 25(1):3–9. doi: 10.1016/j.jval.2021.11.1351
40. Saslow D, Boetes C, Burke W, Harms S, Leach MO, Lehman CD, et al. American Cancer Society guidelines for breast screening with MRI as an adjunct to mammography. CA Cancer J Clin (2007) 57(2):75–89. doi: 10.3322/canjclin.57.2.75
41. Oeffinger KC, Fontham ETH, Etzioni R, Herzig A, Michaelson JS, Shih YCT, et al. Breast cancer screening for women at average risk: 2015 guideline update from the american cancer society. JAMA (2015) 314(15):1599–614. doi: 10.1001/jama.2015.12783
42. Taneja C, Edelsberg J, Weycker D, Guo A, Oster G, Weinreb J. Cost effectiveness of breast cancer screening with contrast-enhanced MRI in high-risk women. J Am Coll Radiol (2009) 6(3):171–9. doi: 10.1016/j.jacr.2008.10.003
43. Lee JM, McMahon PM, Kong CY, Kopans DB, Ryan PD, Ozanne EM, et al. Cost-effectiveness of breast MR imaging and screen-film mammography for screening BRCA1 gene mutation carriers. Radiology (2010) 254(3):793–800. doi: 10.1148/radiol.09091086
44. Grann VR, Patel PR, Jacobson JS, Warner E, Heitjan DF, Ashby-Thompson M, et al. Comparative effectiveness of screening and prevention strategies among BRCA1/2-affected mutation carriers. Breast Cancer Res Treat (2011) 125(3):837–47. doi: 10.1007/s10549-010-1043-4
45. Sardanelli F, Boetes C, Borisch B, Decker T, Federico M, Gilbert FJ, et al. Magnetic resonance imaging of the breast: recommendations from the EUSOMA working group. Eur J Cancer Oxf Engl 1990 (2010) 46(8):1296–316. doi: 10.1016/j.ejca.2010.02.015
46. Mann RM, Athanasiou A, Baltzer PAT, Camps-Herrero J, Clauser P, Fallenberg EM, et al. Breast cancer screening in women with extremely dense breasts recommendations of the European Society of Breast Imaging (EUSOBI). Eur Radiol (2022) 32(6):4036–45. doi: 10.1007/s00330-022-08617-6
47. Sardanelli F, Aase HS, Álvarez M, Azavedo E, Baarslag HJ, Balleyguier C, et al. Position paper on screening for breast cancer by the European Society of Breast Imaging (EUSOBI) and 30 national breast radiology bodies from Austria, Belgium, Bosnia and Herzegovina, Bulgaria, Croatia, Czech Republic, Denmark, Estonia, Finland, France, Germany, Greece, Hungary, Iceland, Ireland, Italy, Israel, Lithuania, Moldova, The Netherlands, Norway, Poland, Portugal, Romania, Serbia, Slovakia, Spain, Sweden, Switzerland and Turkey. Eur Radiol (2017) 27(7):2737–43. doi: 10.1007/s00330-016-4612-z
48. Plevritis SK, Kurian AW, Sigal BM, Daniel BL, Ikeda DM, Stockdale FE, et al. Cost-effectiveness of screening BRCA1/2 mutation carriers with breast magnetic resonance imaging. JAMA (2006) 295(20):2374–84. doi: 10.1001/jama.295.20.2374
49. Griebsch I, Brown J, Boggis C, Dixon A, Dixon M, Easton D, et al. Cost-effectiveness of screening with contrast enhanced magnetic resonance imaging vs X-ray mammography of women at a high familial risk of breast cancer. Br J Cancer (2006) 95(7):801–10. doi: 10.1038/sj.bjc.6603356
50. Norman RPA, Evans DG, Easton DF, Young KC. The cost-utility of magnetic resonance imaging for breast cancer in BRCA1 mutation carriers aged 30-49. Eur J Health Econ HEPAC Health Econ Prev Care (2007) 8(2):137–44. doi: 10.1007/s10198-007-0042-9
51. Moore SG, Shenoy PJ, Fanucchi L, Tumeh JW, Flowers CR. Cost-effectiveness of MRI compared to mammography for breast cancer screening in a high risk population. BMC Health Serv Res (2009) 9:9. doi: 10.1186/1472-6963-9-9
52. Cott Chubiz JE, Lee JM, Gilmore ME, Kong CY, Lowry KP, Halpern EF, et al. Cost-effectiveness of alternating magnetic resonance imaging and digital mammography screening in BRCA1 and BRCA2 gene mutation carriers. Cancer (2013) 119(6):1266–76. doi: 10.1002/cncr.27864
53. de Bock GH, Vermeulen KM, Jansen L, Oosterwijk JC, Siesling S, Dorrius MD, et al. Which screening strategy should be offered to women with BRCA1 or BRCA2 mutations? A simulation of comparative cost-effectiveness. Br J Cancer (2013) 108(8):1579–86. doi: 10.1038/bjc.2013.149
54. Pataky R, Armstrong L, Chia S, Coldman AJ, Kim-Sing C, McGillivray B, et al. Cost-effectiveness of MRI for breast cancer screening in BRCA1/2 mutation carriers. BMC Cancer (2013) 13:339. doi: 10.1186/1471-2407-13-339
55. Saadatmand S, Tilanus-Linthorst MMA, Rutgers EJT, Hoogerbrugge N, Oosterwijk JC, Tollenaar RAEM, et al. Cost-effectiveness of screening women with familial risk for breast cancer with magnetic resonance imaging. J Natl Cancer Inst (2013) 105(17):1314–21. doi: 10.1093/jnci/djt203
56. Ahern CH, Shih YCT, Dong W, Parmigiani G, Shen Y. Cost-effectiveness of alternative strategies for integrating MRI into breast cancer screening for women at high risk. Br J Cancer (2014) 111(8):1542–51. doi: 10.1038/bjc.2014.458
57. Obdeijn IM, Heijnsdijk EAM, Hunink MGM, Tilanus-Linthorst MMA, de Koning HJ. Mammographic screening in BRCA1 mutation carriers postponed until age 40: Evaluation of benefits, costs and radiation risks using models. Eur J Cancer Oxf Engl 1990 (2016) 63:135–42. doi: 10.1016/j.ejca.2016.05.012
58. Phi XA, Greuter MJW, Obdeijn IM, Oosterwijk JC, Feenstra TL, Houssami N, et al. Should women with a BRCA1/2 mutation aged 60 and older be offered intensified breast cancer screening? - A cost-effectiveness analysis. Breast Edinb Scotl (2019) 45:82–8. doi: 10.1016/j.breast.2019.03.004
59. Geuzinge HA, Obdeijn IM, Rutgers EJT, Saadatmand S, Mann RM, Oosterwijk JC, et al. Cost-effectiveness of breast cancer screening with magnetic resonance imaging for women at familial risk. JAMA Oncol (2020) 6(9):1381–9. doi: 10.1001/jamaoncol.2020.2922
60. Kaiser CG, Dietzel M, Vag T, Rübenthaler J, Froelich MF, Tollens F. Impact of specificity on cost-effectiveness of screening women at high risk of breast cancer with magnetic resonance imaging, mammography and ultrasound. Eur J Radiol (2021) 137:109576. doi: 10.1016/j.ejrad.2021.109576
61. Li J, Jia Z, Zhang M, Liu G, Xing Z, Wang X, et al. Cost-effectiveness analysis of imaging modalities for breast cancer surveillance among BRCA1/2 mutation carriers: A systematic review. Front Oncol (2021) 11:763161. doi: 10.3389/fonc.2021.763161
62. Kaiser CG, Dietzel M, Vag T, Froelich MF. Cost-effectiveness of MR-mammography vs. conventional mammography in screening patients at intermediate risk of breast cancer - A model-based economic evaluation. Eur J Radiol (2020) 136:109355. doi: 10.1016/j.ejrad.2020.109355
63. Tollens F, Baltzer PAT, Dietzel M, Schnitzer ML, Kunz WG, Rink J, et al. Cost-effectiveness of MR-mammography in breast cancer screening of women with extremely dense breasts after two rounds of screening. Front Oncol (2021) 11:724543. doi: 10.3389/fonc.2021.724543
64. Geuzinge HA, Bakker MF, Heijnsdijk EAM, van Ravesteyn NT, Veldhuis WB, Pijnappel RM, et al. Cost-effectiveness of magnetic resonance imaging screening for women with extremely dense breast tissue. J Natl Cancer Inst (2021) 113(11):1476–83. doi: 10.1093/jnci/djab119
65. Wang J, Greuter MJW, Vermeulen KM, Brokken FB, Dorrius MD, Lu W, et al. Cost-effectiveness of abbreviated-protocol MRI screening for women with mammographically dense breasts in a national breast cancer screening program. Breast Edinb Scotl (2022) 61:58–65. doi: 10.1016/j.breast.2021.12.004
66. Tollens F, Baltzer PAT, Dietzel M, Schnitzer ML, Schwarze V, Kunz WG, et al. Economic potential of abbreviated breast MRI for screening women with dense breast tissue for breast cancer. Eur Radiol (2022) 32(11):7409–19. doi: 10.1007/s00330-022-08777-5
67. Tollens F, Baltzer PAT, Dietzel M, Rübenthaler J, Froelich MF, Kaiser CG. Cost-effectiveness of digital breast tomosynthesis vs. Abbreviated breast MRI for screening women with intermediate risk of breast cancer-how low-cost must MRI be? Cancers (2021) 13(6):1241. doi: 10.3390/cancers13061241
68. Kuhl CK, Strobel K, Bieling H, Leutner C, Schild HH, Schrading S. Supplemental breast MR imaging screening of women with average risk of breast cancer. Radiology (2017) 283(2):361–70. doi: 10.1148/radiol.2016161444
69. Mango VL, Goel A, Mema E, Kwak E, Ha R. Breast MRI screening for average-risk women: A monte carlo simulation cost-benefit analysis. J Magn Reson Imaging JMRI (2019) 49(7):e216–21. doi: 10.1002/jmri.26334
70. Monticciolo DL, Newell MS, Moy L, Niell B, Monsees B, Sickles EA. Breast cancer screening in women at higher-than-average risk: recommendations from the ACR. J Am Coll Radiol JACR (2018) 15(3 Pt A):408–14. doi: 10.1016/j.jacr.2017.11.034
71. Monticciolo DL, Newell MS, Hendrick RE, Helvie MA, Moy L, Monsees B, et al. Breast cancer screening for average-risk women: recommendations from the ACR commission on breast imaging. J Am Coll Radiol JACR (2017) 14(9):1137–43. doi: 10.1016/j.jacr.2017.06.001
72. Monticciolo DL, Malak SF, Friedewald SM, Eby PR, Newell MS, Moy L, et al. Breast cancer screening recommendations inclusive of all women at average risk: update from the ACR and society of breast imaging. J Am Coll Radiol JACR (2021) 18(9):1280–8. doi: 10.1016/j.jacr.2021.04.021
73. Bennani-Baiti B, Krug B, Giese D, Hellmich M, Bartsch S, Helbich TH, et al. Evaluation of 3.0-T MRI brain signal after exposure to gadoterate meglumine in women with high breast cancer risk and screening breast MRI. Radiology (2019) 293(3):523–30. doi: 10.1148/radiol.2019190847
74. Leach MO, Boggis CRM, Dixon AK, Easton DF, Eeles RA, Evans DGR, et al. Screening with magnetic resonance imaging and mammography of a UK population at high familial risk of breast cancer: a prospective multicentre cohort study (MARIBS). Lancet Lond Engl (2005) 365(9473):1769–78. doi: 10.1016/S0140-6736(05)66481-1
75. Warner E, Plewes DB, Hill KA, Causer PA, Zubovits JT, Jong RA, et al. Surveillance of BRCA1 and BRCA2 mutation carriers with magnetic resonance imaging, ultrasound, mammography, and clinical breast examination. JAMA (2004) 292(11):1317–25. doi: 10.1001/jama.292.11.1317
76. Kriege M, Brekelmans CTM, Boetes C, Besnard PE, Zonderland HM, Obdeijn IM, et al. Efficacy of MRI and mammography for breast-cancer screening in women with a familial or genetic predisposition. N Engl J Med (2004) 351(5):427–37. doi: 10.1056/NEJMoa031759
77. Kaiser CG, Reich C, Dietzel M, Baltzer P a. T, Krammer J, Wasser K, et al. DCE-MRI of the breast in a stand-alone setting outside a complementary strategy - results of the TK-study. Eur Radiol (2015) 25(6):1793–800. doi: 10.1007/s00330-014-3580-4
78. Pages EB, Millet I, Hoa D, Doyon FC, Taourel P. Undiagnosed breast cancer at MR imaging: analysis of causes. Radiology (2012) 264(1):40–50. doi: 10.1148/radiol.12111917
79. Milos RI, Pipan F, Kalovidouri A, Clauser P, Kapetas P, Bernathova M, et al. The Kaiser score reliably excludes Malignancy in benign contrast-enhancing lesions classified as BI-RADS 4 on breast MRI high-risk screening exams. Eur Radiol (2020) 30(11):6052–61. doi: 10.1007/s00330-020-06945-z
80. Dietzel M, Krug B, Clauser P, Burke C, Hellmich M, Maintz D, et al. A multicentric comparison of apparent diffusion coefficient mapping and the kaiser score in the assessment of breast lesions. Invest Radiol (2021) 56(5):274–82. doi: 10.1097/RLI.0000000000000739
81. Clauser P, Krug B, Bickel H, Dietzel M, Pinker K, Neuhaus VF, et al. Diffusion-weighted imaging allows for downgrading MR BI-RADS 4 lesions in contrast-enhanced MRI of the breast to avoid unnecessary biopsy. Clin Cancer Res Off J Am Assoc Cancer Res (2021) 27(7):1941–8. doi: 10.1158/1078-0432.CCR-20-3037
82. Pötsch N, Korajac A, Stelzer P, Kapetas P, Milos RI, Dietzel M, et al. Breast MRI: does a clinical decision algorithm outweigh reader experience? Eur Radiol (2022) 32(10):6557–64. doi: 10.1007/s00330-022-09015-8
83. Bleyer A, Welch HG. Effect of three decades of screening mammography on breast-cancer incidence. N Engl J Med (2012) 367(21):1998–2005. doi: 10.1056/NEJMoa1206809
84. Puliti D, Duffy SW, Miccinesi G, de Koning H, Lynge E, Zappa M, et al. Overdiagnosis in mammographic screening for breast cancer in Europe: a literature review. J Med Screen (2012) 19 Suppl 1:42–56. doi: 10.1258/jms.2012.012082
85. Monticciolo DL, Helvie MA, Hendrick RE. Current issues in the overdiagnosis and overtreatment of breast cancer. AJR Am J Roentgenol (2018) 210(2):285–91. doi: 10.2214/AJR.17.18629
86. Dietzel M, Schulz-Wendtland R, Ellmann S, Zoubi R, Wenkel E, Hammon M, et al. Automated volumetric radiomic analysis of breast cancer vascularization improves survival prediction in primary breast cancer. Sci Rep (2020) 10(1):3664. doi: 10.1038/s41598-020-60393-9
87. Tollens F, Baltzer PA, Froelich MF, Kaiser CG. Comment on: cost-effectiveness of magnetic resonance imaging screening for women with extremely dense breast tissue. Eur J Radiol (2022) 149:110186. doi: 10.1016/j.ejrad.2022.110186
88. Hernández ML, Osorio S, Florez K, Ospino A, Díaz GM. Abbreviated magnetic resonance imaging in breast cancer: A systematic review of literature. Eur J Radiol Open (2021) 8:100307. doi: 10.1016/j.ejro.2020.100307
89. Marshall HN, Plecha DM. Setting up an abbreviated breast MRI program: our two-year implementation experience. J Breast Imaging (2020) 2(6):603–8. doi: 10.1093/jbi/wbaa060
90. Filli L, Ghafoor S, Kenkel D, Liu W, Weiland E, Andreisek G, et al. Simultaneous multi-slice readout-segmented echo planar imaging for accelerated diffusion-weighted imaging of the breast. Eur J Radiol (2016) 85(1):274–8. doi: 10.1016/j.ejrad.2015.10.009
91. Riffel J, Kannengiesser S, Schoenberg SO, Kaiser AK, Overhoff D, Riffel P, et al. T2-weighted imaging of the breast at 1.5T using simultaneous multi-slice acceleration. Anticancer Res (2021) 41(9):4423–9. doi: 10.21873/anticanres.15249
92. Marseille E, Kahn JG. Utilitarianism and the ethical foundations of cost-effectiveness analysis in resource allocation for global health. Philos Ethics Humanit Med PEHM (2019) 14(1):5. doi: 10.1186/s13010-019-0074-7
Keywords: breast cancer screening, breast MRI, abbreviated breast MRI, MR-mammography, cost-effectiveness analysis, economic evaluation
Citation: Tollens F, Baltzer PAT, Froelich MF and Kaiser CG (2023) Economic evaluation of breast MRI in screening - a systematic review and basic approach to cost-effectiveness analyses. Front. Oncol. 13:1292268. doi: 10.3389/fonc.2023.1292268
Received: 11 September 2023; Accepted: 20 November 2023;
Published: 07 December 2023.
Edited by:
Kevin Ni, St. George Hospital Cancer Care Centre, AustraliaReviewed by:
Umamaheswaran Gurusamy, Nationwide Children’s Hospital, United StatesLaszlo Tabar, Uppsala University, Sweden
Copyright © 2023 Tollens, Baltzer, Froelich and Kaiser. This is an open-access article distributed under the terms of the Creative Commons Attribution License (CC BY). The use, distribution or reproduction in other forums is permitted, provided the original author(s) and the copyright owner(s) are credited and that the original publication in this journal is cited, in accordance with accepted academic practice. No use, distribution or reproduction is permitted which does not comply with these terms.
*Correspondence: Clemens G. Kaiser, Y2xlbWVucy5rYWlzZXJAdW1tLmRl